Protein free microcapsules obtained from plant spores as a model for drug delivery: ibuprofen encapsulation, release and taste masking
Received 10th October 2012, Accepted 8th November 2012
First published on 9th November 2012
Abstract
Sporopollenin exine capsules (SEC) extracted from Lycopodium clavatum spores were shown to encapsulate ibuprofen as a drug model, with 97 ± 1% efficiency as measured by recovery of the loaded drug and absence of the drug on the SEC surface by scanning electron microscopy (SEM). The encapsulated ibuprofen was shown to be unchanged from its bulk crystalline form by solid state NMR, FTIR and XRD. Essential for drug delivery applications, SEC were shown to be non-toxic to human endothelial cells and free of allergenic protein epitopes by MALDI-TOF-MS and ESI-QqToF-MS. Potential application for targeted release into the intestinal region of the gastrointestinal tract (GIT) was demonstrated by 88 ± 1% of the drug being retained in simulated gastric fluid (SGF) after 45 minutes and 85 ± 2% being released after 5 min in buffer (PBS; pH 7.4). The SEC were shown to provide significant taste masking of encapsulated ibuprofen in a double blind trial with 10 human volunteers.
Introduction
Drug delivery using microcapsules is becoming increasingly important, endowing desirable features such as drug stability, targeting and controlled release.1–4 Microcapsule manufacture can be costly and difficult, particularly to obtain microcapsules with uniform morphology, size, chemical structure, and carrier capacity, together with efficient biodegradability and payload release. Plant spores were chosen as the source for the microcapsules used for this study, since along with pollen they have a number of identifiable advantages over conventional materials; however, materials from this source are often associated with the potential of being allergenic which is addressed in this paper. Advantageously, microcapsules extracted from pollen and spores are almost identical in size and morphology for a given species, readily obtainable in large bulk quantities in some cases, and renewable. A choice of size can be made from 5 μm (Myostis: forget-me-not) to 250 μm diameter (Cucurbita: pumpkin) although only some species can be obtained currently in quantities that would render them economically viable in large amounts: examples are Lycopodium clavatum spores and the pollen of Secale cereale and various species of pine. Currently pollens and spores are marketed as herbal remedies,5 nutraceuticals6,7 as well as being used in fireworks. The outer wall (the exine) of pollen and spores is constructed primarily of the bio-polymer sporopollenin. In comparison to other bio-polymers it is extraordinarily resistant to temperature, pressure and most chemicals,8 with the exception of strong oxidising. Such stability is reflected in sporopollenin being the sole bio-polymer to survive chemically and morphologically intact after millions of years in sedimentary rocks.9 Evacuation of the protoplasmic contents of plant spores and pollen give sporopollenin exine capsules (SEC). The protocols are simple and use inexpensive, non-toxic agents such as strong mineral acids and bases.8,10 SEC can also be obtained using a one-pot procedure.11,12 The exact structure and biosynthesis of sporopollenin are not fully understood, but it has been described as an oxidative polymer of carotenoids13 or polyunsaturated fatty acids.8,14–16 The high physical and chemical stability and uniformity characteristics of SEC from L. clavatum have led to their use as a solid phase support for peptide synthesis17,18 as a ion-exchange resin19 and micro-reaction vessel.20 Particular properties of SEC are their ability to readily microencapsulate a wide range of materials with high loadings.10,21 Additional properties of SEC include elasticity22 and permeability.23 The latter is due to the many narrow (20–40 nm diameter) multi-directional channels that penetrate the SEC giving passage to actives in and out of the relatively large chamber within the SEC. In addition, the morphology of the outer surface of SEC is peculiar to individual plant species. This property has been of use to forensic scientists24 over many years and may be important in adhesion properties required of pollen during pollination. Therefore, it might be that the outer surface architecture of SEC, such as shown in Fig. 1, could play a role in mucosal adhesion and assist in drug delivery properties.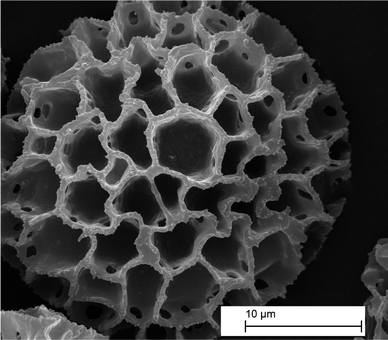 |
| Fig. 1 Showing surface architecture of SEC encapsulated with ibuprofen (1 : 1, w/w) but with no evidence of any solid deposits of ibuprofen. | |
The range of properties exhibited by SEC offer the potential for a wide range of applications in the field of microencapsulation including drug delivery.25,26 Of particular note, it has been shown that eicosapentaenoic acid (EPA) can be delivered into the bloodstream of humans with a 10-fold increase in bioavailability when administered orally as the ethyl ester encapsulated into SEC obtained from L. clavatum.25
The morphology of pollen and spore shells including their outer surfaces has been copied. Inorganic and polystyrene replicas of spore exines have been synthesised27,28 as microcapsules (size range 10–15 μm) and shown, in in vitro studies, that they can release encapsulated ibuprofen as the active component into buffer solution.27,28 Perhaps surprisingly, this property for this drug has not been demonstrated by the natural sporopollenin microcapsule, SEC, and nor has the ability been shown to model selective delivery to one part of gastrointestinal tract (GIT). Furthermore, the natural SEC have been shown to taste mask29 and partially degrade in human plasma30 but such properties have not been reported for replicas of SEC.
