DOI:
10.1039/C3OB26537D
(Emerging Area)
Org. Biomol. Chem., 2013,
11, 2740-2755
New trends in bismuth-catalyzed synthetic transformations
Received 3rd August 2012, Accepted 14th January 2013
First published on 14th January 2013
Abstract
This review covers uses of bismuth catalysts since 2005 with a special emphasis on the emerging applications of such catalysts. Low toxicity, low catalytic loading, synergistic effects with other catalysts, and some hydrocompatibility properties confer to bismuth salts major advantages. The expanding activity in the field clearly highlights the growing potential of bismuth catalysts. The article is not a comprehensive review on bismuth catalysis but a selection of its most promising uses in challenging synthetic transformations.
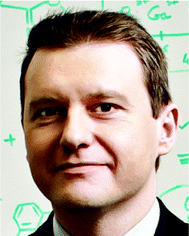 Thierry Ollevier | Born in Brussels, Thierry Ollevier obtained his B.Sc. (1991) and Ph.D. (1997) at the Université de Namur, Belgium, and was a postdoctorate fellow at the Université catholique de Louvain, Belgium under Istvan E. Markó (1997), a NATO postdoctorate fellow at Stanford University under Barry M. Trost (1998–2000), then a postdoctorate fellow at the Université de Montréal under André B. Charette (2000–2001). After an Assistant Professor appointment (2001) at Université Laval, he became an Associate (2006) and is currently a Full Professor. Current research in his group aims at designing novel catalysts, developing catalytic reactions and applying these methods to chemical synthesis. He is active in the areas of Lewis acids, asymmetric catalysis, organic chemistry under aqueous conditions, and synthetic green chemistry. |
1. Introduction
Bismuth is known as an environmentally benign element and has been used in a growing number of applications over the last few years. The role of bismuth(III) salts as Lewis acids has only been studied since the late 1980s. Pioneering work by Dubac, Wada and others paved the way to wide and general methods using bismuth(III) catalysts.1 The versatile use of bismuth salts in synthesis has clearly been highlighted by the increasing number of publications in the field. Low toxicity of bismuth salts, associated with low cost, make them attractive and practical catalysts. During the last decade, the chemical community finally began considering the previously under-used chemistry of organobismuth derivatives and bismuth catalysts. Now, many academic groups around the world are entering the area. As a result of increasing concern about green catalysts, bismuth catalysts have become a main focus.Various books and reviews have been published in the field of bismuth catalysis.1,2 The present review covers uses of bismuth catalysts since 2005 with a special emphasis on the emerging applications in terms of efficiency and novelty.
2. Aldol reactions
Since the original work by Dubac in the bismuth-catalyzed Mukaiyama aldol reaction,1,2 considerable progress has been made in addressing a variety of more complex systems.2.1. Vinylogous Mukaiyama aldol reaction
An efficient vinylogous Mukaiyama aldol reaction of 2-(trimethylsilyloxy)furan with various aromatic aldehydes mediated by Bi(OTf)3 in a low catalyst loading (1 mol%) was developed by our group (Scheme 1).3 The reaction proceeds rapidly and affords the corresponding 5-(hydroxy(aryl)methyl)furan-2(5H)-ones in high yields with good to very good diastereoselectivities (dr up to >98
:
2). Such selectivities, albeit previously reported with other Lewis acids, could this time be achieved with a much lower catalyst loading. 5-(Hydroxy(alkyl)methyl)furan-2(5H)-ones derived from ketones could also be obtained with good diastereoselectivities. |
| Scheme 1 | |
2.2. Asymmetric Mukaiyama aldol reaction
The development of organic reactions in aqueous media is essential because water is a key solvent for environmentally benign chemical synthesis. Catalytic asymmetric hydroxymethylation of silyl enolates with aqueous formaldehyde has been achieved using a chiral bismuth complex.4 Bismuth triflate was conjointly used with Bolm's ligand in dimethoxyethane–water solvent mixtures (Scheme 2). High enantioselectivities and high yields have been obtained. Kobayashi's work provided a new entry to “water-compatible Lewis acids”.5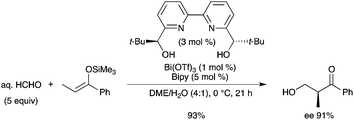 |
| Scheme 2 | |
2.3. Crossed condensation of ketones and aldehydes
A cationic organobismuth perfluorooctanesulfonate (cat. A, Scheme 3) showed high catalytic activity in the one-pot synthesis of (E)-α,β-unsaturated ketones through highly selective crossed condensation of ketones and aldehydes in water (Scheme 3).6 The catalyst is air-stable and exhibits both acidic and basic characteristics.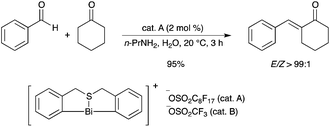 |
| Scheme 3 | |
2.4. Mukaiyama–Michael addition reaction
