DOI:
10.1039/C4BM00111G
(Paper)
Biomater. Sci., 2014,
2, 1230-1243
Lithium release from β-tricalcium phosphate inducing cementogenic and osteogenic differentiation of both hPDLCs and hBMSCs
Received
7th April 2014
, Accepted 16th May 2014
First published on 5th June 2014
Abstract
It is accepted that the accelerated differentiation of tissue cells on bioactive materials is of great importance to regenerate the lost tissues. It was previously reported that lithium (Li) ions could enhance the in vitro proliferation and differentiation of retinoblastoma cells and endometrium epithelia by activating the Wnt canonical signalling pathway. It is interesting to incorporate Li ions into bioactive ceramics, such as β-tricalcium phosphate (Li-β-TCP), in order to stimulate both osteogenic and cementogenic differentiation of different stem cells for the regeneration of bone/periodontal tissues. Therefore, the aim of this study was to investigate the interactions of human periodontal ligament cells (hPDLCs) and human bone marrow stromal cells (hBMSCs) with Li-β-TCP bioceramic bulks and their ionic extracts, and further explore the osteogenic and cementogenic stimulation of Li-β-TCP bioceramics and the possible molecular mechanisms. The results showed that Li-β-TCP bioceramic disks supported the cell attachment and proliferation, and significantly enhanced bone/cementum-related gene expression, Wnt canonical signalling pathway activation for both hPDLCs and hBMSCs, compared to conventional β-TCP bioceramic disks without Li. The release of Li from Li-β-TCP powders could significantly promote the bone/cementum-related gene expression for both hPDLCs and hBMSCs compared to pure β-TCP extracts without Li release. Our results suggest that the combination of Li with β-TCP bioceramics may be a promising method to enhance bone/cementum regeneration as Li-β-TCP possesses excellent in vitro osteogenic and cementogenic stimulation properties by inducing bone/cementum-related gene expression in both hPDLCs and hBMSCs.
1. Introduction
For bone/periodontal tissue regeneration application, it is generally accepted that the quick differentiation of tissue cells on bioactive materials is of great importance to regenerate the deficient and injured tissues.1–3 To achieve this target, the strategy for applying growth factors to accelerate the differentiation of tissue cells has been widely studied;4,5 however, the usage of growth factors is highly expensive and may have potential health risks.6,7 The third-generation of biomaterials should possess the ability to stimulate the differentiation of stem cells for tissue regeneration.8–11 Increasing studies have shown that bioactive materials with specific bioactive compositions and microstructures can induce the differentiation of stem cells and promote tissue regeneration. For this reason, it is of great significance to prepare proper bioceramics to release inorganic stimulus to stimulate the response of stem cells for bone and periodontal tissue regeneration without the usage of additional growth factors.12,13 It is well known that beta-tricalcium phosphate (β-TCP) is the most conventionally used bioceramics for the regeneration of bone/periodontal tissues due to its high osteoconductivity and degradability; however, there are few reports stating that β-TCP bioceramics can significantly induce the differentiation of stem cells and present the significant osteostimulation for the regeneration of bone/periodontal tissues.14–17 Although β-TCP bioceramics are generally biodegradable and biocompatible, they are still far from optimal for stimulating the osteogenic/periodontal differentiation of stem cells to achieve the optimal healing time of tissue defects.18,19 In the past several years, strontium modified β-TCP bioceramics capable of enhancing the cell viability and differentiation of osteoblast-like cells with improved bioactivity have been prepared for bone regeneration application.20 This suggested that bioactive ion modification of β-TCP bioceramics may be a viable method to stimulate differentiation of mesenchymal stem cells for better tissue regeneration.
Lithium chloride (LiCl) has been used as a mood stabiliser which can stimulate the proliferation of retinoblastoma cells and endometrium epithelia cells via elevated Wnt canonical signalling.21–24 Li can enhance fracture healing in mice, while clinically it reduces the incidence of fractures with increased bone mass and density with increasing accumulated dose of Li.25–27 Its role in fracture healing is further demonstrated by increased β-catenin expression in fractured tissue in patients taking Li.28 Yet, there are some reports that argue against the pharmacological benefit of reducing fracture risks with Li.29 Cohen's findings demonstrated that the clinical data suggested an increase in bone turnover associated with lithium carbonate therapy treatment; however, no distinct effect was observed on the bone density after short and long-term lithium carbonate therapy, indicating that treatment with Li is not associated with increased risk for osteoporosis.30 The results suggest that the biological role of Li ions in bone is not fully clear. Although there are several studies showing that Li ions could regulate the apatite mineralization of bioglass and enhance osteoblast proliferation of hydroxyapatite,31–33 the effect of Li-containing bioceramics on the differentiation of different tissue cells, such as hBMSCs and hPDLCs, as well as the related molecular mechanisms are unclear. It is believed that multipotent hBMSCs are involved in bone and periodontal defect healing.34 As an attractive cell source for tissue engineering, hBMSCs are easily available, highly proliferative and multipotent to differentiate into osteoblasts, chondrocytes, adipose cells, ligament cells, and neural cells.35–39 hPDLCs are considered to be able to regenerate the alveolar bone, periodontal ligament and cementum.40 Activation of osteogenic/cementogenic potential of both hPDLCs and hBMSCs by bioactive materials would greatly accelerate the new bone and cementum formation in the bone/periodontal defect site.
Therefore, the main aim of this study was to prepare Li-containing β-TCP bioceramics in an effort to improve their osteo/cementogenic capabilities compared to conventionally used β-TCP bioceramics, and to further elucidate the involved mechanism in the activation of the pro-osteo/cementogenic Wnt canonical signalling pathway in hPDLCs and hBMSCs by release of Li ions from bioceramics. To achieve this aim, the effect of both Li-β-TCP bioceramic disks and their ionic products on the differentiation of hPDLCs and hBMSCs has been systematically explored in comparison with conventional β-TCP bioceramics.
2. Experimental section
2.1. Preparation and characterization of 5Li-β-TCP bioceramic powders and disks
The Li-containing β-TCP (5Li-β-TCP) powders with the chemical composition of (Li0.10Ca0.95)3(PO4)2 were synthesized by a chemical precipitation method using Ca(NO3)2·4H2O, (NH4)2HPO4 and lithium chloride (LiCl) as starting materials, in which 5% molar Ca was substituted by Li. The solution containing 0.5 M Ca(NO3)2 and LiCl in the desired molar ratio was added dropwise to 0.5 M (NH4)2HPO4 solution while stirring to produce the target (Ca + Li)/P ratio of 1.5 (stoichiometric for β-TCP). The pH of the solution was maintained between 7.5 and 8 during precipitation with the aid of ammonia. After the precipitation, the solution was aged overnight, washed sequentially in distilled water and anhydrous ethanol, dried at 60 °C for 24 h and finally calcined at 800 °C for 3 h. Pure β-TCP powders were synthesized by a similar method without adding LiCl and used as controls. The synthesized powders were observed by scanning electron microscopy (SEM, FEI Company, USA). The real Li contents in Li-β-TCP powders were tested by inductive coupled plasma atomic emission spectrometry (ICP-AES, Perkin-Elmer Optima 7000DV).
To prepare ceramic discs, the synthesized 5Li-β-TCP powders were uniaxially pressed at 10 MPa in a mould of Φ10, using 6% polyvinyl alcohol as a binder. The green compacts were subsequently sintered in air at 1100 °C for 3 h at a heating rate of 2 °C min−1. Pure β-TCP ceramic discs were prepared by a similar method and used as controls. The crystal phase and surface morphology of the sintered ceramic discs were characterized by X-ray diffraction (XRD, Geigerflex, Rigaku, Japan) and SEM.
