DOI:
10.1039/C3FO60432B
(Paper)
Food Funct., 2014,
5, 894-899
Grain sorghum muffin reduces glucose and insulin responses in men
Received
20th September 2013
, Accepted 27th January 2014
First published on 7th March 2014
Abstract
Diabetes and obesity have sparked interest in identifying healthy, dietary carbohydrates as functional ingredients for controlling blood glucose and insulin levels. Grain sorghum has been known to be a slowly digestible cereal; however, research is limited on its health effects in humans. The objectives of this study were to measure the contents of functional starch fractions, SDS (slowly-digestible starch) and RS (resistant starch), and to investigate the effects of grain sorghum on postprandial plasma glucose and insulin levels in 10 healthy men. A whole-wheat flour muffin (control) was compared with the grain sorghum muffin with both muffins containing 50 g of total starch. Using a randomized-crossover design, male subjects consumed treatments within a one-week washout period, and glucose and insulin levels were observed at 15 minutes before and 0, 15, 30, 45, 60, 75, 90, 120, 180 minutes after consumption. The mean glucose responses reduced after consuming grain sorghum, particularly at 45–120 minute intervals, and mean insulin responses reduced at 15–90 minute intervals compared to control (P < 0.05). The mean incremental area under the curve (iAUC) was significantly lowered for plasma glucose responses about an average of 35% from 3863 ± 443 to 2871 ± 163 mg (∼3 h) dL−1 (P < 0.05). Insulin responses also reduced significantly from 3029 ± 965 μU (∼3 h) L−1 for wheat to 1357 ± 204 with sorghum (P < 0.05). Results suggest that grain sorghum is a good functional ingredient to assist in managing glucose and insulin levels in healthy individuals.
Introduction
Maintenance of glucose and insulin is essential for the health of both normal and diabetic individuals. Diabetes mellitus has been steadily increasing in the U.S. over the last two decades and 25.8 million people or 8% of the population has been diagnosed with diabetes mellitus.1 Diet is crucial for maintaining a healthy weight and preventing chronic illness such as diabetes. Starches and sugars are targeted to maintain low glucose levels, and conversely, some starch sources can slow the release of glucose because of structures and/or processing methods. Investigating starch digestion rates has helped to identify potential nutritional applications for a variety of starches.2 Starch digestion is commonly referred to as three fractions based on the hydrolysis rates of starch: rapidly-digestible starch (RDS), slowly-digestible starch (SDS), and resistant starch (RS).3
Grain sorghum, Sorghum bicolor L. Moench, is the fifth most produced cereal crop and is grown predominately in the U.S., India, Mexico, Nigeria, and Argentina as reported in 2010 by the FAO.4
Grain sorghum is typically produced and consumed as food stuff in Africa, Asia, and Latin America; in contrast, grain sorghum is mainly used as animal feed in Australia and the U.S.5 However, demand for finding alternative and functional ingredients has prompted expanded use in markets. Similar to other cereal grains, sorghum is mostly composed of starch including the pericarp of the grain, and the starch content may range from 55–76% depending upon crop and cultivar.5–9 Grain sorghum is comparable to maize in terms of composition and the starch structure,5,8–11 however, its protein properties distinguish the grain from other cereals.5–9 The protein matrix has been identified as being one of the key components to its indigestibility; however, processing methods have also shown to increase digestibility.8–10 Research has identified grain sorghum as a potential functional ingredient in food applications,8,12 however, researchers have also reported conflicting results based on sorghum sources and processing conditions.7 Despite conflicting results, studies using whole grain sorghum have shown strong evidence of reduced plasma glucose levels after consumption in both human and animal models.6,12–15
When analyzing grain sorghum, the health benefits associated with consumption have been attributed to the indigestible properties due to its structure and composition. Dietary fiber and tannin content in grain sorghum have been highlighted for nutritional potential,6,8 and additionally, sorghum is also an excellent alternative for gluten-free applications. With increasing demand of both healthier and allergen-free products in the market place, grain sorghum has a great potential for incorporation in numerous food applications as a healthy, dietary carbohydrate and could also potentially assist in the control of glucose and insulin levels in humans. The objective of this study was to investigate the efficacy of grain sorghum flour as a functional food ingredient by measuring the contents of functional starch fractions and the effects on postprandial plasma glucose and insulin responses in healthy humans.
