DOI:
10.1039/C3PY00879G
(Communication)
Polym. Chem., 2014,
5, 37-42
Manganese(III) acetylacetonate initiated RAFT polymerizations: an alternative and versatile RAFT initiator†
Received
4th July 2013
, Accepted 26th July 2013
First published on 31st July 2013
Abstract
This work describes RAFT polymerization with manganese(III) acetylacetonate (Mn(acac)3) as an initiator. Initiated by Mn(acac)3, RAFT polymerization of poly(vinyl acetate) was achieved at 80 °C mediated by a xanthate, methyl 2-(ethoxycarbonothioylthio)propanoate. The polymerization manifested good control at a low concentration of Mn(acac)3 (approximately 157 ppm). The polymer was endowed with a high chain end fidelity (97%), which was comparable or even better in comparison with the azobisisobutyronitrile-initiated polymerization. The polymerization behavior was revealed to comply with the features of a typical RAFT process. Successful Mn(acac)3-initiated RAFT polymerization of methyl methacrylate at 25 °C with a typical dithioester as a RAFT agent, and styrene at 100 °C with a dithiocarbamate as a RAFT agent demonstrated that Mn(acac)3 was a versatile and superior RAFT initiator.
Introduction
In the last few decades, there has been a rapid increase in attention being paid to so-called macromolecular engineering, that is, the synthesis of novel polymeric materials with well-defined molecular parameters. In this respect, controlled radical polymerization (CRP) has been proved to be a robust and versatile strategy due to its mild conditions, widely available monomers and simplicity. To date, nitroxide-mediated polymerization (NMP),1 atom transfer radical polymerization (ATRP),2 and reversible addition–fragmentation chain-transfer (RAFT)3 polymerization are among the most popular CRP techniques for polymer synthesis with tailor-made architectures. Among them, RAFT polymerization is one of the most frequently used techniques by addition of an appropriate RAFT agent (chain transfer agent) in a conventional radical polymerization. Several different kinds of RAFT agent have been developed, including dithioesters, trithiocarbonates, xanthates, dithiocarbamates, etc. It is crucial to select an appropriate RAFT agent for a particular monomer by following the RAFT criteria.4 For example, different kinds of RAFT agent should be used for active monomers5 and less active monomers.6–8 As for the initiator, any source of radicals for conventional radical polymerization can be used for RAFT polymerization, such as 2,2′-azobisisobutyronitrile,9 benzoyl peroxide,10 4,4′-azobis(4-cyanopentanoic acid), potassium persulfate,6 and tetraethylthiuram disulfide.11 RAFT polymerization of styrene (St) can also be initiated thermally between 100 and 120 °C.12 UV irradiation,13 γ-sources,14 single-pulse pulsed-laser techniques15 and plasma initiation16 have also been reported as initiation methods for RAFT polymerization. RAFT polymerization of methyl methacrylate (MMA) has been initiated via thermal initiation by our group.17,18 For RAFT polymerization, higher temperatures (above 60 °C) are usually applied to achieve higher polymerization rates and narrower molecular weight distributions.19
It has been reported that transition metal acetylacetonate (Mt(acac)x) was able to initiate radical polymerizations of vinyl monomers.20,21 Mn(acac)3 in combination with a cocatalyst such as acetic acid22 or benzyl bromide (BzBr)20e can act as a highly active radical initiator for the radical polymerization of various monomers, such as St and MMA. It has also been reported that the polymerization of MMA with Mn(acac)3 in the presence of organic halides at high temperatures (60 °C or 80 °C) can provide PMMA with controlled molecular weights; however, broader molecular weight distributions (Mw/Mn ≈ 2.0–3.0) were obtained.20b Polymerization proceeded with Mn(acac)3–BzBr and it was also possible to control the molecular weight of poly(styrene) at 80 °C; nevertheless, a lower monomer conversion (lower than 60%) and broader molecular weight distribution (Mw/Mn ≈ 2.0–3.0) were found.20e
It has been reported that a typical RAFT agent can be used as a pseudoalkyl halide initiator for CRP while maintaining good control over molecular weights and living nature of the polymerization.23 In these polymerizations, a concurrent CRP mechanism was proposed wherein the RAFT agent played a dual role as both the pseudoalkyl halide initiator and the chain transfer agent. Inspired by the initiator capability of Mn(acac)3 and the moderate control over molecular weight in the presence of a Mn(acac)3–alkyl halide, in this work, polymerization with a typical RAFT agent instead of an alkyl halide compound, i.e., Mn(acac)3–RAFT agent, was explored. Different types of monomers and RAFT agents as well as different polymerization temperatures were tested for wide usability.