2.2. The cell morphology of hPDLCS and hBMSCs on 5Li-β-TCP bioceramic disks
Human periodontal ligament cells (hPDLCs) and human bone marrow stromal cells (hBMSCs) were isolated and cultured according to our previously published protocols.10,41 The ethics approval of hPDLCs and hBMSCs in this study was granted by the Human Ethics Committee of Queensland University of Technology and The Prince Charles Hospital, following informed consents taken from all the participants. While hPDLCs were isolated from the teeth of healthy patients (18–25 years old) undergoing third molar extraction surgery, hBMSCs were isolated from patients (mean age of 65 years) undergoing elective knee and hip replacement surgery. hPDLCs and hBMSCs were seeded on 5Li-β-TCP ceramic disks at the initial seeding density of 105 cells per disk in 48-well plates and cultured in osteogenic induction medium with the supplement of 0.1 μM dexamethasone (Sigma-Aldrich, Australia), 10 mM β-glycerophosphate (Sigma-Aldrich, Australia) and 50 μg mL−1 ascorbate-2-phosphate (Sigma-Aldrich, Australia). In this study, all experiments were conducted in triplicate. Thus, the cells cultured on three β-TCP ceramic disks were used as controls in this study; while gene expression was assessed from samples harvested from 6 ceramic disks of both 5Li-β-TCP and β-TCP groups.
The cell morphology on the disks was observed by SEM. The cell samples were fixed in 2.5% glutaraldehyde solution and then dehydrated with graded ethanol series (30, 50, 70, 90, and 100%) and dried with hexamethyldisilizane (HMDS). Then, the disks were sputter-coated with gold and observed by SEM (Quanta™ 200) for the morphological characteristics of hPDLCs and hBMSCs on two ceramic disks.
Confocal laser scanning microscopy (CLSM) was carried out to further determine the morphology of the cell skeleton and nuclei of hPDLCs and hBMSCs on the two ceramic disks from high-resolution optical images. After 1 and 7 days culture, the cell-ceramic complex was fixed with a 4% paraformaldehyde (PFA) solution for 30 min at room temperature and then was permeabilized with a 0.2% Triton X-100/PBS solution for 5 min after washing with PBS once. Samples were then incubated for 1 h with 0.5% BSA/PBS containing 0.8 U mL−1 fluorescein isothiocyanate (FITC, Sigma-Aldrich, Australia) and 5 μg mL−1 DAPI (Sigma-Aldrich, Australia). The cells were visualized with a Leica SP5 confocal microscope (Leica Microsystems, Germany).
To investigate whether pH values of the medium will affect the cell proliferation and differentiation, the culture medium was collected for both hBMSCs and hPDLCs after 7 days culture on 5Li-β-TCP and β-TCP bioceramic disks. Then the pH values of the medium were tested using a pH meter (Thermo Scientific, Australia).
2.3. Cell proliferation and alkaline phosphatase (ALP) activity assay for hPDLCs and hBMSCs on 5Li-β-TCP bioceramic disks
For the cell proliferation assay, the 3-(4,5-dimethylthiazol-2-yl)-2,5-diphenyltetrazolium bromide (MTT) assay was performed in triplicate as described in our previous study protocol.41,42 The formazan crystals were prepared by adding 0.5 mg mL−1 MTT solution (Sigma-Aldrich) for 4 h and then solubilising with dimethyl sulfoxide (DMSO, Sigma-Aldrich, Australia). The absorbance of formazan–DMSO solution was measured at λ = 495 nm using a SpectraMax Microplate Reader (Molecular Devices, Inc., USA).
After 7 and 14 days, the relative ALP activity was determined for both cell lines cultured on 5Li-β-TCP ceramic disks using previous protocols.9 At each time point, the cells were lysed in 200 μL of 0.2% Triton X-100 and then mixed with ALP working solution according to the manufacturer's protocol (QuantiChrom™ alkaline phosphatase assay kit, BioAssay Systems, USA). The optical density (OD) value was measured at 405 nm on a plate reader. The relative ALP activity was calculated as the changed OD values divided by the reaction time and the total protein content was measured using the bicinchoninic acid protein assay kit (Thermo Fisher Scientific, Australia).
2.4. Real-time quantitative (RT-qPCR) analysis of cementogenic/osteogenic gene expression and signalling pathway for hPDLCs and hBMSCs on 5Li-β-TCP bioceramic disks
Total RNA was extracted from the cells on 5Li-β-TCP and β-TCP ceramic disks using the TRIzol® Reagent (Ambion®, Life Technologies Pty Ltd, Australia). RT-qPCR was performed on osteogenic-related markers of alkaline phosphatase (ALP) and osteocalcin (OCN), cementum-specific markers of cementum protein 1 (CEMP1) and cementum attachment protein (CAP), and Wnt canonical-related genes of Wingless-3A (WNT3A) and axis inhibition protein 2 (AXIN2) as shown in Table 1. All reactions were conducted in triplicate for three independent experiments. The mean cycle threshold (Ct) value of target genes was normalized against the Ct value of the house-keeping gene glyceraldehyde-3-phosphate-dehydrogenase (GAPDH) and 18S ribosomal RNA (18S rRNA) and then the relative expression was calculated by the following formula: 2−(normalized average Cts) × 104.
Table 1 Primer pairs used in qRT-PCR analysis
Gene |
Forward primer |
Reverse primer |
ALP |
5′ TCAGAAGCTAACACCAACG 3′ |
5′ TTGTACGTCTTGGAGAGGGC 3′ |
OCN |
5′ GCAAAGGTGCAGCCTTTGTG 3′ |
5′ GGCTCCCAGCCATTGATACAG 3′ |
CEMP1 |
5′ GGGCACATCAAGCACTGACAG 3′ |
5′ CCCTTAGGAAGTGGCTGTCCAG3′ |
CAP |
5′ CTGCGCGCTGCACATGG 3′ |
5′ GCGATGTCGTAGAAGGTGAGCC 3′ |
WNT3A |
5′ TGGACAAAGCTACCAGGGAGT 3′ |
5′ CCCACCAAACTCGATGTCCTC 3′ |
AXIN2 |
5′ CCCCAAAGCAGCGGTGC 3′ |
5′ GCGTGGACACCTGCCAG 3′ |
CTNNB |
5′ GCTACTGTTGGATTGATTCGAAATC 3′ |
5′ CCCTGCTCACGCAAAGGT 3′ |
GAPDH |
5′ TCAGCAATGCCTCCTGCAC 3′ |
5′ TCTGGGTGGCAGTGATGGC 3′ |
18s |
5′ TTCGGAACTGAGGCCATGAT 3′ |
5′ CGAACCTCCGACTTCGTTC 3′ |
2.5. Ionic concentration analysis for the cell culture medium after culturing with 5Li-β-TCP bioceramic disks
To investigate the ion release from 5Li-β-TCP ceramic disks, the cell culture medium was collected after culturing of hPDLCs and hBMSCs after a fixed culture time; then the concentrations of Li, Ca and P ions in the medium were measured by inductive coupled plasma atomic emission spectrometry (ICP-AES, Perkin-Elmer Optima 7000DV).
2.6. The effects of ionic extracts from 5Li-β-TCP powders on the proliferation and cementum/bone-related gene expression for hPDLCs and hBMSCs
The effect of ionic extracts from 5Li-β-TCP powders was further investigated on the cell proliferation, and osteogenic/cementogenic-related gene expression of hPDLCs and hBMSCs.43 According to International Standard Organization (ISO/EN) 10993-5, the dissolution extracts were prepared by soaking 5Li-β-TCP powders in serum-free DMEM at a concentration of 200 mg mL−1. Then serial dilutions of extracts (200, 50 and 6.25 mg mL−1) were prepared after sterilization using a 0.2 μm filter in the following studies. The ionic concentrations of Ca, P and Li in the graded extracts were measured by ICP-AES.
After hPDLCs and hBMSCs were cultured with the β-TCP and 5Li-β-TCP extracts, respectively, the cell proliferation assay was performed as described in section 2.3. The RT-qPCR analysis for the cementogenic/osteogenic gene expression was performed as described in section 2.4. All experiments were conducted in triplicate.
