DOI:
10.1039/C3FO60370A
(Review Article)
Food Funct., 2014,
5, 12-17
Chemopreventative effects of tetrahydrocurcumin on human diseases
Received
29th August 2013
, Accepted 6th October 2013
First published on 7th October 2013
Abstract
Chemoprevention is a relatively new and promising strategy to prevent human degenerative diseases, including cancer, and is defined as the use of natural dietary compounds and/or synthetic substances to block, inhibit, reverse, or retard the progress of human diseases. Tetrahydrocurcumin (THC) is a major metabolite of curcumin (extracted from the roots of the Curcuma longa Linn). THC has been demonstrated to prevent oxidative stress and inflammation, to act against neurodegeneration, and to possess anti-cancer activity. In this review, we summarize the current knowledge and underlying molecular mechanisms of the chemopreventative activities of THC and its potential effects on the development of various human diseases.
1. Introduction
In recent years, natural dietary compounds have become a focus for disease prevention and treatment. Orally administered dietary compounds are absorbed and metabolized in the stomach and intestine. Therefore, the key considerations for treatment with these compounds are bioavailability and drug efficiency of the dietary compound or its metabolites.1 Curcumin (CUR), a bis-α,β-unsaturated β-diketone polyphenol obtained from the rhizome (turmeric) of the herb Curcuma longa is widely used as a yellow coloring agent and spice in foods. CUR has been shown to possess anti-inflammatory properties, anti-cancer activity and to prevent Alzheimer's disease (AD).2–4 The safety, bioavailability and efficiency of CUR have been investigated in a number of clinical trials.5–7 However, CUR is rapidly metabolized by reductase and enteric microflora in the liver and intestine, respectively.
In 1978, Holder et al. first identified tetrahydrocurcumin (THC; 1,7-bis(4-hydroxy-3-methoxyphenyl)heptane-3,5-dione) as one of the major colorless metabolites of CUR.8 THC has been shown to exhibit protective physiological and pharmacological properties similar to curcumin, such as anti-oxidant, radical-scavenging, anti-metastatic, and anti-carcinogenic activities.9–13 Additionally, THC exhibited increased activity compared to CUR in protecting against chloroquine-induced oxidative kidney damage, suppressing azoxymethane (AOM)-induced colon carcinogenesis, and extending the life span of Drosophila sp.9,14,15 Several studies have suggested that THC shows a greater number of bioactive functions that are beneficial to human health than CUR. Additionally, for the dietary administration of CUR or THC, the amounts of THC and its conjugates (as sulfates and glucuronides) were larger than those of CUR and its conjugates in the liver and serum. Hence, THC is more easily absorbed from the gastrointestinal tract than CUR.16 Moreover, Sermkaew et al. used self-microemulsifying drug delivery systems (SMEDDS) to improve the solubility and enhance the absorption of THC.17 In this review, we discuss the current knowledge regarding the chemopreventative effects of THC on human associated diseases (Fig. 1).
