DOI:
10.1039/C4CC00773E
(Communication)
Chem. Commun., 2014,
50, 4319-4321
Two C2-symmetric chelating P2-bisphosphazene superbases connected via a binaphthyl backbone – synthesis, structural features and preparation of a cationic alkyl aluminum complex†
Received
28th January 2014
, Accepted 26th February 2014
First published on 26th February 2014
Abstract
Two P2-phosphazenyl groups were linked via a C2-symmetric binaphthyl backbone resulting in two novel chiral superbases with dimethylamino and pyrrolidino substituents. We investigated their basic properties and coordination chemistry towards a cationic alkyl aluminum fragment. The outstanding basicity of the chiral tetrasphosphazenes presented herein leads to interesting perspectives for application in asymmetric Brønsted base catalysis.
Chiral amines play a very important role in asymmetric catalysis – especially with regard to extensive scientific activities in the field of organocatalysis during the past decade.1 However, due to their low basicity the role of chiral amines in enantioselective reactions in which they act as Brønsted-bases is strongly limited to comparably acidic substrates. Thus, chiral representatives of all classes of organic superbases such as amidines,2 guanidines,3 azaphosphatranes,4 proton sponges5 or phosphazenes6 have been prepared and investigated with respect to their applicability to asymmetric synthesis.7 This renaissance of well-established concepts of superbasicity becomes manifest in a cluster on superbases published in Synlett quite recently.8 So far, the class of chiral guanidines is the most advanced with respect to its structural variety and utilization in enantioselective reactions. The area has already been the subject of review articles by Ishikawa and Tan.9 Chiral guanidines were applied to asymmetric Michael addition, silylation, nucleophilic epoxidation and TMS cyanation reactions.3a,b Taking into consideration that the pKBH+ values of organic superbases range from 25 to about 45 on the acetonitrile scale, it must be stated that chiral guanidines presented to date only populate the very low end of the basicity scale (pentamethylguanidine: pKBH+ (MeCN) = 25.0010). Extension of the synthetic scope of chiral superbases requires the design of compounds with higher pKBH+ values by making use of the high intrinsic basicity of phosphazenes with multiple PN units or the combination of several superbasic features in one molecule. In this context Terada et al. recently published helically chiral spirocyclic P3 phosphazenes and a series of highly basic chiral guanidinophosphazenes that were used in enantioselective amination reactions of ketones.11 Raab12 and Himmel et al.13 linked two guanidinyl moieties via a C2-symmetric binaphthyl backbone allowing the formation of an energetically favorable [N–H⋯N] hydrogen bond after protonation. Since we have thoroughly studied the basicity-enhancing effect of proton chelation,14 we were interested to combine this proton pincer ligand concept with a chiral binaphthyl skeleton to achieve a unique interaction of two amino-substituted Schwesinger-P2 bases in a chiral environment. A related bis(monophosphazene) with two PPh3 units was prepared by Reetz et al. in 1998, but the electron-withdrawing triphenylphosphonio substituents were not able to grant a high basicity or even superbasicity with a pKBH+ value (MeCN) > 25.00.15
We chose a Kirsanov condensation to achieve the linkage between two P2 fragments and the aromatic diamine. The required bromophosphonium bromide precursors 1 and 2 were prepared by the bromination of the literature known P(III) compounds that were accessible via three-step procedures from tris(dimethylamino) phosphane and tris(pyrrolidino)phosphane.14b,17,18 The reaction of (S)-(–)-1,1′-binaphthyl-2,2′-diamine with the bromophosphonium bromides in the presence of the auxiliary base triethylamine and subsequent deprotonation with metal bis(trimethylsilyl)amides gave the two chiral tetraphosphazenes 3 and 4. They were characterized via1H, 13C and 31P spectroscopy, ESI mass spectrometry, elemental analysis and in the case of the dimethylamino-substituted species also X-ray crystallographic analysis (Fig. 1). The two independent molecules found in the asymmetric unit reveal a large distance of 3.98(1) and 4.10(1) Å between the two basicity centers. Unlike in proton sponges, their N-centered lone pairs avoid each other by suitable torsion of the two naphthalene moieties around the axial carbon–carbon bond. Hence, no steric strain in the aromatic backbones or repulsion of the lone pairs of the nitrogen atoms destabilizing the free base form of tetraphosphazene is observed.