New spiro-cyclopropanated 1-aminopyrrol-2-ones were regioselectively prepared in high yields by Bi(OTf)3-catalyzed one-pot Mukaiyama–Michael addition/cyclization/ring-contraction reactions of 1,2-bis(trimethylsilyloxy)cyclobutene with 1,2-diaza-1,3-butadienes (Scheme 4).7 The reaction conditions are mild and the products were afforded with excellent yields.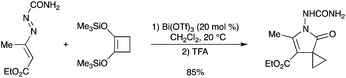 |
| Scheme 4 | |
3. Epoxide opening reactions
3.1. Organobismuth-catalyzed epoxide opening reaction
A related organobismuth triflate (cat. B, Scheme 3) was used as an air-stable catalyst for the epoxide opening reaction.8 It was found to exhibit high catalytic activity towards the ring opening reaction of epoxides in aqueous media with aromatic amines at room temperature (Scheme 5). The catalyst showed good stability, recyclability and reusability. The catalytic system afforded a simple and efficient method for the synthesis of β-amino alcohols. |
| Scheme 5 | |
These air-stable hypervalent organobismuth(III) salts have also been used as catalysts for other reactions, such as the allylation reaction of aldehydes using tetrallyltin and the Mannich reaction in water.9
3.2. Asymmetric epoxide opening reaction
The use of Bi(OTf)3 with Bolm's ligand has been successfully applied by Kobayashi in an asymmetric epoxide opening reaction with anilines in water.10 The reaction of aniline with cis-stilbene oxide occurred in pure water with sodium dodecylbenzene sulfonate (SDBS) as a surfactant and afforded the corresponding enantioenriched β-amino alcohol with a good enantioselectivity and a good yield (Scheme 6). Such applications are particularly important, as Bi(OTf)3 itself is unstable in the presence of water,11 but is stabilized by the basic ligands in such a way that hydrolysis is prevented.5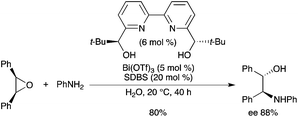 |
| Scheme 6 | |
4. Hydroamination and amination reactions
4.1. Intermolecular hydroamination reactions
A Bi(OTf)3–Cu(CH3CN)4PF6 system efficiently promoted intermolecular 1
:
1 hydroamination of 1,3-dienes with various carbamates, sulfonamides, and carboxamides to afford allylic amines in good yields.12 The reaction proceeded with 0.5–10 mol% catalyst loading in 1,4-dioxane (Scheme 7). This Bi(OTf)3–Cu(CH3CN)4PF6 system using a main group metal constitutes a new entry into a series of hydroamination catalyses. The hydroamination reaction gave unsatisfactory results with either Bi(OTf)3 or Cu(CH3CN)4PF6 alone. The combination of Bi(OTf)3 with MPF6 (M = [Cu(CH3CN)4] or K) was essential to achieve high yields. The authors also suggested that PF6−, rather than Cu, plays an important role. Mechanistic studies suggested that the active species is cationic Bi(OTf)2·PF6 generated by cation exchange, which acts as a π acid to activate 1,3-dienes to generate carbenium intermediates. The ability of Bi to interact with the carbonyl group of benzamide, chosen as the nucleophile, was confirmed by IR and NMR analysis.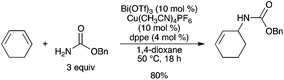 |
| Scheme 7 | |
The Bi(OTf)3–Cu(CH3CN)4PF6 system was further applied to hydroaminations of styrene with sulfonamides.13 With p-TsNH2, the reaction proceeded smoothly at 25 °C and the corresponding product was obtained with a good yield (Scheme 8). However, due to limitations with the scope of the reaction, other metal triflates were also investigated. A new Hf(OTf)4–Cu(CH3CN)4PF6 system was found to be very efficient to promote the hydroamination of various vinyl arenes with electron-withdrawing groups. This method was successfully applied to sulfonamides, carbamates, and carboxamides.
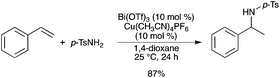 |
| Scheme 8 | |
The BiCl3-catalyzed intermolecular hydroamination of norbornene with various aniline derivatives was studied.14 Hydroamination products were obtained as the major products (Scheme 9). However, in some cases, hydroarylation occurred as a side reaction.
 |
| Scheme 9 | |
4.2. Intramolecular hydroamination reactions
4.2.1. Hydroamination reaction of alkenyl sulfonamides. A catalytic intramolecular hydroamination of unactivated alkenyl sulfonamides was developed using Bi(OTf)3.15 The reaction proceeds under simple conditions and provides an easy access to 2-methylpyrrolidines in high yields (Scheme 10). Control experiments supported the hypothesis that the actual hydroamination catalyst might be HOTf generated from Bi(OTf)3.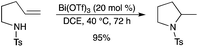 |
| Scheme 10 | |
Bi(OTf)3 was proven to be an effective catalyst for tandem hydroamination of amino-alkene and amino-allene derivatives with benzyl alcohols or vinyl ketones, affording functionalized N-heterocycles in good yields under mild conditions (Scheme 11).16 This method could be further applied to other types of amino-olefins, such as one-carbon-elongated amino-alkenes and trisubstituted ones.