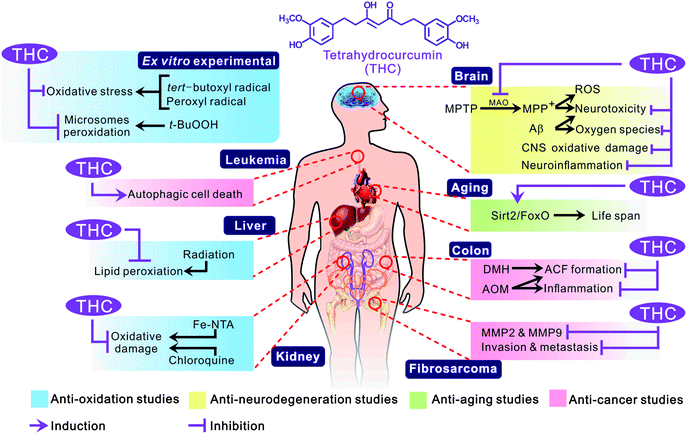 |
| Fig. 1 Schematic representation of THC regulative activity on human associated diseases. | |
2. Anti-oxidant effects of tetrahydrocurcumin
Oxidative stress is induced by free radicals, including oxygen free radicals, reactive oxygen species (ROS), and reactive nitrogen species (RNS), which are products of normal cellular metabolism.18 Free radicals are reactive chemical species that have a single unpaired electron in the outer orbit, leading to the nonspecific modification of lipids, proteins, and nucleic acids, which can contribute to the initiation of various diseases, including aging, diabetes, neurodegeneration, and cancer.19,20
The structure of THC includes a phenol and a β-diketone functional group, which is a common structural feature found on antioxidative molecules. In 1996, Sugiyama et al. found that THC generated four oxidation products derived from β-diketone after being incubated with peroxyl radicals.13 These results suggest that the β-diketone structure plays an important role in the antioxidative activity of THC because C–C bond cleavage was observed in the β-diketone moiety during the antioxidative process.13
Several different in vitro studies have demonstrated that THC scavenges free radicals and possesses antioxidative activity. In 1995, Osawa et al. suggested that THC inhibited the auto-oxidation of linoleic acid and the peroxidation of t-BuOOH-induced microsomes, which led to a proposed mechanism for the antioxidative activity of THC.21 In animal liver and intestine, CUR is metabolized into THC, hexahydrocurcumin (HHC) and octahydrocurcumin (OHC).22,23 Using the 2,2-diphenyl-1-picrylhydrazyl (DPPH) scavenging activity assay, the following order was observed for the scavenging activity of these metabolites: THC > HHC = OHC.24 Similarly, THC exhibited the greatest inhibition of 2,2′-azobis (2-amidinopropane)dihydrochloride (AAPH) activity and radiation-induced oxidation.24,25 THC showed greater antioxidative activity than CUR in in vivo studies. Naito et al. found that the addition of THC (5000 ppm) to the diet significantly inhibited the generation of an early peroxidation product, Nε-(hexanoyl) lysine (HEL), in the liver tissue of cholesterol-fed rabbits.26 In a kidney oxidative damage model, rats were fed chloroquine, which resulted in increased lipid peroxidation and decreased levels of enzymatic and non-enzymatic antioxidants in vivo.27,28 However, the oral administration of THC (80 mg/kg body weight) significantly prevented chloroquine-induced renal oxidative damage in Wistar rats.14Nω-nitro-L-arginine methyl ester (L-NAME) is a well-known hypertension inducer, and it induces oxidative stress in vivo through increased superoxide (O2−) and hepatic malondialdehyde (MDA) levels and reduced plasma superoxide dismutase (SOD) activity.29 In a L-NAME-induced rat model, a diet containing THC significantly suppressed O2− and MDA formation and increased endogenous reduced glutathione (GSH) production.30 Additionally, L-NAME-induced aortic wall thickness and stiffness caused hypertension was significantly reversed by THC.31
Oxidative stress has been proposed as a possible mechanism for the initiation of several human diseases. Because THC demonstrates strong antioxidative activity in different in vitro and in vivo models, it may have the potential to prevent oxidation-associated human diseases.
