DOI:
10.1039/C3AY41630E
(Paper)
Anal. Methods, 2014,
6, 147-155
Unraveling the modified surface of the photographic paper “Japine”
Received
19th September 2013
, Accepted 28th October 2013
First published on 29th October 2013
Abstract
Modification of the surface of a paper intended for coating with a photographic sensitizer will influence both its chemical and physical characteristics. A scientific examination was performed on the photographic paper, Japine, produced by the Platinotype Company from 1906 to 1937 for their platinum, silver, and palladium printing processes. Scanning electron microscopy (SEM) and non-invasive imaging by optical coherence tomography (OCT) reveal the presence of a very thin layer at the paper surface. Detailed investigation by attenuated total internal reflectance-Fourier transform infrared spectroscopy (ATR-FTIR) and gas chromatography-mass spectrometry (GCMS) analyses determined that this surface consists of a parchmentized layer created by chemical modification of the paper support rather than the presence of an applied coating. Reverse engineering studies suggest that the paper was prepared by treatment with sulfuric acid under select conditions to modify the structure of the cellulose only at the paper surface. SEM imaging demonstrates that the distribution of the platinum particles that make up the image is significantly altered on surface parchmentized papers compared to unmodified papers.
Introduction
The appearance of a photograph is strongly influenced by its surface character. The many photographic processes created on paper supports exhibit a large range of surface properties with the metal particles that form the image either nestled within the paper fibers (e.g., salted paper prints and platinum prints) or suspended above the paper surface in a matrix (e.g., albumen and gelatin prints). Modifying the paper support not only will influence the aesthetic appearance of plain paper prints, but will impact their manufacture (e.g., the application of sensitizing salts), storage, durability while handling, and longevity.
The platinum printing process was invented by William Willis, Jr,1 whose Platinotype Company (1878–1937) manufactured sensitized paper and chemicals for the production of platinum, platinum–silver (Satista), palladium, and silver prints. Willis refined these processes over the decades, and competitors offered their own platinum papers. Platinum prints were offered on papers that varied in tone, weight, roughness, and sheen. In 1906, the Platinotype Co. introduced a new platinum paper under the trade name, “Japine,” which was touted as possessing an eggshell finish, resistance to dampness, and increased printing latitude.2 This and subsequent Japine papers, including Japine Silver (introduced in 1916)3 and Japine Palladiotype (introduced in 1917),4 became highly regarded and were used by noteworthy photographers, such as Alfred Stieglitz and Paul Strand. However, no specific information was given on the preparation of this unique paper support. Unraveling the chemistry of this important photographic paper would broaden our knowledge about the manufacture and identification of such papers, their use by photographers, and aid long-term preservation efforts.
Sensitized photographic paper products and prints explicitly labeled as made on Japine paper are extremely rare. Few prints labeled “Japine” have been located, and only one unopened container of Japine Platinotype paper, collected by The Metropolitan Museum of Art (MMA), has been found. Collection prints are typically inappropriate for analyses requiring removal of samples, which may be necessary for a full characterization of the paper surface chemistry. Additionally, unrecorded information regarding photographers' post-processing, such as coating with wax or oil, can complicate the interpretation of these analyses. MMA's recent acquisition of the unopened container of Japine paper provided the unique opportunity to investigate an identified example of this photographic paper.5 During the initial examination of the paper, as detailed below, a superficial surface layer, or “crust,” was observed. Speculation exists as to the nature of the Japine surface, yet remarks from staff of the Platinotype Co. indicate that it was a property of the paper rather than an applied coating.6–8 While Willis never patented his Japine paper, his patent for the platinum-silver paper refers to parchmentization by sulfuric acid,9 potentially alluding to the manufacture of Japine.
Vegetable parchment, which mimics animal parchment, is prepared by immersion of cellulose-based paper in sulfuric acid. It was noted to possess the desired properties of high mechanical strength and resistance to swelling in water.10 The manufacture of these papers could be tailored to obtain full-sheet parchmentization or only surface parchmentization.11 Parchmentization occurs by disruption of the cellulose intracrystalline hydrogen-bonding by sulfuric acid within a window of acid concentration.12 Early literature on parchmentization by sulfuric acid differs in the specifics of the acid concentrations employed. Most commonly the concentrations are in the range of 65% to 75% (w/w),10,13 though higher values up to 84% (ref. 14) or even ca. 98% are reported.15 Bücher examined the impact of sulfuric acid concentration, time, and temperature on the resulting paper.16 Each of these factors impacted the appearance of the paper fibers or the formation of a surface film. The optimal acid concentration for parchmentization was found to lie between 62.5% and 70%. At 62.5% acid, the swelling of cellulose fibers induced by sulfur acid was determined to be solely interfibrillar,17 with higher concentrations leading to inter- and intrafibrillar swelling.18 At concentrations between 63 and 74% acid the crystallinity of the cellulose chains can be altered, disrupting the naturally found cellulose I structure and allowing reprecipitation as the more stable cellulose II after immersion into water and neutralization.19,20 These precipitated macromolecular chains form a film at the paper surface rather than discrete fibers.