Experimental section
Materials
Vinyl acetate (VAc) (>99%), methyl methacrylate (MMA) (>99%) and styrene (St) (>99%) monomers were purchased from Shanghai Chemical Reagents (Shanghai, China). They were all purified by passing through a column of basic alumina and kept in a refrigerator at −18 °C for short-term storage. Manganese(III) acetylacetonate (Mn(acac)3, metals basis) and zero-valent iron powder (<200 mesh, 99+%, metals basis) were purchased from Alfa Aesar and used without further purification. Copper (75 μm powder, 99%) was obtained from Sigma-Aldrich. 2,2′-Azobisisobutyronitrile (AIBN, >99%, Shanghai Chemical Reagent Co.) was recrystallized three times from ethanol and stored in a refrigerator at 0 °C. Methyl 2-(ethoxycarbonothioylthio)propanoate (MECTP) was synthesized according to the literature,24 with a purity higher than 95% by HPLC (Waters 515 HPLC) and kept in a refrigerator at 0 °C for short-term storage: 1H NMR, δ: 4.56–4.68 (q, 2H), 4.35–4.45 (q, H), 3.75 (s, 3H), 1.54–1.60 (d, 3H), 1.38–1.44 (t, 3H)). 2-Cyanoprop-2-yl 1-dithionaphthalate (CPDN) was synthesized according to a method reported elsewhere,25 with a purity higher than 96% (Waters 515 HPLC; 1H NMR, δ: 1.95 (s, 6H), 7.42 (m, 2H), 7.51 (m, 2H), 7.85 (m, 2H), and 8.10 (m, 1H)). Benzyl 4H-1,2,4-triazole-4-carbodithioate (BTCD) was synthesized according to the literature26 with a purity of 99% by HPLC (Waters 515 HPLC, 1H NMR, δ: 8.40 (s, 2H), 7.02–7.11 (m, 5H), 4.13 (s, 2H)) and kept in a refrigerator at 0 °C. All other chemicals were purchased from Shanghai Chemical Reagents and used as received unless specifically indicated.
Characterization
The number-average molecular weights (Mn) and molecular weight distributions (Mw/Mn) of the polymers were determined with Waters 1515 gel permeation chromatograph (SEC) equipped with a refractive index detector (Waters 2412), using HR 1 (pore size: 100 Å, 100–5000 Da), HR 2 (pore size: 500 Å, 500–20
000 Da), and HR 4 (pore size 10
000 Å, 50–100
000 Da) columns (7.8 × 300 mm, 5 μm bead size) with molecular weights in the range 102–5 × 105 g mol−1. THF was used as an eluent at a flow rate of 1.0 mL min−1 at 30 °C. The SEC samples were injected using a Waters 717 plus autosampler and calibrated with a poly(methyl methacrylate) standard from Waters for the PMMA & PVAc samples and a poly(styrene) standard for the PSt samples. The molecular weight of poly(vinyl acetate) (PVAc) was calculated using the universal calibration principle and Mark–Houwink parameters (PMMA: K = 7.56 × 10−5 dL g−1, α = 0.731; PVAc: K = 4.94 × 10−5 dL g−1, α = 0.829).271H NMR spectra of the precipitated polymers were recorded on an INOVA 400 MHz nuclear magnetic resonance instrument using CDCl3 as the solvent and tetramethylsilane (TMS) as the internal standard.
Typical procedures for RAFT polymerization of VAc
Monomer (VAc, 1.0 mL, 10.8 mmol), xanthate (MECTP, 11.8 μL, 0.054 mmol), and Mn(acac)3 (19.2 mg, 0.054 mmol) were added to a 5 mL ampoule in the following order: Mn(acac)3, VAc, and xanthate. The solution was deoxygenated with six standard freeze–pump–thaw cycles. The ampoule was then flame sealed and placed in the RCT basic S25 magnetic stirrer of IKA stirring at 900 rpm at 80 ± 0.1 °C. After 1 h, the ampoule was cooled by immersion in ice water. Afterward, the ampoule was opened and the content was dissolved in 5 mL THF, and then precipitated into 200 mL of cool n-hexane with stirring. The polymer was isolated by filtration and dried under vacuum until a constant weight was obtained at room temperature. The monomer conversion was 38.5% as determined by gravimetric calculation. Mn and Mw/Mn values were determined by SEC with poly(methyl methacrylate) (PMMA) standards (Mn,SEC = 5560 g mol−1, Mw/Mn = 1.23).
The polymerization procedure of MMA and St was similar to that for VAc, except that the flame sealed ampoule was placed in a stirring water bath equipped with a thermostat at 25 ± 0.1 °C for MMA and a oil bath at 100 ± 0.1 °C for St. The final obtained solution was precipitated into 200 mL of cool methanol (0 °C) with stirring.
Chain extension of PVAc using PVAc as the macroinitiator
A predetermined quantity of obtained PVAc was dissolved in a predetermined quantity of VAc with [VAc]0
:
[PVAc]0
:
[Mn(acac)3]0 = 500
:
1
:
1. The polymerization temperature was then stabilized at 80 ± 0.1 °C. The rest of the procedure was identical to that described earlier except that xanthate was replaced by obtained PVAc.