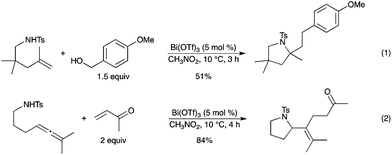 |
| Scheme 11 | |
4.2.2. Heck hydroamination cascade reaction. The spiro(pyrrolidine-3,3′-oxindole) skeleton, found in hemiterpene spirooxindole alkaloids, was efficiently constructed by using a tandem Pd0-catalyzed Heck cyclization reaction and BiIII-catalyzed hydroamination (Scheme 12).17 The spiro derivative could also be prepared from the same carbamoyl chloride in better yield and with better diastereoselectivity by a stepwise Pd0-catalyzed Heck reaction and Bi(OTf)3-catalyzed hydroamination (Bi(OTf)3 (10 mol%), KPF6 (10 mol%), 1,4-dioxane, 50 °C, 83%, dr 3
:
1).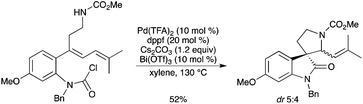 |
| Scheme 12 | |
4.2.3. Tandem hydroamination reaction of amino-1,6-enynes. Bi(OTf)3 efficiently catalyzed the cyclization of amino-1,6-enynes, leading to bicylic amines (Scheme 13).18 The reaction drastically depended on the stability of the cationic intermediate formed after the addition of the alkene on the alkyne moiety. |
| Scheme 13 | |
4.3. Hydroamidation reaction
A highly selective 6-exo-dig cyclization of alkynylamides was described using Bi(OTf)3.19 The synthesis of piperazin-2-ones was afforded by a 6-exo-dig intramolecular hydroamidation of 2-(prop-2-ynylamino)acetamides. The reaction with Bi(OTf)3 proceeded slowly at room temperature and afforded the 6-exo-cyclized product as a single product (Scheme 14). It is noteworthy that the use of Bi(OTf)3 resulted in the opposite regioselectivity to that of PtCl2 leading to the 7-endo-dig cyclization. The mechanism occurs through initial π-activation of the triple bond, followed by nucleophilic addition of the amide to the internal carbon of the triple bond. Subsequent protodemetalation followed by isomerization of the exocyclic double bond to the endocyclic position gave the observed product. The authors noted that in a few cases of the bismuth-catalyzed cyclization, the addition of five equivalents of water or catalytic amounts of HOTf gave better yields as well as complete conversions.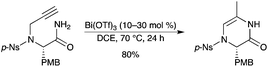 |
| Scheme 14 | |
4.4. Amination reactions
Bismuth catalysis is also suitable for the direct substitution of allylic, propargylic, and benzylic alcohols with sulfonamides, carbamates, and carboxamides under mild reaction conditions. A combination of Bi(OTf)3 and KPF6 (1–5 mol%) promoted the amination reactions at room temperature to give the products in up to 99% yield (Scheme 15).20 According to the authors, the possibility that HOTf, generated from Bi(OTf)3 and H2O, promotes the reaction cannot be ruled out completely, even in the presence of a dessicant. However, control experiments support the hypothesis that Bi(OTf)3/KPF6 functions as a combined catalyst.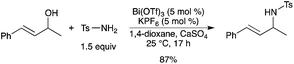 |
| Scheme 15 | |
Substitution of a propargylic alcohol with benzamide was promoted by BiCl3 as the catalyst (Scheme 16).21 Under mild conditions, the corresponding amide was obtained in good yield.