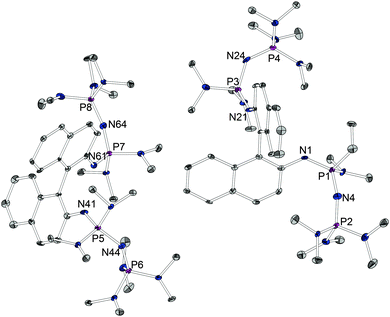 |
| Fig. 1 Molecular structure of 3 (ellipsoids with 30% probability). Hydrogen atoms are omitted for clarity. Selected bond lengths/Å and angles/°: d(N1⋯N21) 3.98(1), d(N1–P1) 1.567(6), d(P1–N4) 1.593(7), d(N4–P2) 1.536(7), d(N21–P3) 1.561(6), d(P3–N24) 1.627(7), d(N24–P4) 1.545(7), d(N41⋯N61) 4.10(1), d(N41–P5) 1.557(6), d(P5–N44) 1.609(6), d(N44–P6) 1.549(6), d(N61–P7) 1.542(7), d(P7–N64) 1.596(6), d(N64–P8) 1.539(7).16 | |
Protonation of the two tetraphosphazenes was studied with p-toluenesulfonic acid. It is accompanied by a low-field shift of the signals in the 31P NMR spectrum and an increase in the 2J(P,P) coupling constants. In the case of the pyrrolidino-substituted species, the acidic proton exhibits a triplet with a 2J(P,H) coupling constant of 7.4 Hz indicating interaction with two phosphorous atoms and thus the formation of an intramolecular [N–H⋯N] hydrogen bond.
The pKBH+ values were determined via NMR titration experiments versus bisphosphazene proton sponges.20 As expected, 4 exhibits a higher pKBH+ value of 30.8 on the acetonitrile scale than its dimethylamino-substituted counterpart 3 which shows a pKBH+ value of 29.3. These results also suggest the energetically favorable chelation of the acidic proton since the basicity of 4 exceeds the pKBH+ value of the non-chelating naphthalene-substituted P2 Schwesinger base (pyr)P2-1-Naph (pKBH+ (MeCN) (calc.) = 26.0)14b by nearly five orders of magnitude. For Terada's chiral guanidinophosphazenes pKBH+ values (THF) close to 26.8 were estimated.11
The coordination behavior of proton sponges21 or other chelating superbases towards electrophiles other than protons has attracted considerable scientific interest.13,22 However, whereas metal complexes of macrocyclic phosphazenes have been reported,23 the coordination chemistry of classical open chain Schwesinger superbases is widely unexplored.24 We were interested to demonstrate the use of the title compounds not only as a base but also as a chiral donor ligand towards Lewis acids of catalytic interest. The reaction of 4 with two equivalents of trimethylaluminum resulted in the asymmetric dissociation and formation of [4-AlMe2]+[AlMe4]− (Scheme 1) which could be investigated concerning its structural features (Fig. 2). An angle of 69.3(9)° is observed between the two naphthalene planes. The cationic fragment [AlMe2]+ is chelated by the two basic nitrogen atoms showing an N–Al–N angle of 98.3(2)° and a non-bonding N⋯N distance of 2.931(6) Å.