The scientific examination of the Japine paper surface detailed herein supports the hypothesis that it was manufactured by treatment of the paper surface with sulfuric acid achieving partial parchmentization. Surface parchmentization would provide the desired sheen while retaining an opaque and reflective support for the photographic image. Reverse-engineering experiments shed light on the challenges in preparing such surfaces, the final physical and chemical properties of these unique photographic papers, and serve as an aid for their identification and long-term preservation.
Experimental
Paper analysis
Two sensitized unprocessed platinum paper samples from the Platinotype Co. were analyzed: Japine and “KK,” an alpha designation that indicated a heavy smooth paper with an unmodified surface.21 The Japine paper tested positive for rosin by the Raspail microchemical test.22 Scrapings from a 2 mm2 area of the Japine “crust” and paper base were taken for resin, carbohydrate, and amino acid analyses via gas chromatography-mass spectrometry (GCMS) methods as described below.
Resin analysis
Resin analysis was conducted by in situ methylation using tetramethylammonium hydroxide. Two microliters of tetramethylammonium hydroxide (TMAH) were placed onto the sample in a 50 μL deactivated stainless steel cup. The sample cup was then dropped into the helium-purged furnace of a Frontier Labs PY-2020iD Double-Shot Pyrolyzer (furnace: 600 °C, interface: 320 °C) and the pyrolysates were sent directly to a Varian 3800 gas chromatograph (GC) for 6 s. The Varian 3800 GC was equipped with an 1177 injector (held at 320 °C; split 50
:
1) with electronic flow control. The capillary column was a VF-5 ms; 30 m × 0.25 mm i.d.; 0.25 micron film thickness; He flow of 1.2 mL min−1. The GC oven was programmed with an initial temperature of 40 °C, which is held for 5 min. The temperature was increased at a rate of 20 °C per min to 300 °C and held for 5 min. The Varian 3800 GC was interfaced to a Varian Saturn 2000 ion trap, the transfer line being held at 300 °C. Operating conditions for the trap were: trap 200 °C, manifold 50 °C; electron multiplier 1550 V; scan range 45–650 amu; scan time 1 s; data analysis Saturn GC/MS Workstation 6.9.2 software and the NIST 2008 spectral libraries.
Carbohydrate and amino acid analysis
The sample was added to a 0.1 mL conical glass vial insert, which in turn was inserted into a 1 mL Pierce Reacti-vial (Vial #1). 20 μL of water was added to the sample, which was then homogenized with a stainless steel syringe plunger. The Reacti-vial was tightly sealed with a Teflon-lined cap and heated to 50 °C for 48 hours. After cooling and centrifugation, the supernatant was divided into two conical glass inserts, which were placed into Vials #2 and #3 and evaporated to dryness at 50 °C in a stream of nitrogen. The residues from Vials #1 and #3 were then analyzed for amino acids as described below.
Carbohydrate analysis was conducted by first hydrolyzing the carbohydrate in methanolic HCl and subsequent silylation of the resulting monosaccharides using Sylon HTP (Supelco, hexamethyldisilazane + trimethylchlorosilane + pyridine, 3
:
1
:
9).23,24 20 μL of 0.4 N methanolic HCl was added to the supernatant residue in Vial #2 and heated at 80 °C for 24 hours. The solution was then evaporated to dryness at 50 °C under a stream of nitrogen. 20 μL of Sylon HTP was added to the residue and heated at 80 °C for 2 hours. The solution was then evaporated to dryness at 50 °C under a stream of nitrogen and dissolved in iso-octane for injection in the Varian Saturn 2100T GCMS.
Amino acid analysis was performed by adding 50 μL of 6 N HCl to the residues in Vials #1 and #3. Vial #1 would contain any insoluble proteins present (casein, egg, and/or glues) while Vial #2 would contain any soluble proteins present (glues). The vials were capped with Teflon-lined septa, and the air in the vials was evacuated using a vacuum pump. The samples were heated at 105 °C for 24 hours, cooled to room temperature, centrifuged, and evaporated to dryness at 50 °C in a stream of nitrogen. 20 μL of the silylating reagent N-methyl-N-tert-butyldimethylsilyltrifluoroacetamide/tert-butyl dimethylchlorosilane (MTBSTFA/TBDMCS) was added to the vials, which were heated at 50 °C for 30 min and then at 105 °C for 5 hours. The conical glass inserts containing the derivatized samples were transferred to autosampler vials for injection in the Varian Saturn 2100T GCMS.
The Varian Saturn 2100T GCMS was equipped with a Varian CP-8400 autosampler. One microliter of the solution was injected by the autosampler onto a capillary column (VF-5ms; 30 m × 0.25 mm i.d.; 0.25 micron film thickness; He flow of 1.2 mL min−1; splitless) in a Varian 3900 gas chromatograph equipped with electronic flow control. The injector was held at 300 °C and the GC oven was programmed with an initial temperature of 55 °C, which was held for 2 min. The column temperature was increased at a rate of 14 °C per min to 300 °C and then at a rate of 8 °C per min to 320 °C and held for 5 min for a total runtime of 27 min. The Varian 3900 GC was interfaced to a Varian Saturn 2000 ion trap, the transfer line being held at 250 °C. Operating conditions for the trap were: trap 150 °C, manifold 50 °C; electron multiplier 1550 V; scan range 42–650 amu; scan time 1 s; trap off from 0 to 3.5 min and 6 to 7 min for solvents; data analysis Saturn GC/MS Workstation 6.9.2 software and the NIST 2008 spectral libraries.