Materials and methods
Materials
Whole grain sorghum flours from Bob's Red Mill (Milwaukie, OR, U.S.A.) and Archer Daniels Midland (Plainview, TX, U.S.A.) and whole wheat flour (Gold Medal, Minneapolis, MN, U.S.A.) were analyzed for starch fractions. All solvents and chemicals for the experiment were purchased from VWR international, Inc. (Suwanee, GA, U.S.A) or Sigma Chemical Co. (St. Louis, Mo., U.S.A). A kit for total starch determination was purchased from Megazyme International Ireland Ltd. (Bray Business Park, Wicklow, Ireland). A kit for plasma insulin determination was purchased from Mercodia (Uppsala, Sweden).
Participant profile and study design
A human study was approved by IRB at University of Arkansas and participants were recruited from University of Arkansas (Fayetteville, AR, U.S.A.). Written informed consent was obtained for all study participants. The study used a randomized-crossover design to investigate glucose and insulin responses. For the study, 10 healthy male subjects were selected after a screening session prior to the experiment to confirm fasting blood glucose levels <100 mg dL−1 in addition to not diagnosed with any disease or illness and not taking any medication within the last 6 months. All subjects were non-smokers and did not frequently consume more than two alcohol servings per week. The mean age, BMI and fasting blood glucose levels of the participant group were 25.1 ± 4 years, 24.2 ± 2.8 kg m−2, and 92.2 ± 6.4 mg dL−1, respectively. Nine participants identified themselves as Asian or Asian American and one participant identified himself as Caucasian. All participants consented to experiment protocols and attended two Saturdays with a one-week washout period. Each subject consumed treatments after 10 hour fasting.
Muffin preparation
After the analyses of grain sorghum flours, the flour high in SDS and RS contents was used for the muffin product. For each experiment, two muffins which contained a total serving of 50 g of total starch were used. All raw materials were weighed separately for each treatment as shown in Table 1. Differences in water amounts were used to accommodate different amounts of flour. Dry ingredients were mixed first followed by wet ingredients, and then both were combined and mixed together. The batter was then weighed into individually greased-muffin liners to weigh approximate product samples. Muffins were baked at 425 °F for 15 minutes. Muffins were cooled for 10 minutes and stored until the following morning of experiment. Batter preparation and baking were conducted consistently each afternoon prior to experiment days. Analysis of total starch (TS) and starch fractions for the final muffin product was also carried out on each experiment day. Each subject consumed two muffins per serving for each treatment on respective experiment days. All ingredients were purchased at a local grocery store.
Table 1 Muffin ingredients
Ingredient |
Wheat muffin (g) |
Sorghum muffin (g) |
Flour |
77.5 |
66.8 |
Water |
46.3 |
37.7 |
Egg |
28.8 |
28.8 |
Butter |
20.0 |
20.0 |
Vegetable oil |
7.5 |
7.5 |
Sucralose |
3.0 |
3.0 |
Baking soda |
1.8 |
1.8 |
Salt |
1.3 |
1.3 |
Vanilla extract |
1.8 |
1.8 |
Total starch content determination
The total starch content of raw material flour samples and finished muffin products was determined following the manual (KOH format). A sample size of 100 mg was dissolved in 2 M KOH in a test tube and neutralized with sodium acetate buffer (1.2 M, pH 3.8) followed by 100 μL of thermostable α-amylase and 100 μL of amyloglucosidase were immediately added. After the incubation period, samples were then diluted to 100 mL and 100 μL of the homogenized sample was added to 3 mL of the glucose determination reagent (GOPOD) and incubated for 20 minutes at 50 °C. After final incubation, the absorbance was immediately read at 510 nm and the content was determined according to the formula included in the manual.