Controlled release throughout the GIT using microcapsules is attracting a variety of approaches currently using such as sodium alginate hydrogels,31 pectin–casein complexes32 and chitosan-denatured β-lactoglobin.33 In contrast to such materials, the sporopollenin of SEC is highly resilient to strong acid, strong alkali and a large range of different enzymes34,35 normally found in the GIT. From an in vivo perspective, pollen exines have been detected in human faeces in a number of studies.36,37 These reports are strongly in support of sporopollenin not being biodegraded throughout digestion in the GIT. Our recent oral drug delivery study, involving human volunteers, using SEC obtained from L. clavatum spores25 showed that the majority (circa 10-fold) of the drug (EPA) was released during passage through the intestinal region of the GIT. Therefore it was of interest to demonstrate the ability of an active to be released from the SEC triggered by the pH conditions found in the GIT. Ibuprofen was chosen as the active since it is conveniently accessed, assayed28 and provides a comparative model with other release studies performed using synthetic microcapsules such as ethyl cellulose microspheres36 and in particular, inorganic porous micron-sized particles made from silica, calcium carbonate or calcium phosphate.27 However, before SEC can be offered as a microcapsule for oral drug delivery, important factors that require addressing are the absence of allergenic proteins and non-toxicity. An additional bonus for oral delivery is the ability to taste mask. This study used SEC obtained from L. clavatum spores since the spores are commercially available and the SEC can be readily extracted by simple non-toxic reagents.
Results and discussion
Protein free and non-toxic SEC from L. clavatum spores
Exine preparation in this study employed a modification of the method described by Zetsche and Vicari37 from L. clavatum spores, which avoids the use of toxic reagents. Briefly, hot acetone was used initially to remove lipid followed by aqueous potassium hydroxide to evacuate sporoplasmic contents. The product obtained at this stage possesses two layers, an inner wall (the intine) composed of polysaccharides and outer (the exine) composed of sporopollenin. For this study the intine was removed by harsh treatment with orthophosphoric acid to give the final sporopollenin exine microcapsules (SEC). Despite robust processing, the SEC retains almost the same size and morphology of the original spores harvested from the L. clavatum plant. The spores are commercial availability in large quantities (e.g., Manoj Tibrewala, Nepal). They have a rounded form of 25 μm with a relatively large single internal cavity as depicted in an earlier study.21As reported previously,10,21,38 SEC extracted from L. clavatum spores have zero% N by combustion elemental analysis, which is indicative of the absence of allergenic proteins on the surface of SEC. However, legislative bodies such as the UK's Food Standards Agency provide guidance39 on more accurate methods to assure absence of allergenic protein residues in food products. Electrophoresis is proposed, but of particular note is the use of mass spectrometric (MS) techniques such as MALDI-TOF-MS and ESI-QqToF-MS. Therefore, a series of rigorous assays were repeated using sodium dodecyl sulphate-polyacrylamide gel electrophoresis (SDS-PAGE) and a variety of MS techniques to detect trace amounts of proteins or peptides. Initially, standard extraction procedures were followed that are normally used for the disruption of cells.40 Thus, repeated detergent-based lysis and corresponding sonication methods were applied to six repeat extraction samples of SEC.41 Attempts to detect the presence of proteins in the extracts by SDS-PAGE and the traditional Lowry method proved unsuccessful with all of the samples. In support of these observations none of the extracts obtained from any of the six repeat extracted SEC samples revealed the presence of protein by either electrospray ionization with a hybrid QqTOF instrument (ESI-QqTOF-MS) or MALDI-TOF/TOF-MS techniques in the positive ion mode. Therefore it can be concluded that SEC extracted from L. clavatum by the method used in this study, are free of allergenic protein, at least to the standards required by advisory bodies on food legislation.39 Based on the study herein, it is plausible that similar extraction methods might also generate sporopollenin microcapsules that are free of allergenic proteins thus offering scope for a wider range of sporopollenin microcapsules available with different sizes and morphologies to enlarge the range of applications in such as drug delivery, food, beverages, cosmetics and household products where the absence of protein allergens is essential.