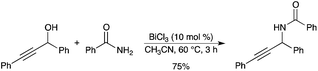 |
| Scheme 16 | |
The Bi(OTf)3–KPF6 catalytic system was successfully used in an SN′ substitution of tertiary allylic alcohols with benzyl carbamate.22 The corresponding allylic carbamate was obtained in good yield (Scheme 17). A related SN′ hydroamination has also been reported in an intramolecular case using Bi(OTf)3, in a moderate yield however.23
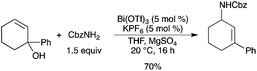 |
| Scheme 17 | |
4.5. Chirality transfer
The Bi(OTf)3-catalyzed intramolecular 1,3-chirality transfer reaction of chiral amino alcohols was developed to construct chiral 1-substituted tetrahydroquinolines (Scheme 18).24 The mechanism was believed to involve σ-activation of the hydroxyl group with the BiIII salt, followed by a concerted SN2′-type substitution.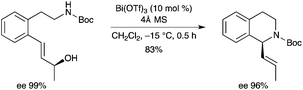 |
| Scheme 18 | |
4.6. Cascade reaction
The oxidative dearomatization of para-substituted o-alkynylanilines affords 2-alkynyl cyclohexadienimines, which can act as an active substrate with electron-rich styrenes upon catalysis with Bi(OTf)3 to give 3,4-dihydro-cyclopenta[c,d]indoles (Scheme 19).25 After the first oxidation step and a work-up process to remove methanol, the crude oxidative dearomatization mixture was used in the cascade reaction. The cascade reaction is metal-controlled; AgOTf provided tricyclic pyrrole derivatives instead of 3,4-dihydro-cyclopenta[c,d]indoles. |
| Scheme 19 | |
5. Alkylation reactions
5.1. Silyl-derived etherification reactions
Various one-pot methods for the conversion of aldehydes to homoallylic ethers and esters catalyzed by Bi(OTf)3 have been reported.26 A wide variety of homoallylic ethers including allyl, benzyl and methyl ethers can be synthesized using allyltrimethylsilane and alkoxytrimethylsilanes in the presence of Bi(OTf)3 (Scheme 20).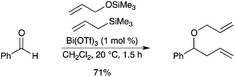 |
| Scheme 20 | |
Bismuth salts have been proven to be efficient catalysts for stereoselective etherification reactions.27 Evans reported that BiBr3 could catalyze the reaction of δ-trialkylsilyloxy aldehydes and ketones, using various trialkylsilyl nucleophiles for the construction of cis- and trans-2,6-di- and trisubstituted tetrahydropyrans (Scheme 21). The authors demonstrated that the bismuth salt was acting as a Brønsted acid source. Other carbon nucleophiles, such as a propargyl trimethylsilane or an enoxy trimethylsilane, could be used. A related strategy using BiBr3 and tert-butyldimethylsilane has been part of the total synthesis of (−)-mucocin.27 Evans’ protocol could be advantageously extended to a bromoenoxysilane in a polypropionate synthesis by Guindon.28
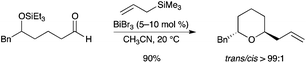 |
| Scheme 21 | |
A tandem intermolecular addition/intramolecular silyl-Prins (silyl-modified Sakurai reaction–ISMS) reaction efficiently afforded cis-2,6-disubstituted dihydropyrans using 5 mol% of BiBr3 in CH2Cl2 (Scheme 22).29 The reaction occurred between δ-triethylsilyloxyvinyltrimethylsilanes and a variety of aldehydes to give good to excellent yields of dihydropyran derivatives. The procedure is a mild alternative to the use of other Lewis acids such as BF3·OEt2 or TMSOTf and provides the dihydropyrans with an excellent diastereoselectivity.
 |
| Scheme 22 | |
Extension of this work to a cascade reaction involving epoxide rearrangement30 followed by a tandem addition/silyl-Prins reaction was reported by the same group.31 The cascade reaction sequence using isobutylene oxide afforded the pure cis isomer of the corresponding 2,6-disubstituted 3,6-dihydro-2H-pyran (Scheme 23). These studies indicate an initial Lewis acid/base interaction between Bi(OTf)3 and the substrates providing HOTf in situ.
 |
| Scheme 23 | |
A related strategy has been reported with an initial Mukaiyama aldol reaction followed by a second addition/silyl-Prins step (ISMS).32 BiBr3-mediated addition of silyl ketene acetals or silyl enol ethers to β,γ-unsaturated cis-4-trimethylsilyl-3-butenal provided an intermediate Mukaiyama aldol product containing a vinylsilane moiety tethered to a silyl ether (Scheme 24). The addition of a second aldehyde initiated a tandem sequence involving intermolecular addition followed by an ISMS reaction.
 |
| Scheme 24 | |
cis-Tetrahydropyrans have also been obtained in high yield via the intramolecular Sakurai allylation of a functionalized allylsilane with n-butyraldehyde in the presence of a stoichiometric amount of Bi(OTf)3 (Scheme 25).33
 |
| Scheme 25 | |
A diastereoselective synthesis of 4-amidotetrahydropyrans was achieved by a single-step Sakurai–Prins–Ritter reaction sequence in a tandem fashion by the reaction of an aldehyde and allytrimethylsilane in acetonitrile using Bi(OTf)3 as a catalyst (Scheme 26).34
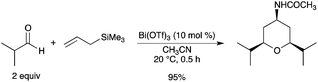 |
| Scheme 26 | |
A diastereoselective construction of syn-1,3-dioxanes was reported via a bismuth-mediated two-component hemiacetal/oxa-conjugate addition reaction.35 δ-Trialkylsilyloxy and δ-hydroxy α,β-unsaturated aldehydes and ketones react with alkyl aldehydes in a highly efficient and stereoselective manner (Scheme 27). Interestingly, the analogous process with nitric acid provided the 1,3-dioxane in significantly lower yield, illustrating the superiority of bismuth salts.
 |
| Scheme 27 | |
The same group reported a one-pot diastereoselective sequential two-component etherification, followed by an oxa-conjugate addition.36 The reaction of an anomeric acetal with a trimethylsilyloxy diene afforded a bis(tetrahydropyran) core used in the total synthesis of (+)-leucascandrolide A (Scheme 28). Bi(NO)3 was used as a Brønsted acid source. The necessity of a dual Lewis and Brønsted acid catalyzed process to affect this type of sequential process was demonstrated.