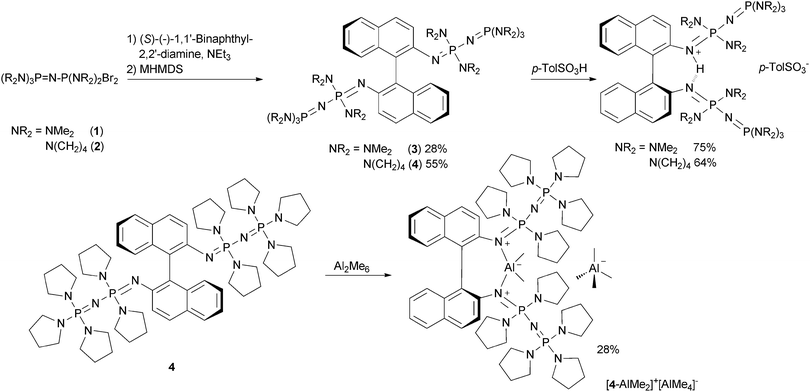 |
| Scheme 1 Preparation of the chiral tetraphosphazenes 3 and 4via a Kirsanov reaction, protonation with para-toluenesulfonic acid and reaction of 4 with trimethylaluminum. | |
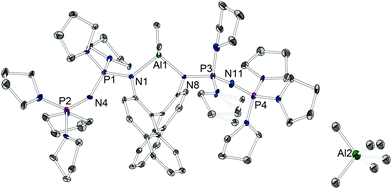 |
| Fig. 2 Molecular structure of [4-AlMe2]+[AlMe4]− (ellipsoids with 30% probability). The disorder in the [AlMe4]− anion is not displayed. Hydrogen atoms are omitted for clarity. Selected bond lengths/Å and angles/°: d(N1–Al1) 1.938(5), d(N1–P1) 1.633(5), d(P1–N4) 1.582(5), d(N4–P2) 1.558(4), d(N8–Al1) 1.938(5), d(N8–P3), d(P3–N11) 1.576(5), d(N11–P4) 1.548(5), N1–Al1–N8 98.3(2).19 | |
In the state of the art the concept of Schwesinger polyphosphazene superbases has not been extended to chelating chiral representatives, favorably with a rigid backbone. Furthermore, Schwesinger polyphosphazene bases have not been used as superstrong chelating neutral donor ligands in coordination chemistry and catalysis. In this respect the chiral tetraphosphazenes communicated herein add valuable perspectives to the highly topical field of chiral superbases with expected applications as enantioselective organocatalysts with an extraordinarily high Brønsted-basicity and as chiral superdonor ligands for cationic metal complexes of catalytic interest.
We thank Fabian Schröder and Lars Finger for their valuable support with crystal structure refinement. Financial support by the Fonds der Chemischen Industrie (doctoral scholarship for J.K.) is gratefully acknowledged.
Notes and references
-
(a) F. Fache, E. Schulz, M. L. Tommasino and M. Lemaire, Chem. Rev., 2000, 100, 2159 CrossRef CAS PubMed;
(b) S. France, D. J. Guerin, S. J. Miller and T. Lectka, Chem. Rev., 2003, 103, 2985 CrossRef CAS PubMed;
(c) J. Seayad and B. List, Org. Biomol. Chem., 2005, 3, 719 RSC;
(d) H. Pellissier, Tetrahedron, 2007, 63, 9267 CrossRef CAS;
(e) L.-W. Xu, J. Luo and Y. Lu, Chem. Commun., 2009, 1807 RSC;
(f) T. Kano and K. Maruoka, Bull. Chem. Soc. Jpn., 2010, 83, 1421 CrossRef CAS.
-
(a) J. Clews, A. D. M. Curtis and H. Malkin, Tetrahedron, 2000, 56, 8735 CrossRef CAS;
(b) C. Dauwe and J. Buddrus, Synthesis, 1995, 171 CrossRef CAS.
-
(a) T. Isobe, K. Fukuda, Y. Araki and T. Ishikawa, Chem. Commun., 2001, 243 RSC;
(b) T. Ishikawa and T. Isobe, Chem.–Eur. J., 2002, 8, 552 CrossRef CAS PubMed;
(c) A. Castiglia, H. El Sehrawi, T. Orbegozo, D. Spitzner, B. Claasen, W. Frey, W. Kantlehner and V. Jäger, Z. Naturforsch., B: J. Chem. Sci., 2012, 67, 337 CrossRef CAS.