3. Chemoprevention of neurodegenerative disorders by tetrahydrocurcumin
In recent years, there has been an emphasis on identifying natural dietary compounds that can reduce amyloid-β (Aβ)-induced neurotoxicity and neurodegenerative disorders, such as Parkinson's disease (PD) and AD.32–34 Parkinson's disease is a neurodegenerative disorder associated with aging and is most prevalent in individuals over the age of 85.35 With age, dopamine (DA) is rapidly metabolized into 3,4-dihydroxyphenylacetaldehyde (DOPAL) by monoamine oxidase (MAO) in intra-neuronal spaces.36 DOPAL damages the dopaminergic neurons and contributes to the loss of DA-containing terminals, both characteristics of PD.36,37 DOPAL-induced neurotoxicity may be a result of protein cross-linking, the oxidative reactivity of quinones and the production of hydroxyl radicals.38–40
In 1982, several young adults in California mysteriously developed Parkinson's-like symptoms after an intravenous dose of synthetic heroin that contained a major toxin, 1-methyl-4-phenyl-1,2,3,6-tetrahydropyridine (MPTP).41 MPTP, a synthetic compound, is a mimetic used to induce Parkinson's-like symptoms, and its activity targets the nigrostriatal system.42 When MPTP is injected into animals, it enters the brain through the blood brain barrier (BBB) within minutes, and it is metabolized by MAO to 1-methyl-4-phenylpyridinium (MPP+), which can selectively cross into dopaminergic neurons via the dopamine transporter (DAT). It then accumulates in the mitochondria,43–45 where it causes toxicity through the induction of ROS, resulting in neuronal cell death.46,47 Rajeswari and Sabesan have demonstrated that Swiss albino mice treated intraperitoneally with MPTP show a significant DA degradation compared with a control group. In an MPTP-induced Parkinson's model, THC increased the levels of DA in mice after MPTP treatment through the inhibition of MAO-B activity.48 Although, the addition of THC led to a recovery of the DA levels and inhibition of MAO-B activity in MPTP treated mice, the mechanism of action was not clear. THC may have the potential to be used as a therapeutic for the prevention or treatment of PD.
AD is characterized by tau hyperphosphorylation, oxidative stress, neurotoxicity, neuroinflammation, and the accumulation of amyloid plaques.49 Amyloid plaques are generated from Aβ aggregation and affect intracellular signal transduction, induce oxidative stress, and cause neurotoxicity.50,51 Mishra et al. have shown that THC reduces Aβ-induced neurotoxicity by regenerating the mitochondrial membrane potential and inhibiting ROS generation and caspase-3 activity in rat hippocampal and human neuronal cells.32 In in vivo studies, THC significantly reduced neuroinflammation through the inhibition of inducible nitric oxide synthase (iNOS) and interleukin (IL)-1β mRNA expression, the EC50 values were 0.701 and 1.286 μM in LPS-induced C57BL6/J mouse brains, respectively. Furthermore, this study used a Tg2576 transgenic mouse model, in which aggregation of Aβ spontaneously occurs in cortical and limbic brain regions at approximately 9 months of age.52 This study demonstrated that THC does not affect Aβ plaque accumulation; it significantly reduced 75% of soluble Aβ, which is a more cytotoxic species than Aβ fiber and causes AD.53,54 These observations suggest that THC may have beneficial effects on neurodegenerative diseases like PD and AD.
4. Chemoprevention of aging by tetrahydrocurcumin
Aging is an important biological process that manifests as a decline in performance and fitness with advancing years. For most species, the aging process is a progressive accumulation of damage, loss of the physiological functions of cells and organs, and reduced physical activity. The radical theory of aging is a proposed mechanism that was advocated by Harman.55 This theory hypothesizes that ROS generation and increased oxidative stress lead to progressive macromolecular oxidative damage and dysfunction, which induces aging and age-related diseases.56 Recently, studies have shown that several signal transduction pathways are involved in the aging process, such as the mammalian target of rapamycin (mTOR) pathway, insulin/insulin-like growth factor (IGF) and Forkhead box O (FOXO) signaling,57,58 which is made up of a large family of transcription factors.59 FOXO is activated by silent information regulator 2 (Sir2), which is a key factor in the regulation of the relationship between oxidative stress and life span.60 In 2007, Kitani et al. demonstrated that THC could have the potential to beneficially modify animal life spans. This study used C57BL/6 mice and demonstrated that the dietary administration of 0.2% THC increased the life span of the mice by 11.7% over the control group.61 However, the mechanism is still not clear. In 2011, Xiang et al.15 suggested that signal transduction by Sirt2/FOXO may be a key mechanistic pathway in THC-induced life span increases. This hypothesis is supported by the finding that THC extends the life span of wild-type Drosophila, and this effect is lost when Foxo or Sirt2 deleted strains are used.