2.7. Statistical analysis
The statistical analysis was performed using one-way ANOVA followed by Tukey's HSD test, a post-hoc test. A p-value <0.05 was considered statistically significant.
3. Results
3.1. Characterization of 5Li-β-TCP bioceramic disks
SEM analysis showed the particle size of 5Li-β-TCP and β-TCP powders to be around 287 ± 46 nm and 282 ± 47 nm, respectively. There is no significant difference in the particles size of two kinds of powders (Fig. 1a and b). SEM analysis showed that both the prepared 5Li-β-TCPand β-TCP bioceramics were fully sintered with dense surface microstructure and clear crystal boundaries (Fig. 1c and d). The crystal size of 5Li-β-TCP bioceramics is generally smaller than that of β-TCP bioceramics (Fig. 1c and d). XRD analysis showed that 5Li-β-TCP ceramics exhibits the same characteristic peaks as β-TCP ceramics with the pure β-TCP crystal phase (JCPD 09-0169) (Fig. 1e). The molar concentration of Li in β-TCP is around 1.83%.
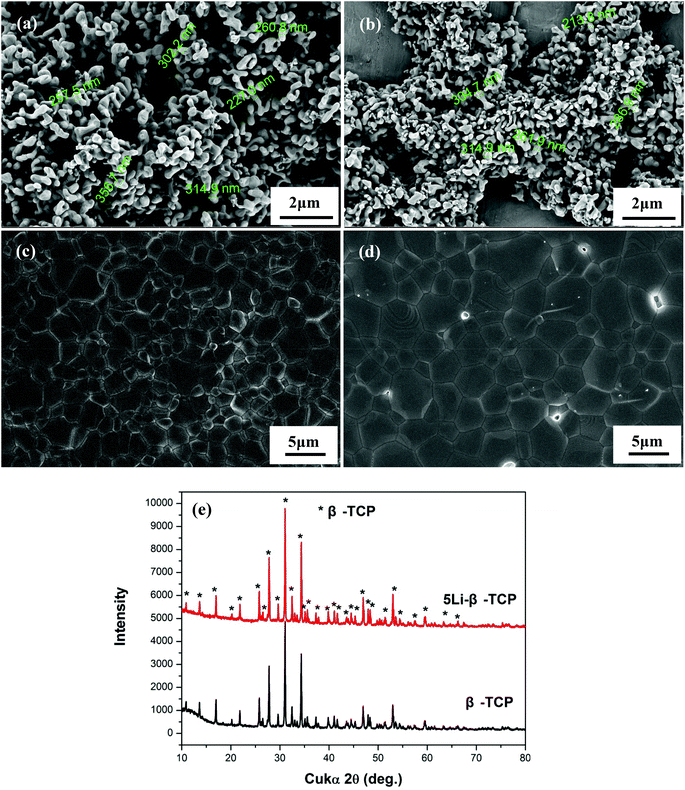 |
| Fig. 1 SEM analysis for 5Li-β-TCP (a) and β-TCP (b) powders, 5Li-β-TCP (c) and β-TCP (d) bioceramic disks. The XRD analysis (e) for 5Li-β-TCP and β-TCP bioceramic disks. The particle size of 5Li-β-TCP and β-TCP powders is around 287 ± 46 nm and 282 ± 47 nm, respectively. There is no significant difference in the particle size of two kinds of powders (a and b). | |
3.2. The cell morphology, proliferation and ALP activity of hPDLCs and hBMSCs on 5Li-β-TCP bioceramic disks
The cell morphology of hPDLCs and hBMSCs was analysed by SEM after 7 days culture on 5Li-β-TCP and β-TCP bioceramic disks. Both hPDLCs and hBMSCs attached and spread well on the surface of two ceramic disks with close contact with ceramics at day 7 (Fig. 2). We conducted confocal laser scanning microscopy imaging to further confirm the cell morphology on the surface of two bioceramic disks, and it was noted that both hPDLCs and hBMSCs were healthy and attached on the surface of disks very well after culturing on two bioceramic disks for 1 and 7 days (Fig. 3). The CLSM results also demonstrated that 5Li-β-TCP bioceramic disks could enhance cell proliferation compared to β-TCP bioceramic disks for both hPDLCs and hBMSCs at both day 1 and 7 (Fig. 3).
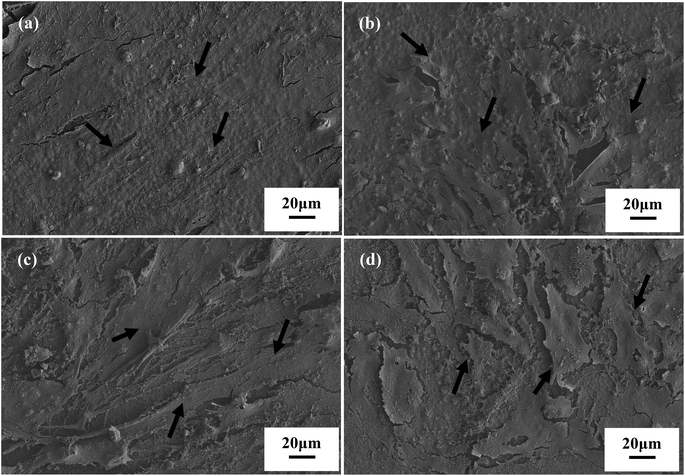 |
| Fig. 2 The SEM analysis for the cell morphology of (a) hPDLCs on 5Li-β-TCP, (b) hBMSCs on 5Li-β-TCP, (c) hPDLCs on β-TCP and (d) hBMSCs on β-TCP bioceramic disks after culturing for 7 days. The arrows indicate the represented cells. | |
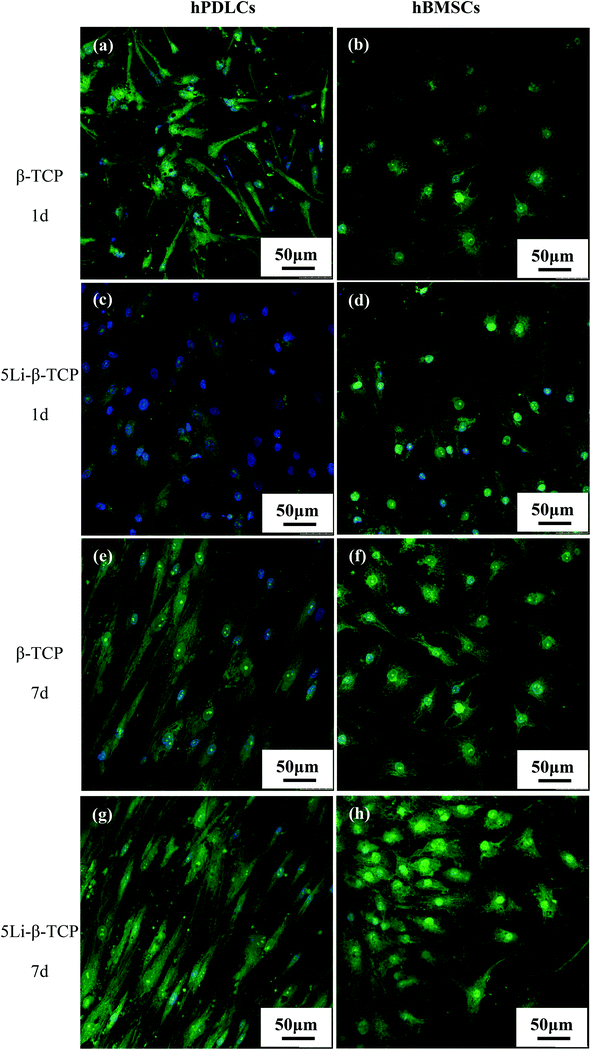 |
| Fig. 3 The confocal fluorescence images for hPDLCs (a, e) and hBMSCs (b, f) on β-TCP bioceramics and hPDLCs (c, g) and hBMSCs (d, h) on 5Li-β-TCP bioceramics after culturing for 1, 7 days, respectively. | |
The MTT assay showed that the proliferation of both hPDLCs and hBMSCs increased obviously in a time dependent manner in each group. The proliferation rate of hPDLCs on 5Li-β-TCP bioceramic disks was significantly higher (∼1.2 fold increase) than that on β-TCP ceramic disks at both day 1 and 7 (Fig. 4a). The proliferation of hBMSCs was higher (∼1.3 fold higher) on 5Li-β-TCP than that on β-TCP ceramic disks at day 1 (Fig. 4b).