Results and discussions
Polymerization of VAc mediated by xanthate–Mn(acac)3
As is well known, the CRP of vinyl acetate (VAc) in the presence of a transition metal is always a challenging topic. The controlled polymerization of VAc was successfully realized when mediated with a cobalt(II) acetylacetonate complex (Co(acac)2).28 In this work, the polymerization of VAc in the presence of Mn(acac)3 and a xanthate, methyl 2-(ethoxycarbonothioylthio)propanoate, (MECTP), was investigated at 80 °C. The behavior of the polymerization, including the polymerization rate and the living polymerization characteristics, was the main focus. Fig. 1 summarizes the results of the polymerization of VAc with different concentrations of Mn(acac)3. The molar ratios of [VAc]0
:
[MECTP]0
:
[Mn(acac)3]0 were 200
:
1
:
0.2, 200
:
1
:
1 and 200
:
1
:
4 (200
:
1
:
0.5, 200
:
1
:
2 and 200
:
1
:
3 in Fig. S1, ESI†), respectively, with a constant concentration of MECTP. As shown in Fig. 1a, the polymerization proceeded smoothly and the rate of the polymerization was slightly lower with the considerable decrease of the Mn(acac)3 concentration. Even with a very low concentration of Mn(acac)3, such as [VAc]0
:
[MECTP]0
:
[Mn(acac)3]0 = 200
:
1
:
0.1 or 0.05 ([Mn]0 = 157 ppm), the polymerization was able to produce considerable conversion. The kinetics plots were not pseudo first-order linear ones but curvatures, indicating the occurrence of irreversible transfer reactions or termination at high conversions.29 Similar results were also found for the RAFT polymerizations of VAc.30 The very reactive VAc propagating radical was supposed to be responsible for irreversible transfer reactions or terminations,30 which became pronounced at high molecular weights as well as with high viscosity of the polymerization medium. Fig. 1b describes the number-average molecular weights (Mn,SEC) and molecular weight distributions (Mw/Mn) as functions of VAc conversion at different Mn(acac)3 feed concentrations. The molecular weights of PVAc increased linearly with monomer conversion and were somewhat lower than their corresponding theoretical ones. The molecular weight distributions remained narrow in most cases (Mw/Mn < 1.50) and became narrower with decreasing Mn(acac)3 concentration (Mw/Mn < 1.35 for [VAc]0
:
[MECTP]0
:
[Mn(acac)3]0 = 200
:
1
:
0.2, Table 1: entries 1–3, Mw/Mn < 1.25), although the polymerization rate was obviously slow. SEC traces of PVAc showed unimodal profiles with different ratios of [VAc]0
:
[MECTP]0
:
[Mn(acac)3]0 (Fig. S2, ESI†). The living nature of the polymer was also confirmed by 1H NMR (Fig. S3a, ESI†) with high chain-end fidelity (Fn = 97.1%) and successful chain extension reaction (Fig. S4, ESI†). All this evidence shows that the polymerization proceeded in a controlled manner in the presence of MECTP and Mn(acac)3.
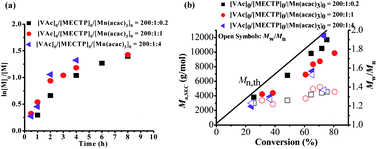 |
| Fig. 1 (a) Kinetic investigation (ln([M]0/[M]) versus time) and (b) dependence of Mn,SEC and Mw/Mn on the conversion of the bulk polymerization of VAc mediated by Mn(acac)3 in combination with MECTP at 80 °C with different amounts of Mn(acac)3. VAc = 1.0 mL. [M]0 and [M] refer to the initial concentration and instant concentration of VAc, respectively. Mn,th = ([VAc]0/[MECTP]0) × MVAc × conversion + MMECTP, where MVAc and MMECTP represent the molecular weights of VAc and MECTP, respectively. | |
Table 1 Control polymerizations of VAc under various conditions
Entrya |
Conditions |
Time (h) |
Conv. (%) |
M
n,SEC (g mol−1) |
M
w/Mn |
M
n,th (g mol−1) |
Polymerization conditions: VAc = 1.0 mL, VAc = 200 equiv., temperature = 80 °C. MECTP: methyl 2-(ethoxycarbonothioylthio)propanoate. AIBN: 2,2′-azobisisobutyronitrile. Entries 1, 2, 3, 6, 7, 8 and 9: MECTP = 1.0 equiv.
Entry 3: [Mn]0 = 157 ppm.