5. Cancer chemoprevention by tetrahydrocurcumin
Cancer development is a long-term process that includes many complex factors. There is a stepwise progression that involves four stages: initiation, promotion, progression, and metastasis.62,63 Metastasis, the final stage, is defined as an uncontrolled spreading and growth of cancerous cells throughout the body. Metastasis is an important stage that affects the mortality of patients with cancer. Currently, there are several well-known factors that influence cancer development, including lifestyle, oxidative stress, DNA damage or mutation, inflammation and exposure to carcinogens.64,65 Several different in vitro and in vivo studies have demonstrated that THC possesses anti-cancer activity, and it has proven effective in reducing colorectal carcinogenesis, increasing leukemia cell death, and inhibiting fibrosarcoma metastasis.9,66–68 However, the use of THC against 12-O-tetradecanoylphorbol-13-acetate (TPA)-induced skin tumors only resulted in weak anti-cancer activity.69 When used to treat 1,2-dimethylhydrazine (DMH)-induced colorectal carcinogenesis, THC was reported to significantly decrease the amount of aberrant crypt foci (ACF) formation through the inhibition of cell proliferation, as measured by 5-bromo-2′-deoxyuridine (BrdU) incorporation.68 Additionally, we have observed similar results in our studies of THC reduced ACF and polyp formation through a reduction of inflammatory enzymes and the down regulation of the Wnt/β-catenin signaling pathway, which is an important signaling pathway required to induce colorectal cancer proliferation.9,70 A number of studies have suggested that the biological activity of THC is similar to that of CUR. We first found that THC induced autophagic cell death, also known as type II programmed cell death, in human leukemia HL-60 cells, but CUR did not.67 Recent studies suggest that CUR may be able to induce autophagic cell death in different cell types and under different conditions.71,72 Additionally, Yodkeeree et al. have suggested that THC exhibits anti-metastatic activity through reducing the levels of matrix metalloproteinase (MMP)-2 and MMP-9 and that it inhibits cell invasion and migration by human fibrosarcoma HT1080 cells.66 Taken together, these studies have demonstrated that THC may have potential as a cancer chemopreventative agent.
6. Conclusion
Although human diseases are the result of complicated processes, the clarification and understanding of the causes, signaling networks and molecular mechanisms involved in disease are critical for finding applicable and effective approaches to chemoprevention. THC has exhibited many beneficial effects, including the regulation of oxidative stress, the prevention of neurodegenerative disorders, life span extension, and the inhibition of carcinogenesis and malignity (Table 1). Regulation of these processes by THC provides a potential mechanism to prevent or treat human diseases. However, THC has yet to be investigated clinically.
Table 1 Chemopreventative mechanisms of THC on human associated diseases
Disease |
Targeted functions |
Ref. |
Oxidative damage |
THC scavenged tert-butoxyl radicals and peroxyl radicals |
13
|
THC inhibited linoleic acid auto-oxidation and t-BuOOH-induced microsome peroxidation |
21
|
THC inhibited linoleic acid peroxidation and reduced free radical-induced red blood cell hemolysis |
24
|
THC inhibited radiation-induced lipid peroxidation |
25
|
THC protected against Fe-NTA-induced oxidative renal damage in ddY mice |