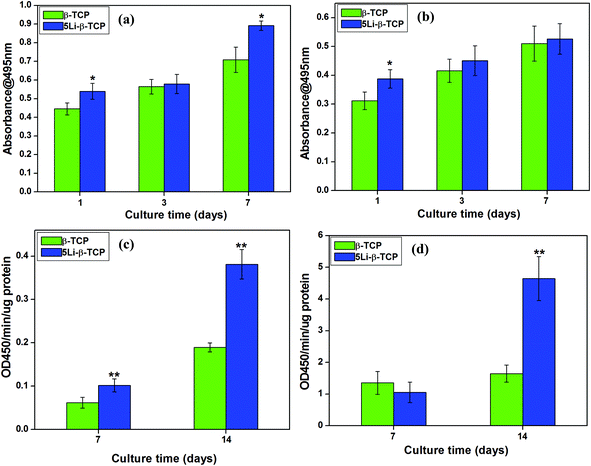 |
| Fig. 4 The cell proliferation and relative ALP activity for hPDLCs (a, c) and hBMSCs (b, d) on β-TCP and 5Li-β-TCP bioceramics. *Significant difference (p < 0.05) for the 5Li-β-TCP group compared to the β-TCP group with respect to the cell proliferation rate. **Significant difference (p < 0.05) for the 5Li-β-TCP group compared to the β-TCP group regarding relative ALP activity. | |
The relative ALP activity of hPDLCs on the 5Li-β-TCP ceramic disks showed a significant increase of around 2 fold compared to the β-TCP group at both 7 and 14 days (p < 0.05, Fig. 4c). Fig. 4d revealed that 5Li-β-TCP ceramic disks enhanced the relative activity of ALP in hBMSCs (∼3 fold increase) compared to the β-TCP group at day 14.
3.3. Cementogenic/osteogenic gene expression and the Wnt-related signalling pathway for hPDLCs and hBMSCs on 5Li-β-TCP bioceramic disks
At day 7, RT-qPCR analysis showed that 5Li-β-TCP ceramics could significantly enhance the osteogenic/cementogenic gene expression for both hPDLCs and hBMSCs compared to β-TCP ceramics (Fig. 5). The 5Li-β-TCP ceramic disks significantly stimulated bone-related gene expression of ALP and OCN (∼3 fold increase for hPDLCs, ∼5 fold enhancement for hBMSCs) and cementum-specific genes of CEMP1 and CAP (∼6 fold increase for hPDLCs, ∼3 fold enhancement for hBMSCs) compared to those of other groups for both hPDLCs and hBMSCs at day 7 (Fig. 5a–h).
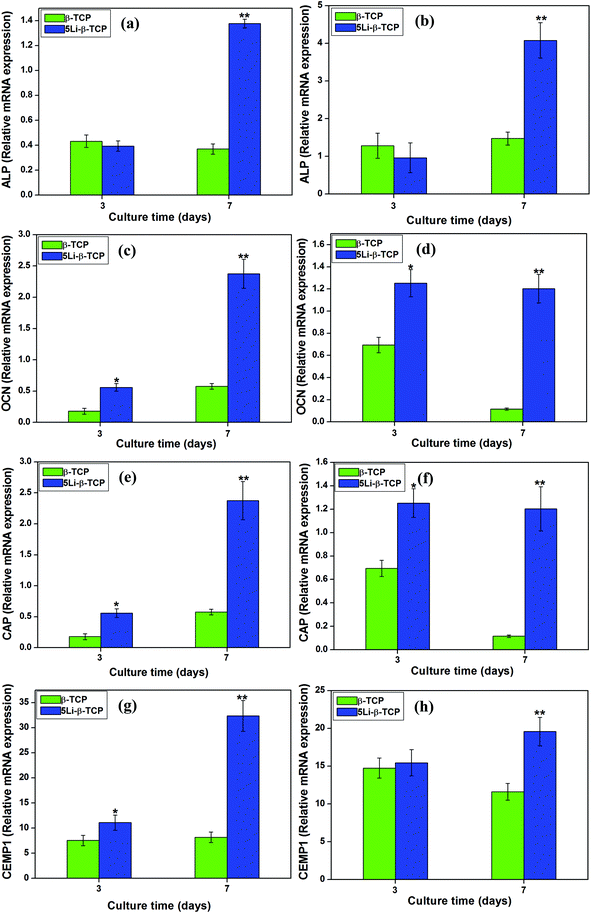 |
| Fig. 5 The bone-related gene expression of ALP, OCN and cementum-specific markers of CAP and CEMP1 for hPDLCs (a, c, e, g) and hBMSCs (b, d, f, h) on β-TCP and 5Li-β-TCP bioceramics. *Significant difference (p < 0.05) for the 5Li-β-TCP group compared to other two groups at day 3. **Significant difference (p < 0.05) for the 5Li-β-TCP group compared to two other groups at day 7. | |
The analysis of the expression of Wnt canonical-related genes such as WNT3A and AXIN2 was performed by RT-PCR after culturing both hPDLCs and hBMSCs on two ceramic disks for 3 and 7 days. The results showed that the expression of WNT3A and AXIN2 genes in both hPDLCs (∼6 fold increase) and hBMSCs (∼4 fold increase) on 5Li-β-TCP bioceramic disks was significantly higher than that on β-TCP ceramic disks at day 7 (Fig. 6a–d).
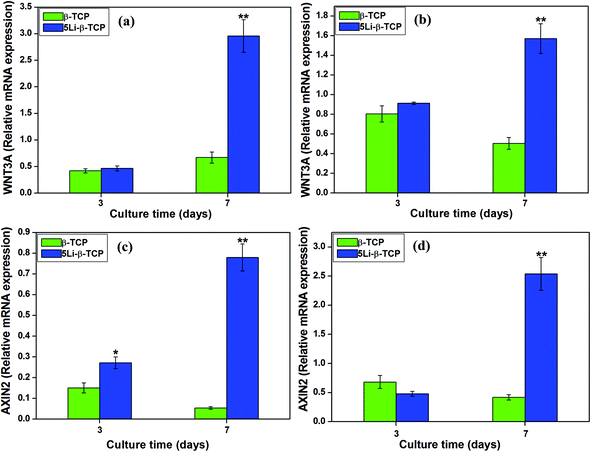 |
| Fig. 6 The WNT canonical-related gene expression of WNT3A and AXIN2 for hPDLCs (a, c) and hBMSCs (b, d) on β-TCP and 5Li-β-TCP bioceramics. *Significant difference (p < 0.05) for 5Li-β-TCP group compared to other two groups at day 3. **Significant difference (p < 0.05) for the 5Li-β-TCP group compared to two other groups at day 7. | |
3.4. Ionic concentration and pH analysis for the cell culture medium after culturing on 5Li-β-TCP bioceramic disks
It was noted that there were no Li ions in DMEM and TCP ceramics only (0 mg mL−1); however, there were some Li ions released from 5Li-β-TCP bioceramic disks when cultured with hPDLCs (0.34 mg mL−1 at day 3, 0.44 mg mL−1 at day 7) and hBMSCs (1.23 mg mL−1 at day 3, 0.89 mg mL−1 at day 7). As expected, the released Li ions from 5Li-β-TCP bioceramic disks are significantly higher than those from β-TCP cultured with hPDLCs (5Li-β-TCP vs. β-TCP groups, p < 0.0001, Table 2) and hBMSCs (5Li-β-TCP vs. β-TCP groups, p < 0.00001, Table 3) at days 3 and 7. Interestingly, the released Li ions from hBMSCs cultured on 5Li-β-TCP disks were about 2 fold higher than those from hPDLCs cultured on 5Li-β-TCP disks (5Li-β-TCP groups from hBMSCs vs. hPDLCs, p < 0.0001). There was no significant difference for P ions between 5Li-β-TCP and β-TCP groups cultured with two cells; however, the concentration of Ca ions for 5Li-β-TCP was slightly lower (5Li-β-TCP groups from hPDLCs vs. hBMSCs, ∼1.3 fold lower, p < 0.0001) than those for β-TCP groups cultured with two cells (Tables 2 and 3). The data as shown in Table 4 demonstrated that the pH value of cell culture medium with hBMSCs was slightly lower (around 0.1 lower) than that of hPDLCs.