Entries 4 and 5: no MECTP.
|
1 |
Mn(acac)3 = 0.1 equiv. |
3 |
10.3 |
1900 |
1.20 |
2000 |
2 |
Mn(acac)3 = 0.1 equiv. |
7.5 |
40.9 |
8500 |
1.23 |
7200 |
3b |
Mn(acac)3 = 0.05 equiv. |
57 |
23.7 |
4000 |
1.18 |
4300 |
4c |
Mn(acac)3 = 1.0 equiv. |
0.5 |
38.2 |
99 900 |
1.65 |
— |
5c |
Mn(acac)3 = 1.0 equiv. |
1 |
58.7 |
98 900 |
1.84 |
— |
6 |
AIBN = 0.1 equiv. |
1 |
32.4 |
7600 |
1.29 |
5500 |
7 |
AIBN = 0.1 equiv. |
2.3 |
81.7 |
18 900 |
1.47 |
14 000 |
8 |
AIBN = 0.05 equiv. |
2.5 |
42.8 |
8400 |
1.36 |
7400 |
9 |
AIBN = 0.05 equiv. |
6 |
55.1 |
10 200 |
1.39 |
9500 |
10 |
No radical source |
168 |
0 |
— |
— |
— |
11 |
No radical source |
600 |
0 |
— |
— |
— |
The effect of MECTP concentration on the polymerization was also investigated. The molar ratio of [VAc]0
:
[Mn(acac)3]0 was kept at 200
:
1. It was found from Fig. 2a that the polymerization proceeded smoothly at various MECTP concentrations (here, the polymerization results of [VAc]0
:
[MECTP]0
:
[Mn(acac)3]0 = 200
:
1
:
1 was repeatedly illustrated for a better comparison). The polymerization rate was slightly lowered as the concentration of MECTP increased from [VAc]0
:
[MECTP]0
:
[Mn(acac)3]0 = 200
:
0.5
:
1 through 200
:
1
:
1 to 200
:
2
:
1, in accordance with the RAFT polymerization mechanism.33Fig. 2b shows that the Mn,SEC increased linearly with VAc conversion and deviated slightly from the theoretical ones. The Mw/Mn were below 1.45 in most cases, and decreased with increasing MECTP concentration (with [VAc]0
:
[MECTP]0
:
[Mn(acac)3]0 = 200
:
2
:
1, Mw/Mn < 1.30). SEC elution traces showed typical unimodal distributions for all cases (Fig. S5, ESI†). However, in the absence of MECTP, the polymerizations were out of control with uncontrollable molecular weights and much higher Mw/Mn values as evidenced from entries 4 & 5 (Table 1). The above results indicated that the polymerization in the presence of MECTP–Mn(acac)3 was most probably via a RAFT process with MECTP acting as RAFT agent and Mn(acac)3 as the radical initiator. Meanwhile, in order to make a comparison between the polymerization initiated by AIBN and Mn(acac)3, the AIBN initiated polymerization was conducted under equivalent conditions and the polymerization profiles are shown in Table 1 (entries 6–9) and Fig. S6 (ESI†). The polymerizations proceeded faster, even with low concentrations of AIBN. An induction period (less than 30 minutes) uniformly occurred in these polymerizations. An induction period in a RAFT process is frequently observed due to the pre-equilibrium process in the RAFT mechanism.31 During the pre-equilibrium process, the radicals generated from the initiators react with the RAFT agent to generate new initiating radicals. Some authors defined this induction period in RAFT polymerization as “initialization” as the process by which the starting RAFT agent was consumed.32 The molecular weights of PVAc coincided well with the theoretical ones with Mw/Mn < 1.50 in the cases of [VAc]0
:
[MECTP]0
:
[AIBN]0 = 200
:
1
:
0.2 and 200
:
1
:
1, while the polymerization was totally uncontrolled with [VAc]0
:
[MECTP]0
:
[AIBN]0 = 200
:
1
:
4. Nevertheless, the polydispersities of the polymer initiated by Mn(acac)3 are slightly lower than those from AIBN initiated polymerization as shown in entries 1–9. Also, the chain end fidelity of the former (Fn = 97.1%) was much higher than that of the latter (Fn = 80.2%, Fig. S3 in ESI†) with an approximately equal degree of polymerization. These results demonstrated that the controllability of Mn(acac)3 initiated polymerization of VAc was comparable or even better in comparison with those initiated by AIBN, despite its slower polymerization. Control experiments with [VAc]0
:
[MECTP]0 = 200
:
1 at 80 °C were conducted in the absence of Mn(acac)3 (Table 1, entries 10 & 11). There was no polymer yield within a week, suggesting that the combination of VAc and xanthate (MECTP) could not produce the radical initiating species. This result combined with the control experiments (radical trapped by 2,2,6,6-tetramethylpiperidinyl-1-oxy: TEMPO, Fig. S8) validated further the initiator role of Mn(acac)3. Based on the above discussion, it can be concluded that the polymerization mediated by MECTP–Mn(acac)3 complied with a typical RAFT mechanism with METCP as the RAFT agent, and Mn(acac)3 as the radical initiator, as illustrated in Scheme 1.