-
(a) X. Liu, P. Ilankumaran, I. A. Guzei and J. G. Verkade, J. Org. Chem., 2000, 65, 701 CrossRef CAS;
(b) J. S. You, A. E. Wroblewski and J. G. Verkade, Tetrahedron, 2004, 60, 7877 CrossRef CAS;
(c) K. Ishihara, Y. Karumi, S. Kondo and H. Yamamoto, J. Org. Chem., 1998, 63, 5692 CrossRef CAS.
-
(a) R. W. Alder, J. Am. Chem. Soc., 2005, 127, 7924 CrossRef CAS PubMed;
(b) A. Singh, S. Chakraborty and B. Ganguly, Eur. J. Org. Chem., 2006, 4938 CrossRef CAS;
(c) J.-P. Mazaleyrat and K. Wright, Tetrahedron Lett., 2008, 49, 4537 CrossRef CAS;
(d) G. Brancatelli, D. Drommi, G. Femino, M. Saporita, G. Bottari and F. Faraone, New J. Chem., 2010, 34, 2853 RSC.
-
(a) J. M. Brunel, O. Legrand, S. Reymond and G. Buono, J. Am. Chem. Soc., 1999, 121, 5807 CrossRef CAS;
(b) M. Alajarín, C. López-Leonardo and J. Berná, Tetrahedron, 2006, 62, 6190 CrossRef;
(c) U. Köhn, M. Schulz, A. Schramm, W. Günther, H. Görls, S. Schenk and E. Anders, Eur. J. Org. Chem., 2006, 4128 CrossRef.
-
T. Ishikawa, in Superbases for Organic Synthesis: Guanidines, Amidines and Phosphazenes and Related Organocatalysts, ed. T. Ishikawa, John Wiley & Sons Ltd., Chichester, UK, 2009 Search PubMed.
- T. Ishikawa and L. M. Harwood, Synlett, 2013, 2507 CrossRef CAS.
-
(a) T. Ishikawa and T. Kumamoto, Synthesis, 2006, 737–752 CrossRef CAS;
(b) D. Leow and C.-H. Tan, Synlett, 2010, 1589 CAS.
-
(a) R. Schwesinger, J. Willaredt, H. Schlemper, M. Keller, D. Schmitt and H. Fritz, Chem. Ber., 1994, 127, 2435 CrossRef CAS . The preparation of non-chiral guanidines with considerably higher pKBH+ values was achieved recently: ;
(b) R. A. Kunetskiy, S. M. Polyakova, J. Vavǐík, I. Císaǐová, J. Saame, E. R. Nerut, I. Koppel, I. A. Koppel, A. Kütt, I. Leito and I. M. Lyapkalo, Chem.–Eur. J., 2012, 18, 3621–3630 CrossRef CAS PubMed;
(c) K. Vazdar, R. Kunetskiy, J. Saame, K. Kaupmees, I. Leito and U. Jahn, Angew. Chem., 2014, 126, 1459–1462 (
Angew. Chem., Int. Ed.
, 2014
, 53
, 1435–1438
) CrossRef . Those examples could serve as blueprints for powerful chiral superbases.
-
(a) M. Terada, K. Goto, M. Oishi, T. Takeda, E. Kwon and A. Kondoh, Synlett, 2013, 2531 CrossRef CAS;
(b) T. Takeda and M. Terada, J. Am. Chem. Soc., 2013, 135, 15306 CrossRef CAS PubMed.
-
V. Raab, Peralkyl Guanidines in Copper Catalysed Oxidative Transformations and Novel Proton Sponges, Dissertation Marburg 2001, Klaus Bielefeld Verlag 2002, ISBN 3-89833-053-2.