16
|
THC decreased liver TBARS formation and LDL oxidation in cholesterol-fed NZW rabbits |
26
|
THC protected against chloroquine-induced kidney oxidative damage in Wistar rats |
14
|
THC reduced the L-NAME-induced superoxide production, oxidative stress, and increased GSH in SD rats |
30
|
Neurodegenerative disorder |
THC inhibited MPTP-induced monoamine oxidase activity and neurotoxicity in Swiss albino mice |
48
|
THC decreased Aβ-induced oxygen species, caspase activity, and neurotoxicity in hippocampal cells and neural progenitor cells |
32
|
THC reduced CNS oxidative damage, neuroinflammation, and soluble Aβ |
54
|
Aging |
C57BL/6 mice administered 0.2% THC in their diet showed increases in lifespan by 11.7% |
61
|
The life span of several species of Drosophila that were fed THC increased between 1 to10 days |
15
|
Cancer |
THC decreased DMH-induced ACF formation and cell proliferation in B6C3F1 mice |
68
|
THC inhibited migration and invasion of human fibrosarcoma cells by downregulation of MMPs and uPA in HT1080 cells |
66
|
THC induced autophagic cell death through modulation of PI3K/Akt-mTOR and MAPK signaling pathway in human leukemia cells |
67
|
THC decreased AOM-induced ACF formation and pro-inflammatory enzymes in ICR mice |
9
|
Abbreviations
AAPH | 2,2′-Azobis (2-amidinopropane)dihydrochloride |
ACF | Aberrant crypt foci |
AD | Alzheimer's disease |
Aβ | Amyloid-β |
AOM | Azoxymethane |
BBB | Blood brain barrier |
BrdU | 5-Bromo-2′-deoxyuridine |
CUR | Curcumin |
DA | Dopamine |
DAT | Dopamine transporter |
DMH | 1,2-Dimethylhydrazine |
DOPAL | 3,4Dihydroxyphenylacetaldehyde |
DPPH | 2,2-Diphenyl-1-picrylhydrazyl |
FOXO | Forkhead box O |
GSH | Glutathione |
HHC | Hexahydrocurcumin |
iNOS | Inducible nitric oxide synthase |
IGF | Insulin-like growth factor |
IL | Interleukin |
MDA | Malondialdehyde |
mTOR | Mammalian target of rapamycin |
MMP | Matrix metalloproteinase |
MAO | Monoamine oxidase |
HEL |
N
ε-(Hexanoyl) lysine |
L-NAME |
N
ω-Nitro-L-arginine methyl ester |
OHC | Octahydrocurcumin |
PD | Parkinson's disease |
RNS | Reactive nitrogen species |
ROS | Reactive oxygen species |
Sir2 | Silent information regulator 2 |
SMEDDS | Self-microemulsifying drug delivery systems |
O2− | Superoxide |
SOD | Superoxide dismutase |
THC | Tetrahydrocurcumin |
TPA | 12-O-Tetradecanoylphorbol-13-acetate. |
Acknowledgements
This study was supported by the National Science Council NSC 101-2628-B-022-001-MY4, 102-2628-B-002-053-MY3.
References
-
A. El-Kattan and M. Varma, in Topics on drug metabolism, ed. J. Paxton, 3.0 edn, 2012, ch. 1, pp. 1–34 Search PubMed.
- G. P. Nagaraju, S. Aliya, S. F. Zafar, R. Basha, R. Diaz and B. F. El-Rayes, Integr. Biol., 2012, 4, 996 RSC.
- O. B. Villaflores, Y. J. Chen, C. P. Chen, J. M. Yeh and T. Y. Wu, Taiwan. J. Obstet. Gynecol., 2012, 51, 554 CrossRef PubMed.
- A. Shehzad, G. Rehman and Y. S. Lee, BioFactors, 2013, 39, 69 CrossRef CAS PubMed.
- M. S. Baliga, N. Joseph, M. V. Venkataranganna, A. Saxena, V. Ponemone and R. Fayad, Food Funct., 2012, 3, 1109 CAS.
- R. E. Carroll, R. V. Benya, D. K. Turgeon, S. Vareed, M. Neuman, L. Rodriguez, M. Kakarala, P. M. Carpenter, C. McLaren, F. L. Meyskens, Jr and D. E. Brenner, Cancer Prev. Res., 2011, 4, 354 CrossRef CAS PubMed.
- R. A. Sharma, S. A. Euden, S. L. Platton, D. N. Cooke, A. Shafayat, H. R. Hewitt, T. H. Marczylo, B. Morgan, D. Hemingway, S. M. Plummer, M. Pirmohamed, A. J. Gescher and W. P. Steward, Clin. Cancer Res., 2004, 10, 6847 CrossRef CAS PubMed.