Encapsulation of ibuprofen into SEC and release of the drug into simulated biological media
Encapsulation of a substance into SEC is perhaps more simple than conventional means of microencapsulation, such as spray drying. Different methods have been reported to fill SEC with an active;10,21 however, in this study an ethanolic solution of ibuprofen was drawn into the SEC under vacuum to achieve a loading of 1
:
1 w/w. The efficiency of loading was assessed by: (i) assaying the amount ibuprofen extracted from the SEC into ethanol; (ii) examination of the surface of the SEC for any evidence of drug deposits. Thus 97 ± 1% of ibuprofen was recovered from the SEC and found to be identical with the original drug without any additional peaks in the NMR or FTIR spectra indicating the absence of degradation by-products or contaminants extracted from the SEC. The drug loading of, 1
:
1 w/w (50% w/w) chosen for the study is at a competitive level with other types of microencapsulation.42,43 At this loading and on repeat runs, no evidence was observed, by scanning electron microscopy, of ibuprofen precipitating on the surface of the SEC (Fig. 1). A comparison of FTIR spectra of a ground mixture of bulk ibuprofen and SEC (1
:
1 w/w) and the encapsulated formulation showed no difference in wave numbers, suggesting no morphological changes or no detectable interaction between the two components. This was further supported by a similar comparative study using solid state 13C CP MAS NMR involving SEC alone, SEC plus bulk ibuprofen, ibuprofen encapsulated within SEC and bulk ibuprofen indicating that the bulk ibuprofen is unaltered in the encapsulated form (Fig. 2).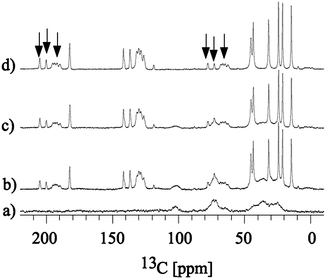 |
| Fig. 2 13C CP MAS NMR spectrum of (a) SEC, (b) SEC plus bulk ibuprofen, (c) ibuprofen encapsulated within SEC and (d) bulk ibuprofen. Spectra were collected at 20 °C, with 8 kHz sample spinning. Peaks labelled with arrows indicate spinning side bands. | |
Confirmation of encapsulated ibuprofen being in its crystalline form comparable with its bulk form was evident from a similar comparative study using XRD (Fig. 3). This result is in contrast to such as ibuprofen encapsulated into meso-structured silica which was found by XRD to be amorphous.44
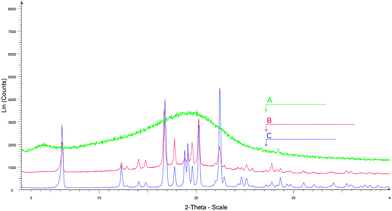 |
| Fig. 3 XRD of (A) SEC, (B) ibuprofen encapsulated within SEC and (C) bulk ibuprofen. | |
Release of ibuprofen into agitated environments of excess simulated gastric fluid (SGF) and phosphate buffer was followed over 45 minutes (Fig. 4; Table 1). To maintain comparability with release into each medium, each time point was achieved by a separate experiment in which the loaded SEC were charged to a fresh medium and assayed by determining the ibuprofen remaining in the SEC by extracting into ethanol. In SGF, the release profile formed a plateau after 5–10 min which was maintained over the duration (45 min) of the study, with an overall loss of some 12 ± 1% of the drug from the microcapsules indicating the feasibility of the SEC to a retain the majority (88 ± 1%) of the drug during transit through the stomach. In contrast when SEC at the same loading level of the drug, were agitated in an excess of phosphate buffer (pH 7.4) to mimic the change from the gastric environment, most (85 ± 2%) of the payload was released after 5 min. This comparative release study is indicative of suitably loaded SEC, with either active or excipient, being able to release the payload in accordance with the different pH conditions such as found in the GIT. It is of note that the morphology of SEC remained unchanged as viewed by SEM following exposure to SGF and PBS over 1 month.
