DOI:
10.1039/C4CC01364F
(Feature Article)
Chem. Commun., 2014,
50, 7921-7928
Labile alkoxyamines: past, present, and future
Received
21st February 2014
, Accepted 1st May 2014
First published on 2nd May 2014
Abstract
Alkoxyamines – per-alkylated derivatives of hydroxylamine R1R2NO–R3 – can undergo C–ON bond homolysis to release a persistent nitroxyl radical R1R2NO˙ and a transient alkyl radical R3˙. Although they were considered as an oddity when discovered in 1974, their properties have been extensively studied since the seminal work of Solomon, Rizzardo and Cacioli (Chem. Abstr., 102, 221335q), who patented the key role of alkoxyamines in nitroxide-mediated polymerization (NMP) in 1985. This feature article surveys and assesses the various applications of alkoxyamines: in tin-free radical chemistry, e.g., for the elaboration of carbo- or hetero-cycles, for the development of new reactions, for total synthesis of natural products; in polymerization under thermal conditions (NMP) or photochemical conditions (nitroxide-mediated photo-polymerization, NMP2); and in the design of smart materials. In this feature article, we also describe our recent findings concerning the chemical triggering of the C–ON bond homolysis in alkoxyamines, affording the controlled generation of alkyl radicals at room temperature. Based on these results, we describe herein some new opportunities for applications in the field of smart materials, and of course, some possible developments as new initiators for NMP as well as an entirely new field of application: the use of alkoxyamines as theranostic agents. Indeed, each of the radicals released after homolysis can play an appealing role: the nitroxide, through dynamic nuclear polarization (DNP), can be used for imagery purposes (diagnostic properties), while the alkyl radical can be used to induce cellular disorders in abnormal cells (therapeutic activity).
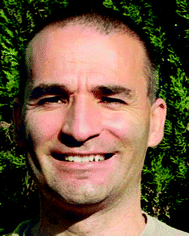
Gérard Audran
| Gerard Audran defended his PhD in 1995, and was appointed full professor in 2009 at Aix-Marseille University. He is the co-author of more than 60 scientific papers. His main research topics are the enantioselective synthesis of five- and six-membered ring natural and bioactive products (terpenes, carbasugars, carbonucleosides) with the aid of biocatalysts. Recently, he turned also his interest to the field of radical chemistry, particularly in the chemistry of nitroxides and alkoxyamines. |
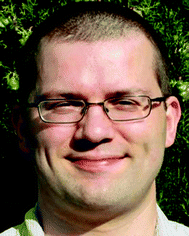
Paul Brémond
| Paul Brémond obtained his PhD in 2008 under the supervision of Prof. Honoré Monti and Prof. Gérard Audran at the University Paul Cézanne at Marseille, France. He became a lecturer for one year in Marseille, and then he moved to Harvard University in Cambridge, Massachusetts, U.S.A. to join the group of Prof. Yoshito Kishi as a postdoctoral associate. In 2010, he was recruited as an assistant professor (Maître de Conférences) at the University of Provence (now Aix-Marseille Université), at Marseille, France. He works in the Institute of Radical Chemistry on the synthesis and physical, chemical and biological evaluation of alkoxyamines. |
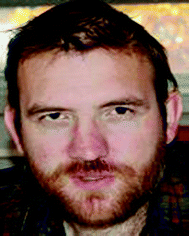
Sylvain. R. A. Marque
| Sylvain Marque was awarded a PhD from Paul Cézanne University (Marseille) in 1996. In 1997, he spent one year as a postdoctoral fellow with Prof. D. H. R. Barton at Texas A&M University. From 1998 to 2000, he joined Prof. H. Fischer's team (University of Zürich). In 2000, he was appointed Assistant Professor at University of Provence, and in 2008, he was promoted to a Professorship position. His research is focused on the reactivity of organic radicals investigated by means of EPR, NMR and calculations, and applied in Organic Chemistry, (controlled) Polymerization, Spectroscopy, and Biology. |
Introduction
Alkoxyamines (trialkylhydroxylamines) have been known since the early 20th century1 and their chemistry, either as reactants/products or intermediates, has been reviewed several times.2–4 However, this family of molecules, especially the labile ones, was considered as seemingly trivial and of minor use until the 1990s,5 when they started to be used as initiators for one of the most promising techniques for controlling radical polymerization:6 Nitroxide Mediated Polymerization (NMP, vide infra).7 In fact, the renewed interest in this family is only due to the radical reactivity displayed by some of its members. In this feature article, we propose to describe the major milestones, from the discovery of the radical reactivity of alkoxyamines to their implementation in industry,8,9 and their potential new applications (Fig. 1).