Table 2 Ion release of 5Li-TCP ceramic disks in DMEM cultured with hPDLCs
Culture time |
Materials |
Li(mg L−1) |
P(mg L−1) |
Ca(mg L−1) |
3 d |
DMEM |
0 |
323.9 ± 5.15 |
78.03 ± 0.50 |
β-TCP |
0 |
304.1 ± 1.37 |
65.37 ± 0.24 |
5Li-β-TCP |
0.34 ± 0.01 |
301.2 ± 1.17 |
62.58 ± 0.18 |
7 d |
DMEM |
0 |
295.4 ± 0.75 |
75.38 ± 0.26 |
β-TCP |
0 |
280.4 ± 2.05 |
65.12 ± 0.41 |
5Li-β-TCP |
0.44 ± 0.02 |
272 ± 1.22 |
63.14 ± 0.29 |
Table 3 Ion release of 5Li-TCP ceramic disks in DMEM cultured with hBMSCs
Culture time |
Materials |
Li(mg L−1) |
P(mg L−1) |
Ca(mg L−1) |
3 d |
DMEM |
0 |
317 ± 3.2 |
74.94 ± 0.83 |
β-TCP |
0 |
285.6 ± 1.99 |
62.55 ± 0.03 |
5Li-β-TCP |
1.23 ± 0.01 |
281.7 ± 5.77 |
54.89 ± 0.24 |
7 d |
DMEM |
0 |
290.2 ± 1.58 |
67.26 ± 0.81 |
β-TCP |
0 |
269.5 ± 0.70 |
62.59 ± 0.20 |
5Li-β-TCP |
0.89 ± 0.04 |
269.3 ± 0.61 |
57.8 ± 0.44 |
Table 4 The pH values of medium for hPDLCs and hBMSCs cultured on β-TCP and 5Li-β-TCP ceramic disks
|
hPDLCs |
hBMSCs |
β-TCP |
8.02 ± 0.36 |
7.92 ± 0.35 |
5Li-β-TCP |
7.92 ± 0.56 |
7.86 ± 0.32 |
Blank |
7.93 ± 0.45 |
7.82 ± 0.45 |
3.5. The effects of ionic extracts from 5Li-β-TCP powders on the proliferation and bone/cementum-related gene expression of hPDLCs and hBMSCs
The ionic concentrations of Li, Ca and P in the extracts from β-TCP and 5Li-β-TCP powders are shown in Table 5. At the concentration of 200 mg mL−1, it was obvious that the Li concentrations reached 7.42 mg L−1; however, there were no Li ions in β-TCP extracts (Table 5). The concentrations of Ca and P ions in β-TCP and 5Li-β-TCP groups were comparable.
Table 5 The ionic concentrations of the extracts from β-TCP and 5Li-β-TCP powders
Extract concentrations (mg mL−1) |
Materials |
Li(mg L−1) |
P(mg L−1) |
Ca(mg L−1) |
200 mg mL−1 |
β-TCP |
0 |
205.1 ± 3.15 |
55.53 ± 1.05 |
5Li-β-TCP |
7.42 ± 0.05 |
202.2 ± 1.01 |
54.45 ± 1.75 |
50 mg mL−1 |
β-TCP |
0 |
291.3 ± 3.12 |
71.3 ± 2.02 |
5Li-β-TCP |
1.86 ± 0.02 |
290.5 ± 4.23 |
71.0 ± 1.65 |
6.25 mg mL−1 |
β-TCP |
0 |
316.3 ± 5.32 |
75.9 ± 1.36 |
5Li-β-TCP |
0.23 ± 0.01 |
316.4 ± 4.21 |
75.8 ± 0.96 |
As determined by the MTT assay, the cell proliferation rate of both hBMSCs and hPDLCs cultured in the presence of 5Li-β-TCP extracts (∼1.2 fold increase) in a wide range of concentrations (200, 100, 50 and 6.25 mg mL−1) was significantly higher than that cultured with the β-TCP group at day 7 (Fig. 7a and b).
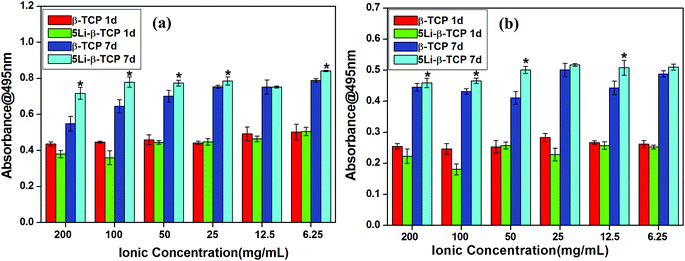 |
| Fig. 7 The proliferation for hPDLCs (a) and hBMSCs (b) after culturing with different concentrations of β-TCP and 5Li-β-TCP powder extracts for 1 and 7 days. *Significant difference (p < 0.05) for the 5Li-β-TCP group compared to the β-TCP group at day 7. | |
Results of bone/cementum-related gene expression analysis of ALP, OCN and CEMP1 showed that the 5Li-β-TCP extracts at all the concentrations enhanced hPDLC osteogenic and cementogenic differentiation compared to the β-TCP extract group at day 7 (>1 fold) (Fig. 8a, c and e). At day 7, the expression of ALP for hBMSCs at 6.25 mg mL−1 of 5Li-β-TCP was about 2 fold higher than that at 6.25 mg mL−1 of β-TCP (Fig. 8b). The expression of OCN and CEMP1 in both hPDLCs and hBMSCs was enhanced by 5Li-β-TCP extracts at all the concentrations compared with that in the β-TCP extract group (>1.5 fold) (Fig. 8c–f).
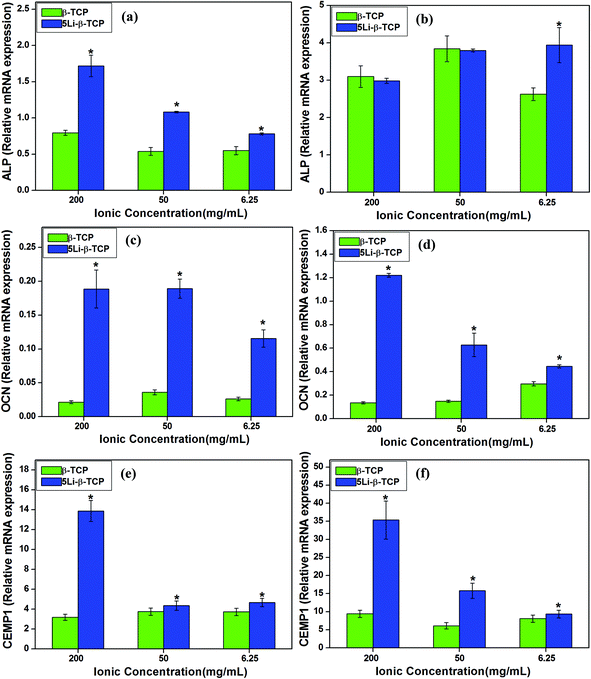 |
| Fig. 8 The relative bone-related gene expression of ALP, OCN and cementum-specific markers of CEMP1 for hPDLCs (a, c, e) and hBMSCs (b, d, f) after culturing with different concentrations of β-TCP and 5Li-β-TCP extracts for 7 days. *Significant difference (p < 0.05) for the 5Li-β-TCP group compared to the β-TCP group. | |
4. Discussion
Our present study conducted a systematic investigation of the cell attachment, proliferation, bone/cementum and Wnt canonical related gene expression for both hPDLCs and hBMSCs cultured on 5Li-β-TCP ceramic disks and with 5Li-β-TCP powder extracts. To the best of our knowledge, this is the first study on preparation of Li-containing β-TCP bioceramics. We have successfully incorporated Li into β-TCP by a conventional chemical precipitation method. The results showed that incorporating parts of Li into β-TCP bioceramics did not change the crystal phase structures. ICP analysis of both Li-β-TCP ceramic disks and powder extracts further confirmed that Li ions had been incorporated into β-TCP.