 |
| Fig. 4 Release of ibuprofen from SEC into simulated gastric fluid (SGF) and phosphate buffer (PBS) to mimic intestinal fluid. | |
Table 1 Release of drug from ibuprofen loaded SEC (1
:
1 w/w) over time
Time (s) | % of ibuprofen released |
---|
SGF | PBS |
---|
5.0 | 8.0 ± 1.6 | 84.8 ± 2.2 |
15.0 | 10.0 ± 1.4 | 88.0 ± 2.0 |
30.0 | 12.1 ± 1.9 | 89.5 ± 1.2 |
45.0 | 12.2 ± 1.1 | 93.0 ± 2.1 |
Toxicity testing of SEC
Whilst pollen and plant spores are found in many foods and have been used as foods and medicines4 only little data has been published on toxicological assessment of sporopollenin in which sporopollenin from Chlorella vulgaris was fed in large quantities to rats with no ill effect.45 However, no assessments have been made with well recognised in vitro tests such as toxicity to human endothelial cells.46 Therefore SEC were exposed to two types of cells, namely human umbilical vein endothelial cells (HUVEC), which are endothelial cells extracted from the vein of an umbilical cord of the immortalised endothelial cell line EAhy 926. Four different assays were used to assess the effects of the SEC on endothelial cells: (i) WST-1 proliferation assay; (ii) cell death ELISA for apoptosis; (iii) LDH assay for necrosis; (iv) microscopic examination of the cells to look for cell damage and apoptotic bodies. All of the tests undertaken showed that SEC did not reduce the proliferation or induce cell death in human endothelial cells in vitro at concentrations between 100ng and 10ug. Furthermore, no cell damage was observed by SEM within this concentration range.Taste masking of ibuprofen by SEC
In keeping with the perspective of using SEC for oral delivery, a limited taste trial was undertaken using human volunteers. A comparison was made by administering powders to the tongues of the volunteers of ibuprofen alone, as a mixture with SEC and encapsulated into SEC. We have reported earlier that SEC obtained from L. clavatum exhibit taste masking properties for fish oils which are unpalatable to some people.29 However, ibuprofen is solid at room temperature and has a distinctly different taste to fish oil. It has a strong, astringent and bitter taste that is normally masked to make it easier to ingest.47 It is of note that human taste discrimination is highly evolved: there are 25 putatively bitter taste receptor genes coding for G protein-coupled receptors, referred to as the T2T family that can distinguish bitter stimuli and pungent compounds at low thresholds.48 With such high sensitivity it seemed that a taste masking study could reflect on the efficiency of encapsulation to give an indication of whether such a formulation may have viability for oral delivery without any additional excipient. Powdered drugs are a useful dosing form for patients who may experience difficulty in swallowing tablets or capsules. In addition, the development of medicines that are easier to ingest has attracted special interest from the viewpoints of treatment compliance and marketability.49 Other microspheres as powders have been developed for taste masking to protect the bitter tasting drugs from coming in to contact with the patients' taste buds.50 The double blind taste masking study reported herein (Table 2) was conducted with 10 human volunteers to compare, at randomized time points, the fine powders: bulk ibuprofen with SEC; bulk ibuprofen with ibuprofen encapsulated into SEC in the ratio 1
:
1 w/w; SEC with ibuprofen encapsulated into SEC in the ratio 1
:
1 w/w. This albeit limited study showed some clear distinctions. It was found that there was a significant chance that volunteers could distinguish between ibuprofen and empty SEC with a discordance ratio of 3
:
2. Also, the chance of a volunteer finding the same taste between SEC alone with ibuprofen encapsulated into SEC was marked with a discordant ratio of 3
:
1. However, most strikingly was that volunteers were 5 times (discordance ration of 5
:
1) more likely to detect a difference between ibuprofen alone and the ibuprofen encapsulated into SEC formulation. These data indicate that SEC offer a significant degree of taste masking which perhaps reflects that only a small proportion of the loaded ibuprofen emerges as being detectable, by taste, on the surface of the SEC.
Table 2 Double blind taste trial involving 10 volunteers comparing:a bulk ibuprofen with SEC;b bulk ibuprofen with ibuprofen encapsulated into SEC in the ratio 1
:
1 w/w;c SEC with ibuprofen encapsulated into SEC in the ratio 1
:
1 w/w
Time 2 | Time 1 |
---|
Taste same | Taste different | Total pairs |
---|
p-Value = 0.10; discordant odds ratio = 3 : 2. p-Value = 0.10; discordant odds ratio = 5 : 1. p-Value = 0.10; discordant odds ratio = 3 : 1. |
---|
Taste same | 1a, 6b, 6c | 3a, 5b, 3c | 4a, 5b, 9c |
Taste different | 2a, 1b, 1c | 4a, 4b, 0c | 6a, 5b, 1c |
Total pairs | 3a, 1b, 7c | 7a, 9b, 3c | 10a, 10b, 10c |
Experimental
Materials
Lycopodium clavatum (common club moss) spores (27 μm type) were purchased from Tibrewala International (Kathmandu, Nepal). Chemicals used were purchased from Sigma-Aldrich, or Fisher. Sporopollenin exine capsules (SEC) were prepared according the procedure reported previously. Ibuprofen was purchased from Acros. SGF was freshly prepared containing NaCl (2 g l−1) and having a pH of 1.5 adjusted with HCl 2 M.51General experimental procedures