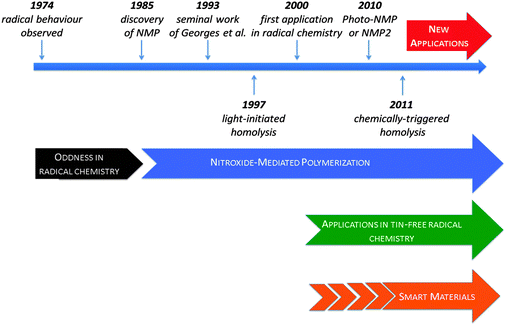 |
| Fig. 1 Alkoxyamines used as radical initiators: timescale and milestones. | |
The discovery of the radical reactivity of alkoxyamines and of the nitroxide mediated polymerization
Indeed, in 1974, for the first time Kovtun et al.10 reported that the stability of alkoxyamines was unexpectedly dependent on experimental conditions.11
† They observed that the decomposition of alkoxyamines into nitroxides and alkyl radicals (Scheme 1) was dramatically dependent on the presence and amount of scavengers such as oxygen or iodine. This chemistry did not arouse too much interest12,13 for one decade, except for a few articles related to the degradation of polymers.14–17 In fact, the kinetics underlying this amazing result were unveiled 24 years later by Fischer et al.18 Indeed, the apparent high stability of alkoxyamines is governed by the so-called “Persistent Radical Effect”.19–21
‡ Using alkoxyamine 1 (Fig. 2), Fischer et al.18 showed that it was completely decomposed in ca. 90 minutes at 80 °C when the experiment was performed in the presence of an alkyl radical scavenger (galvinoxyl) whereas only 2% was decomposed in ca. 10 hours in its absence!7
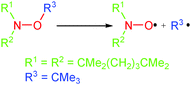 |
| Scheme 1 Homolysis of the C–ON bond in alkoxyamines. An example of the lability of alkoxyamine – R1 and R2 for the nitroxyl fragment and R3 as an alkyl fragment – investigated in the presence of oxygen by Kovtun et al.10 | |
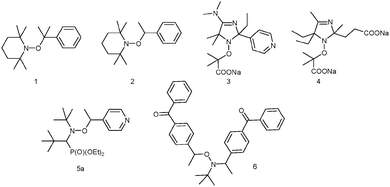 |
| Fig. 2 Some examples of alkoxyamines developed for new modes of initiation. | |
In 1985, Solomon, Rizzardo, and Cacioli22 patented the concept of Nitroxide Mediated Polymerization, which relies on the reversible homolysis of the C–ON bond of alkoxyamines (Scheme 2a and b).23,24 They were the first25 to improve the conventional 3-stage scheme for radical polymerization by proposing additional steps in each stage (Scheme 2b). This improved scheme is often displayed in its oversimplified form (Scheme 2c).26
§ This major discovery did not arouse too much interest in the community of polymer chemists, until the seminal work of Georges et al.27,28 8 years later (Scheme 2d). Using a bi-component system based on 2,2,6,6-tetramethylpiperidinyl-1-N-oxyl radical (TEMPO) and dibenzoylperoxide, they showed that the radical polymerization of styrene is nicely controlled, affording polymers exhibiting narrow polydispersity indices (<1.5). Keeping the robustness of the radical polymerization, NMP now plays a major role in the preparation of well controlled and defined polymer structures.5–7,26,29–35 Since then, it has triggered a tremendous amount of work in different fields, spanning from kinetics investigations,29 design of new initiators,36,37 preparation of new materials,30 to industrial applications.33 All the results reported in the cited reviews show that NMP is now a mature technique that is currently used in industry6 to prepare new polymers and new materials and this aspect will not be discussed any further.5,6,33,34
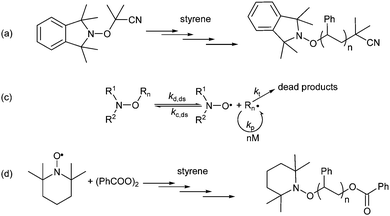 |
| Scheme 2 (a) An example of the first NMP experiment proposed by Solomon et al.;22 (b) complete kinetic scheme for NMP, and (c) its oversimplified scheme; and (d) bi-component system for NMP proposed by Georges et al.27,28 For the experimental conditions in (a) and (d), see the references cited. kd and kc: C–ON bond homolysis and reformation rate constants of the initiator; kd,ds and kc,ds: C–ON bond homolysis and reformation rate constants of the initiator and the macro-alkoxyamine (dormant species ds), respectively; kadd: rate constant for the addition of the initiating alkyl radical onto the monomer; kp: propagation rate constant; kt,ds: termination rate constants of the polymer radical (in general, a magnitude close to that of molecular species); kdD: rate constant for the intramolecular proton transfer (IPT) in molecular and macromolecular alkoxyamines, kcD: rate constant for the intermolecular hydrogen-atom transfer (HAT) between the nitroxide and the alkyl radical; kdec: rate constant for the nitroxide degradation processes. | |
Fundamentals of the radical reactivity of alkoxyamines
During the last 20 years, the effects ruling C–ON bond homolysis and its reformation have been carefully and extensively studied. As these effects are not the topic of this article, they will be addressed briefly. The reformation of the alkoxyamine C–ON bond has been the purpose of a recent review whose main lines are displayed in Fig. 3.38 Interestingly, the substituents of the alkyl radical and the nitroxide involve effects that are either additive or synergistic, except for the effect of the penultimate unit which is not yet clear. As expected, the main effects involved in ruling kc are the stabilization, the bulkiness, and the polarity of both the alkyl radical and the nitroxide, each to a different extent depending on the species. The solvent effects were also reported in this review.
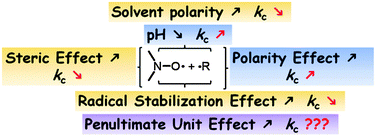 |
| Fig. 3 Effects ruling the reformation rate constant kc. Reprinted with permission from E. G. Bagryaskaya, S. R. A. Marque, Chem. Rev., 2014, ASAP. Copyright 2014 American Chemical Society. | |
The alkoxyamine C–ON bond homolysis, although shortly discussed in several reviews, has not yet been the topic of a devoted review.5–7,21,29 The main effects are displayed in Fig. 4 and briefly discussed. Interestingly, all the main effects of the R3 group on kd exhibit the same trend whereas, on the nitroxyl fragment R1R2NO, the steric and polar effects afford antagonistic trends. Moreover, when the whole structure is considered some synergistic antagonistic effects can arise. Furthermore, the impact of these effects can be strikingly modified by minor/side effects such as intramolecular hydrogen bonding, anomeric and anchimeric effects, long range effects etc.7,26 Nevertheless, it has been possible to develop structure reactivity relationships (SRR) robust enough to predict either the right value of kd or at least the trend expected.39–41 Taking into account the versatility of the structures and the potential applications (vide infra), it is not possible to provide accurate and reliable rules on what the “ideal” or “perfect” structure is. Nevertheless, theoretical42,43 and empirical39–41 tools are available to determine kd and kc values, so that the success or the failure of the aimed application might be envisioned.