- G. M. Holder, J. L. Plummer and A. J. Ryan, Xenobiotica, 1978, 8, 761 CrossRef CAS.
- C. S. Lai, J. C. Wu, S. F. Yu, V. Badmaev, K. Nagabhushanam, C. T. Ho and M. H. Pan, Mol. Nutr. Food Res., 2011, 55, 1819 CAS.
- P. Anand, S. G. Thomas, A. B. Kunnumakkara, C. Sundaram, K. B. Harikumar, B. Sung, S. T. Tharakan, K. Misra, I. K. Priyadarsini, K. N. Rajasekharan and B. B. Aggarwal, Biochem. Pharmacol., 2008, 76, 1590 CrossRef CAS PubMed.
- T. Atsumi, K. Tonosaki and S. Fujisawa, Anticancer Res., 2007, 27, 363 CAS.
- Y. Kadoma and S. Fujisawa, In Vivo, 2007, 21, 979 CAS.
- Y. Sugiyama, S. Kawakishi and T. Osawa, Biochem. Pharmacol., 1996, 52, 519 CrossRef CAS.
- L. Pari and P. Murugan, Basic Clin. Pharmacol. Toxicol., 2006, 99, 329 CrossRef CAS PubMed.
- L. Xiang, Y. Nakamura, Y. M. Lim, Y. Yamasaki, Y. Kurokawa-Nose, W. Maruyama, T. Osawa, A. Matsuura, N. Motoyama and L. Tsuda, Aging, 2011, 3, 1098 CAS.
- K. Okada, C. Wangpoengtrakul, T. Tanaka, S. Toyokuni, K. Uchida and T. Osawa, J. Nutr., 2001, 131, 2090 CAS.
- N. Sermkaew, K. Wiwattanawongsa, W. Ketjinda and R. Wiwattanapatapee, AAPS PharmSciTech, 2013, 14, 321 CrossRef CAS PubMed.
- M. Valko, D. Leibfritz, J. Moncol, M. T. Cronin, M. Mazur and J. Telser, Int. J. Biochem. Cell Biol., 2007, 39, 44 CrossRef CAS PubMed.
- C. K. Ferrari, Biogerontology, 2004, 5, 275 CrossRef CAS PubMed.
- K. Rahman, Clin. Interventions Aging, 2007, 2, 219 CAS.
- T. Osawa, Y. Sugiyama, M. Inayoshi and S. Kawakishi, Biosci., Biotechnol., Biochem., 1995, 59, 1609 CrossRef CAS.
- V. Ravindranath and N. Chandrasekhara, Toxicology, 1981, 22, 337 CrossRef.
- C. R. Ireson, D. J. Jones, S. Orr, M. W. Coughtrie, D. J. Boocock, M. L. Williams, P. B. Farmer, W. P. Steward and A. J. Gescher, Cancer Epidemiol., Biomarkers Prev., 2002, 11, 105 CAS.
- P. Somparn, C. Phisalaphong, S. Nakornchai, S. Unchern and N. P. Morales, Biol. Pharm. Bull., 2007, 30, 74 CAS.
- S. M. Khopde, K. I. Priyadarsini, S. N. Guha, J. G. Satav, P. Venkatesan and M. N. Rao, Biosci., Biotechnol., Biochem., 2000, 64, 503 CrossRef CAS.
- M. Naito, X. Wu, H. Nomura, M. Kodama, Y. Kato, Y. Kato and T. Osawa, J. Atheroscler. Thromb., 2002, 9, 243 CrossRef CAS.
- T. Magwere, Y. S. Naik and J. A. Hasler, Free Radical Biol. Med., 1997, 22, 321 CrossRef CAS.
- P. Murugavel and L. Pari, Renal Failure, 2004, 26, 517 CrossRef CAS PubMed.
- F. B. Priviero, C. E. Teixeira, M. A. Claudino, N. G. De, A. Zanesco and E. Antunes, Eur. J. Pharmacol., 2007, 571, 189 CrossRef CAS PubMed.