All NMR experiments were carried out at 20 °C on a Bruker Avance II 500 MHz spectrometer using a 4 mm magic angle spinning (MAS) probe operating at 125.7546 MHz for 13C measurements. Spectra were externally referenced to tetramethylsilane at 0 ppm. Experiments were carried out with 8 kHz sample spinning. Spectra were collected using cross polarization (CP) and TPPM decoupling during the acquisition period of 30 ms. All NMR data were processed using ‘Topspin’ Version 1.3 (Bruker Instruments, Karlsruhe, Germany). 100 mg of SEC or ibuprofen encapsulated into SEC or bulk ibuprofen was packed into 4 mm MAS rotors, accordingly. Scanning electron micrographs were obtained using a Leica Cambridge Stereoscan 360 Scanning Electron Microscope. Samples were mounted on a metal stub with an adhesive and coated under vacuum with carbon before being coated with 200 Å gold film. API-QSTAR XL QqTOF-MS/MS hybrid tandem mass spectrometer used an Applied Biosystems International-MDS Sciex, Foster City, CA, USA equipped with a nano-electrospray source (Protana XYZ manipulator) which produces the electrospray through a PicoTip needle (10 mm i.d., New Objectives, Wobum, MA, USA) carrying a voltage of 2400 V. The samples were introduced by direct injection in an acetonitrile
:
water (3
:
1) solvent. The mass spectra were acquired from m/z 100 to m/z 2000. MALDI-TOF-MS analysis was carried out on a 4700 Proteomics analyzer with TOF-TOF optics (Applied Biosystems Foster City, CA, USA) and a 200 Hz 10 frequency-tripled Nd:YAG laser.α-cyano-4-hydroxycinnamic acid (α-CHCA) was used as matrix for the analysis of the so-called protein extract with an average of 5000 to 8000 laser shots per spectra. X-ray diffraction measurements were made using a PANalytical CubiX PRO diffractometer (Amelo, Netherlands) at a wavelength of 1.5418 Å generated by a copper long-fine focus tube operated at 45 kV and 40 mA. Samples were mounted on a silicon wafer mount and analysed in reflection geometry in θ–2θ configuration over the scan range 2° to 40° 2θ with 1.905 seconds exposure per 0.0025067° increment.Encapsulation of ibuprofen into SEC
A solution of ibuprofen (1.03 g) in ethanol (1 cm3) was added to SEC (1.10 g) with gentle stirring and vortexed for 1 min. to obtain a homogeneous mixture, which was subjected to a vacuum (ca. 10 hPa) for 1.5 h. The sample was freeze dried to a constant weight.Loading efficiency and in vitro release into simulated gastric fluid and phosphate buffer
Ibuprofen was assayed in accordance with a published method using UV-vis spectroscopy.52 Calibration were made using standard solutions of ibuprofen in ethanol over the concentration range 16.5–404.0 μg cm−3 by plotting absorbance of ibuprofen (A) obtained by UV-vis spectroscopy at 238 nm versus ibuprofen concentration [c]. The ibuprofen samples were prepared in six different concentrations. Linear regression analysis of A versus [c] was attained using Microsoft Excel® and gave the equation A = 0.0032[c] + 0.0168. The loading efficiency was determined by stirring the ibuprofen loaded SEC (20 mg) in ethanol (10 cm3) for 10 min after which the SEC were removed by filtration (porosity grade 3). This operation was repeated twice, the filtrate was diluted up to 50 cm3 and the loading efficiency of ibuprofen calculated to be 97 ± 1%.For drug release studies, the drug-loaded SEC (1
:
1 w/w) (0.02 g) were stirred in simulated gastric fluid (10 cm3) pH 1.5 and phosphate buffer saline (10 cm3) pH 7.4, respectively. After the prescribed period Table 2; Fig. 4, the suspension was filtered. The remaining ibuprofen was extracted from the exines into ethanol (10 mL) and filtered. Each operation was repeated twice and the filtrate was diluted up to 50 cm3 and the amount of ibuprofen remaining determined by UV-vis spectroscopy. Percentage data are based on 100% being the initial loading of ibuprofen.
Taste trial
Ten volunteers participated in the taste trial. The tasters had not been trained and were blinded during the test. The samples consisted of SEC loaded with ibuprofen (1
:
1 w/w), empty SEC and bulk ibuprofen alone. The volunteers were informed that the samples possibly contained ibuprofen, ibuprofen and SEC or simply SEC. Each one of the ten volunteers tasted, in a random order, ca. 20 mg of 6 lots of paired samples, using a clean plastic straw to deliver the sample directly to the tongue. Samples were given as follows: 2 samples of each of bulk ibuprofen, empty SEC and ibuprofen loaded SEC for control. Subsequently, each volunteer tasted a sample of bulk ibuprofen and a sample of empty SEC; a sample of ibuprofen and ibuprofen loaded SEC; a sample of empty SEC and a sample of ibuprofen loaded SEC. Between each sample tasting, the volunteers rinsed their mouths thoroughly with water. Samples were assessed throughout the process, with volunteers attempting to identify if the samples were the same or different. Pairs of samples were marked with a one if the volunteers thought that they were the same and marked with a zero if they thought that the paired samples were different. Thus there were three experiments carried out at two time-points by each of 10 observers as described previously. There were no missing data. The results of the first set of experiments are presented as frequencies only. By combining data from both time points we calculated between observer reliability. Thus, three separate 2 × 2 tables, were generated representing matched pairs, combined as Table 2.53 Discordant (for taste) pairs are shown in Table 2 on the top right hand cell and bottom left hand cell respectively, concordant (for taste) otherwise. Although this was not a powered study p-values were calculated using McNemar's test.47Analysis of SEC extracts using electrophoresis and mass spectrometry
Detergent-based lysis protein extraction of SEC. SEC (1 g) were extracted using ice-cold buffer containing 50 mM sodium acetate (pH 5.2), (100 cm3) 1 mM ascorbic acid, 0.5 mM phenylmethylsulfonyl fluoride and 0.5% polyvinyl polypyrrolidone. Subsequent to centrifugation, the supernatant was precipitated with 30% ammonium sulphate, at 4 °C, overnight. The pellet was discarded and the supernatant was desalted using a Sephadex G-50 column and the eluent (acetonitrile–water) was evaporated: the residue was dissolved in water, lyophilized and used for protein identification.40 Sonication lysis protein extraction of sporopollenin. The protocol followed was in accordance with that report by Bruggeman and Westerhoff41 SEC (100 mg) were pulverized in liquid nitrogen using a mortar and pestle, sonicated in trichloroacetic acid