X-Ray fluorescence
X-ray fluorescence (XRF) analyses were performed on an ArtTax Pro (Bruker) using a Rh tube (50 KV, 200 μA) equipped with capillary optics. Spectra were collected for 200 s with a He flush to aid detection of lighter elements.
Scanning electron microscopy
Cross-sections of photographic paper samples were prepared on a modified microtome (Jung Multicut) equipped with diamond-coated stainless steel blades (Sturkey). The prepared sections were typically 40 μm to 60 μm thick. Samples were directly mounted on carbon tape on carbon stubs for examination on a variable pressure scanning electron microscope (SEM, Hitachi S 3400N) equipped with an energy dispersive spectroscopy (EDS) detector (Oxford X-Max). Samples were not embedded in a polymer matrix, nor was sample coating performed. Samples were imaged at 20 kV at 40 Pa at a 10 mm working distance detecting back-scattered electrons (BSE).
Attenuated total internal reflectance-Fourier transform infrared spectroscopy
Attenuated total internal reflectance-Fourier transform infrared spectroscopy (ATR-FTIR) was performed with a Nicolet Nexus 670 coupled to a Continuum microscope with a single-pass Ge ATR crystal. Spectra were collected at 4 cm−1 resolution from 4000 cm−1 to 650 cm−1 averaging 512 scans.
Parchmentization
A ca. 1980 Crane & Co. alum-rosin sized 100% cotton paper, without alkaline buffers or optical brightening agents, was selected as the most appropriate analog for the parchmentization tests. 65% (w/w) sulfuric acid was prepared and allowed to cool to room temperature (22 °C). Three initial moisture conditions were selected for the paper: dry (ambient conditions – no initial treatment), humidified (placed in a chamber over water for two hours, ca. 90% RH), and pre-wet (placed in water for 60 s). The papers were placed in the acid solution for 10 s and then hung in the air for 30 s (10 s in the case of the initially dry paper). Samples then were washed in distilled water for 60 s, neutralized in a 10% (v/v) NH4OH solution for 60 s, and further washed in two distilled water baths for 30 s and 60 s, respectively. The papers were hung to dry. Once dry, the curled papers were humidified and flattened between spun polyester and smooth paper board. The pH of the papers before and after treatment were comparable (pH ca. 5.5).
Printing
Details of the historic platinum printing methodology may be found elsewhere.25 Briefly, samples of both the untreated and parchmentized Crane & Co. cotton papers were coated with a solution of potassium tetrachloroplatinate and iron(III) oxalate (acidified with oxalic acid). Once dry, the sheets were printed in contact with a step wedge negative under UV light and developed with a potassium oxalate solution. The samples were cleared by dilute HCl baths, washed in distilled water, and hung to dry. Both the untreated and parchmentized papers yielded prints of good quality, demonstrating that both the unmodified paper and the parchmentized papers were suitable for use as a platinum paper.
Optical coherence tomography
Spectral domain optical coherence tomography (OCT) was performed using a custom system from Bioptigen with a center wavelength of 840 nm with a full-width at half the maximum bandwidth of 93 nm. Details and characterization of this system have been published previously.26 A 0.5 mm by 0.5 mm region of the paper was analyzed collecting 200 B-scans.
Results and discussion
Characterization of Japine
Visual and microscopic characterization of the surfaces indicated that the KK paper exhibited a plain, unmodified surface matte finish while the Japine paper had a semi-gloss finish. It should be noted that when Japine Platinotype was first marketed it was initially offered in two finishes, semi-matte and glossy,2 with a range of other surfaces offered later. The semi-gloss Japine paper examined here appeared to have a superficial surface layer when viewed under an optical microscope as evidenced by a “crust” with cracks that were particularly noticeable at the edges of the paper. The metal content of the papers was examined by XRF, with both sensitized papers containing primarily platinum and iron (Fig. 1). This corresponds with the patents from the Platinotype Co. that indicate the sensitizer contains potassium tetrachloroplatinate and ferric oxalate.1 Salts of metals such as mercury and lead were often added to change the tone and sensitivity of these papers and were mentioned in Willis's patents.27,28 The Japine and KK papers examined vary slightly in metal content, particularly the presence of mercury or lead; however, as the Platinotype papers were sold in a variety of tones (e.g., sepia, black, and warm black) it is expected that variation occurred across the product line. More identified samples are required for detailed comparisons.