- S. Nakmareong, U. Kukongviriyapan, P. Pakdeechote, W. Donpunha, V. Kukongviriyapan, B. Kongyingyoes, K. Sompamit and C. Phisalaphong, Naunyn-Schmiedebergs Arch. Pharmacol., 2011, 383, 519 CrossRef CAS PubMed.
- S. Nakmareong, U. Kukongviriyapan, P. Pakdeechote, V. Kukongviriyapan, B. Kongyingyoes, W. Donpunha, P. Prachaney and C. Phisalaphong, Hypertens. Res., 2012, 35, 418 CrossRef CAS PubMed.
- S. Mishra, M. Mishra, P. Seth and S. K. Sharma, NeuroReport, 2011, 22, 23 CrossRef CAS PubMed.
- D. Vauzour, Oxid. Med. Cell. Longevity, 2012, 2012, 914273 CrossRef PubMed.
- S. D. Smid, J. L. Maag and I. F. Musgrave, Food Funct., 2012, 3, 1242 Search PubMed.
- L. M. de Lau, P. C. Giesbergen, M. C. de Rijk, A. Hofman, P. J. Koudstaal and M. M. Breteler, Neurology, 2004, 63, 1240 CrossRef CAS.
- D. S. Goldstein, P. Sullivan, C. Holmes, G. W. Miller, S. Alter, R. Strong, D. C. Mash, I. J. Kopin and Y. Sharabi, J. Neurochem., 2013, 126, 591 CrossRef CAS PubMed.
- I. Rybakowska, G. Szreder, K. Kaletha, M. Barwina, W. Waldman and A. J. Sein, Przegl. Lek., 2011, 68, 486 Search PubMed.
- D. G. Anderson, S. V. Mariappan, G. R. Buettner and J. A. Doorn, J. Biol. Chem., 2011, 286, 26978 CrossRef CAS PubMed.
- S. W. Li, T. S. Lin, S. Minteer and W. J. Burke, Mol. Brain Res., 2001, 93, 1 CrossRef CAS.
- J. N. Rees, V. R. Florang, L. L. Eckert and J. A. Doorn, Chem. Res. Toxicol., 2009, 22, 1256 CrossRef CAS PubMed.
- J. N. Johannessen and S. P. Markey, Drug Alcohol Depend., 1984, 13, 367 CrossRef CAS.
- S. A. Mandel, Y. Sagi and T. Amit, Neurochem. Res., 2007, 32, 1694 CrossRef CAS PubMed.
- K. Chiba, A. J. Trevor and N. Castagnoli, Jr, Biochem. Biophys. Res. Commun., 1985, 128, 1228 CrossRef CAS.
- N. J. Riachi, J. C. LaManna and S. I. Harik, J. Pharmacol. Exp. Ther., 1989, 249, 744 CAS.
- P. Bajpai, M. C. Sangar, S. Singh, W. Tang, S. Bansal, G. Chowdhury, Q. Cheng, J. K. Fang, M. V. Martin, F. P. Guengerich and N. G. Avadhani, J. Biol. Chem., 2013, 288, 4436 CrossRef CAS PubMed.
- A. H. Schapira, Cell Death Differ., 2007, 14, 1261 CrossRef CAS PubMed.
- Y. Mizuno, N. Sone and T. Saitoh, J. Neurochem., 1987, 48, 1787 CrossRef CAS.
- A. Rajeswari and M. Sabesan, Inflammopharmacology, 2008, 16, 96 CrossRef CAS PubMed.
- H. Zheng, T. Amit, O. Bar-Am, M. Fridkin, M. B. Youdim and S. A. Mandel, J. Alzheimer’s Dis., 2012, 30, 1 CrossRef CAS PubMed.
- Q. L. Ma, M. E. Harris-White, O. J. Ubeda, M. Simmons, W. Beech, G. P. Lim, B. Teter, S. A. Frautschy and G. M. Cole, J. Neurochem., 2007, 103, 1594 CrossRef CAS PubMed.