In this study, the cells cultured on β-TCP bioceramic disks were used as controls. Our SEM and CLSM analysis results showed that both hPDLCs and hBMSCs are well attached on the surface of 5Li-β-TCP and β-TCP bioceramics. ALP is known as an early marker for osteoblastic differentiation; OCN is another important gene related to the bone mineralization.44,45 CEMP1 and CAP are the cementum-specific markers which have been identified to have limited and specific expression in cementum or cementum-like tissues.46–48 Our results showed that 5Li-β-TCP had enhanced osteogenic and cementogenic differentiation of both cell lines over time. These data indicate that 5Li-β-TCP ceramic disks show excellent in vitro osteogenic and cementogenic stimulation for both hPDLCs and hBMSCs. Interestingly, in our study, hPDLCs showed a slightly enhanced proliferation rate than hBMSCs which may be due to the age difference between the donors who donated the periodontal ligament and bone marrow stromal cells (i.e. 18–25 vs. 65 years).
Previous reports have shown that many bioactive ions containing β-TCP ceramics could enhance the in vitro bioactivity. Among them, strontium (Sr) is an element proposed to promote bone growth. Sr might have a positive effect on bone formation by inhibiting bone resorption and enhancing endosteal and trabecular bone formation.49,50 There are increasing studies on the stimulatory effect of Sr2+ ions from Sr-TCP on the osteogenic differentiation of osteoblast-like cells and regeneration of lost bone.51 Silicon/strontium (Si or Sr)-containing β-TCP ceramics have been proven to stimulate bone cell proliferation and osteogenic differentiation by releasing Si/Sr ion products.18,52 Similar results were also obtained for Zn or Mg-TCP ceramics. Zn-doped TCP exhibited good bioactivity, which enhanced cell differentiation and ALP expression for the osteoblast MC3T3-E1 cell line.53,54 Further studies indicated that the presence of Mg-TCP ceramics stabilized the cell–material interface and thus improved cell attachment and growth of osteoblasts.54 It is obvious that most of these bioactive ions, such as Sr, Si, Zn and Mg, play an important role in inducing the osteogenic differentiation of bone cells. In this study, it was found that the released Li+ ions from β-TCP stimulate the osteogenic and cementogenic differentiation of both PDLCs and BMSCs. In addition, we found that Li+ ions released from mesoporous bioactive glass scaffolds could promote the regeneration of bone and cartilage.55 All these results suggest that Li+ ions have a multidirectional effect on the PDLCs, BMSCs and chondrocyte cells. Therefore, Li+ ions may have more universal advantages than other ions for inducing the differentiation of different kinds of stem cells.
Li is an element and it is reported that Li may enhance fracture healing in mice, while clinically it reduces the incidence of fractures with increasing accumulated dose of Li. In this study, Li+ ions released from 5Li-β-TCP bioceramics stimulated an increased osteogenic and cementogenic differentiation of both hPDLCs and hBMSCs in a general trend. Notably, Li+ ions could stimulate the Wnt canonical signalling pathway, suggesting that 5Li-β-TCP biomaterial could be potentially applied for periodontal regeneration due to its excellent osteogenic/cementogenic differentiation via activation of the Wnt canonical signalling pathway.
It is known that the chemical composition and surface properties of bioceramics are two important factors to influence the cell response.56,57 Our results showed that the incorporation of Li into β-TCP ceramic disks did not influence the surface morphology.58–62 Previous studies showed that the crystal size of bioceramics also significantly influenced the cell attachment and proliferation.63,64 Therefore, it is reasonable to speculate that the decreased crystal size of Li-containing β-TCP ceramics may be the other important factor to influence the cell differentiation. In this study, 5Li-β-TCP ceramics have smaller particle size and grains which may influence the cell differentiation, while the crystal size is still in the range for the cell growth and differentiation. To further investigate whether the composition of 5Li-β-TCP plays the key role in stimulating osteogenic/cementogenic differentiation of hPDLCs and hBMSCs, the ionic extracts of 5Li-β-TCP powders were prepared, which mainly contain Li+, Ca2+ and PO43− ionic products released from 5Li-β-TCP (Table 5). Similar results were obtained; ionic products from 5Li-β-TCP also significantly promoted bone/cementum-related gene expression of both hPDLCs and hBMSCs. Therefore, it is reasonable to speculate that the Li-containing ionic products play a key role in stimulating osteogenic/cementogenic differentiation of both hPDLCs and hBMSCs. It is known that LiCl has an effect in a dose-dependent manner on cell proliferation and differentiation. Recent findings showed that osteogenic differentiation of primary osteoblasts was enhanced by LiCl at the concentration of 1 mM;65 however, a higher concentration of 10 mM showed an inhibitory effect on osteoblastic differentiation.66 In this study, the Li+ ion concentration was only 0.2 mM released from 5Li-β-TCP bioceramic disks; however, it showed higher Li+ ion release at the concentration of 1.1 mM when 5Li-β-TCP extracts were of the concentration of 200 mg L−1. Therefore, the concentration range for Li ions is around 0.2–1.1 mM, which is comparable to that reported by Galli et al. We further analysed the ionic composition of cell culture medium for β-TCP and 5Li-β-TCP ceramic disks. Interestingly, it is also found that the concentrations of Li+ ions in the culture medium containing 5Li-β-TCP ceramic disks with hPDLCs are obviously lower than those with hBMSCs as shown in Tables 2 and 3. The possible reason is that different cell types may lead to different surface dissolution in the microenvironment of cell and material surfaces. Furthermore, two types of cells may also possess different uptaking abilities for Li+ ions. The pH value of cell culture medium with hBMSCs was slightly lower than that of hPDLCs. The lower pH value may be the main reason to improve the Li+ ion release from materials. However, further study is needed to confirm the difference between hPDLCs and hBMSCs on these material surfaces.
The effect of 5Li-β-TCP bioceramics on the Wnt signalling pathway for both hPDLCs and hBMSCs was further investigated to explore the possible underlying mechanisms for 5Li-β-TCP bioceramics to stimulate osteogenic/cementogenic differentiation of hPDLCs and hBMSCs. The Wnt signalling pathway plays a major role in various processes during development including cell proliferation and differentiation.67 WNT3a is a secreted glycoprotein that interacts with cell membrane-associated proteins and plays an important role in intracellular canonical Wnt signal transduction through the phosphorylation and accumulation of β-catenin which is another critical member of the Wnt/β-catenin signalling pathway.35,68 Axin2, also known as axin-like protein (Axil) or axis inhibition protein 2 (Axin2), is recognised as the most accurate reporter gene in the Wnt canonical pathway since it is a direct target gene for Wnt ligand binding and activation of the Wnt signalling pathway.69 To date, there have been few reports involving the interaction of the Wnt canonical signalling and hPDLC osteogenic differentiation. Our results showed that 5Li-β-TCP bioceramics significantly enhanced the Wnt canonical-related gene expression (WNT3A and AXIN2) in both hPDLCs and hBMSCs compared to β-TCP bioceramics, suggesting that Li+ ions from 5Li-β-TCP ceramics may be involved in the activation of the Wnt canonical signalling pathway for enhancing the osteogenic/cementogenic differentiation.