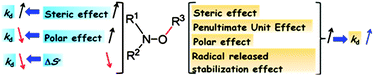 |
| Fig. 4 Main effects involved in the C–ON bond homolysis of alkoxyamines. | |
Alkoxyamines for tin-free radical chemistry
In 2000, Studer44 reported the first application of alkoxyamines as substitutes in tin-free radical chemistry, as highlighted by the preparation of triquinane via radical cascade cyclizations (Scheme 3). Several applications have followed: the formation of lactones or lactames45,46via the Ueno–Stork reaction,47,48 conjugative addition/cyclization/elimination,49–51 intramolecular homolytic aromatic substitution,51,52 1,2-intermolecular radical addition,50,53,54 carboxyaminoxylation,55 isonitrilation,56or metal free-carbonylation (Fig. 5).57 Theodorakis et al.,58 in their preparation of (−)-fusarisetin A (Scheme 4), highlighted the efficiency and the interest to use alkoxyamine as a radical initiator.
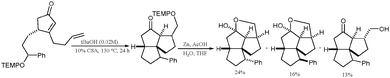 |
| Scheme 3 First example of an alkoxyamine used as an initiator in tin free-radical chemistry. | |
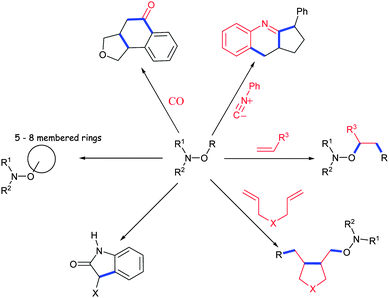 |
| Fig. 5 Various applications of alkoxyamines as synthetic tools (in blue, newly formed bonds; in red, new fragments added). | |
 |
| Scheme 4 Preparation of (−)-fusarisetin A based on the radical cyclization (in blue, newly formed bonds) involving the use of an alkoxyamine as a thermal radical initiator. | |
Photolysis of alkoxyamines and photo-NMP
In 1997, Scaiano et al.59 showed that laser-flash irradiation of alkoxyamines 1 and 2 (Fig. 2) generates nitroxides and alkyl radicals through a homolytic process. It was a decade later60–62
¶ that NMP under irradiation (photo-NMP or NMP2, Fig. 6) was developed simultaneously by Gigmes et al.63,64 and Yoshida et al.65 Gigmes et al.63 showed that the photo-labile alkoxyamine 6 was suitable for controlling the photo-polymerization of n-butyl acrylate, and developed nice applications in the preparation of covalently bonded multilayered micropatterns.66
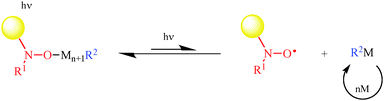 |
| Fig. 6 Principles of nitroxide-mediated photopolymerization ( as chromophore group). Copyright 2012 Wiley. Used with permission from D. Gigmes, S. R. A. Marque, Nitroxide Mediated Polymerization and its Applications, in Encyclopedia of Radicals in Chemistry, Biology, and Materials, ed. C. Chatgilialoglu, A. Studer, Wiley, Chichester, U.K., 2012, vol. 4, pp. 1813–1850. | |
Chemical (de)activation of alkoxyamines
In 2009, the possible (de)activation of the alkoxyamine C–ON bond homolysis based on chemical changes in the nitroxyl fragment was suggested by Marx et al.,67 and, independently, a striking effect of the protonation of the nitroxyl moiety on the decomposition pathways during ESI-MS experiments was reported by Mazarin et al.68 However, the first kinetic evidence of the effect of protonation on the C–ON homolysis was only reported in 2011 by Brémond and Marque69 who showed, in sharp contrast to the earlier observations,70–73
|| that kd values were strikingly increased upon protonation (5b) of the alkyl fragment of alkoxyamine 5a (Scheme 5), as well as upon oxidation (5c), acylation (5d), alkylation (5e,f), and complexation (5g) of the pyridinyl moiety (Scheme 5),74 as expected from structure–reactivity relationships.41 In the same year, Bagryanskaya et al.75 reported the reverse effect of the protonation for 3 and 4, i.e., the strengthening of the alkoxyamine C–ON bond upon protonation of the nitroxyl fragment. Hence, a clear decrease in the homolysis rate constant kd was observed from basic to acidic pH (Fig. 7), as expected from the polar effect of the nitroxide fragment (Fig. 4).39,40 The activation/deactivation events were efficiently applied to NMP.75,76
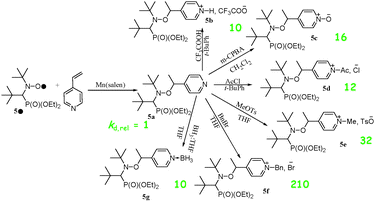 |
| Scheme 5 Different types of chemical activation for alkoxyamine 5a. Green values are for kd,rel = kd,5b–5g/kd,5a. | |
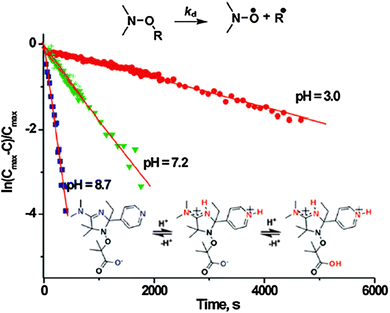 |
| Fig. 7 Effect of the protonation on kd of 3. (■) pH=8.7, (▼) pH=7.2 and (●) pH = 3.0. Reprinted with permission from M. V. Edeleva, I. A. Kirilyuk, I. F. Zhurko, D. A. Parkhomenko, Y. P. Tsentalovich, E. G. Bagryanskaya, J. Org. Chem., 2011, 76, 5558–5573. Copyright 2014 American Chemical Society. | |
Consequently, the same mode of activation, i.e., protonation of a nitrogen atom, has an antagonistic effect on the alkoxyamine C–ON bond homolysis, whether the protonation occurs on the nitroxyl fragment or on the alkyl fragment. That is, upon protonation, the electron-withdrawing properties of the substituents are increased, leading either to a striking decrease in kd for the nitroxyl moiety or to a dramatic increase in kd for the alkyl fragment (Fig. 8). This effect depends only on the increase/decrease in the electron withdrawing properties of the substituents,39–41 implying a change in kd, as displayed in Fig. 8 and as highlighted with 5a–5g and 3 in Scheme 5 and in Fig. 7, respectively.