:
acetone (1
:
9 w/v) solution in a sonic water bath. The SEC were washed successively with methanol (3 × 100 cm3), acetone (3 × 100 cm3), and phenol (3 × 50 cm3). The addition of excess ammonium acetate–methanol failed to give precipitate. Electrophoretic separation of proteins. Attempts to detect proteins in SEC extracts were made using sodium dodecyl sulphate-polyacrylamide gel electrophoresis (SDS-PAGE) at different acrylamide/bisacrylamide concentrations (12% resolving/4% stacking gels) using a vertical slab gel electrophoresis apparatus. Each lane received 50 μL of the SEC extract. Electrophoresis was conducted at 50–100 V. No proteins were detected in the gels by staining with coomassie brilliant blue R250 for 2 to 4 hours.
ESI-QqTOF-MS and CID-MS/MS analysis. A solution of acetonitrile
:
water (7
:
3) and SEC extracts was injected directly into the ESI source of an ESI-QqTOF-MS recorded in the positive ion mode. Neither single fragment ions nor multi-charged ions were noted in the ESI-MS, from any of the six repeated extraction preparations; hence it was concluded that none of the SEC extracts contained any protein or peptide. MALDI-TOF-MS analysis. MALDI-TOF-MS analysis of the SEC extracts used a YAG laser.α-cyano-4-hydroxycinnamic acid (α-CHCA) as matrix with an average of 5000 to 8000 laser shots per spectra. 1 mL of a 20 mg mL−1 solution of α-CHCA (dissolved in acetone) and 0.1% trifluoroacetic acid (TFA) was spotted on the MALDI plate and dried at room temperature (the use of acetone allowed good homogeneity of the matrix). Then, an aliquot of 1 mL of each SEC extract was spotted on the top of the dried matrix and left to dry before the MALDI-MS experiments. The analysis was achieved in the linear mode and the MALDI-TOF mass spectrometer was calibrated using BSA. Neither peptide nor protein was detected in any of the repeat extracted SEC extractions.
Toxicity testing using human umbilical vein endothelial cells (HUVEC) and an endothelial cell line EAhy 926
Effects of SEC on HUVEC proliferation. HUVECs (25
000 per well 12 well plate) (2500 per well 96 well plate) were allowed to adhere to cell culture plates for two hours before SEC (1 ng mL−1 to 10 μg mL−1) (diluted in culture media) were added. After two days the effects of the SEC were assessed microscopically (12 well plate) and for proliferation by WST-1 (96 well plate). To avoid any problems with the SEC affecting the absorbance readings which are an integral part of the WST-1, the plate for the WST-1 assay was washed with PBS and fresh growth media was added to remove the SEC prior to starting the 3 h. Photomicrographs of HUVE cells treated with the SEC showed no sign of any cellular damage at the concentrations 100 ng to 10 μg. In addition the SEC did not reduce proliferation of the HUVEC when compared to the untreated control. Effects of SEC on EAhy 926 cells. EAhy 926 cells (25
000 per well 12 well plate) (2500 per well 96 well plate) were allowed to adhere to cell culture plates overnight before spores (1 ng mL−1 to 10 μg mL−1) (diluted in media) were added. After two days the effects of the SEC were assessed microscopically (12 well plate) and for proliferation by WST-1 (96 well plate). A further two days later cell death in the samples was assessed using the cell death ELISA for apoptosis and LDH for necrosis assays. To avoid any problems with the SEC affecting the absorbance readings which are an integral part of the WST-1, the plate for the WST-1 assay was washed with PBS and fresh growth media was added to remove the SEC prior to starting the 3 h. It was found that SEC at concentrations 100 ng to 10 μg did not reduce proliferation of EAhy 926 cells when compared to the untreated control and did not increase the amount of cell death. Conclusion
Ibuprofen can be encapsulated into SEC efficiently (97 ± 1%) by a simple and rapid method to generate uniform microcapsules with a loading of 1
:
1 w/w, which have a surface free of the drug as detected by SEM. Solid state NMR, FTIR and XRD measurements are consistent with ibuprofen being encapsulated as in its bulk crystalline form. Release profiles of encapsulated ibuprofen into simulated gastric and intestinal fluids respectively, indicate that an encapsulated material can be released rapidly in high yield from SEC triggered by pH. This observation is perhaps a model for other actives or excipients which might be released in a similar manner. Importantly, exhaustive analysis of extracts of the SEC revealed no protein content by mass spectrometric methods approved by food advisory bodies. In addition, SEC were shown not to reduce the proliferation or induce cell death in human endothelial cells in vitro at concentrations between 100 ng and 10 μg. The SEC were shown to mask the taste of the bitter tasting ibuprofen when encapsulated suggesting a low drug availability on the surface of the SEC and the potential for use of SEC in powdered drug formulations for oral dosing. The evidence presented herein point to SEC having a potential for safe application to such as drug delivery and other areas such as food, cosmetics and beverages.Acknowledgements
We thank Anthony D. Sinclair for his technical assistance and AstraZeneca for the provision of XRD data. We are grateful to Sporomex Ltd and the University of Hull for providing funding for the work.References
- N. A. Wagdare, A. T. M. Marcelis, R. M. Boom and C. J. M. van Rijn, Colloids Surf., B, 2011, 88, 175–180 CrossRef CAS.