Therefore, it is speculated that 5Li-β-TCP bioceramics possess distinct osteogenic/cementogenic stimulation properties for promoting the bone/cementum-related gene expression. The schematic illustration of the possible mechanism is shown in Fig. 9, in which Wnt canonical signalling pathways in both hPDLCs and hBMSCs have been distinctively regulated by 5Li-β-TCP bioceramics. However, this concept needs to be further investigated for other Wnt pathway regulators and a future in vivo evaluation of the bone/cementum forming capacity is also needed to demonstrate that the 5Li-β-TCP bioceramic scaffolds can initiate bone/cementum regeneration.
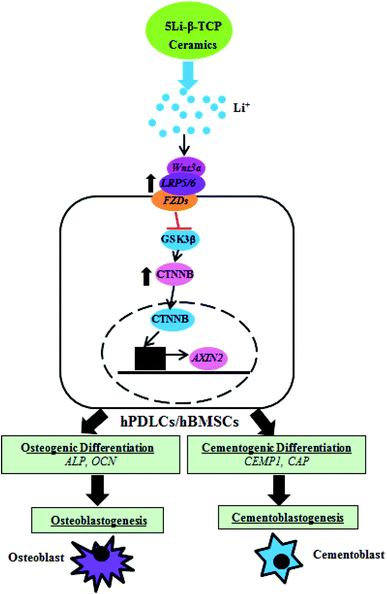 |
| Fig. 9 Schematic illustration for the effect of 5Li-β-TCP bioactive ceramics on osteogenic and cementogenic differentiation of both hPDLCs and hBMSCs. | |
5. Conclusion
In summary, Li-containing β-TCP bioceramics were successfully prepared. 5Li-β-TCP bioceramics supported cell attachment and proliferation. The release of Li from Li-β-TCP bioceramics significantly enhanced bone/cementum-related gene expression maybe via the regulation of Wnt canonical signalling pathways in both hPDLCs and hBMSCs. Li-β-TCP bioceramics show promising application in bone/cementum tissue regeneration due to their distinctively osteogenic and cementogenic stimulation properties.
Acknowledgements
The authors thank the Pujiang Talent Program of Shanghai (12PJ1409500), the Recruitment Program of Global Young Talent, China (Dr Wu), the Natural Science Foundation of China (Grant 81201202 and 81190132), ARC Discovery (DP120103697) and The State Key Lab of High Performance Ceramics and Superfine microstructure, SICCAS (SKL201203SIC) for the support of research.
References
- P. M. Bartold, Y. Xiao, S. P. Lyngstaadas, M. L. Paine and M. L. Snead, Periodontology 2000, 2006, 41, 123–135 CrossRef PubMed.
- J. E. Ellingsen, P. Thomsen and S. P. Lyngstadaas, Periodontology 2000, 2006, 41, 136–156 CrossRef PubMed.
- L. F. Wolff and B. Mullally, Int. Dent. J., 2000, 50, 235–244 CrossRef CAS PubMed.
- P. Koria, Biodrugs, 2012, 26, 163–175 CrossRef CAS PubMed.
- R. Vasita and D. S. Katti, Expert Rev. Med. Devices, 2006, 3, 29–47 CrossRef CAS PubMed.
- L. L. Hench and J. M. Polak, Science, 2002, 295, 1014–1017 CrossRef CAS PubMed.
- L. L. Hench and I. Thompson, J. R. Soc. Interface, 2010, 7(Suppl 4), S379–S391 CrossRef CAS PubMed.
- C. Wu, Y. Zhang, Y. Zhu, T. Friis and Y. Xiao, Biomaterials, 2010, 31, 3429–3438 CrossRef CAS PubMed.
- C. Wu, Y. Zhou, W. Fan, P. Han, J. Chang, J. Yuen, M. Zhang and Y. Xiao, Biomaterials, 2012, 33, 2076–2085 CrossRef CAS PubMed.
- C. Wu, Y. Zhou, M. Xu, P. Han, L. Chen, J. Chang and Y. Xiao, Biomaterials, 2013, 34, 422–433 CrossRef CAS PubMed.
- C. Wu, Y. Zhang, Y. Zhou, W. Fan and Y. Xiao, Acta Biomater., 2011, 7, 2229–2236 CrossRef CAS PubMed.
- P. Asvanund and P. Chunhabundit, Implant Dent., 2012, 21, 248–253 CrossRef PubMed.
- U. W. Jung, J. S. Lee, W. Y. Park, J. K. Cha, J. W. Hwang, J. C. Park, C. S. Kim, K. S. Cho, J. K. Chai and S. H. Choi, J. Periodontal Implant Sci., 2011, 41, 285–292 CrossRef CAS PubMed.
- L. Xia, Z. Zhang, L. Chen, W. Zhang, D. Zeng, X. Zhang, J. Chang and X. Jiang, Eur. Cell. Mater., 2011, 22, 68–82 CAS.
- S. Ni, K. Lin, J. Chang and L. Chou, J. Biomed. Mater. Res., Part A, 2008, 85, 72–82 CrossRef PubMed.
- C. Wang, Y. Xue, K. Lin, J. Lu, J. Chang and J. Sun, Acta Biomater., 2012, 8, 350–360 CrossRef CAS PubMed.
- C. T. Wu, W. Fan, Y. H. Zhou, Y. X. Luo, M. Gelinsky, J. Chang and Y. Xiao, J. Mater. Chem., 2012, 22, 12288–12295 RSC.
- M. Roy and S. Bose, J. Biomed. Mater. Res., Part A, 2012, 100, 2450–2461 Search PubMed.
- M. Sayer, A. D. Stratilatov, J. Reid, L. Calderin, M. J. Stott, X. Yin, M. MacKenzie, T. J. Smith, J. A. Hendry and S. D. Langstaff, Biomaterials, 2003, 24, 369–382 CrossRef CAS.
- H. W. Kim, Y. H. Koh, Y. M. Kong, J. G. Kang and H. E. Kim, J. Mater. Sci. Mater. Med., 2004, 15, 1129–1134 CrossRef CAS.
- A. J. Harwood, Mol. Psychiatry, 2005, 10, 117–126 CrossRef CAS PubMed.
- O. F. O'Leary, R. M. O'Connor and J. F. Cryan, Neuropharmacology, 2012, 62, 247–255 CrossRef CAS PubMed.
- A. J. Polotsky, L. Y. Zhu, N. Santoro and J. W. Pollard, Hum. Reprod., 2009, 24, 1960–1967 CrossRef CAS PubMed.
- A. K. Silva, H. Yi, S. H. Hayes, G. M. Seigel and A. S. Hackam, Mol. Vis, 2010, 16, 36–45 CAS.
- Y. Chen, H. C. Whetstone, A. C. Lin, P. Nadesan, Q. Wei, R. Poon and B. A. Alman, PLoS Med., 2007, 4, e249 Search PubMed.
- P. Vestergaard, L. Rejnmark and L. Mosekilde, Calcif. Tissue Int., 2005, 77, 1–8 CrossRef CAS PubMed.
- A. Zamani, G. R. Omrani and M. M. Nasab, Bone, 2009, 44, 331–334 CrossRef CAS PubMed.
- Y. Chen and B. A. Alman, J. Cell. Biochem., 2009, 106, 353–362 CrossRef CAS PubMed.
- I. Wilting, F. de Vries, B. M. Thio, C. Cooper, E. R. Heerdink, H. G. Leufkens, W. A. Nolen, A. C. Egberts and T. P. van Staa, Bone, 2007, 40, 1252–1258 CrossRef CAS PubMed.