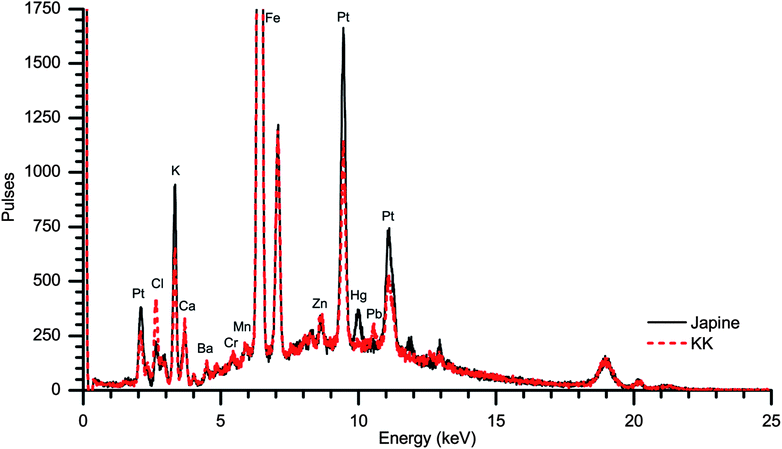 |
| Fig. 1 XRF spectra of the Platinotype Co. papers Japine (solid black) and KK (dashed red). The elemental profile of these papers is exceptionally similar. The presence of mercury was detected in the Japine sample as opposed to lead in the KK paper. | |
Structural information of the photographic papers was provided by SEM. In particular, cross-sectional imaging was particularly enlightening (Fig. 2). In these images the sensitized portion of the paper appears near the top and is brighter due to the presence of electron-rich metals. Platinum and iron salts were confirmed at these surfaces by EDS. The sensitized sides of the two papers are visibly quite different: the KK surface consists of paper fibers while the Japine surface appears smooth suggesting the presence of a coating. The thin layer on the Japine surface is 5 μm to 8 μm thick, and the sensitizer is primarily contained within the modified surface, although the sensitizing salts are detectable beneath this layer. It is not known whether the sensitizing salts always had this distribution or if they migrated out of the surface layer during the past century. The sensitized surfaces of both naturally aged papers have become notably brittle, as observed by the cracking of the Japine surface and the easy detachment of the top surface of the KK paper during preparation of the cross-sections.
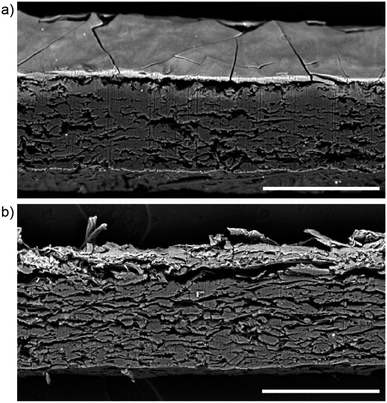 |
| Fig. 2 BSE SEM images of the Platinotype Co. platinum papers (a) Japine and (b) KK. The Japine paper was tilted to allow visualization of the very thin surface layer (ca. 5–8 μm). Both papers are sensitized and unprocessed. The metals (Pt, Fe, etc.) of the sensitizer are visible as a lighter band near the surface of the paper. Scale bar is 200 μm. | |
Pyrolysis-GCMS analysis indicated the presence of diterpenoid resins, consistent with rosin sizing of the paper. No amino acids were detected, removing the possibility of a protein emulsion such as albumen or gelatin being responsible for the unique surface of Japine. Carbohydrate analysis only detected the presence of the glucose silyl ester in both the paper base and the surface crust. This indicates that the surface layer was still based on glucose monomers, as would be expected for cellulose fibers. No other carbohydrates were detected, ruling out the application of starch or gum to the paper surface.
To better understand the chemical composition of the paper surfaces, ATR-FTIR analyses were performed. ATR-FTIR provides an ideal minimally invasive method to analyze the chemical features of cellulosic fibers.29 The infrared transitions of newly prepared and aged cellulose from different plant sources have been described.30,31 The paper base of the Platinotype Co. papers is best examined by ATR-FTIR measurements of the non-sensitized side (verso), thereby avoiding contributions from the sensitizing agents (Fig. 3). The IR spectra of cellulosic materials is complex and detailed assignments from different cellulose sources can be found in the literature.32–36 The Platinotype Co. base papers conform to typical cellulose ATR-FTIR spectra. Broadly the signals observed in these samples are 3350 cm−1: O–H stretching; 2900 cm−1: C–H stretching; 1500–1200 cm−1: O–H and C–H bending modes; 1200–1000 cm−1: C–O stretching and ring C–O–C modes; and 900 cm−1: ring C–O–C stretching associated with amorphous cellulose.