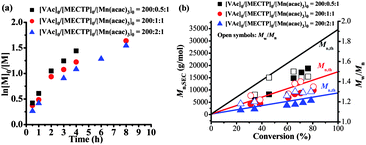 |
| Fig. 2 (a) Kinetic investigation (ln([M]0/[M]) versus time); (b) dependence of Mn,SEC and Mw/Mn on the conversion of the bulk polymerization of VAc mediated by Mn(acac)3 at 80 °C with different concentrations of MECTP. VAc = 1.0 mL. [M]0 and [M] refer to the initial concentration and instant concentration of VAc, respectively. Mn,th = ([VAc]0/[MECTP]0) × MVAc × conversion + MMECTP, where MVAc and MMECTP represent the molecular weights of VAc and MECTP, respectively. | |
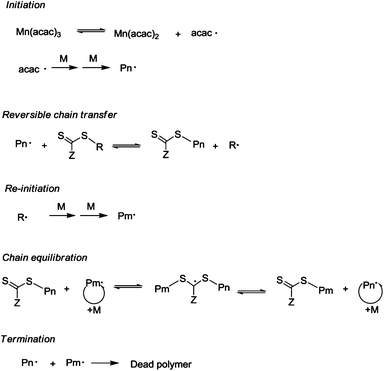 |
| Scheme 1 Proposed mechanism of Mn(acac)3 mediated polymerization controlled by CTAs. | |
Polymerization of other monomers in the presence of RAFT agent–Mn(acac)3
The versatility of the RAFT agent–Mn(acac)3 mediated controlled polymerization was assessed by using different monomers. The widely commercial monomers, methyl methacrylate (MMA) and styrene (St), were used as model monomers. Fig. 3 and 4 summarize the polymerization data for MMA in the presence of Mn(acac)3 in combination with 2-cyanoprop-2-yl 1-dithionaphthalate (CPDN) in DMSO at room temperature. It can be seen from Fig. 3a and 4a that the polymerizations proceeded with pseudo first-order linear kinetic plots at different CPDN and Mn(acac)3 concentrations despite the occurrence of induction periods (about 3 hours), indicating a “living” process. The prolonged induction period here was due to the different RAFT agent used with respect to the cases in Fig. S6.† The polymerization rates were elevated by the increase of the Mn(acac)3 concentration (Fig. 3a) and the decrease of the CPDN concentration (Fig. 4a), agreeing with the RAFT mechanism. At room temperature, the polymerizations can produce considerable monomer conversions (>90% conversion) within 12 hours. Fig. 3b and 4b show Mn,SEC and Mw/Mn as functions of MMA conversion with different Mn(acac)3 and CPDN concentrations, respectively. The molecular weights of PMMA increased linearly with monomer conversion and the molecular weight distributions remained narrow (Mw/Mn < 1.25) for all cases. The Mn,SECs of PMMA were a little higher than the theoretical molecular weights. 1H NMR spectrum (Fig. S9, ESI†) showed that the PMMA was end-capped by a CPDN species with high fidelity (Fn = 92.7%). Analogously, the control experiments in Table S1 (ESI†) demonstrated that the polymerization of MMA mediated with CPDN–Mn(acac)3 was via a RAFT mechanism, and the initiating species from Mn(acac)3 could also be generated even at room temperature. All this evidence showed that the polymerization of MMA can also be well regulated by CPDN–Mn(acac)3via a RAFT mechanism at ambient temperature.
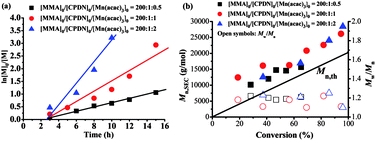 |
| Fig. 3 (a) ln([M]0/[M]) versus time and (b) dependence of Mn,SEC and Mw/Mn on the conversion of the polymerization of MMA at various concentrations of Mn(acac)3. MMA = 1.0 mL, DMSO = 0.5 mL, temperature = 25 °C. [M]0 and [M] refer to the initial concentration and instant concentration of MMA, respectively. Mn,th = ([MMA]0/[CPDN]0) × MMMA × conversion + MCPDN, where MMMA and MCPDN represent the molecular weights of MMA and CPDN, respectively. | |
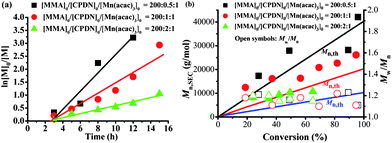 |
| Fig. 4 (a) ln([M]0/[M]) versus time and (b) dependence of Mn,SEC and Mw/Mn on the conversion of the polymerization of MMA mediated with Mn(acac)3 at various concentrations of CPDN. MMA = 1.0 mL, DMSO = 0.5 mL, temperature = 25 °C. [M]0 and [M] refer to the initial concentration and instant concentration of MMA, respectively. Mn,th = ([MMA]0/[CPDN]0) × MMMA × conversion + MCPDN, where MMMA and MCPDN represent the molecular weights of MMA and CPDN, respectively. | |
The bulk polymerization of St at 100 °C in the presence of Mn(acac)3 with a dithiocarbamate (benzyl 4H-1,2,4-triazole-4-carbodithioate, BTCD) as the RAFT agent was also explored. As shown in Table 2, the polymerization behavior was analogous with that of the polymerizations of MMA and VAc. The Mn,SECs of PSt were close to the theoretical molecular weights and the molecular weight distributions became narrower with increasing RAFT agent concentration (Mw/Mn < 1.30 with [St]0
:
[BTCD]0
:
[Mn(acac)3]0 = 200
:
2
:
1). The successful realization of the RAFT polymerization of St mediated by BTCD–Mn(acac)3 at 100 °C demonstrated that Mn(acac)3 was a versatile initiator for RAFT polymerization, which can be utilised for different kinds of monomers and RAFT agents; most importantly, a wide range of temperatures can be used with Mn(acac)3 as the initiator.