Starch fractions determination
Starch fractions (RDS, SDS, and RS) were determined with the Englyst method3 at the same time when subjects consumed muffins. Enzyme solution was prepared by dispersing 450 mg of pancreatin in 20 mL of deionized water with stirring for 10 minutes, and after centrifugation 54 mL of the supernatant was mixed with 6 mL of amyloglucosidase (140 unit/mL). Muffin samples were ground and weighed in 50 mL centrifuge tubes on the basis of starch content, which were calculated to be the same as 800 mg of flour, and 20 mL of sodium acetate buffer (0.5 M, pH 5.2) was added to the tubes and mixed well. After adding with 5 mL prepared enzyme in each tube, all the tubes were incubated horizontally in a water bath at 37 °C and 160 strokes per min. At 20 min, an aliquot of 0.5 mL was pipetted from the tube and mixed with 20 mL of 80% ethanol for glucose determination (G20). Samples were replaced in the water bath in 30 s. At 120 min, another aliquot of 0.5 mL was pipetted from the tube to 20 mL of 80% ethanol and mixed well for glucose determination (G120). Glucose standard (20 mL of 25 mg mL−1D-glucose in sodium acetate buffer introduced above) and buffer blank (20 mL of sodium acetate buffer) were carried out. A supernatant of 0.1 mL was pipetted into 3 mL of the GOPOD reagent and incubated at 50 °C for 20 minutes. The absorbance was read at 510 nm. The starch fractions were calculated as described in Englyst et al.3
Protein and lipid determination
The crude protein of sorghum and wheat muffins was determined with the micro Kjeldahl method16 using a Kjeltec® 2300 Analyzer (Foss Tecator, Hoganas, Sweden). Muffins were ground and a 0.5 g sample was completely digested in 5 mL concentrated sulfuric acid with a catalyst tablet. The distillation and titration were automatically conducted by the Kjeldahl system. The crude fat in muffins was determined with a Soxtec Avanti 2055 system (Foss North America, MN, U.S.).17 The ground muffin samples were weighed into porous thimbles and extracted in ether, and the lipid was collected in extraction cups. After the evaporation of solvent and the drying process, the lipid was weighed and the crude fat percentage was calculated.
Plasma glucose and insulin analysis
Blood samples were collected at 15 minutes before and 0, 15, 30, 45, 60, 75, 90, 120, and 180 minutes after ingestion. Approximately 0.4 mL blood samples were collected with capillary tubes into a centrifuge tube and centrifuged at 5500 × g with Microfuge® 22R Centrifuge (Beckman Coulter, Inc., Brea, CA, U.S.). Plasma was transferred and stored at −20 °C. Plasma glucose concentrations were measured with an ACE AleraTM Clinical Analyzer (West Caldwell, NJ, U.S.). Plasma insulin concentrations were measured using an insulin ELISA kit from Mercodia (Uppsala, Sweden).
Statistical analysis
All statistical analyses were conducted with the Statistical Analysis System (SAS, Release 9.2, SAS Institute Inc, Cary, NC). Values were expressed as means ± standard error of the mean (SEM). The significance of differences among mean values was determined by analysis of variance (ANOVA). Differences were considered significant at P < 0.05.