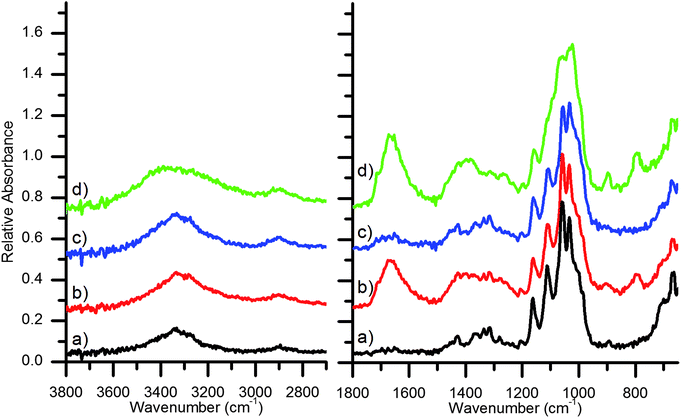 |
| Fig. 3 ATR-FTIR spectra from two sensitized papers from the Platinotype Company. Measurements were performed on the sensitized (recto) and unsensitized (verso) sides of the papers. The spectra are (a) KK verso, (b) KK recto, (c) Japine verso, (d) Japine recto. Spectra are separated into high and low wavenumber ranges and are offset for clarity. | |
Examination of the spectra of the sensitized side of the paper (recto) is complicated by a lack of complete knowledge of the different sensitizing agents and other additives to the photographic paper. However, the presence of potassium tetrachloroplatinate, iron(III) oxalate and oxalic acid are expected on the sensitized side of the paper. Vibrational modes of the chloroplatinate are outside the range of these ATR-FTIR measurements. The iron(III) oxalate gives rise to the strong peaks at 1715 cm−1 and 1670 cm−1 (C–O stretching modes) and 1380 cm−1 (C–O and C–C stretching modes)37 with overlapping contributions C–O stretching contributions from the small quantity of oxalic acid.38,39 Accounting for these new sensitizer peaks it is observed that the KK paper has similar cellulosic spectral peaks on both sides. Contrarily, there are differences between the Japine paper recto and verso. Specifically, for the Japine recto there is a broadening and shifting of the O–H stretching modes to higher energy, a broadening of the 1200–1000 cm−1 region, and an increase in the intensity of the 900 cm−1 peak, indicating a change in the crystallinity of the surface cellulose.40 Further discussion of the spectral changes is presented in the next section. No vibrational modes indicative of proteins were detected.
Surface parchmentization
To better understand the proposed surface modification of the Japine, model parchmentization experiments were carried out that produce a similar surface. An alum-rosin sized 100% cotton paper was chosen as the most appropriate test material. Many conditions will affect the rate of parchmentization. Selecting the appropriate acid concentration is crucial to maximize the swelling of the cellulose fibers while minimizing degradation through hydrolysis or oxidation of the cellulose. The use of 65% (w/w) sulfuric acid proved optimal for the paper surface parchmentization. A lower concentration (53%) test exhibited no parchmentization. At 75% acid parchmentization was observed; however a significant quantity of residual sulfur remained in the paper, as measured by an increased sulfur signal in the SEM-EDS spectrum and ATR-FTIR peaks at 1250 cm−1 and 810 cm−1, indicating the presence of sulfate esters.41,42 It has been noted that increasing the sulfuric acid concentration from 70% to 75% resulted in an increase in the mineral content of the paper.43
The moisture content of the starting paper is rarely stated in the discussion of parchmentizing methods for early papers. It was observed that for lower acid concentrations (55% to 62%), a moisture content of up to 16% increased the hydrolyzability of the cellulose.44 The addition of moisture to the paper will speed the parchmentization reaction by pre-swelling the cotton fibers, allowing penetration of the acid deeper into the paper and increase the temperature of the reaction due to the exothermic solvation of the water in the acid. To determine the importance of moisture in the base paper, three conditions with increasing initial moisture content were selected: dry (equilibrated to the room RH: c.a. 55% RH), humidified (ca. 90% RH), and pre-wet. SEM images of the unmodified paper and parchmentized papers after sensitizing, exposure, and processing are shown in Fig. 4. In the unmodified paper the cotton fibers are readily visible at the paper surface with the printed platinum nestled on, in and under the top fiber layer. A smooth surface layer is visible on each of the parchmentized samples, with the layer thickness increasing for the samples with greater initial moisture content. Note that the platinum particles that form the image do not penetrate as deeply into parchmentized paper as into unmodified paper.
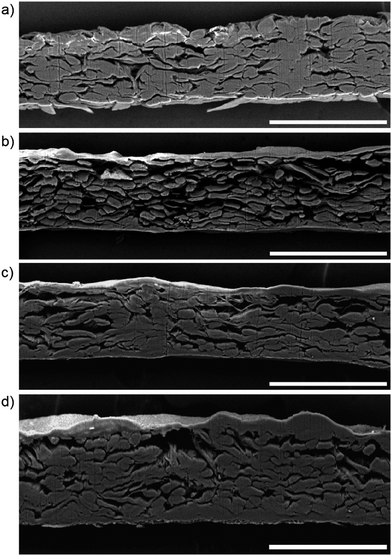 |
| Fig. 4 BSE SEM images of the prints made on 100% cotton paper samples after sensitizing, exposure and processing. (a) The print was prepared on unmodified paper. Prints were also made on parchmentized papers prepared with initial moisture conditions of (b) dry, (c) humidified, and (d) pre-wet. Platinum metal forming the image is indicated by the bright signal at or near the surface of the papers. Scale bar is 200 μm. | |
The chemical changes to the surface of the parchmentized Crane & Co. paper were detected by ATR-FTIR (Fig. 5). Similar to the Platinotype Co. samples, the signals from the cellulose structure dominate the spectra. Significant differences are noted between the unmodified and parchmentized paper samples. Increased signals at 1020 cm−1 are observed for the three parchmentized samples, indicating a change in the C–O stretching modes. For the parchmentized papers a very small peak appears at 1260 cm−1 in the ATR-FTIR spectra and a minimal sulfur signal was observed by SEM-EDS, indicating the formation of a small amount of cellulose sulfate esters. Correspondingly, small peaks near 1650 cm−1 indicate the presence of cellulose esters in the parchmentized samples. Such peaks may be present in the Platinotype Japine sample, albeit very weak. Similar to the Japine sample, there is a shift of the O–H stretching modes to higher energies, indicating a change in the crystal structure of the surface cellulose. Likewise, there is an increase in the peak at 900 cm−1, which is associated with an increase in amorphous cellulose.40 A small sharp peak at 1110 cm−1 (in plane ring stretching)35,36,45 is observed in the untreated papers and significantly broadens in the parchmentized papers (Japine and test papers), indicating a loss of cellulose crystallinity. It is observed that the features and intensities of the dry, humidified, and pre-wet samples appear nearly identical. The penetration depth of the ATR-FTIR is shallow enough not to be sensitive to the increased thickness of the parchmentized layer. This suggests that there was only a change in the thickness of the layer as a function of moisture content, but the chemical composition remains the same (e.g., there is no discernible change in sulfonation).
