DOI:
10.1039/C4BM00026A
(Minireview)
Biomater. Sci., 2014,
2, 951-955
Application of biomaterials for the detection of amyloid aggregates
Received
23rd January 2014
, Accepted 17th March 2014
First published on 15th April 2014
Abstract
Amyloid aggregates, which include oligomers and fibrils, are considered to cause various diseases, and their detection is important. In this minireview, recent developments on biomaterials, such as proteins, nanoparticles, and chemical reagents, for detecting amyloid aggregates are discussed. In particular, the molecular chaperone prefoldin (PFD) exhibits interesting properties for the interaction and detection of amyloid oligomers. In fact, any molecule that can bind amyloid aggregates could be useful for their detection.
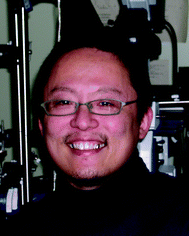 Tamotsu Zako | Tamotsu Zako is a senior research scientist at the Bioengineering laboratory, RIKEN Institute. He received his B.S., M.S. and Ph.D. degrees (Chemistry and Biotechnology) from the University of Tokyo, and studied at Waseda University and Tokyo University of Agriculture and Technology as a postdoctoral fellow. He was a recipient of a Research Fellowship for Young Scientist from the Japan Society for the Promotion of Science (JSPS). His research interests include the investigation of the mechanism of molecular chaperone proteins, and their relation to protein misfolding diseases such as Alzheimer's disease. He is also interested in the application of molecular chaperones as functional biomaterials. |
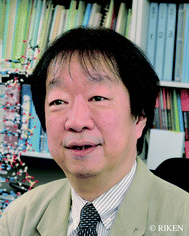 Mizuo Maeda | Mizuo Maeda is a chief scientist and the director of the Bioengineering laboratory at RIKEN Institute. He received his B.S., M.S. and Ph.D. degrees (Synthetic Chemistry) from the University of Tokyo. He is also a visiting professor at the University of Tokyo and Tokyo University of Science. His research expertise is focused on exploring a new frontier of research field which fuses biological science and engineering. On the basis of polymer chemistry, analytical chemistry and biochemistry, new materials comprising of biological components, and novel methodology for bioanalysis and medical diagnosis are investigated. His honor includes Fellow, Biomaterials Science and Engineering. |
Introduction
Protein aggregation, and amyloid formation in particular, has recently attracted considerable interest, because the associated processes are likely to be key issues in the pathology of more than 50 types of diseases, including Alzheimer's disease (AD) and Prion disease.1–3 Amyloids are typically rigid and exhibit unbranched structures with the diameter of 8–13 nm and length up to several micrometres. They also feature common properties, including a cross-β structure, in which the β-strands are aligned perpendicular to the long axis of the fibril. Interestingly, a number of non-disease-associated proteins, almost all proteins perhaps, can form similar amyloids under appropriate conditions.2 These amyloids are cytotoxic, and their physiological presence and tissue deposition are associated with neurodegenerative diseases, such as AD and other types of amyloidosis. The extracellular and intracellular aggregates of amyloid-β peptides (Aβ), including soluble oligomers, protofibrils, and mature amyloid fibrils, are considered to be the main cause of AD.1,2–6 Thus, the detection of these aggregates is of great importance for the early recognition of AD. In this minireview, recent developments on biomaterials, such as proteins, nanoparticles, and chemical reagents, for detecting amyloid aggregates are discussed (Fig. 1).