 |
| Scheme 28 | |
5.2. Hydroalkoxylation reactions
It was found that both terminal and internal olefins underwent a Bi(OTf)3-catalyzed hydroalkoxylation to afford tetrahydrofurans in high yield with preference for the anti diastereoisomer (Scheme 29, eqn (1)).37 A Bi(OTf)3-catalyzed nucleophilic addition/hydroalkoxylation has also been disclosed for the coupling of a variety of nucleophilic partners and aldehydes, in the presence of a small amount of MeOH (Scheme 29, eqn (2)). Triflic acid was demonstrated to be the actual catalyst of the hydroalkoxylation step.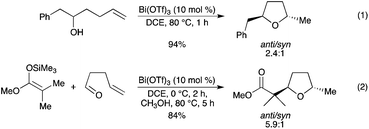 |
| Scheme 29 | |
5.3. Tandem cationic cyclization
A pyridone alkaloid, citridone A, has been prepared in a highly stereoselective way by using a tandem cationic cyclization and heterocyclization promoted by bismuth (Scheme 30).38 Bi(OTf)3 was the reagent of choice to avoid decomposition. The mechanism is believed to involve the formation of a bismuth amide complex, the ionization of the OTBS group, followed by internal etherification to quench an intermediately formed cation. Bi(OTf)3 was used in a stoichiometric quantity however.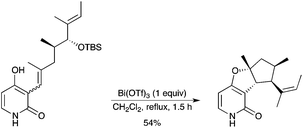 |
| Scheme 30 | |
5.4. Allylation reaction
A highly diastereoselective Bi(OTf)3-catalyzed reaction of chiral propargylic acetates with various weak carbon nucleophiles, i.e. silyl enol ethers, allyltrimethylsilane, and various arenes was disclosed.39 Among a wide array of potential catalysts (such as FeCl3, InCl3, AuCl3, Cu(OTf)2), Bi(OTf)3 proved to be the most effective. Propargylic acetates react with high diastereoselectivity under SN1 conditions to give the corresponding substitution products (Scheme 31).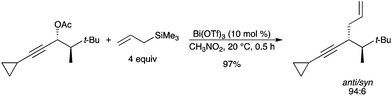 |
| Scheme 31 | |
5.5. Enantioselective allylation reaction
A highly enantioselective method for the catalytic addition of allyltributylstannane to aromatic aldehydes was developed by our group using Bi(OTf)3 and Trost's (R,R)-ProPhenol ligand.40 The allylation of a variety of aromatic aldehydes afforded the desired homoallylic alcohols in good yields with enantioselectivities ranging from 93
:
7 to 96
:
4 er (Scheme 32). The reaction also proved to proceed in good yield and high enantioselectivity using naphthyl carboxaldehydes or heteroaromatic aldehydes as electrophiles. The conditions have also been applied to aliphatic aldehydes. In preliminary experiments, enantioselectivities up to 96
:
4 have been obtained. Characterization data from 1H NMR spectroscopy and mass spectrometry have been provided as the first evidence of the pre-catalyst structure.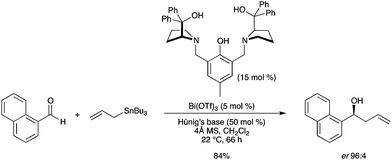 |
| Scheme 32 | |
5.6. Benzylation reactions
An efficient bismuth-catalyzed direct benzylation of 2,4-pentanediones was developed using various free benzyl alcohols and dicarbonyl compounds.41 The corresponding products of this direct C–C bond-forming reaction have been isolated in good to excellent yields. The procedure was extended to a direct allylic alkylation of 2,4-pentanediones affording the linear, unbranched products (Scheme 33). In this process, the only by-product was water. |
| Scheme 33 | |
The Bi(OTf)3-catalyzed benzylation was successfully applied to 4-hydroxycoumarin for the synthesis of numerous differently substituted warfarin derivatives (Scheme 34).42
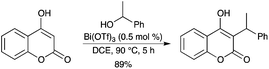 |
| Scheme 34 | |
A highly efficient Bi(OTf)3-catalyzed benzylation of arenes and heteroarenes has also been developed.43 Several electron-rich arenes, such as 1-methylnaphthalene, gave the corresponding diarylmethane products in good to excellent yields (Scheme 35).
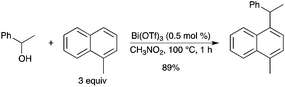 |
| Scheme 35 | |
The benzylation of several arenes was also performed by employing benzyl alcohol and benzyl acetate as the electrophiles (Scheme 36). Isolated yields of the products were slightly lower for the benzyl alcohol as compared to the benzyl acetate.
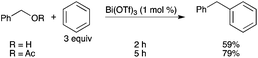 |
| Scheme 36 | |
A highly effective allylation reaction of substituted benzylic alcohols with allyltrimethylsilane has been developed using BiCl3 as the catalyst (Scheme 37).44 Bi(OTf)3 was not effective as a catalyst under these conditions.