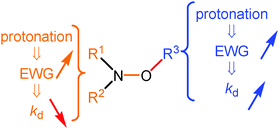 |
| Fig. 8 Consequences of the protonation depending on the site of protonation. | |
In fact, the chemical triggering of the C–ON bond homolysis led us to develop the concept of smart spin probes (doted red line in Fig. 9). Indeed, alkoxyamines can be gathered in 3 families, depending on the strength of the C–ON bond (Bond Dissociation Energy, BDE): for BDE < 100 kJ mol−1 (t1/2, 20 °C < 30 min), a family of alkoxyamines that are too unstable to be handled and stored easily and safely, and that have been of no use up to now; for 100 kJ mol−1 < BDE < 140 kJ mol−1, a family which comprises all alkoxyamines currently applied to NMP and radical chemistry; and for BDE > 140 kJ mol−1 (t1/2, 20 °C > 800 years or t1/2, 200 °C > 8 s),** a family of alkoxyamines that are too stable to be involved under controlled radical reactions. In fact, the concept of smart spin probes relies on the activation of highly stable alkoxyamines into highly labile alkoxyamines (red dotted arrow in Fig. 9) for new applications in biology and for smart materials. Our recent results nicely support this concept, as we observed a clear activation (green arrow in Fig. 9). Thus our research is now focused on the development of very stable alkoxyamines that can be chemically or biologically switched to highly labile alkoxyamines (red dotted arrow in Fig. 9). It has already been possible, by combining solvent effects and chemical activation, to develop alkoxyamine 5e that exhibits t1/2 = 48 min at 37 °C in water,77 which led us to envision some applications of alkoxyamines in some fields of biology such as theranostics.
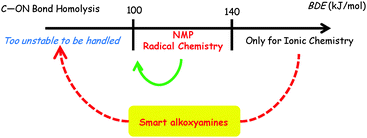 |
| Fig. 9 Smart spin probe concept. Reproduced from ref. 79. | |
Application of alkoxyamines as theranostic agents
Ten years ago a new field emerged: theranostics78
†† where concomitant therapeutic and diagnostic properties can be exhibited by a single molecule, which makes it possible to monitor in situ and directly the efficiency of drugs. Interestingly, alkoxyamines are able to release two different types of radicals: a rather persistent nitroxide (several minutes to several hours of life-time under biological conditions) and a transient, generally highly reactive, alkyl radical. Our recent results led us to propose the concept displayed in Fig. 10 and an approach to the use of alkoxyamines as theranostic agents (Fig. 11).79
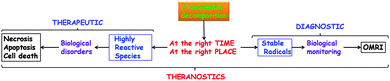 |
| Fig. 10 Theranostic concept based on the radical chemistry of alkoxyamines. Reproduced from ref. 79. | |
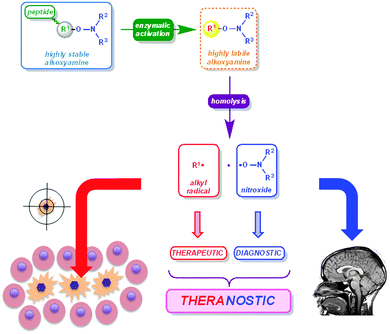 |
| Fig. 11 Theranostic concept applied to alkoxyamines. | |
When the decomposition of an alkoxyamine occurs at the right time and at the right place – rather quickly and in unhealthy cells – it generates alkyl radical and nitroxide, each being endorsed with a specific role. Alkyl radicals are highly reactive transient species that generate biological disorders80 – by H-abstraction, addition onto unsaturated bonds, electron transfer, etc. – which in turn trigger the cell death process81 (necrosis and apoptosis). Hence, they exhibit therapeutic activity (Fig. 10). Nitroxides are persistent radical species which can be detected readily or through the modifications they cause in the magnetic properties of their surroundings. They can be used to monitor the efficiency of the drug when using techniques such as Electron Paramagnetic Resonance Imaging (EPRI) or Overhauser-enhanced Magnetic Resonance Imaging (OMRI).82,83 Hence, they exhibit diagnostic property. This concept (Fig. 10) will be successful only if the homolysis of the alkoxyamine is selectively triggered at the right time, at the right place, and with a rate high enough to ensure a nitroxide concentration suitable for monitoring.