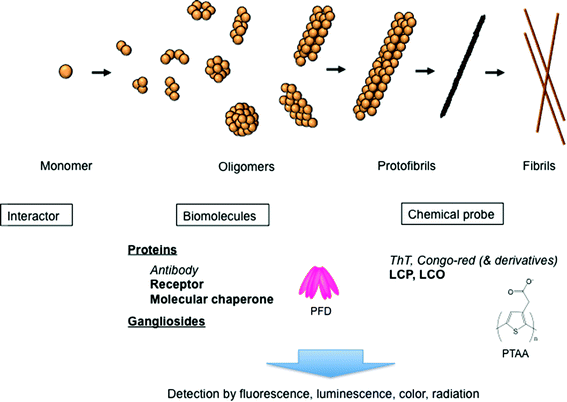 |
| Fig. 1 Various methods for the detection of amyloid aggregates, including oligomers, protofibrils and fibrils. Molecules that bind amyloid aggregates (interactors) are shown. Conventional interactors (antibody, Thioflavin T (ThT) and Congo red (and their derivatives such as PiB)) are shown in italic. The molecular chaperone prefoldin (PFD) and polythiophene acetic acid (PTAA) are shown as an example of molecular chaperone and luminescent conjugated polythiophene (LCP)/-oligothiophene (LCO), respectively. These interactors are detected by fluorescence, luminescence, color, etc. | |
Amyloid detection with various biomaterials
Rapid naked-eye detection of amyloids using gold nanoparticles
Aβ detection in vitro is mainly carried out by enzyme-linked immunosorbent assays (ELISA) or polyacrylamide gel electrophoresis (PAGE)/Western blotting using anti-Aβ antibody.7,8 However, these methods are unsuitable for a rapid analysis, as the signal detection usually takes up to a few hours. Thus, we developed a rapid and simple method for detecting Aβ aggregates using Aβ antibody-conjugated gold nanoparticles (AuNPs) (Fig. 2A).9 Recently, AuNPs have attracted great attention due to their advantageous properties, including stability, activity, and surface chemistry.10 For example, the aggregation of AuNPs accompanied by a surface plasmon shift can be recognized from a pronounced color change (from red to purple or to transparent), enabling their potential application as sensors for DNA, heavy metal ions and proteins.11 For the detection of Aβ, Aβ antibody was conjugated onto the surface of AuNPs. In the presence of Aβ aggregates such as oligomers and fibrils, AuNPs could produce precipitates via interactions between Aβ aggregates and Aβ antibody on the surface of AuNPs, while no change in the color of the solution was observed in the presence of Aβ monomers. The AuNP precipitates were observed within 1 h of reaction with the naked eye, suggesting that the AuNPs modified with the Aβ antibody were effective for the simple and rapid detection of Aβ aggregates. Further, this method could be applied for the detection of alternative amyloid aggregates. For example, it has been reported that prion protein can be detected by the aggregation of AuNPs modified with dihydrolipotic acid.12
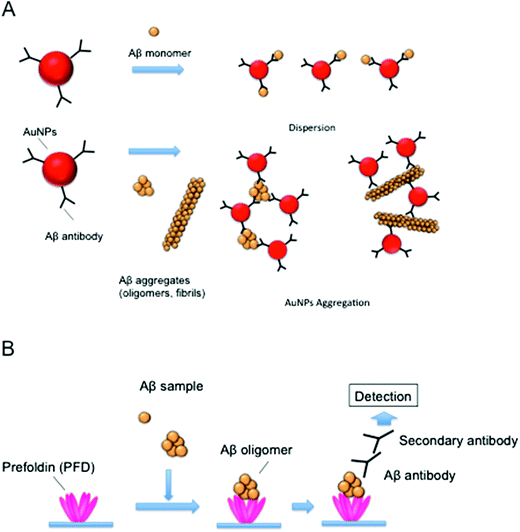 |
| Fig. 2 (A) Amyloid aggregate detection system using antibody-conjugated gold nanoparticles (AuNPs). In the presence of Aβ aggregates, such as oligomers and fibrils, AuNPs could produce precipitates or show color change via interactions between Aβ aggregates and Aβ antibody on the surface of AuNPs, while no change in the color of AuNPs solution was observed in the presence of Aβ monomers. (B) Amyloid oligomer detection system using the molecular chaperone prefoldin (PFD). Aβ oligomers captured by immobilized PFD can be detected with Aβ antibody and secondary antibody, similarly to the ELISA method. | |
Chemical amyloid probes
Conventional amyloid probes, such as thioflavin T (ThT) and Congo red, have been used for the detection of amyloids (Fig. 1).13 A variety of chemical amyloid probes have been developed utilizing these agents as scaffolds. Since positive charge of ThT is unfavorable for brain uptake, neutral ThT derivatives containing uncharged benzothiazole have been developed for in vivo amyloid imaging such as positron emission tomography (PET) and single photon emission computed tomography (SPECT).14 For example, one of the radioactive neutral ThT analogs, Pittsburgh compound B (PiB), has successfully been applied in human AD patients for PET imaging.15 Notably, these neutral ThT derivatives showed higher affinity to amyloids than the charged ThT.14 Methoxy-X04, a neutral Congo red derivative, was also applied in multiphoton in vivo imaging of amyloids in animal models.16 More recently, 18F-labeled Florbetapir, a stilbene derivative that has a longer lifetime than PiB, has been developed for PET imaging.17