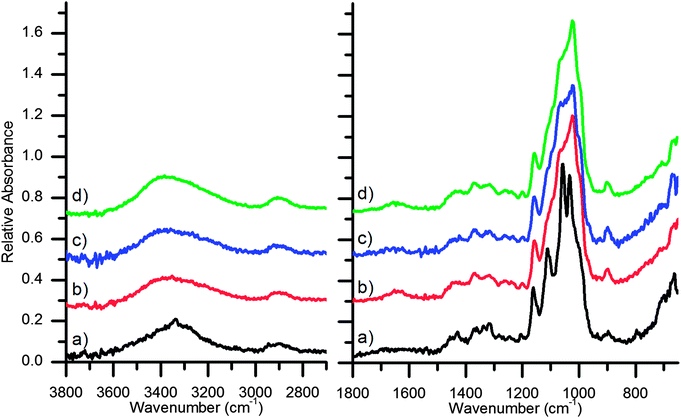 |
| Fig. 5 ATR-FTIR spectra from a sample of 100% cotton paper (a) prior to and (b–d) after sulfuric acid parchmentization with different paper moisture conditions of (b) dry, (c) humid and (d) pre-wet. Spectra are separated into high and low wavenumber ranges and are offset for clarity. | |
Optical coherence tomography
Identifying the presence of a chemically modified surface of an unknown print remains challenging. Visual examination after comparison between many samples informs the initial classification; however analytical testing would be preferred for confirmation. Identification of surface chemical treatments by SEM is severally limited by its sampling requirement. ATR-FTIR is hampered by the addition of coating layers on the paper surface. For instance, it was common practice to apply thin layers of beeswax or linseed oil to improve the final appearance of a photograph.46,47 Depending on the coating thickness, ATR-FTIR measurements will be complicated if not fully obscured. For this reason, OCT was investigated as a noninvasive method to examine the surface of the Platinotype Co. papers and the unmodified and parchmentized Crane & Co. papers. OCT has been demonstrated as a non-invasive tool that allows visualization and characterization of works of art,48,49 including paper and animal parchment.50-52 While the thickness of the Japine layer is on the order of the resolution of the OCT system (ca. 4 μm) the significant change in the reflectivity and effective refractive index across this interface can be observed and is readily distinguishable from the KK paper (Fig. 6). Similarly, the parchmentized cotton papers all exhibit an identifiable top surface layer compared to the unmodified cotton paper. Note that the increased thickness of the parchmentized layer for the humidified and pre-wet samples are not readily discernible. Thin coatings of linseed oil (applied by swab) or beeswax (applied by a petroleum ether-moistened swab) were applied to sections of the surface of the humidified parchmentized paper and examined by OCT. While there were subtle changes to the images, particularly in the relative intensities, the parchmentized surface layer remained identifiable. Combining non-invasive OCT with ATR-FTIR analysis is a promising method to aid the identification of Japine and related papers.
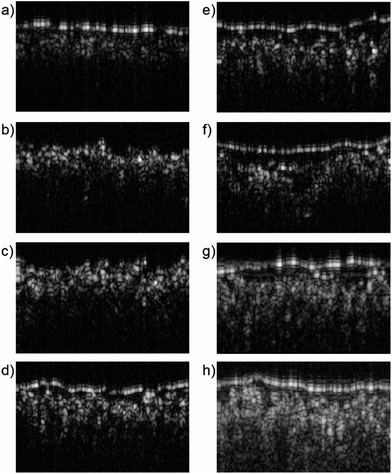 |
| Fig. 6 OCT B-scans of (a) Japine, (b) KK, (c) unmodified cotton paper and parchmentized cotton papers with initial moisture conditions of (d) dry, (e) humidified, and (f) pre-wet. A portion of the humidified parchmentized paper was coated with (g) linseed oil or (h) beeswax. Three adjacent B-scans were averaged to enhance the signal-to-noise. The image width corresponds to 0.5 mm. The z-axis is not to scale. | |
Conclusions
Both non-sampling (ATR-FTIR) and sampling (GCMS) analyses point to the conclusion that the Japine surface is the result of chemical modification of the paper fibers rather than the application of a coating. While the model parchmentized cotton papers correlate very well with Japine papers, it cannot be claimed that the methodologies used by the authors are those of the Platinotype Co. Examining the SEM and OCT images of all samples shows that the prepared parchmentized papers possessed a rougher surface than the Japine paper. It was observed by Bücher that trapping of air during the acid treatment could lead to air bubbles, which could form ruptures on the paper surface during drying.16 Parchmentization of the cotton paper was performed by hand and involved minimal treatment after preparation (air drying, humidification, and flattening) and before sensitization and printing. The commercial papers produced by the Platinotype Co. were prepared on industrial machinery and produced a number of finishes (e.g., matte to semi-gloss). Unfortunately, only the one identified sample was available for the current study.