To fulfill the requirements described above, a 3-fragment alkoxyamine should be designed (Fig. 11): the activation/addressing fragment (green part), the virtual alkyl radical (yellow circle), and the virtual nitroxide (blue part). The triggering of the alkoxyamine homolysis can be performed either through chemical or physical activation combined to selective addressing or through a chemical reaction activated by the addressing event.79 The activation will release a transient alkoxyamine (dotted frame Fig. 11) that will decompose into a highly reactive radical81 (red frame) – increasing the amount of reactive oxygen species (ROS)81 or reacting with biomolecules – and into a persistent nitroxide (dark blue frame) which can be used to monitor the biological process involved using OMRI.82,83
Alkoxyamines for smart materials
For the past decade, alkoxyamines have been used in the development of smart materials. Indeed, the preliminary experiments performed by Otsuka et al.,84 about 10 years ago, on the scrambling of polymer chains based on the homolysis of the alkoxyamine led Rong et al.85,86 to propose self-healing materials based on reversible alkoxyamine homolysis (Fig. 12). Recently, Studer et al.87 showed that reversible homolysis can be applied to the development of dynamic microcrystal assemblies, as highlighted by the alternate green and red zeolites in Fig. 13. Such types of structure open up new opportunities in photonics.
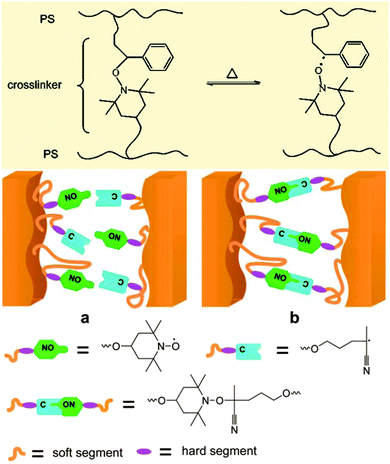 |
| Fig. 12 Self-healing polymer based on the reversible alkoxyamine homolysis. Top: Reprinted with permission from C. Yuan, M. Z. Rong, M. Q. Zhang, Z. P. Zhang, Y. C. Yuan, Chem. Mater., 2011, 23, 5076–5081. Copyright 2011 American Chemical Society. Bottom: Reproduced from ref. 86. | |
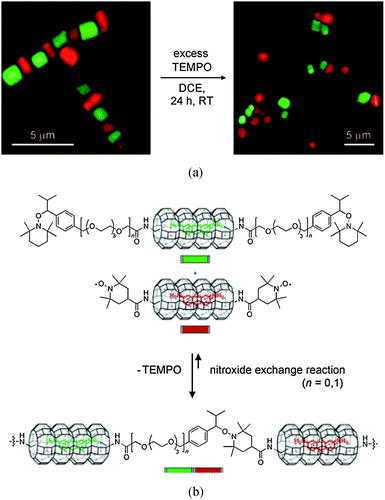 |
| Fig. 13 (a) Confocal microscopy images showing the reversibility of the chain formation with zeolites. (b) Nitroxide exchange reaction of alkoxyamine functionalized zeolite L crystal (green zeolites) with nitroxide-modified (red zeolites) to form ordered zeolite chains. Copyright 2010 Wiley. Used with permission from B. Schulte, M. Tsotsalas, M. Becker, A. Studer, L. De Cola, Angew. Chem., Int. Ed., 2010, 49, 6881–6884. | |
However, up to now, all applications have relied on a change in temperature and so for each application a specific alkoxyamine must be designed. Chemical triggering and biological triggering will lead to the development of new materials with innovative self-healing or optoelectronic properties. One can imagine C–ON homolysis controlled by chemical or biological stimuli allowing the modulation of channel accessibility, of the permeability of materials, and of the magnetic properties of materials, everything being performed from room to physiological temperature. For example, the reversible homolysis of an alkoxyamine C–ON bond triggered by a change in pH has the potential to be applied to the control of access to channels in membranes, as highlighted in Fig. 14.
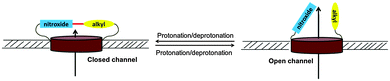 |
| Fig. 14 Model for controlling the passage of a solvent through the channels of a membrane. | |
Conclusion
Thirty years after the discovery of NMP, labile alkoxyamines can be considered as valuable initiators for radical reactions. Indeed, they are currently used in industry to prepare tailored polymers by NMP, just as peroxides and homologues are used to prepare basic/standard polymers by radical polymerization (Fig. 15).5,6,8,9,31 It is clear that alkoxyamines can now be considered as conventional reactants for radical chemistry in industry. Nevertheless, chemically triggered C–ON bond homolysis opens up new perspectives for NMP: (i) new initiators complying with the REACH directives, i.e. easier to store, to handle, to ship and much less hazardous than conventional thermal initiators;88 and (ii) new initiators for surface polymerizations on inorganic core particles which would be triggered only upon complexation. Despite the development of several alkoxyamines-based metal-free radical reactions, the latter are still scarcely used as synthesis tools. Recent results on the reversible activation of alkoxyamines open up new perspectives of applications in Biology, that is, such molecules can be applied as theranostic agents, and they can be tuned so that either the therapeutic activity81 or the diagnostic property is favoured.82,83 Taking into account the generality of the concept and the versatility of alkoxyamines, one may expect many other applications in Biology and (smart) Materials Sciences: orphan diseases, phytochemistry, self-healing materials, switches, etc., as long as specific and selective addressing/activation is performed. External, selective and reversible activation/deactivation of alkoxyamines should open new opportunities for the development of new smart materials or molecular devices.
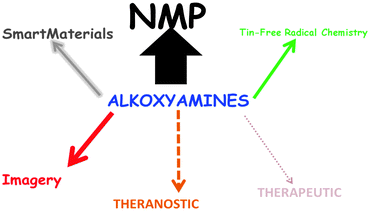 |
| Fig. 15 Fields of application for alkoxyamines. | |
Acknowledgements
Authors thank Aix-Marseille University, CNRS, and Agence Nationale de la Recherhce (grants NITROMRI ANR-09-BLAN-0017-01 and SonRadIs ANR-11-JS07-002-01) for financial support.