Recently, amyloid probes consisting of repetitive thiophene moieties have also been developed. Nilsson and co-workers reported that luminescent conjugated polythiophene (LCP) and -oligothiophene (LCO) can be used as amyloid-specific probes (Fig. 1).18–20 In contrast to sterically rigid ThT and Congo red, LCPs exhibit a flexible thiophene backbone, providing a correlation between the conformation of the probe and the spectral characteristics of the particular conformation. Since recent studies showed that amyloids exhibit diverse structures some of which could not be stained with classical probes,21 LCPs/LCOs could be powerful tools to study amyloids. For example, insulin peptides form needle-like fibrils and noodle-like filamentous amyloids under different conditions.22 Although the binding of ThT or Congo red to the insulin filaments was weak, insulin filaments were stained with these LCPs and LCOs,23,24 implying the usefulness of LCPs/LCOs as a universal amyloid probe. Importantly, LCPs can serve as conformationally sensitive probes.18–20 For example, the polythiophene acetic acid (PTAA) spectra of insulin fibrils and those of filaments were different. Since the spectral red shift could be observed in planar and in closely stacked PTAA packing, this result suggests that the inner β-sheet packing of fibrils is relatively tighter than that of filaments.24 LCPs can also distinguish two different types of Aβ amyloids formed under quiescent and agitated conditions, while no difference in ThT binding can be observed.18 Importantly, LCPs/LCOs can also discriminate Aβ aggregates, including fibrils and oligomers, formed in the brain of transgenic mice.20 These results suggest that LCPs/LCOs can be used as a powerful probe for in vivo and in vitro amyloid detection.
Detection of amyloid oligomers with antibodies and chemical probes
Recent studies revealed that soluble oligomeric species are more toxic than amyloid fibrils and could cause various diseases.3,4,6,25,26 The term ‘soluble’ refers to any form of oligomeric species that remains in the soluble fractions after ultracentrifugation, indicating that it is the soluble form of the species. Various Aβ oligomers ranging from 2- to 24-mers, or those of higher molecular weight (MW), have been reported to be the cause of AD (Fig. 1). For example, small Aβ globular oligomers (5 nm in diameter), which were regarded as Aβ-derived diffusible ligands (ADDLs), were strongly bound with the dendritic arbours of cultured neurons, resulting in neuronal cell death and blockage of neuron functions.8,27 Further, large neurotoxic globular assemblies with diameters of 10–15 nm were also reported.28 It should be noted that structural variants could even exist among morphologically similar Aβ oligomers. Importantly, it has been reported that various proteins can form toxic oligomers, suggesting a common pathology of oligomer formation.29
To date, conformation-dependent antibodies have been used for specific detection of Aβ oligomers. ADDL was detected in brain fraction by using ADDL-specific antibody.30 It was also shown that prefibrillar oligomers, which were considered to be transient intermediates in the fibril formation, could be recognized by A11 anti-oligomer antibody.25 Interestingly, A11 antibody can recognize oligomers produced by different proteins, suggesting that prefibrillar oligomers that share a common structure may be formed by different proteins regardless of their amino acid sequences.29 In a recent study, A11-reactive oligomeric species were found in the brain of a patient suffering from sporadic Creutzfeldt–Jakob disease.31 However, because of the diverse structures and sizes of oligomers,6 the development of antibodies specific to each oligomer is still necessary.