- T. Liu and Z. Zhang, Biotechnol. Bioeng., 2004, 85, 770–774 CrossRef CAS.
- J. H. Banoub, Science Branch, Fisheries and Oceans Canada, 2012 Search PubMed.
- H. F. Linskens and W. Jorde, Econ. Bot., 1997, 51, 78–86 CrossRef.
- W. A. M. Wolken, J. Tramper and M. J. van der Werf, Trends Biotechnol., 2003, 21, 338–345 CrossRef CAS.
- J. Elist, Urology, 2006, 67, 60–63 CrossRef.
- K. Winther, E. Rein and C. Hedman, Climacteric, 2005, 8, 162–170 CrossRef CAS.
- G. Shaw, in Sporopollenin, ed. J. Brooks, P. R. Grant, M. Muir, P. Van Gijzel and G. Shaw, Academic Press, London & New York, 1971, pp. 305–348 Search PubMed.
- J. Brooks and G. Shaw, Chem. Geol., 1972, 10, 69 CrossRef CAS.
- A. Diego-Taboada, P. Cousson, E. Raynaud, Y. Huang, M. Lorch, B. P. Binks, Y. Queneau, A. N. Boa, S. L. Atkin, S. T. Beckett and G. Mackenzie, J. Mater. Chem., 2012, 22, 9767–9773 RSC.
- G. Erdtman, Sven. Bot. Tidskr., 1960, 54, 561–564 Search PubMed.
- S. L. Atkin, S. T. Beckett and G. Mackenzie, Patent GB2453931A, 2007.
- G. Shaw, Phytochemical Phylogeny. Symposium, ed. J. B. Harborne, 335p. Illus., Academic Press, New York, N.Y., U.S.A.; London, England, 1970, pp. 31–58 Search PubMed.
- E. Dominguez, J. A. Mercado, M. A. Quesada and A. Heredia, Sex. Plant Reprod., 1999, 12, 171–178 CrossRef CAS.
- J. S. Watson, W. T. Fraser and M. A. Sephton, J. Anal. Appl. Pyrolysis, 2012, 95, 138–144 CrossRef CAS.
- A. R. Hemsley, A. C. Scott, P. J. Barrie and W. G. Chaloner, Ann. Bot., 1996, 78, 83–94 CrossRef.
- R. Adamson, S. Gregson and G. Shaw, Int. J. Pept. Protein Res., 1983, 22, 560–564 CrossRef CAS.
- G. Mackenzie and G. Shaw, Int. J. Pept. Protein Res., 1980, 15, 298–300 CrossRef CAS.
- G. Shaw, M. Sykes, R. W. Humble, G. Mackenzie, D. Marsden and E. Pehlivan, React. Polym., Ion Exch., Sorbents, 1988, 9, 211–217 CrossRef CAS.
- V. N. Paunov, G. Mackenzie and S. D. Stoyanov, J. Mater. Chem., 2007, 17, 609–612 RSC.
- S. Barrier, A. Diego-Taboada, M. J. Thomasson, L. Madden, J. C. Pointon, J. D. Wadhawan, S. T. Beckett, S. L. Atkin and G. Mackenzie, J. Mater. Chem., 2011, 21, 975–981 RSC.
- J. R. Rowley and J. J. Skvarla, Grana, 2000, 39, 1–7 CrossRef.
- J. R. Rowley, J. J. Skvarla and G. El-Ghazaly, Can. J. Bot., 2003, 81, 1070–1082 CrossRef.
- K. Holt, G. Allen, R. Hodgson, S. Marsland and J. Flenley, Rev. Palaeobot. Palyno., 2011, 167, 175–183 CrossRef.
- A. Wakil, G. Mackenzie, A. Diego-Taboada, J. G. Bell and S. L. Atkin, Lipids, 2010, 45, 645–649 CrossRef CAS.