Notes and references
- L. W. Jones and R. T. Major, J. Am. Chem. Soc., 1927, 49, 1527–1540 CrossRef CAS.
-
J. S. Roberts, in Comprehensive Organic Chemistry, Book ed. D. H. R. Barton and W. D. Ollis, Series ed. I. O. Sutherland, Pergamon Press, Oxford, 1979, vol. 2, p. 185 Search PubMed.
-
R. Askani and D. F. Taber, in Comprehensive Organic Synthesis, Book ed. B. M. Trost and I. Fleming, Series ed. E. Winterfeld, Pergamon Press, Oxford, 1991, vol. 6, p. 104 Search PubMed.
-
C. M. Marson and A. D. Hobson, in Comprehensive Organic Functional Groups, Book ed. A. R. Katritzky, O. Meth-Cohn and C. W. Rees, Series ed. S. V. Ley, Pergamon Press, Oxford, 1995, vol. 2, p. 298 Search PubMed.
-
G. Moad and D. H. Solomon, The Chemistry of Radical Polymerization, Elsevier, Amsterdam, 2nd edn, 2006, and references cited therein Search PubMed.
- J. Nicolas, Y. Guillaneuf, C. Lefay, D. Bertin, D. Gigmes and B. Charleux, Prog. Polym. Sci., 2013, 38(1), 63–235 CrossRef CAS PubMed.
-
D. Gigmes and S. R. A. Marque, Nitroxide Mediated Polymerization and its Applications, in Encyclopedia of Radicals in Chemistry, Biology, and Materials, ed. C. Chatgilialoglu and A. Studer, Wiley, Chichester, U.K., 2012, vol. 4, pp. 1813–1850 Search PubMed.
-
P. Nesvadba, A. Kramer, A. Steinmann and W. Stauffer, PCT Int. Appl., WO 99/03894, 1999 Search PubMed.
-
J.-L. Couturier, O. Guerret, D. Gigmes, S. Marque, F. Chauvin, P.-E. Dufils, D. Bertin and P. Tordo, Patent no FR2843394, 2004 Search PubMed;
J.-L. Couturier, O. Guerret, D. Gigmes, S. Marque, F. Chauvin, P.-E. Dufils, D. Bertin and P. Tordo, WO 2004014926, 2004 Search PubMed.
- G. A. Kovtun, A. L. Aleksandrov and V. A. Golubev, Bull. Acad. Sci. USSR, Div. Chem. Sci., 1974, 2115–2121 CrossRef CAS; G. A. Kovtun, A. L. Aleksandrov and V. A. Golubev, Izv. Akad. Nauk SSSR, Ser. Khim., 1974, 2197–2203 Search PubMed.
-
K. U. Ingold, Radical Reaction Rates in Liquids, in Landolt-Börnstein, Group II, ed. H. Fischer, Springer-Verlag, Berlin, 1983, vol. 13c, p. 166 Search PubMed.
- J. A. Howard and J. C. Tait, J. Org. Chem., 1978, 43, 4279–4283 CrossRef CAS.
- D. W. Grattan, D. J. Carlsson, J. A. Howard and D. M. Wiles, Can. J. Chem., 1979, 57, 2834–2842 CrossRef CAS PubMed.
- D. W. Grattan, D. J. Carlsson and D. M. Wiles, Polym. Degrad. Stab., 1979, 1, 69–84 CrossRef CAS.
- T. A. B. M. Bolsman, A. P. Blok and J. H. G. Frijns, Recl. Trav. Chim. Pays-Bas, 1978, 97(12), 310–313 CrossRef CAS.
- T. A. B. M. Bolsman, A. P. Blok and J. H. G. Frijns, Recl. Trav. Chim. Pays-Bas, 1978, 97(12), 313–319 CrossRef CAS.
- P. Stipa, L. Greci, P. Carloni and E. Damiani, Polym. Degrad. Stab., 1997, 55, 323 CrossRef CAS.
- T. Kothe, S. Marque, R. Martschke, M. Popov and H. Fischer, J. Chem. Soc., Perkin Trans. 2, 1998, 1553–1559 RSC.
- H. Fischer, J. Am. Chem. Soc., 1986, 108, 3925–3927 CrossRef CAS.
- B. E. Daikh and R. G. Finke, J. Am. Chem. Soc., 1992, 114, 2938–2943 CrossRef CAS.
- H. Fischer, Chem. Rev., 2001, 101, 3581–3610 CrossRef CAS PubMed.
-
D. H. Solomon, E. Rizzardo and P. Cacioli, Eur. Pat. Appl., 135280, 1985 Search PubMed;
D. H. Solomon, E. Rizzardo and P. Cacioli, US Pat., 4,581,429, 1986 Search PubMed; H. Solomon, E. Rizzardo and P. Cacioli, Chem. Abstr., 1985, 102, 221335q Search PubMed.
- E. Rizzardo, Chem. Aust., 1987, 54, 32–33 Search PubMed.
- G. Moad and E. Rizzardo, Macromolecules, 1995, 28, 8722–8728 CrossRef CAS.
- C. H. J. Johnson, G. Moad, D. H. Solomon, T. H. Spurling and D. J. Vearing, Aust. J. Chem., 1990, 43, 1215–1230 CrossRef CAS.
- D. Bertin, D. Gigmes, S. R. A. Marque and P. Tordo, Chem. Soc. Rev., 2011, 40, 2189–2198 RSC.
- M. K. Georges, R. P. N. Veregin, P. M. Kazmaier and G. K. Hamer, Macromolecules, 1993, 26, 2987–2988 CrossRef CAS.