Only a few chemical probes have been reported for amyloid oligomer detection. It was shown that p-FTAA (formyl thiophene acetic acid), one of the anionic LCOs, could detect various amyloid oligomers made from Aβ, insulin and prion.32 Recent studies also revealed that anionic LCOs consisting of five to seven thiophene units would be suitable for detection of amyloid oligomers.33
Detection of amyloid oligomers with molecular chaperone
The formation mechanism of soluble Aβ oligomers remains unclear. It was shown that various Aβ oligomer conformations were produced via different pathways, indicating the complexity of the mechanism.4,25 Aβ oligomers could be formed by the interactions of cellular components such as proteins and gangliosides.4 For example, toxic oligomers could be formed in the presence of αB-crystallin protein and GM1 ganglioside. In a recent report, Narayan et al. showed that small Aβ oligomers (dimers to 50-mers) can be recognized and sequestered by the molecular chaperone clusterin.34
Molecular chaperones are proteins that can recognize and bind exposed hydrophobic surfaces of non-native proteins. They can subsequently prevent protein aggregation and assist in their correct folding into a native conformation. We also found that soluble Aβ oligomers could be formed in the presence of the molecular chaperone prefoldin (PFD).35,36 The unique jellyfish-like structure of PFD consists of six long and protruding coiled-coil tentacles that interact with non-native proteins through hydrophobic interactions. It is reported that PFD can capture and deliver denatured proteins to another cytosolic chaperone, chaperonin.37
It was suggested that Aβ oligomers were produced by the interactions between PFD and Aβ oligomers, which could prevent their further aggregation and could stabilize oligomers.35 The study of these interactions revealed that PFD might recognize Aβ oligomers with various molecular sizes (Fig. 1). Thus, we expected that PFD could be used as a sensor for the development of the Aβ oligomer detection system.38 In this system, archaeal PFD, which was shown to strongly bind Aβ oligomers,35 was immobilized onto a microplate, and PFD-captured Aβ oligomers were detected with Aβ antibody (Fig. 2B). We demonstrated that the physiological concentrations of ADDL (10 nM) could be detected using this system. Our recent study also showed that small oligomers (2–4-mers) of polyglutamine protein, which causes Huntington's disease, were formed by human PFD.39 Thus, PFD-coated microplates following ELISA may be useful for the detection of various amyloid oligomers.
This finding leads to another idea that any molecule that can interact with amyloid oligomers might be useful as a detection probe. For example, several other cell surface receptors known to bind Aβ oligomers and to mediate toxicity, such as NGF, insulin and NMDA receptors,4,26 could be used for oligomer detection (Fig. 1).
Conclusions
We have discussed recent developments on biomaterials such as proteins, nanoparticles and chemical reagents for detecting amyloid aggregates. In particular, molecular chaperone proteins provide interesting properties for the interaction and detection of amyloid oligomers, and PFD is one such molecular chaperone for the detection of amyloid oligomers with various sizes. It should be emphasized that any molecule that can bind amyloid oligomers, including molecular chaperone proteins and receptors, could be useful as a detection probe.
Acknowledgements
The authors acknowledge financial support from JSPS KAKENHI no. 24570143 and RIKEN.
Notes and references
- F. Chiti and C. M. Dobson, Annu. Rev. Biochem., 2006, 75, 333 CrossRef CAS PubMed; M. Jucker and L. C. Walker, Nature, 2013, 501, 45 CrossRef PubMed.
- V. N. Uversky and A. L. Fink, Biochim. Biophys. Acta, 2004, 1698, 131 CrossRef CAS PubMed.
- M. Stefani, FEBS J., 2010, 277, 4602 CrossRef CAS PubMed.
- M. Sakono and T. Zako, FEBS J., 2010, 277, 1348 CrossRef CAS PubMed.
- F. M. Laferla, K. N. Green and S. Oddo, Nat. Rev. Neurosci., 2007, 8, 499 CrossRef CAS PubMed.
- V. N. Uversky, FEBS J., 2010, 277, 2940 CrossRef CAS PubMed.
- S. A. Gravina, L. Ho, C. B. Eckman, K. E. Long, L. Otvos Jr., L. H. Younkin, N. Suzuki and S. G. Younkin, J. Biol. Chem., 1995, 270, 7013 CrossRef CAS PubMed.