- S. T. Beckett, S. L. Atkin and G. Mackenzie, Patent, Dosage Form WO2005000280A3, 2005.
- S. R. Hall, H. Bolger and S. Mann, Chem. Commun., 2003, 2784–2785 RSC.
- A. R. Hemsley, B. Vincent, M. Collinson and P. C. Griffiths, Ann. Bot., 1998, 82, 105–109 CrossRef.
- S. Barrier, A. S. Rigby, A. Diego-Taboada, M. J. Thomasson, G. Mackenzie and S. L. Atkin, LWT–Food Sci. Technol., 2010, 43, 73–76 CrossRef CAS.
- M. Lorch, M. J. Thomasson, A. Diego-Taboada, S. Barrier, S. L. Atkin, G. Mackenzie and S. J. Archibald, Chem. Commun., 2009, 6442–6444 RSC.
- M. A. Abd El-Ghaffar, M. S. Hashem, M. K. El-Awady and A. M. Rabie, Carbohydr. Polym., 2012, 89, 667–675 CrossRef CAS.
- M. M. Baracat, A. M. Nakagawa, R. Casagrande, S. R. Georgetti, W. A. Verri, Jr and O. de Freitas, AAPS PharmSciTech, 2012, 13, 364–372 CrossRef CAS.
- P. S. Lee, S. G. Yim, Y. Choi, H. Thi Van Anh and S. Ko, Food Chem., 2012, 134, 992–998 CrossRef CAS.
- R. Wiermann and S. Gubatz, Int. Rev. Cytol., 1992, 140, 35–72 CrossRef CAS.
- S. Herminghaus, S. Gubatz, S. Arendt and R. Wiermann, Z. Naturforsch., C: J. Biosci., 1988, 43, 491–500 CAS.
- C. Dubernet, J. P. Benoit, N. A. Peppas and F. Puisieux, J. Microencapsulation, 1990, 7, 555–565 CrossRef CAS.
- F. Zetsche and H. Vicari, Helv. Chim. Acta, 1931, 14, 62–67 CrossRef.
- S. Barrier, A. Loebbert, A. J. Boasman, A. N. Boa, M. Lorch, S. L. Atkin and G. Mackenzie, Green Chem., 2010, 12, 234–240 RSC.
- Advisory Committee on Novel Foods and Processes July 2011, http://www.food.gov.uk/multimedia/pdfs/proteinsinnovelfoodsissuesforconsid.pdf.
- M. Ghosh, D. Thangamani, M. Thapliyal, R. Yasodha and K. Gurumurthi, Acta Bot. Croat., 2004, 63, 75–81 Search PubMed.
- F. J. Bruggeman and H. V. Westerhoff, Trends Microbiol., 2007, 15, 45–50 CrossRef CAS.
- P. Stloukal, P. Kucharczyk, V. Sedlarik, P. Bazant and M. Koutny, J. Agric. Food Chem., 2012, 60, 4111–4119 CrossRef CAS.
- P. S. Kumar, S. Ramakrishna, T. R. Saini and P. V. Diwan, Pharmazie, 2006, 61, 613–617 CAS.
- A. Hillerstrom, J. van Stam and M. Andersson, Green Chem., 2009, 11, 662–667 RSC.
- A. Maack, in Polymerix, Rennes, France, 2003 Search PubMed.
- B. Ekwall, V. Silana, A. Paganuzzi-Stammati and F. Zucco, Toxicity Tests with Mammalian Cell Cultures, ed. P. Bourdeau, Published by John Wiley & Sons Ltd, 1990, pp. 75–97 Search PubMed.
- Q. McNemar, Psychometrika, 1947, 12, 153–157 CrossRef CAS.
- C. P. des Gachons, G. K. Beauchamp and P. A. S. Breslin, in International Symposium on Olfaction and Taste, ed. T. E. Finger, 2009, pp. 140–144 Search PubMed.
- Y. Gao, F. D. Cui, Y. Guan, L. Yang, Y. S. Wang and L. N. Zhang, Int. J. Pharm., 2006, 318, 62–69 CrossRef CAS.
- J. Xu, L. L. Bovet and K. Zhao, Int. J. Pharm., 2008, 359, 63–69 CrossRef CAS.
- N. D. Polovic, T. C. Velickovic, M. Gacrovic-Jankulovic, L. Burazer, D. Dergovic-Petrovic, O. Vuckonic and R. M. Jantkov, J. Serb. Chem. Soc., 2004, 69, 533–540 CrossRef CAS.
- M. R. Khoshayand, H. Abdollahi, M. Shariatpanahi, A. Saadatfard and A. Mohammadi, Spectrochim. Acta, Part A, 2008, 70, 491–499 CrossRef CAS.
- A. S. Rigby and M. B. Robinson, Disabil. Rehabil., 2000, 22, 259–265 CrossRef CAS.
|
This journal is © The Royal Society of Chemistry 2013 |