- F. G. De Felice, P. T. Velasco, M. P. Lambert, K. Viola, S. J. Fernandez, S. T. Ferreira and W. L. Klein, J. Biol. Chem., 2007, 282, 11590 CrossRef CAS PubMed.
- K. Hsiao, P. Chapman, S. Nilsen, C. Eckman, Y. Harigaya, S. Younkin, F. Yang and G. Cole, Science, 1996, 274, 99 CrossRef CAS.
- M. Sakono, T. Zako, H. Ueda, M. Yohda and M. Maeda, FEBS J., 2008, 275, 5982 CrossRef CAS PubMed.
- A. N. Begum, M. R. Jones, G. P. Lim, T. Morihara, P. Kim, D. D. Heath, C. L. Rock, M. A. Pruitt, F. Yang, B. Hudspeth, S. Hu, K. F. Faull, B. Teter, G. M. Cole and S. A. Frautschy, J. Pharmacol. Exp. Ther., 2008, 326, 196 CrossRef CAS PubMed.
- D. Harman, Antioxid. Redox Signaling, 2003, 5, 557 CrossRef CAS PubMed.
- S. Khandhadia and A. Lotery, Expert Rev. Mol. Med., 2010, 12, e34 CrossRef PubMed.
- C. Kenyon, Cell, 2005, 120, 449 CrossRef CAS PubMed.
- V. Dulic, Methods Mol. Biol., 2013, 965, 15 Search PubMed.
- Q. Xie, J. Chen and Z. Yuan, Acta Biochim. Biophys. Sin., 2012, 44, 897 CrossRef CAS PubMed.
- F. Wang, M. Nguyen, F. X. Qin and Q. Tong, Aging Cell, 2007, 6, 505 CrossRef CAS PubMed.
- K. Kitani, T. Osawa and T. Yokozawa, Biogerontology, 2007, 8, 567 CrossRef CAS PubMed.
- M. H. Pan, C. S. Lai and C. T. Ho, Food Funct., 2010, 1, 15 CAS.
- M. H. Pan and C. T. Ho, Chem. Soc. Rev., 2008, 37, 2558 RSC.
- M. H. Pan, Y. S. Chiou, Y. J. Wang, C. T. Ho and J. K. Lin, Food Funct., 2011, 2, 101 CAS.
- J.
G. Scandalios, Braz. J. Med. Biol. Res., 2005, 38, 995 CrossRef CAS PubMed.
- S. Yodkeeree, S. Garbisa and P. Limtrakul, Acta Pharmacol. Sin., 2008, 29, 853 CrossRef CAS PubMed.
- J. C. Wu, C. S. Lai, V. Badmaev, K. Nagabhushanam, C. T. Ho and M. H. Pan, Mol. Nutr. Food Res., 2011, 55, 1646 CAS.
- J. M. Kim, S. Araki, D. J. Kim, C. B. Park, N. Takasuka, H. Baba-Toriyama, T. Ota, Z. Nir, F. Khachik, N. Shimidzu, Y. Tanaka, T. Osawa, T. Uraji, M. Murakoshi, H. Nishino and H. Tsuda, Carcinogenesis, 1998, 19, 81 CrossRef CAS.
- M. T. Huang, W. Ma, Y. P. Lu, R. L. Chang, C. Fisher, P. S. Manchand, H. L. Newmark and A. H. Conney, Carcinogenesis, 1995, 16, 2493 CrossRef CAS PubMed.
- M. H. Pan, C. S. Lai, J. C. Wu and C. T. Ho, Mol. Nutr. Food Res., 2011, 55, 32 CAS.
- J. Han, X. Y. Pan, Y. Xu, Y. Xiao, Y. An, L. Tie, Y. Pan and X. J. Li, Autophagy, 2012, 8, 812 CrossRef CAS PubMed.
- K. Ranjan, A. Sharma, A. Surolia and C. Pathak, BioFactors, 2013 DOI:10.1002/biof.1098.
Footnote |
† The authors contributed equally to this work. |
|
This journal is © The Royal Society of Chemistry 2014 |