- M. P. Lambert, A. K. Barlow, B. A. Chromy, C. Edwards, R. Freed, M. Liosatos, T. E. Morgan, I. Rozovsky, B. Trommer, K. L. Viola, P. Wals, C. Zhang, C. E. Finch, G. A. Krafft and W. L. Klein, Proc. Natl. Acad. Sci. U. S. A., 1998, 95, 6448 CrossRef CAS.
- M. Sakono, T. Zako and M. Maeda, Anal. Sci., 2012, 28, 73 CrossRef CAS.
- M. C. Daniel and D. Astruc, Chem. Rev., 2004, 104, 293 CrossRef CAS PubMed.
- N. Kanayama, T. Takarada and M. Maeda, Chem. Commun., 2011, 47, 2077 RSC; R. de la Rica and M. M. Stevens, Nat. Nanotechnol., 2012, 7, 821 CrossRef CAS PubMed; T. Bu, T. Zako, M. Fujita and M. Maeda, Chem. Commun., 2013, 49, 7531 RSC.
- H. J. Zhang, H. Z. Zheng, Y. J. Long, G. F. Xiao, L. Y. Zhang, Q. L. Wang, M. Gao and W. J. Bai, Talanta, 2012, 89, 401 CrossRef CAS PubMed.
- R. Eisert, L. Felau and L. R. Brown, Anal. Biochem., 2006, 353, 144 CrossRef CAS PubMed.
- Y. Wang, W. E. Klunk, M. L. Debnath, G. F. Huang, D. P. Holt, L. Shao and C. A. Mathis, J. Mol. Neurosci., 2004, 24, 55 CrossRef CAS.
- W. E. Klunk, H. Engler, A. Nordberg, Y. Wang, G. Blomqvist, D. P. Holt, M. Bergstrom, I. Savitcheva, G. F. Huang, S. Estrada, B. Ausen, M. L. Debnath, J. Barletta, J. C. Price, J. Sandell, B. J. Lopresti, A. Wall, P. Koivisto, G. Antoni, C. A. Mathis and B. Langstrom, Ann. Neurol., 2004, 55, 306 CrossRef CAS PubMed.
- J. Dong, R. Revilla-Sanchez, S. Moss and P. G. Haydon, Neuropharmacology, 2010, 59, 268 CrossRef CAS PubMed; W. E. Klunk, B. J. Bacskai, C. A. Mathis, S. T. Kajdasz, M. E. McLellan, M. P. Frosch, M. L. Debnath, D. P. Holt, Y. Wang and B. T. Hyman, J. Neuropathol. Exp. Neurol., 2002, 61, 797 Search PubMed.
- D. F. Wong, P. B. Rosenberg, Y. Zhou, A. Kumar, V. Raymont, H. T. Ravert, R. F. Dannals, A. Nandi, J. R. Brasic, W. Ye, J. Hilton, C. Lyketsos, H. F. Kung, A. D. Joshi, D. M. Skovronsky and M. J. Pontecorvo, J. Nucl. Med., 2010, 51, 913 CrossRef CAS PubMed.
- K. P. Nilsson, A. Aslund, I. Berg, S. Nystrom, P. Konradsson, A. Herland, O. Inganas, F. Stabo-Eeg, M. Lindgren, G. T. Westermark, L. Lannfelt, L. N. Nilsson and P. Hammarstrom, ACS Chem. Biol., 2007, 2, 553 CrossRef CAS PubMed.
- M. Lindgren and P. Hammarstrom, FEBS J., 2010, 277, 1380 CrossRef CAS PubMed.
- T. Klingstedt and K. P. Nilsson, Biochem. Soc. Trans., 2012, 40, 704 CrossRef CAS PubMed.
- J. S. Pedersen, C. B. Andersen and D. E. Otzen, FEBS J., 2010, 277, 4591 CrossRef CAS PubMed.
- T. Zako, M. Sakono, N. Hashimoto, M. Hara and M. Maeda, Biophys. J., 2009, 96, 3331 CrossRef CAS PubMed.