Results and discussion
Flour composition and starch analysis
For in vitro analysis, grain sorghum flour contained 22.7% ± 0.8, 43.8% ± 0.8, and 33.5% ± 0.1 for RDS, SDS, and RS, respectively. In comparison, wheat flour contained 37.5% ± 0.3, 47.4% ± 0.4, and 15.1% ± 0.1 for RDS, SDS, and RS measurement (Table 2). Raw grain sorghum flour exhibited significantly lower RDS content and significantly higher SDS and RS contents in comparison to raw wheat flour (P < 0.05). In a study investigating starch digestibility of cereals, researchers reported that unprocessed sorghum contained the highest amount of RS (2–3×) and the lowest amount of RDS (g kg−1 DM) compared with wheat, barley, oat, maize, and rice.18 The present results also demonstrate a similar starch results with grain sorghum exhibiting 2× amount of RS and almost half the amount of RDS. The TS content for the two raw material flours measured 74.9% for grain sorghum flour and 64.5% for wheat flour. Nearly identical grain sorghum TS content was also described in a study conducted by Austin et al.19 for Texas white sorghum of 74% TS and Sudanese grain sorghum flour from Abelgadir et al.11 In the present study, amylose contents of the initial flour material were measured to be 30.9% ± 1.5 for whole grain sorghum flour and 21.8% ± 1.8 for whole wheat flour. Dicko et al.5 reported the sorghum grain containing 60–75 g starch per 100 g fresh matter, consistent with current study data, and the amylose content of 12–22 g per 100 g of fresh matter. Gaffa et al.21 reported two Nigerian sorghum cultivars containing 25.5 and 25.7% amylose content and Sang et al.10 reported 24% amylose content in normal grain sorghum.
Table 2
In vitro total starch and starch fraction measurement of wheat and sorghum flours and muffinsa
Starch composition |
Wheat |
Sorghum |
Flour |
Muffin |
Flour |
Muffin |
TS is represented on dry-basis; RDS, SDS, and SDS percentages are based on TS. Values reflect means ± standard deviation; *, † indicates p < 0.05 significance.
|
TS% |
64.5 ± 0.5 |
43.8 ± 0.3 |
74.9 ± 0.7 |
49.2 ± 0.8 |
RDS% |
37.5 ± 0.2 |
88.2 ± 0.3 |
22.7 ± 0.8* |
78.9 ± 0.5† |
SDS% |
47.4 ± 0.3 |
11.5 ± 0.1 |
43.8 ± 0.8* |
17.5 ± 0.3† |
RS% |
15.1 ± 0.1 |
0.5 ± 0.1 |
33.5 ± 0.1* |
3.6 ± 0.3† |
Muffin composition and starch analysis
The moisture content varied slightly, 35.2% ± 0.3 for sorghum muffins and 39.5% ± 0.3 for wheat muffins. Differences in batter water amounts correspond to moisture sample variance as explained in Materials and methods. Mean crude fat (±SD) contents of sorghum and wheat muffins measured 18.5% ± 0.6 and 16.0% ± 0.8, respectively. Mean crude protein contents were 5.2% ± 0.2 for sorghum muffin and 7.8% ± 0.3 for wheat muffin, and ash contents were nearly identical for both samples with 2.2% ± 0.1 for sorghum and 2.0% ± 0.1 for wheat. Muffin ingredients were added identically between treatments, as listed in Table 1, except for flour and water amounts. Since more wheat flour was needed to provide 50 g of starch for each treatment, differences in protein content can be explained because of the different flour amount used owing to total starch content of wheat and sorghum flour. As Table 1 indicates, approximately 10.7 g more flour was required for wheat muffin treatments. Nutrition label information from material package stated both flour sources as containing identical amounts of protein, fat, sugar, and fiber. Differences in the crude fat and protein contents for final products can be explained by small differences of initial flour (not shown) when compositional analysis was carried out and results given on a dry-weight basis.