Table 2 BTCD–Mn(acac)3 mediated polymerizations of St at 100 °C
Entrya |
BTCD (equiv.) |
Time (h) |
Conv. (%) |
M
n,SEC (g mol−1) |
M
w/Mn |
M
n,th (g mol−1) |
Polymerization conditions: St = 1.0 mL, St = 200 equiv., Mn(acac)3 = 1.0 equiv.; temperature = 100 °C. BTCD = benzyl 4H-1,2,4-triazole-4-carbodithioate.
|
1 |
0.5 |
3 |
47.8 |
22 600 |
1.72 |
20 100 |
2 |
0.5 |
6 |
52.6 |
24 600 |
1.88 |
22 100 |
3 |
1.0 |
3 |
37.9 |
10 200 |
1.37 |
8100 |
4 |
1.0 |
10 |
64.9 |
17 900 |
1.47 |
13 700 |
5 |
2.0 |
3 |
30.5 |
4600 |
1.23 |
3400 |
6 |
2.0 |
6 |
36.5 |
6400 |
1.28 |
4000 |
Conclusions
In this work, Mn(acac)3 was demonstrated to be a versatile initiator for the RAFT polymerization of VAc, MMA and St with xanthate, dithioester or dithiocarbamate as the RAFT agent at 80 °C, 25 °C or 100 °C. The RAFT polymerizations initiated by Mn(acac)3 possessed RAFT features. The wide range of temperatures applicable for Mn(acac)3 made it a versatile and superior RAFT initiator. Also, this work supplied a new initiation approach for the room-temperature RAFT polymerization of MMA.
Acknowledgements
The financial support from the National Nature Science Foundation of China (21174094), the Nature Science Key Basic Research of Jiangsu Province for Higher Education (12KJA150007), the Priority Academic Program Development (PAPD) of Jiangsu Higher Education Institutions, and the Program of Innovative Research Team of Soochow University are gratefully acknowledged.
Notes and references
- C. J. Hawker, A. W. Bosman and E. Harth, Chem. Rev., 2001, 101, 3661 CrossRef CAS PubMed.
- K. Matyjaszewski and J. Xia, Chem. Rev., 2001, 101, 2921 CrossRef CAS PubMed.
-
(a) G. Moad, J. Chiefari, Y. K. Chong, J. Krstina, R. T. A. Mayadunne, A. Postma, E. Rizzardo and S. H. Thang, Polym. Int., 2000, 49, 993 CrossRef CAS;
(b) C. Boyer, M. H. Stenzel and T. P. Davis, J. Polym. Sci., Part A: Polym. Chem., 2011, 49, 551 CrossRef CAS.
-
(a) G. Moad, E. Rizzardo and S. H. Thang, Polymer, 2008, 49, 1079 CrossRef CAS PubMed;
(b) G. Moad, E. Rizzardo and S. H. Thang, Aust. J. Chem., 2006, 59, 669 CrossRef CAS;
(c) E. Rizzardo, M. Chen, B. Chong, G. Moad, M. Skidmore and S. H. Thang, Macromol. Symp., 2007, 248, 104 CrossRef CAS.
-
(a) Y. K. Chong, G. Moad, E. Rizzardo and S. Thang, Macromolecules, 2007, 40, 4446 CrossRef CAS;
(b) G. Moad, Y. K. Chong, E. Rizzardo, A. Postma and S. H. Thang, Polymer, 2005, 46, 8458 CrossRef CAS PubMed.
- G. Moad, E. Rizzardo and S. H. Thang, Aust. J. Chem., 2005, 58, 379 CrossRef CAS.
- E. Rizzardo, J. Chiefari, R. T. A. Mayadunne, G. Moad and S. H. Thang, ACS Symp. Ser., 2000, 768, 278 CrossRef CAS PubMed.