Further investigation will be required to identify specific conservation challenges presented by these Japine papers. Warwicker noted that cotton treated with sulfuric acid at concentrations above 62.5% underwent a greater extent of acid hydrolysis when later exposed to 1 N HCl for 1 h.18 Exposure of photographic materials to such concentrated acid would not be expected, but it indicates that parchmentized papers are more susceptible to acidic attack. The presence of sulfate groups has been shown to accelerate the thermal degradation of cellulose.52 ATR-FTIR and SEM-EDS analysis of the Japine paper did not indicate the presence of sulfate groups, which suggests the paper was carefully prepared to minimize sulfur content during parchmentization. Cellulose degradation has been the subject of many reports,53–55 with modified papers requiring special attention.56 Unique aging characteristics may be identified for papers with parchmentized surfaces. Additionally, few studies have focused on photographic papers with such modified surfaces, and it is quite possible that some silver papers that have been assumed to be gelatin without a baryta subbing may in fact be silver prints on parchmentized paper.
Acknowledgements
The authors thank Lisa Barro and Silvia Centeno (Metropolitan Museum of Art) and Anna Vila (Statens Museum for Kunst, Copenhagen, Denmark) for the Japine sample and research discussions. We are grateful to Mike Ware for his guidance, printing on the parchmentized papers, and providing the Platinotype KK sample. We thank Ian Leake for transcription of the Willis patents. We also thank Jeeseong Hwang at the National Institute of Standards and Technology for his assistance with the OCT measurements. We thank Sarah Wagner and Alisha Chipman (National Gallery of Art) respectively for helpful comments and preparation of the oiled and waxed samples for OCT. Matthew L. Clarke was supported by a grant from The Andrew W. Mellon Foundation.
Notes and references
-
W. Willis Jr, Br. Pat. 2011, 1873.
-
Photo-Era Mag., ed. T. H Cummings, 1906, 17, 274 Search PubMed.
-
Photo-Miniat., ed. J. A. Tennant, 1916, 13, 229–230 Search PubMed.
-
Amat. Photogr. Photogr. News, ed. F. J. Mortimer, 1917, 65, 180 Search PubMed.
- C. McCabe, M. L. Clarke, C. A. Maines, L. Barro, S. A. Centeno and A. Vila, Top. Photogr. Preserv., 2013, 15 Search PubMed , in press.
- W. H. Smith, Photogr. J., 1915, 55, 282–286 Search PubMed.
- E. A. Salt, Photogr. J., 1929, 69, 509–513 Search PubMed.
- L. Barro, Top. Photogr. Preserv., 2003, 10, 37–54 Search PubMed.
-
W. Willis Jr, Br. Pat., 20022, 1913.
- A. W. Hofmann, Pharm. J. Trans., 1858, 18, 273–278 Search PubMed.
- C. Laroque, Pap. Conserv., 2004, 28, 17–32 CrossRef.
-
R. S. Laxman and A. H. Lachke, in Handbook of Plant-Based Biofuels, ed. A. Pandey, CRC Press, Boca Raton, FL, 2009, p. 129 Search PubMed.
-
L. E. Andés, The Treatment of Paper for Special Purposes, Scott, Greenwood & Son, London, 1907 Search PubMed.
-
J. Bersch, Cellulose, Cellulose Products, and Artificial Rubber, H. C. Baird & Company, London, 1904 Search PubMed.
-
Henley's Twentieth Century Book of Recipes, Formulas and Processes, ed. G. D. Hiscox, The Norman W. Henley Publishing Company, New York, 1907 Search PubMed.
- H. Bücher, Das Papier, 1957, 11, 125–133 Search PubMed.
- J. O. Warwicker, J. Appl. Polym. Sci., 1969, 13, 41–54 CrossRef CAS.
- J. O. Warwicker and J. W. Clayton, J. Appl. Polym. Sci., 1969, 13, 1037–1048 CrossRef CAS.
-
H. A. Krässig, Cellulose, CRC Press, Amsterdam, 1993 Search PubMed.
- A. C. O'Sullivan, Cellulose, 1997, 4, 173–207 CrossRef CAS.
-
The Platinotype: Simplest Photographic Process, Willis & Clements, Philadelphia, 1908 Search PubMed.
-
B. L. Browning, Analysis of Paper, Marcel Dekker, Inc., New York, 1969 Search PubMed.
- S. L. Vallance, B. W. Singer, S. M. Hitchen and J. H. Townsend, J. Am. Inst. Conserv., 1998, 37, 294–311 Search PubMed.