In the whole grain sorghum muffin, RDS contents were lower and SDS and RS contents were significantly higher compared with the control whole wheat muffin (P < 0.05) (Table 2). The starch structure, particularly protein–starch interactions, may explain differences of starch digestion rates for treatments based on past research15,19–23 or perhaps amylose content. In a study conducted by Sang et al.,10 raw isolated grain sorghum starches were investigated for in vitro starch digestibility with varying amylose contents, and authors found that the intermediate (14.0%) amylose content of heterowaxy starch exhibited higher RS content rather than the amylose content of normal (23.7%) starch. The heterowaxy starch with an intermediate amylose content exhibited slightly lower SDS yet the highest RS content, suggesting that amylose content is not necessarily responsible for predicting in vitro digestibility. Researchers also reported similar digestibility results for RDS and RS contents compared to grain sorghum flour used in the present study. The RDS content (21.5%) of waxy grain sorghum starch and RS content (23.7%) of heterowaxy grain sorghum starch are comparable to present materials analysis.10 In contrast, normal, heterowaxy, and waxy raw sorghum starch samples exhibited higher amounts of SDS content (61.7–68.5%), and lower amounts of amylose 23.7, 14, and 0%, respectively. Findings from Benmoussa et al.24 also confirm variations in starch digestibility for three sorghum cultivars, suggesting that amylose content does not necessarily indicate the digestibility rate. Authors investigated different sorghum genotypes with ranging amylose content (19.2–22.4%) and found that sample starch digestibility was mostly influenced by starch granule characteristics such as channel density which allows enzymes to attack the granule and the sorghum protein matrix, depending upon the sorghum genotype, also influences enzyme accessibility to granule.24 The protein content of the final product given to participants in the present study was relatively low; however, research has highlighted the protein–starch relationship in grain sorghum and its influence on starch digestion. In an article by Duodu et al.,25 it has been discussed that exogenous factors such as the grain organizational structure, polyphenols, phytic acid, cell wall components, and starch are primary factors that influence protein digestibility, and additional researchers also confirmed26–29 results which explain the coordinated relationship between sorghum starch and protein digestibility. As researchers have discovered that both starch granules and protein bodies are closely associated with each other; enzymatic accessibility is greatly hindered for starch gelatinization and digestibility, which may contribute to poor protein quality of sorghum.25 In a more recent publication from Mokrane et al.26 regarding the protein, Algerian sorghum cultivars were only evaluated for protein digestibility. Researchers found that the protein content in cultivars could provide good quantity and quality of essential amino acids though the digestibility varied greatly between cultivars and unfortunately starch digestion was not analyzed. The Algerian sorghum flours contained 12.6–16.4% crude protein (DB), approximately 4–8% higher than grain sorghum flour used in the present study.
Incremental glucose and insulin results
Fig. 1 and 2 show incremental changes of plasma glucose and plasma insulin concentrations over experiment duration. With the grain sorghum muffin treatment, the glucose responses from participants were significantly lower at 45, 60, 75, 90, and 120 minute intervals (P < 0.05).
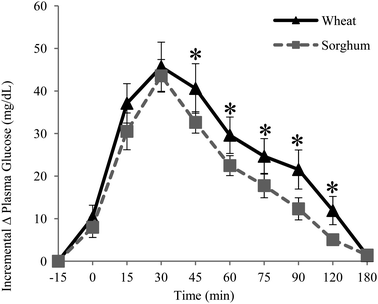 |
| Fig. 1 Mean incremental change of plasma glucose in healthy men (n = 10) with standard error of mean bars (SEM). * p < 0.05 at time intervals. | |
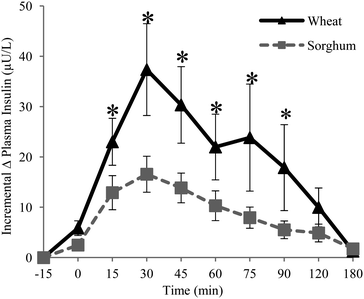 |
| Fig. 2 Mean incremental change of plasma insulin in healthy men (n = 10) with standard error of mean bars (SEM). * p < 0.05 at time intervals. | |
Mean iAUC responses of glucose were significantly reduced about 26% from 3863 ± 443 to 2871 ± 163 mg (∼3 h) dL−1 as shown in Fig. 3 (P < 0.05). Incremental insulin concentrations for the sorghum muffin treatment were significantly lower at 15, 30, 45, 60, 75, and 90 minutes (P < 0.05) and were also significantly reduced about 55% from 3029 ± 965 to 1357 ± 204 μU (∼3 h) L−1 (Fig. 3).