- M. K. Georges, R. P. N. Veregin, P. M. Kazmaier, G. K. Hamer and M. Saban, Macromolecules, 1994, 27, 7228–7229 CrossRef CAS.
- A. Goto and T. Fukuda, Prog. Polym. Sci., 2004, 29, 329–385 CrossRef CAS PubMed and references cited therein.
- C. J. Hawker, A. W. Bosman and E. Harth, Chem. Rev., 2001, 101, 3661–3688 CrossRef CAS PubMed.
- M. Destarac, Macromol. React. Eng., 2010, 4, 165–179 CrossRef CAS.
- B. Charleux and J. Nicolas, Polymer, 2007, 48, 5813–5833 CrossRef CAS PubMed.
- P. Nesvadba, Chimia, 2006, 60, 832–840 CrossRef CAS and references cited therein.
- R. B. Grubbs, Polym. Rev., 2011, 51, 104–137 CrossRef CAS.
- W. A. Braunecker and K. Matyjaszewski, Prog. Polym. Sci., 2007, 32, 93–146 CrossRef CAS PubMed.
- D. Bertin, D. Gigmes and S. R. A. Marque, Recent Res. Dev. Org. Chem., 2006, 10, 63–121 CAS and references cited therein.
- A. C. Greene and R. B. Grubbs, ACS Symp. Ser., 2009, 1024, 81–93 CrossRef CAS PubMed.
- E. G. Bagryaskaya and S. R. A. Marque, Chem. Rev., 2014 DOI:10.1021/cr4000946.
- S. Marque, J. Org. Chem., 2003, 68, 7582–7590 CrossRef CAS PubMed.
- H. Fischer, A. Kramer, S. R. A. Marque and P. Nesvadba, Macromolecules, 2005, 38, 9974–9984 CrossRef CAS.
- D. Bertin, D. Gigmes, S. R. A. Marque and P. Tordo, Macromolecules, 2005, 38, 2638–2650 CrossRef CAS.
- J. L. Hodgson, C.-Y. Lin, M. L. Coote, S. R. A. Marque and K. Matyjaszewski, Macromolecules, 2010, 43(8), 3728–3743 CrossRef CAS.
- C. Y. Lin, S. R. A. Marque, K. Matyjaszewski and M. L. Coote, Macromolecules, 2011, 44(19), 7568–7583 CrossRef CAS.
- A. Studer, Angew. Chem., Int. Ed., 2000, 39, 1108–1111 CrossRef CAS.
- D. Bertin, D. Gigmes, S. R. A. Marque and P. Tordo, Tetrahedron, 2005, 61, 8752–8761 CrossRef CAS PubMed.
- C. Wetter and A. Studer, Chem. Commun., 2004, 174–175 RSC.
- Y. Ueno, K. Chino, M. Watanabe, O. Moriya and M. Okawara, J. Am. Chem. Soc., 1982, 104, 5564–5566 CrossRef CAS.
- G. Stork, R. Mook Jr, S. A. Biller and S. D. Rychnovsky, J. Am. Chem. Soc., 1983, 105, 3741–3742 CrossRef CAS.
- C. Leroi, B. Fenet, J.-L. Couturier, O. Guerret and M. A. Ciufolini, Org. Lett., 2003, 5, 1079–1081 CrossRef CAS PubMed.
- C. Wetter, K. Jantos, K. Woithe and A. Studer, Org. Lett., 2003, 5, 2899–2902 CrossRef CAS PubMed.
- A. Teichert, K. Jantos, K. Harms and A. Studer, Org. Lett., 2004, 6, 3477–3480 CrossRef CAS PubMed.
- C. Leroi, D. Bertin, P.-E. Dufils, D. Gigmes, S. Marque, P. Tordo, J.-L. Couturier, O. Guerret and M. A. Ciufolini, Org. Lett., 2003, 5, 4943–4945 CrossRef CAS PubMed.
- A. J. Herrera and A. Studer, Synthesis, 2005, 1389–1396 CAS.
- P.-E. Dufils, N. Chagneux, D. Gigmes, T. Trimaille, S. R. A. Marque, D. Bertin and P. Tordo, Polymer, 2007, 48, 5219–5225 CrossRef CAS PubMed.
- I. C. Wienhofer, A. Studer, M. T. Rahman, T. Fukuyama and I. Ryu, Org. Lett., 2009, 11, 2457–2460 CrossRef PubMed.
- B. Janza and A. Studer, Org. Lett., 2006, 8, 1875–1878 CrossRef CAS PubMed.
- Y. Uenoyama, M. Tsukida, T. Doi, I. Ryu and A. Studer, Org. Lett., 2005, 7, 2985–2988 CrossRef CAS PubMed.
- J. Xu, E. J. E. Caro-Diaz, M. H. Lacoske, C.-I. Hung, C. Jamora and E. A. Theodorakis, J. Am. Chem. Soc., 2012, 134, 5072–5075 CrossRef CAS PubMed.
- J. C. Scaiano, T. J. Connolly, N. Mohtat and C. N. Pliva, Can. J. Chem., 1997, 75, 92–97 CrossRef CAS.
- E. Yoshida, Colloid Polym. Sci., 2008, 286, 1663–1666 CAS.
- S. Hu, J. H. Malpert, X. Yang and D. C. Neckers, Polymer, 2000, 41, 445–452 CrossRef CAS.
- A. Goto, J. C. Scaiano and L. Maretti, Photochem. Photobiol. Sci., 2007, 6, 833–835 CAS.
- Y. Guillaneuf, D. Bertin, D. Gigmes, D. L. Versace, J. Lalevee and J. P. Fouassier, Macromolecules, 2010, 43, 2204–2212 CrossRef CAS.