-
(a) D. Taton, A.-Z. Wilczewska and M. Destarac, Macromol. Rapid Commun., 2001, 22, 1497 CrossRef CAS;
(b) R. W. Simms, T. P. Davis and M. F. Cunningham, Macromol. Rapid Commun., 2005, 26, 592 CrossRef CAS;
(c) M. Destarac, D. Charmot, X. Frank and S. Z. Zard, Macromol. Rapid Commun., 2000, 21, 1035 CrossRef CAS;
(d) Y. Y. Tong, Y. Q. Dong, F. S. Du and Z. C. Li, Macromolecules, 2008, 41, 7339 CrossRef CAS;
(e) R. W. Simms, T. P. Davis and M. F. Cunningham, Macromol. Rapid Commun., 2005, 26, 592 CrossRef CAS;
(f) P. E. Dufils, G. David, B. Boutevin, G. Woodward, G. Otter, A. Guinaudeau, S. Mazieres and M. Destarac, J. Polym. Sci., Part A: Polym. Chem., 2012, 50, 1997 CrossRef CAS.
-
K. Matyjaszewski and T. P. Davis, Handbook of free radical initiators, Wiley, New York, 1st edn, 2002 Search PubMed.
-
(a) E. N. Savariar and S. Thayumanavan, J. Polym. Sci., Part A: Polym. Chem., 2004, 42, 6340 CrossRef CAS;
(b) M.-F. Llauro, J. Loiseau, F. Boisson, F. Delolme, C. Ladavière and J. Claverie, J. Polym. Sci., Part A: Polym. Chem., 2004, 42, 5439 CrossRef CAS;
(c) Y. C. Hu, Y. Liu and C. Y. Pan, J. Polym. Sci., Part A: Polym. Chem., 2004, 42, 4862 CrossRef CAS.
- X. Y. Wang, J. Zhu, D. Zhou and X. L. Zhu, Polymer, 2005, 46, 3515 CrossRef CAS PubMed.
- J. Chiefari, R. T. A. Mayadunne, C. L. Moad, G. Moad, E. Rizzardo, A. Postma, M. A. Skidmore and S. H. Thang, Macromolecules, 2003, 36, 2273 CrossRef CAS.
- J. F. Quinn, L. Barner, C. Barner-kowollik, E. Rizzardo and T. P. Davis, Macromolecules, 2002, 35, 7620 CrossRef CAS.
- L. Barner, J. F. Quinn, C. Barner-Kowollik, P. Vana and T. P. Davis, Eur. Polym. J., 2003, 39, 449 CrossRef CAS.
- M. Buback, T. Junkers and P. Vana, Macromol. Rapid Commun., 2005, 26, 796 CrossRef CAS.
-
(a) G. J. Chen, X. L. Zhu, J. Zhu and Z. P. Cheng, Macromol. Rapid Commun., 2004, 25, 818 CrossRef CAS;
(b) Z. B. Zhang, X. L. Zhu, J. Zhu and Z. P. Cheng, Polym. Bull., 2006, 56, 539 CrossRef CAS PubMed.
- Z. B. Zhang, X. L. Zhu, J. Zhu, Z. P. Cheng and S. P. Zhu, J. Polym. Sci., Part A: Polym. Chem., 2006, 44, 3343 CrossRef CAS.
- Z. B. Zhang, X. L. Zhu, J. Zhu and Z. P. Cheng, Polymer, 2006, 47, 6970 CrossRef CAS PubMed.
-
J. Chiefari and E. Rizzardo, Control of free radical polymerization by chain transfer methods, in Handbook of radical polymerization, ed. T. P. Davis and K. Matyjaszewski, John Wiley and Sons, Hoboken, NY, 2002, p. 263 Search PubMed.
-
(a) E. G. Kastning, H. Naarmann, H. Reis and C. Berding, Angew. Chem., Int. Ed., 1965, 77, 313 CrossRef CAS;
(b) K. Endo and A. Yachi, Polym. J., 2002, 34, 320 CrossRef CAS;
(c) V. D. L. Pelissoli, D. S. Nicole, J. Dullius, R. Ligabue and S. Einloft, J. Appl. Polym. Sci., 2010, 115, 1797 CrossRef;
(d) J. R. Caille, A. Debuigne and R. Jerome, J. Polym. Sci., Part A: Polym. Chem., 2005, 43, 2723 CrossRef CAS;
(e) K. Endo and A. Yachi, Polym. Bull., 2001, 46, 363 CrossRef CAS.
-
(a) B. B. Snider, Chem. Rev., 1996, 96, 339 CrossRef CAS PubMed;
(b) Y. Ishii and S. Sakaguchi, Catal. Surv. Jpn., 1999, 3, 27 CrossRef CAS.
-
(a) N. A. Lavrov, Russ. J. Appl. Chem., 1994, 67, 1358 Search PubMed;
(b) N. A. Lavrov, Russ. J. Appl. Chem., 1995, 68, 922 Search PubMed.