- O. Cohen, T. Rais, E. Lepkifker and I. Vered, Horm. Metab. Res., 1998, 30, 594–597 CrossRef CAS PubMed.
- M. Khorami, S. Hesaraki, A. Behnamghader, H. Nazarian and S. Shahrabi, Mater. Sci. Eng., C, 2011, 31, 1584–1592 CrossRef CAS PubMed.
- M. Tylkowski and D. S. Brauer, J. Non-Cryst. Solids, 2013, 375, 175–181 CrossRef PubMed.
- A. P. M. Shainberg, P. Valerio, A. Zonari, F. N. Oktar, L. S. Ozyegin, M. P. F. Graca, M. F. Leite and A. M. Goes, Adv. Mater. Sci. Eng., 2012, 650574, 1–10 CrossRef PubMed.
- B. M. Seo, M. Miura, S. Gronthos, P. M. Bartold, S. Batouli, J. Brahim, M. Young, P. G. Robey, C. Y. Wang and S. Shi, Lancet, 2004, 364, 149–155 CrossRef CAS.
- A. Derfoul, A. L. Carlberg, R. S. Tuan and D. J. Hall, Differentiation, 2004, 72, 209–223 CrossRef CAS PubMed.
- R. O. Oreffo, C. Cooper, C. Mason and M. Clements, Stem Cell Rev., 2005, 1, 169–178 CrossRef CAS PubMed.
- T. Mygind, M. Stiehler and A. Baatrup,
et al.
, Biomaterials, 2007, 28, 1036–1047 CrossRef CAS PubMed.
- J. R. Mauneya, T. Nguyena, K. Gillena, C. Kirker-Headb, J. M. Gimblec and D. L. Kaplana, Biomaterials, 2007, 28, 5280–5290 CrossRef PubMed.
- X. Liu, R. K. Fu, R. W. Poon, P. Chen, P. K. Chu and C. Ding, Biomaterials, 2004, 25, 5575–5581 CrossRef CAS PubMed.
-
S. C. Kenneth and M. Hargreaves, Pathways of the pulp, 2011, p. 553 Search PubMed.
- P. Han, C. Wu, J. Chang and Y. Xiao, Biomaterials, 2012, 33, 6370–6379 CrossRef CAS PubMed.
- C. Wu, W. Fan, J. Chang and Y. Xiao, J. Mater. Chem., 2011, 21, 18300–18307 RSC.
-
ISO/EN 10993-5. Biological evaluation of medical devices-part 5 tests for cytotoxicity, in vitro methods 8.2 tests on extracts., ISO/EN 10993–10995. Biological evaluation of medical devices-part 10995 tests for cytotoxicity, in vitro methods 10998.10992 tests on extracts.
- A. I. Alford and K. D. Hankenson, Bone, 2006, 38, 749–757 CrossRef CAS PubMed.
- K. K. Ivaska, T. A. Hentunen, J. Vaaraniemi, H. Ylipahkala, K. Pettersson and H. K. Vaananen, J. Biol. Chem., 2004, 279, 18361–18369 CrossRef CAS PubMed.
- M. A. Alvarez-Perez, S. Narayanan, M. Zeichner-David, B. Rodriguez Carmona and H. Arzate, Bone, 2006, 38, 409–419 CrossRef CAS PubMed.
- H. Arzate, S. W. Olson, R. C. Page, A. M. Gown and A. S. Narayanan, FASEB J., 1992, 6, 2990–2995 CAS.
- D. Wu, K. Ikezawa, T. Parker, M. Saito and A. S. Narayanan, J. Bone Miner. Res., 1996, 11, 686–692 CrossRef CAS PubMed.
- S. G. Dahl, P. Allain, P. J. Marie, Y. Mauras, G. Boivin, P. Ammann, Y. Tsouderos, P. D. Delmas and C. Christiansen, Bone, 2001, 28, 446–453 CrossRef CAS.
- M. D. Grynpas, E. Hamilton, R. Cheung, Y. Tsouderos, P. Deloffre, M. Hott and P. J. Marie, Bone, 1996, 18, 253–259 CrossRef CAS.
- M. Tian, F. Chen, W. Song, Y. C. Song, Y. W. Chen, C. X. Wan, X. X. Yu and X. H. Zhang, J. Mater. Sci. Mater. Med., 2009, 20, 1505–1512 CrossRef CAS PubMed.
- G. Mestres, C. Le Van and M. P. Ginebra, Acta Biomater., 2012, 8, 1169–1179 CrossRef CAS PubMed.
- A. Ito, K. Ojima, H. Naito, N. Ichinose and T. Tateishi, J. Biomed. Mater. Res., 2000, 50, 178–183 CrossRef CAS.
- W. C. Xue, K. Dahlquist, A. Banerjee, A. Bandyopadhyay and S. Bose, J. Mater. Sci. Mater. Med., 2008, 19, 2669–2677 CrossRef CAS PubMed.
- Y. Wu, S. Zhu, C. Wu, P. Lu, C. Hu, S. Xiong, J. Chang, B. C. Heng, Y. Xiao and H. W. Ouyang, Adv. Funct. Mater., 2014 DOI:10.1002/adfm.201304304.
- P. Han, C. Wu and Y. Xiao, Biomater. Sci., 2013, 1, 379–392 RSC.
- M. Xu, Y. Zhang, D. Zhai, J. Chang and C. Wu, Biomater. Sci., 2013, 1, 933–941 RSC.
- P. Han, C. Wu, J. Chang and Y. Xiao, Biomaterials, 2012, 33, 6370–6379 CrossRef CAS PubMed.
- C. Wu, Y. Zhou, C. Lin, J. Chang and Y. Xiao, Acta Biomater., 2012, 8, 3805–3815 CrossRef CAS PubMed.
- E. S. Thian, Z. Ahmad, J. Huang, M. J. Edirisinghe, S. N. Jayasinghe, D. C. Ireland, R. A. Brooks, N. Rushton, W. Bonfield and S. M. Best, Acta Biomater., 2010, 6, 750–755 CrossRef CAS PubMed.
- C. Wu, Y. Ramaswamy, D. Gale, W. Yang, K. Xiao, L. Zhang, Y. Yin and H. Zreiqat, Acta Biomater., 2008, 4, 569–576 CrossRef CAS PubMed.
- A. Hoppe, N. S. Guldal and A. R. Boccaccini, Biomaterials, 2011, 32, 2757–2774 CrossRef CAS PubMed.
- A. Lopez-oriega, D. Arcos, I. Izquierdo-Barb, Y. Sakamoto, O. Terasaki and M. Vallet-Regi, Chem. Mater., 2006, 18, 3137–3144 CrossRef.
- A. J. Dulgar-Tulloch, R. Bizios and R. W. Siegel, J. Biomed. Mater. Res., Part A, 2009, 90, 586–594 CrossRef CAS PubMed.
- C. Galli, M. Piemontese, S. Lumetti, E. Manfredi, G. M. Macaluso and G. Passeri, Clin. Oral Implants Res., 2013, 24, 921–927 CrossRef CAS PubMed.
- J. Li, Z. Khavandgar, S. H. Lin and M. Murshed, Bone, 2011, 48, 321–331 CrossRef CAS PubMed.
- C. Y. Logan and R. Nusse, Annu. Rev. Cell Dev. Biol., 2004, 20, 781–810 CrossRef CAS PubMed.
- L. Schweizer and H. Varmus, BMC Cell Biol, 2003, 4, 4 CrossRef PubMed.
- H. M. Yu, B. Jerchow, T. J. Sheu, B. Liu, F. Costantini, J. E. Puzas, W. Birchmeier and W. Hsu, Development, 2005, 132, 1995–2005 CrossRef CAS PubMed.
|
This journal is © The Royal Society of Chemistry 2014 |
Click here to see how this site uses Cookies. View our privacy policy here.