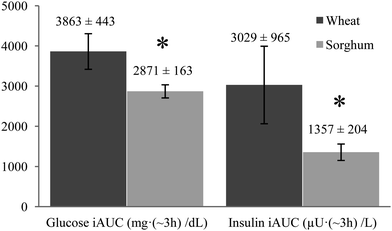 |
| Fig. 3 Total mean incremental area under the curves (iAUC) for plasma glucose and insulin responses of healthy males (n = 10) with standard error of mean (SEM) values. * p < 0.05 significance. | |
Processing and cooking conditions do influence starch hydrolysis.12,30 In one human study conducted by Lakshmi and Vimala,12 the study observed glucose responses from six non-insulin dependent diabetic patients, and researchers compared the three sorghum recipes, both with whole grain and dehulled grains, and also compared to either wheat or rice recipe controls, additionally. Recipes were traditional dietary foods in different preparation forms including a shallow pan-fried product, boiled, and a fermented-steamed product. Researchers used larger treatment samples (85–90 g carbohydrate), but with all three samples, and whole sorghum food recipes exhibited lowest plasma glucose and insulin responses compared to identical dehulled sorghum recipes and controls. In addition, whole grain sorghum foods showed an average of two-fold amount of fiber content compared to dehulled sorghum foods. Researchers investigating Sudanese cereal foods and their glucose and insulin responses have reported wheat and millet as having slightly lower glucose and insulin incremental areas under the curve compared to sorghum,11 however, flours used for the study did not provide total starch content for various treatment grains and forms to confirm identical treatment conditions. With the literature available, it is evident that processing conditions such as de-hulling may greatly influence overall glucose and insulin responses as reported by Taylor and Emmambux,6 so it is important for human studies to test whole grain sorghum for potential functional food ingredients. Cultivars bred for functional starch properties would be excellent candidates for functional food ingredients, however, the preparation, processing, and cooking conditions of the final product remain central to evaluate effectiveness.
Conclusions
Results show whole grain sorghum as a good functional food ingredient for controlling glucose and insulin levels in healthy humans. Compared to wheat, functional starch fractions for sorghum showed increased SDS and RS contents and lower RDS content and decreased glucose and insulin responses in healthy humans. Research implies further investigation with pre-diabetic or diabetic individuals would be beneficial for providing more additional analysis of grain sorghum as a functional ingredient. Additionally, future research may include sensory evaluation for the ingredient profile and functionality. With increasing gluten-intolerance and environmental concerns, grain sorghum will continue to be utilized for future research applications.
Acknowledgements
Support for this research from the Arkansas Grain Sorghum Promotion Board is gratefully appreciated.
References
-
Centers for Disease Control and Prevention, National diabetes fact sheet: national estimates and general information on diabetes and prediabetes in the United States, U.S. Department of Health and Human Services, Centers for Disease Control and Prevention, Atlanta, GA, 2011 Search PubMed.
- G. Annison and D. L. Topping, Annu. Rev. Nutr., 1994, 14, 297–320 CrossRef CAS PubMed.
- H. N. Englyst, S. M. Kingman and J. H. Cummings, Eur. J. Clin. Nutr., 1992, 46(2), s33–s50 Search PubMed.
-
Food and Agriculture Organization (FAO), FAO Statistics of Sorghum Crop Production, 2010 Search PubMed.
- M. H. Dicko, H. Gruppen, A. S. Traoré, A. G. J. Voragen and W. J. H. van Berkel, Afr. J. Biotechnol., 2006, 5(5), 384–395 CAS.
- J. R. N. Taylor and M. N. Emmambux, Molecular Diversity and Health Benefits of Carbohydrates from Cereals and Pulses, 2010, 87(4), 263–271 CAS.
- M. J. Gidley, P. A. Pluschke, P. A. Sopade, G. J. S. Al-Rabadi, A. Sultan, C. Y. Gan, X. Li, D. Zhang and W. L. Bryden, Australian Poultry Science Symposium, 2011, 22, 139–146 Search PubMed.
- R. N. Taylor, T. J. Schober and S. R. Bean, J. Cereal Sci., 2006, 44, 252–271 CrossRef PubMed.
- L. W. Rooney and R. L. Pflugfelder, J. Anim. Sci., 1986, 63, 1607–1623 CAS.