- A. Maronna, E. Bindewald, E. Kaifer, H. Wadepohl and H.-J. Himmel, Eur. J. Inorg. Chem., 2011, 1302 CrossRef CAS.
-
(a) V. Raab, E. Gauchenova, A. Merkoulov, K. Harms, J. Sundermeyer, B. Kovačević and Z. B. Maksić, J. Am. Chem. Soc., 2005, 127, 15738 CrossRef CAS PubMed;
(b) J. F. Kögel, B. Oelkers, B. Kovaĉević and J. Sundermeyer, J. Am. Chem. Soc., 2013, 135, 17768 CrossRef PubMed.
- M. T. Reetz, E. Bohres and R. Goddard, Chem. Commun., 1998, 935 RSC.
-
3 crystallizes in the triclinic space group P1 with the unit cell constants a = 10.5097(10) Å, b = 13.3588(14) Å, c = 16.9068(17) Å, α = 87.901(4)°, β = 89.867(4)° and γ = 82.976(4)°. Detailed crystal data and experimental conditions are given in the ESI†.
- A. P. Marchenko, G. N. Koidan, A. M. Pinchuk and A. V. Kirsanov, Zh. Obshch. Khim., 1983, 54, 1774 Search PubMed.
- A molecular structure of 1 is presented in the ESI† (Fig. S1).
- [4-AlMe2]+[AlMe4]− crystallizes in the monoclinic space group P1211 with the unit cell constants a = 10.751(3) Å, b = 33.100(7) Å, c = 10.900(3) Å, α = γ = 90° and β = 116.309(13)°.
- NMR titration experiments were performed in THF due to the low solubility of the free base forms in acetonitrile. 4 competed with HMPN and TPPN for protons while an unpublished iso-propyl-substituted bisphosphazene proton sponge with a pKBH+ value of 30.4 in acetonitrile was used in the case of 3. A linear correlation between the THF and the acetonitrile scale can be assumed by approximation. The procedure is described in ref. 14a.
-
(a) H.-U. Wüstefeld, W. C. Kaska, F. Schüth, G. D. Stuckly, X. Bu and B. Krebs, Angew. Chem., 2001, 113, 3280 (
Angew. Chem., Int. Ed.
, 2001
, 40
, 3182
) CrossRef;
(b)
H.-U. Wuestefeld, W. C. Kaska, G. D. Stucky, F. Schueth and B. Krebs, PCT Int. Appl., WO 2002059134 A1 20020801, 2002 Search PubMed.
-
(a) T. Yamasaki, N. Ozaki, Y. Saika, K. Ohta, K. Goboh, F. Nakamura, M. Hashimoto and S. Okeye, Chem. Lett., 2004, 33, 928 CrossRef CAS;
(b) P. Roquette, A. Maronna, A. Peters, E. Kaifer, H.-J. Himmel, C. Hauf, V. Herz, E.-W. Scheidt and W. Scherer, Chem.–Eur. J., 2010, 16, 1336 CrossRef CAS PubMed;
(c) A. Maronna, E. Bindewald, E. Kaifer, H. Wadepohl and H.-J. Himmel, Eur. J. Inorg. Chem., 2011, 1302 CrossRef CAS.
-
(a) W. C. Marsh, N. L. Paddock, C. J. Stewart and J. Trotter, Chem. Commun., 1970, 1190 RSC;
(b) J. P. O'Brien, R. W. Allen and H. R. Allcock, Inorg. Chem., 1979, 18, 2230 CrossRef.
- M. Sauthier, J. Forniés-Cámer, L. Toupet and R. Réau, Organometallics, 2000, 19, 553 CrossRef CAS.
Footnote |
† Electronic supplementary information (ESI) available: Detailed synthetic procedures and analytical data. CCDC 977138–9771340. For ESI and crystallographic data in CIF or other electronic format see DOI: 10.1039/c4cc00773e |
|
This journal is © The Royal Society of Chemistry 2014 |
Click here to see how this site uses Cookies. View our privacy policy here.