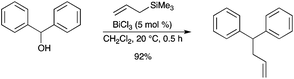 |
| Scheme 37 | |
The reaction of allyltrimethylsilane with various propargylic alcohols was also mediated by BiCl3 as the catalyst and the substituted 1,5-enynes were obtained in high yields (Scheme 38).21 BiCl3 was also an efficient catalyst for the propargylation of several electron-rich arenes and heteroarenes.21
 |
| Scheme 38 | |
High facial diastereoselectivities were observed with acetates derived from chiral α-branched para-methoxybenzylic alcohols.45 The reaction with the silyl enol ether derived from t-butyl methyl ketone afforded the corresponding ketone with a good yield and a high diastereoselectivity (Scheme 39).
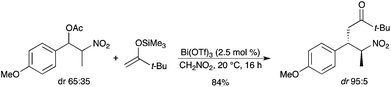 |
| Scheme 39 | |
5.7. N-Alkoxycarbonylamino sulfone allylation reactions
Bi(OTf)3 has been disclosed by our group to be an efficient catalyst in the Sakurai reaction of allyltrimethylsilanes with N-alkoxycarbonylamino sulfones.46 The reaction proceeded smoothly with a low catalyst loading of Bi(OTf)3 (2–5 mol%) to afford the corresponding protected homoallylic amines in very good yields (up to 96%) (Scheme 40, eqn (1)). The generality of our method was further extended to a functionalized allylsilane. Under the same conditions, the corresponding homoallylic amine was obtained and subsequently subjected to ring-closing metathesis using the second generation Grubbs’ catalyst (Scheme 40, eqn (2)).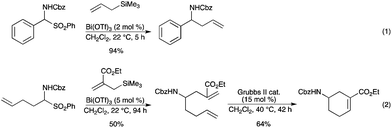 |
| Scheme 40 | |
5.8. Allenylation reaction
A convenient method for the synthesis of the allenyl adduct of a hydrazinoester has been reported using allenyl pinacol boronate and bismuth hydroxide as the catalyst.47 The allenyl vs. propargyl adduct was produced preferentially using Bi(OH)3 in a water–DMF solvent mixture (Scheme 41). |
| Scheme 41 | |
5.9. Prenylation reaction
Electron-rich aryl ethers and phenols react with isoprene in the presence of catalytic Bi(OTf)3 to afford the corresponding prenylated or 2,2-dimethylchroman products in moderate to good yields (Scheme 42).48 Prenylated aryl derivatives could also be obtained by Bi(OTf)3-catalyzed [1,3]-rearrangement of aryl 3-methyl-2-butenyl ethers.49 |
| Scheme 42 | |
6. Arylation and carboarylation reactions
In the presence of a catalytic amount of BiCl3, the reaction of acyl chlorides or vinyl chlorides with arenes afforded 1,1-diarylalkenes (Scheme 43).50 The procedure provides a one-pot method for the synthesis of 1,1-diarylalkenes. The reaction includes both the unusual formation of vinyl chlorides and the subsequent Friedel–Crafts-type vinylation of arenes catalyzed by BiCl3.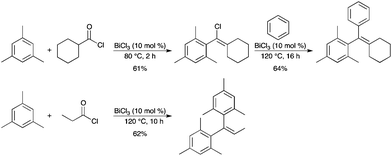 |
| Scheme 43 | |
A new catalytic combination of 5 mol% of Bi(OTf)3 with 3 equiv. of LiClO4 as a co-catalyst was discovered to drive a Friedel–Crafts cyclization of a free lactol, as part of a stereocontrolled synthesis of (−)-platensimycin (Scheme 44).51 The cyclized product was obtained in 94% yield within 3.5 h. The combination of Bi(OTf)3 with LiClO4 was believed to generate a more reactive cationic species with the lactol toward nucleophilic ring closure.
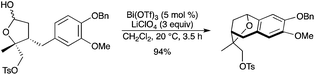 |
| Scheme 44 | |
A Bi(OTf)3-catalyzed Friedel–Crafts reaction of electron-rich aromatic compounds with 3-alkyl-3-hydroxy-2-oxindole has been developed (Scheme 45).52 It was found that In(OTf)3 and Cu(OTf)2 were equally efficient catalysts. Although, under similar conditions, 25–50 mol% of Ce(OTf)3 and Sn(OTf)2 provided high yields of the product, 10 mol% of these catalysts afforded decreased yields.
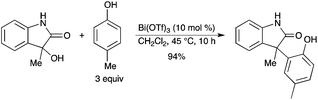 |
| Scheme 45 | |
Bi(OTf)3, as a borderline metal catalyst, was proven to work as a dual activator for alkynes and N,O-acetals via σ,π-chelation, which achieved a new carboarylation reaction of alkynylarenes with N,O-acetals (Scheme 46).53 This reaction tolerated a wide range of alkynylarenes, particularly possessing non-protected heteroatoms, and various N,O-acetals.