- D. L. Versace, J. Lalevee, J. P. Fouassier, D. Gigmes, Y. Guillaneuf and D. Bertin, J. Polym. Sci., Part A: Polym. Chem., 2010, 48, 2910–2915 CrossRef CAS.
- E. Yoshida, Colloid Polym. Sci., 2010, 288, 1639–1643 CAS.
- Y. Guillaneuf, D. L. Versace, D. Bertin, J. Lalevee, D. Gigmes and J.-P. Fouassier, Macromol. Rapid Commun., 2010, 31, 1909–1913 CrossRef CAS PubMed.
- L. Marx and P. Hemery, Polymer, 2009, 50, 2752–2761 CrossRef CAS PubMed.
- M. Mazarin, M. Girod, S. Viel, T. N. T. Phan, S. R. A. Marque, S. Humbel and L. Charles, Macromolecules, 2009, 42, 1849–1859 CrossRef CAS.
- P. Brémond and S. R. A. Marque, Chem. Commun., 2011, 47, 4291–4293 RSC.
- S. Marque, H. Fischer, E. Baier and A. Studer, J. Org. Chem., 2001, 66(4), 1146–1156 CrossRef CAS PubMed.
- R. P. N. Veregin, P. G. Odell, L. M. Michalak and M. K. Georges, Macromolecules, 1996, 29, 4161–4163 CrossRef CAS.
- P. G. Odell, R. P. N. Veregin, L. M. Michalak and M. K. Georges, Macromolecules, 1997, 30, 2232–2237 CrossRef CAS.
- T. J. Conolly and J. C. Scaiano, Tetrahedron Lett., 1997, 38, 1133 CrossRef.
- P. Brémond, A. Koïta, S. R. A. Marque, V. Pesce, V. Roubaud and D. Siri, Org. Lett., 2012, 14, 358–361 CrossRef PubMed.
- M. V. Edeleva, I. A. Kirilyuk, I. F. Zhurko, D. A. Parkhomenko, Y. P. Tsentalovich and E. G. Bagryanskaya, J. Org. Chem., 2011, 76, 5558–5573 CrossRef CAS PubMed.
- E. Bagryanskaya, P. Brémond, M. Edeleva, S. R. A. Marque, D. Parkhomenko, V. Roubaud and D. Siri, Macromol. Rapid Commun., 2012, 33, 152–157 CrossRef CAS PubMed.
- G. Audran, P. Brémond, S. R. A. Marque and G. Obame, J. Org. Chem., 2012, 77, 9634–9640 CrossRef CAS PubMed.
- S. S. Kelkar and T. M. Reineke, Bioconjugate Chem., 2011, 22, 1879–1903 CrossRef CAS PubMed.
- G. Audran, P. Brémond, J.-M. Franconi, S. R. A. Marque, P. Massot, P. Mellet, E. Parzy and E. Thiaudière, Org. Biomol. Chem., 2014, 12, 719–723 CAS.
- J. Wang and J. Yi, Cancer Biol. Ther., 2008, 7, 1875–1884 CrossRef CAS.
- D. Moncelet, P. Voisin, N. Koonjoo, V. Bouchaud, G. Audran, P. Massot, E. Parzy, G. Audran, J.-M. Franconi, E. Thiaudière, S. R. A. Marque, P. Brémond and P. Mellet, Mol. Pharmaceutics Search PubMed , submitted.
- P. Massot, E. Parzy, L. Pourtau, P. Mellet, S. Marque, J.-M. Franconi and E. Thiaudière, Contrast Media Mol. Imaging, 2012, 7, 45–50 CrossRef CAS PubMed.
- E. Parzy, V. Bouchaud, P. Massot, P. Voisin, N. Koonjoo, D. Moncelet, J.-M. Franconi, E. Thiaudière and P. Mellet, PLoS One, 2013, 8, e57946 CAS.
- H. Otsuka, K. Aotani, Y. Higaki and A. Takahara, Chem. Commun., 2002, 2838–2839 RSC.
- C. Yuan, M. Z. Rong, M. Q. Zhang, Z. P. Zhang and Y. C. Yuan, Chem. Mater., 2011, 23, 5076–5081 CrossRef CAS.
- Z. P. Zhang, M. Z. Rong, M. Q. Zhang and C. Yuan, Polym. Chem., 2013, 4, 4648–4654 RSC.
- B. Schulte, M. Tsotsalas, M. Becker, A. Studer and L. De Cola, Angew. Chem., Int. Ed., 2010, 49, 6881–6884 CrossRef CAS PubMed.
- Registration, Evaluation, Authorisation, and Restriction of Chemical Substances (REACH), EC, 1907/2006, http://eur.lex.europa.eu/LexUriServ/LexUriServ.do?uri=CELEX:32006R1907:en:NOT, accessed Sept 19, 2013.
Footnotes |
† Since the late 1960s, the formation of alkoxyamines via the cross-coupling reaction between alkyl radicals and nitroxides has been well known. See ref. 11 and references therein. |
‡ This term was coined by Daikh et al. See ref. 20. |
§ It has taken researchers two decades to confirm the importance of each stage and each step on the fate of NMP experiments. See ref. 26. |
¶ Several preliminary attempts to develop Nitroxide-Mediated Photopolymerization were performed during this decade. See ref. 60–62 as examples. |
|| Alkoxyamines investigated in ref. 70–73 did not carry protonable sites except the strongly acidic N atom of the C–ON bond which can be protonated only under drastic conditions, as in ref. 68. |
** At 200 °C, many other processes of degradation compete with the C–ON bond homolysis. |
†† The issue of Account of Chemical Research published in September 2011 is devoted to Theranostics. |
|
This journal is © The Royal Society of Chemistry 2014 |
Click here to see how this site uses Cookies. View our privacy policy here.