- K. M. Psonka-Antonczyk, J. Duboisset, B. T. Stokke, T. Zako, T. Kobayashi, M. Maeda, S. Nystrom, J. Mason, P. Hammarstrom, K. P. Nilsson and M. Lindgren, Int. J. Mol. Sci., 2012, 13, 1461 CrossRef CAS PubMed.
- T. Zako, M. Sakono, T. Kobayashi, K. Sorgjerd, K. P. Nilsson, P. Hammarstrom, M. Lindgren and M. Maeda, ChemBioChem, 2012, 13, 358 CrossRef CAS PubMed.
- C. G. Glabe, J. Biol. Chem., 2008, 283, 29639 CrossRef CAS PubMed.
- S. T. Ferreira, M. N. Vieira and F. G. De Felice, IUBMB Life, 2007, 59, 332 CrossRef CAS PubMed.
- G. A. Krafft and W. L. Klein, Neuropharmacology, 2010, 59, 230 CrossRef CAS PubMed.
- S. Matsumura, K. Shinoda, M. Yamada, S. Yokojima, M. Inoue, T. Ohnishi, T. Shimada, K. Kikuchi, D. Masui, S. Hashimoto, M. Sato, A. Ito, M. Akioka, S. Takagi, Y. Nakamura, K. Nemoto, Y. Hasegawa, H. Takamoto, H. Inoue, S. Nakamura, Y. Nabeshima, D. B. Teplow, M. Kinjo and M. Hoshi, J. Biol. Chem., 2011, 286, 11555 CrossRef CAS PubMed.
- R. Kayed, E. Head, J. L. Thompson, T. M. McIntire, S. C. Milton, C. W. Cotman and C. G. Glabe, Science, 2003, 300, 486 CrossRef CAS PubMed.
- W. L. Klein, Alzheimers Dement., 2006, 2, 43 CrossRef PubMed.
- F. H. Aidt, L. F. Hasholt, M. Christiansen and H. Laursen, Histopathology, 2013, 62, 994 CrossRef PubMed.
- P. Hammarstrom, R. Simon, S. Nystrom, P. Konradsson, A. Aslund and K. P. Nilsson, Biochemistry, 2010, 49, 6838 CrossRef PubMed.
- T. Klingstedt, A. Aslund, R. A. Simon, L. B. Johansson, J. J. Mason, S. Nystrom, P. Hammarstrom and K. P. Nilsson, Org. Biomol. Chem., 2011, 9, 8356 CAS.
- P. Narayan, A. Orte, R. W. Clarke, B. Bolognesi, S. Hook, K. A. Ganzinger, S. Meehan, M. R. Wilson, C. M. Dobson and D. Klenerman, Nat. Struct. Mol. Biol., 2012, 19, 79 CAS.
- M. Sakono, T. Zako, H. Ueda, M. Yohda and M. Maeda, FEBS J., 2008, 275, 5982 CrossRef CAS PubMed.
- K. M. Sorgjerd, T. Zako, M. Sakono, P. C. Stirling, M. R. Leroux, T. Saito, P. Nilsson, M. Sekimoto, T. C. Saido and M. Maeda, Biochemistry, 2013, 52, 3532 CrossRef CAS PubMed.
- A. Ohtaki, K. Noguchi and M. Yohda, Front. Biosci., 2010, 15, 708 CrossRef CAS PubMed; P. C. Stirling, S. F. Bakhoum, A. B. Feigl and M. R. Leroux, Nat. Struct. Mol. Biol., 2006, 13, 865 Search PubMed.
- M. Sakono, T. Zako, M. Yohda and M. Maeda, Biochem. Eng. J., 2012, 61, 28 CrossRef CAS PubMed.
- E. Tashiro, T. Zako, H. Muto, Y. Itoo, K. Sorgjerd, N. Terada, A. Abe, M. Miyazawa, A. Kitamura, H. Kitaura, H. Kubota, M. Maeda, T. Momoi, S. M. Iguchi-Ariga, M. Kinjo and H. Ariga, J. Biol. Chem., 2013, 288, 19958 CrossRef CAS PubMed.
|
This journal is © The Royal Society of Chemistry 2014 |
Click here to see how this site uses Cookies. View our privacy policy here.