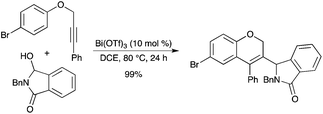 |
| Scheme 46 | |
7. Hydroarylation and hydroalkylation reactions
Further studies by Rueping showed that the above-mentioned benzylic alcohol derivatives (Schemes 35 and 36) could be replaced by a styrene derivative.54 A highly efficient Bi(OTf)3-catalyzed hydroarylation of various styrenes was then developed using arenes and heteroarenes (Scheme 47). The mild reaction conditions render this transformation an attractive approach to the valuable 1,1-diarylalkanes. |
| Scheme 47 | |
BiCl3 was also a catalyst for the hydroarylation of styrenes with electron-rich arenes and the Markovnikov adducts were selectively obtained in good to high yields.55 Under arene-free conditions, the intermolecular hydroarylation of α-substituted styrenes and subsequent intramolecular hydroarylation produced the cyclic dimers of α-substituted styrenes in good yields (Scheme 48).
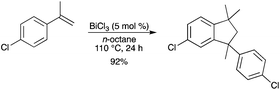 |
| Scheme 48 | |
Rueping's procedure was then extended to the inter- and intramolecular hydroalkylation of styrenes using 1,3-dicarbonyl compound as the nucleophile.56 An efficient Bi(OTf)3-catalyzed hydroalkylation of various styrenes, norbornene, and cyclohexadiene derivatives was developed with different 2,4-pentanediones (Scheme 49).
 |
| Scheme 49 | |
An efficient intramolecular hydroarylation of non-activated substituted olefins with non-activated arenes has recently been disclosed.57 The reaction could be carried out in the presence of 1–5 mol% Bi(OTf)3 and allowed the preparation of various tetralin derivatives in good to excellent yields (Scheme 50). Although Sc(OTf)3 and In(OTf)3 could also efficiently catalyze the hydroarylation reaction, Bi(OTf)3 was chosen because it is less toxic and shows high activity.
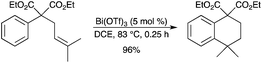 |
| Scheme 50 | |
Intramolecular hydroarylation of allenes was achieved under very mild conditions using Bi(OTf)3 as the catalyst (Scheme 51).58 Interestingly, triflic acid did catalyze this reaction with almost the same yield as Bi(OTf)3. The hydroarylation reaction is quite general and has been used for the synthesis of carbocycles and heterocycles without using noble metals and additional ligands.
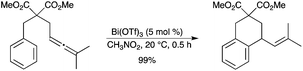 |
| Scheme 51 | |
8. Oxycarbonylation reactions
8.1. Hydro-oxycarbonylation reaction
Bi(OTf)3 was found to be a good catalyst for intramolecular addition of carboxylic acids to alkynes (hydro-oxycarbonylation), which afforded the corresponding 5- and 6-membered lactones in moderate to good yields under mild conditions (Scheme 52).59 BiIII, as a borderline-metal catalyst, was thought to act as a dual activator of both carboxylic and alkyne functions. Fe(OTf)3 and Bi(OTf)3 were found to be equally efficient to catalyze the transformation. The addition of the heteroatom seemed to mainly proceed at the alkynyl carbon attached to the aryl group.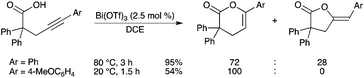 |
| Scheme 52 | |
8.2. Carbo-oxycarbonylation reaction
A Bi(OTf)3-catalyzed intramolecular carbo-oxycarbonylation of alkynyl benzylic esters has been developed by the same authors.60 This cyclization mainly afforded multisubstituted 6-membered lactones in good to high yields under mild conditions (Scheme 53). Evidence has been obtained to support an intramolecular mechanism.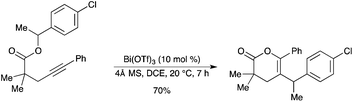 |
| Scheme 53 | |
9. Cycloisomerization reactions
9.1. Synthesis of pyrroles
Bi(OTf)3 was found to be a good catalyst for the synthesis of substituted pyrroles from the readily accessible 2-propynylamine and methylene active compounds.61 The reaction could be applied to various β-dicarbonyl compounds (Scheme 54). However, when applied to β-keto esters, it is noteworthy that the reaction afforded 3-acylpyrroles only. A mechanism involving the formation of 3-aza-1,5-enyne, followed by cycloisomerization of the enyne moiety, has been demonstrated by the authors.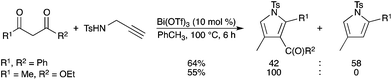 |
| Scheme 54 | |
9.2. Dehydrative alkynylcyclopropanation reaction
A BiIII-catalyzed dehydrative alkynylcyclopropanation of azaenynols to give 1-alkynyl-3-azabicyclo[3.1.0]hexanes has been described by the same authors (Scheme 55).62 The stereochemistry of the adducts strongly depended on that of the starting azaenynols. A dual coordination of the alkyne and the oxygen of the hydroxy group to the bismuth catalyst was believed to be essential.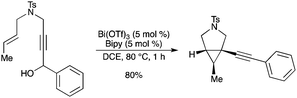 |
| Scheme 55 | |
9.3. Enyne cycloisomerization reaction
A straightforward method for the synthesis of multisubstituted fluorenes with (Z)-pent-2-en-4-yl acetates and ethynylarenes was developed using BiBr3 as a catalyst.63 The domino reaction involves intermolecular electrophilic addition and cycloisomerization aromatization (Scheme 56). Compared with BiBr3, other Bi salts as catalysts were less effective. |
| Scheme 56 | |
BiCl3 was found to be an efficient catalyst for the cycloisomerization of 1,6-enynes (Scheme 57).64 Electron-deficient alkynes appeared to be better substrates for the reaction.