-
(a) Z. B. Zhang, W. Zhang, X. L. Zhu, Z. P. Cheng and J. Zhu, J. Polym. Sci., Part A: Polym. Chem., 2007, 45, 5722 CrossRef CAS;
(b) Y. Kwak, R. Nicolaÿ and K. Matyjaszewski, Aust. J. Chem., 2009, 62, 1384 CrossRef CAS;
(c) R. Nicolaÿ, Y. Kwak and K. Matyjaszewski, Macromolecules, 2008, 41, 4585 CrossRef;
(d) R. Nicolaÿ, Y. Kwak and K. Matyjaszewski, Macromolecules, 2008, 41, 6602 CrossRef;
(e) Y. Kwak and K. Matyjaszewski, Polym. Prepr., 2008, 49, 264 CAS;
(f) S. H. Subramanian, R. P. Babu and R. Dhamodharan, Macromolecules, 2008, 41, 262 CrossRef CAS;
(g) Z. B. Zhang, W. X. Wang, H. D. Xia, J. Zhu, W. Zhang and X. L. Zhu, Macromolecules, 2009, 42, 7360 CrossRef CAS.
- M. H. Stenzel, L. Cummins, G. E. Roberts, T. P. Davis and P. Vana, Macromol. Chem. Phys., 2003, 204, 1160 CrossRef CAS.
- J. Zhu, X. L. Zhu, Z. P. Cheng, F. Liu and J. M. Lu, Polymer, 2002, 43, 7037 CrossRef CAS.
- D. Zhou, X. L. Zhu, J. Zhu and H. S. Yin, J. Polym. Sci., Part A: Polym. Chem., 2005, 43, 4849 CrossRef CAS.
- T. Gruendling, T. Junkers, M. Guilhaus and C. Barner-Kowollik, Macromol. Chem. Phys., 2010, 211, 520 CrossRef CAS.
- A. Debuigne, J. R. Caille and R. Jérôme, Angew. Chem., Int. Ed., 2005, 44, 1101 CrossRef CAS PubMed.
-
K. Matyjaszewski and T. P. Davis, Handbook of radical polymerization, John Wiley & Sons, Inc, 2002, p.365 Search PubMed.
-
(a) A. Favier, C. Barner-Kowollik, T. P. Davis and M. H. Stenzel, Macromol. Chem. Phys., 2004, 205, 925 CrossRef CAS;
(b) M. H. Stenzel, L. Cummins, G. E. Roberts, T. P. Davis, P. Vana and C. Barner-Kowollik, Macromol. Chem. Phys., 2003, 204, 1160 CrossRef CAS;
(c)
C. A. Finch, Polyvinyl Alcohol: Developments and Properties, J. Wiley & Sons, New York, 1992, p. 691 Search PubMed;
(d) D. Britton, F. Heatley and P. A. Lovell, Macromolecules, 1998, 31, 2828 CrossRef CAS.
-
(a) S. Perrier, C. Barner-Kowollik, J. F. Quinn, P. Vana and T. P. Davis, Macromolecules, 2002, 35, 8300 CrossRef CAS;
(b) M. K. Ham, J. H. Youk, Y. K. Kwon and Y. Kwark, J. Polym. Sci., Part A: Polym. Chem., 2012, 50, 2389 CrossRef CAS;
(c) Y. F. Yan, W. Zhang, Y. S. Qiu, Z. B. Zhang, J. Zhu, Z. P. Cheng, W. D. Zhang and X. L. Zhu, J. Polym. Sci., Part A: Polym. Chem., 2010, 48, 5206 CrossRef CAS;
(d) C. Schilli, M. G. Lanzendörfer and A. H. E. Müller, Macromolecules, 2002, 35, 6819 CrossRef CAS;
(e) A. J. Convertine, B. S. Sumerlin, D. B. Thomas, A. B. Lowe and C. L. McCormick, Macromolecules, 2003, 36, 4679 CrossRef CAS;
(f) A. J. Convertine, B. S. Lokitz, Y. Vasileva, L. J. Myrick, C. W. Scales, A. B. Lowe and C. L. McCormick, Macromolecules, 2006, 39, 1724 CrossRef CAS;
(g) B. Ray, Y. Isobe, K. Matsumoto, S. Habaue, Y. Okamoto, M. Kamigaito and M. Sawamoto, Macromolecules, 2004, 37, 1702 CrossRef CAS.
-
(a) J. B. McLeary, F. M. Calitz, J. M. McKenzie, M. P. Tonge, R. D. Sanderson and B. Klumperman, Macromolecules, 2004, 37, 2383 CrossRef CAS;
(b) J. B. McLeary, J. M. Mckenzie, M. P. Tonge, R. D. Sanderson and B. Klumperman, Chem. Commun., 2004, 1950 RSC;
(c) J. B. McLeary, F. M. Calitz, J. M. McKenzie, M. P. Tonge, R. D. Sanderson and B. Klumperman, Macromolecules, 2005, 38, 3151 CrossRef CAS.
-
C. Barner-Kowollik, Handbook of RAFT Polymerization, Wiley-VCH, Weinheim, Germany, 2008 Search PubMed.
Footnote |
† Electronic supplementary information (ESI) available. See DOI: 10.1039/c3py00879g |
|
This journal is © The Royal Society of Chemistry 2014 |