- Y. Sang, S. Bean, P. A. Seib, J. Pedersen and Y. C. Shi, J. Agric. Food Chem., 2008, 56, 6680–6685 CrossRef CAS PubMed.
- M. Abdelgadir, M. Abbas, A. Jarvi, M. Elbagir, M. Elton and C. Berne, Diabetic Med., 2005, 22, 213–217 CrossRef CAS PubMed.
- K. B. Lakshmi and V. Vimala, J. Nutr., 1996, 16, 1651–1658 Search PubMed.
- A. C. Carciofi, F. S. Takakura, L. D. De-Oliverira, E. Teshima, J. T. Jeremais, M. A. Brunetto and F. Prada, J. Anim. Physiol. Anim. Nutr., 2008, 92, 326–336 CrossRef CAS PubMed.
- L. D. De-Oliveira, A. C. Carciofi, M. C. C. Oliveira, R. S. Vascocellos, R. S. Bazolli, G. T. Pereira and F. Prada, J. Anim. Sci., 2008, 86, 2237–2246 CrossRef CAS PubMed.
- J. H. Wong, T. Lau, N. Cai, J. Singh, J. F. Pedersen, W. H. Vensel, W. J. Hurkman, J. D. Wilson, P. G. Lemaux and B. B. Buchanan, J. Cereal Sci., 2009, 49, 73–82 CrossRef CAS PubMed.
- American Association of Cereal Chemists (AACC) international, Approved Methods of Analysis, 10th edn. Method 46-13. Crude Protein – Micro-Kjeldahl Method. Method 30–10. Crude Fat in Flour, Bread, and Baked Cereal Products Not Containing Fruit.
- A. M. AwadElkareem and J. R. N. Taylor, Cereal Chem., 2012, 88(4), 334–348 Search PubMed.
- G. Giuberti, A. Gallo, C. Cerioli and F. Masoero, Anim. Feed Sci. Technol., 2012, 174, 163–173 CrossRef CAS PubMed.
- D. L. Austin, N. D. Turner, C. M. McDonough and L. W. Rooney, Cereal Chem., 2012, 89(4), 190–197 CrossRef CAS.
-
S. Serna-Saldivar and L. W. Rooney, Sorghum and Millets: Chemistry and Technology, ed. D. A. V. Dendy, AACC International, St. Paul, MN, 1995, pp. 69–124 Search PubMed.
- T. Gaffa, Y. Yoshimoto, I. Hanashiro, O. Honda, S. Kawasaki and Y. Takeda, Cereal Chem., 2004, 80(2), 255–260 CrossRef.
- G. Zhang and B. R. Hamaker, Cereal Chem., 1998, 75, 710–713 CrossRef CAS.
- U. Lehmann and F. Robin, Trends Food Sci. Technol., 2007, 18(7), 346–355 CrossRef CAS PubMed.
- M. Benmoussa, B. Suhendra, A. Aboubacar and B. R. Hamaker, Starch/Staerke, 2006, 58(2), 92–99 CrossRef CAS.
- K. G. Duodu, J. R. N. Taylor, P. S. Belton and B. R. Hamaker, J. Cereal Sci., 2003, 38(2), 117–132 CrossRef CAS.
- H. Mokrane, H. Amoura, N. Belhaneche-Bensemra, C. M. Courtin, J. A. Delcour and B. Nadjemi, Food Chem., 2010, 121(3), 719–723 CrossRef CAS PubMed.
- L. I. Ezeogu, K. G. Duodu and J. R. N. Taylor, J. Cereal Sci., 2005, 42(1), 33–44 CrossRef CAS PubMed.
- P. Rooney, J. Agric. Food Chem., 1986, 63, 1607–1623 Search PubMed.
- G. Zhang and B. R. Hamaker, Cereal Chem., 2005, 82, 534–540 CrossRef CAS.
- N. R. Dlamini, J. R. N. Taylor and L. W. Rooney, Food Chem., 2007, 105, 1412–1419 CrossRef CAS PubMed.
|
This journal is © The Royal Society of Chemistry 2014 |