- A. M. Stephen, M. Kaplan, G. L. Taylor and E. C. Leisegang, Tetrahedron, 1966, 22(supp. 7), 233–240 CrossRef.
-
S. W. de W. Abney and L. Clark, Platinotype, its preparation and manipulation, The Scovill & Adams Company of New York, 1889 Search PubMed.
- R. C. Chang, P. Johnson, C. M. Stafford and J. Hwang, Biomed. Opt. Express, 2012, 3, 1326–1339 CrossRef PubMed.
-
W. Willis Jr, Br. Pat., 2800, 1878.
-
W. Willis Jr, Br. Pat., 16003, 1887.
- P. Garside and P. Wyeth, Stud. Conserv., 2003, 48, 269–275 CAS.
- L. M. Proniewicz, C. Paluszkiewicz, A. Wesełucha-Birczyńska, H. Majcherczyk, A. Barański and A. Konieczna, J. Mol. Struct., 2001, 596, 163–169 CrossRef CAS.
- P. Calvini and A. Gorassini, Restaurator, 2002, 23, 48–66 CAS.
- M. L. Nelson and R. T. O'Connor, J. Appl. Polym. Sci., 1964, 8, 1311–1324 CrossRef CAS.
-
R. G. Zhbankov, Infrared Spectra of Cellulose and Its Derivatives, Consultants Bureau, New York, 1966 Search PubMed.
- J. Blackwell, P. D. Vasko and J. L. Koenig, J. Appl. Phys., 1970, 41, 4375–4379 CrossRef CAS.
- C. Gilbert, S. Kokot and U. Meyer, Appl. Spectrosc., 1993, 47, 741–748 CrossRef CAS.
- Y. Sun, L. Lin, H. Deng, J. Li, B. He, R. Sun and P. Ouyang, BioResources, 2008, 3, 297–315 CAS.
- M. C. D'Antonio, A. Wladimirsky, D. Palacios, L. Coggiolaa, A. C. González-Baró, E. J. Baran and R. C. Mercader, J. Braz. Chem. Soc., 2009, 20, 445–450 CrossRef CAS PubMed.
- C. B. Mendive, D. W. Bahnemann and M. A. Blesa, Catal. Today, 2005, 101, 237–244 CrossRef CAS PubMed.
- M. Muruganandham, R. Amutha, B. Ahmmad, E. Repo and M. Sillanpää, J. Phys. Chem. C, 2010, 114, 22493–22501 CAS.
- S. Y. Oh, D. I. Yoo, Y. Shin and G. Seo, Carbohydr. Res., 2005, 340, 417–428 CrossRef CAS PubMed.
- G. Chen, B. Zhang, J. Zhao and H. Chen, Carbohydr. Polym., 2013, 95, 332–337 CrossRef CAS PubMed.
- L. Pereira, S. F. Gheda and P. J. Ribeiro-Claro, Int. J. Carbohydr. Chem., 2013, 2013, 1–7 Search PubMed.
- F. Camacho, P. González-Tello, E. Jurado and A. Robles, J. Chem. Technol. Biotechnol., 1996, 67, 350–356 CrossRef CAS.
-
H. Wenzl, The Chemical Technology of Wood, Academic Press, New York, 1970 Search PubMed.
- C. Y. Liang and R. H. Marchessault, J. Polym. Sci., 1959, 39, 269–278 CrossRef CAS.
- H. B. Berkeley, Pr. Photogr., 1881, 5, 147–149 Search PubMed.
-
C. McCabe, in Coatings on Photographs: Materials, Techniques, and Conservation, ed. C. McCabe, 2005, pp. 300–313 Search PubMed.
- P. Targowski, M. Góra and M. Wojtkowski, Laser Chem., 2006, 2006, 1–11 Search PubMed.
- D. C. Adler, J. Stenger, I. Gorczynska, H. Lie, T. Hensick, R. Spronk, S. Wolohojian, N. Khandekar, J. Y. Jiang, S. Barry, A. E. Cable, R. Huber and J. G. Fujimoto, Opt. Express, 2007, 15, 15972–15986 CrossRef.
- E. Alarousu, L. Krehut, T. Prykäri and R. Myllylä, Meas. Sci. Technol., 2005, 16, 1131 CrossRef CAS.
- M. Góra, M. Pircher, E. Götzinger, T. Bajraszewski, M. Strlic, J. Kolar, C. K. Hitzenberger and P. Targowski, Laser Chem., 2006, 2006, 1–6 Search PubMed.
- M. Roman and W. T. Winter, Biomacromolecules, 2004, 5, 1671–1677 CrossRef CAS PubMed.
- S. Soares, G. Camino and S. Levchik, Polym. Degrad. Stab., 1998, 62, 25–31 CrossRef CAS.
- C. H. Stephens, T. Barrett, P. M. Whitmore, J. A. Wade, J. Mazurek and M. Schilling, J. Am. Inst. Conserv., 2008, 47, 201–215 CrossRef PubMed.
- M. C. Area and H. Cheradame, BioResources, 2011, 6, 5307–5337 CAS.
- D. van der Reyden, C. Hofmann and M. Baker, J. Am. Inst. Conserv., 1993, 32, 177 Search PubMed.
|
This journal is © The Royal Society of Chemistry 2014 |