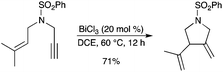 |
| Scheme 57 | |
9.4. Triene cycloisomerization reaction
Non-activated trienes were cyclized into polycyclic compounds in good to excellent yields under Bi(OTf)3 catalysis in a biomimetic fashion.65 The reaction showed broad generality and allowed for the formation of functionalized bicyclic to tetracyclic structures from simple precursors in a one-pot fashion (Scheme 58). A double cyclization occurred via the formation of cationic intermediates and involved a 1,2-methyl shift. It was proposed by the authors that the catalytic cycloisomerization process might proceed via a Lewis acid-assisted Brønsted acid-type activation. The cyclizations proceeded smoothly in 63–99% yields, leading to the formation of up to three cycles and three new C–C bonds in a single operation. Cycloisomerization of malonate-type highly substituted 1,6-dienes could also be catalyzed by Bi(OTf)3 albeit in low yield.66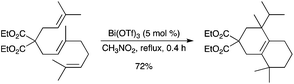 |
| Scheme 58 | |
9.5. Ketone cyclotrimerization reaction
Bi(OTf)3 was found to efficiently catalyze the cyclotrimerization of acetophenones into 1,3,5-triarylbenzenes in good yields (Scheme 59).67 This method was also used for the conversion of ortho-substituted acetophenones, which do not readily undergo cyclotrimerization by other methods.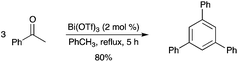 |
| Scheme 59 | |
10. Oxidations
An efficient method for allylic oxidation of unsaturated steroids has been disclosed by Salvador.68 Very good yields and high selectivity have been obtained, using t-BuOOH as the oxidant and various BiIII salts as the catalysts. 17-Oxoandrost-5-en-3β-yl acetate was smoothly converted into the corresponding enone (Scheme 60). Evidence was obtained that BiCl3 was converted into BiOCl during the reaction. Recovered BiOCl gave identical reaction rates and isolated product yields.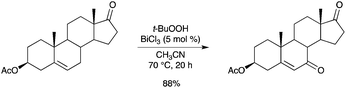 |
| Scheme 60 | |
In parallel, benzylic oxidation of methyl arenes with t-BuOOH in the presence of Bi(OTf)3 gave the corresponding substituted benzoic acids (Scheme 61).69 These results were consistent with the bismuth(III) acting as a Lewis acid and modifying the reactivity of the peroxide rather than acting in a BiIII–BiV cycle. Other metal triflates, such as Hf(OTf)4, Sc(OTf)3 and Yb(OTf)3, showed excellent catalytic activity as well for the oxidation of tetrahydronaphthalene into α-tetralone.
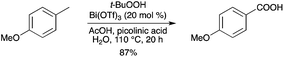 |
| Scheme 61 | |
Bi(OTf)3 was found to be an efficient catalyst for the oxidative rearrangement of tertiary allylic alcohols with the PhIO–TEMPO system (Scheme 62).70 The bismuth(III) salt is believed to isomerize the allylic alcohol via an allylic cation, prior to oxidation. Good yields of 3-substituted cyclic enones were generally obtained.
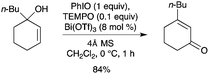 |
| Scheme 62 | |
A sequential one-pot deprotection–oxidation of primary and secondary tert-butyldimethylsilyl ethers was described using catalytic Bi(OTf)3 and TEMPO in the presence of PhI(OAc)2.71 Under such conditions, acid-sensitive substrates could be transformed into the corresponding aldehydes (Scheme 63).
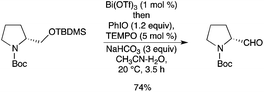 |
| Scheme 63 | |
In a palladium-catalyzed oxidative process, hydrosilane can serve as an activator of a palladium catalyst with bismuth(III) chloride, thus leading to a novel ligand- and silver-free palladium catalytic system for the facile oxidative esterification of a variety of benzylic alcohols in good yields (Scheme 64).72 Other bismuth salts, except BiI3, were also effective for the Pd-catalyzed oxidative esterification of o-tolylmethanol. This silver-free catalytic system has several advantages, i.e. being a ligand-free, environmentally benign system, and the possibility of directly using an air atmosphere as the terminal oxidant.
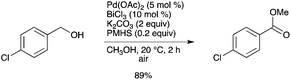 |
| Scheme 64 | |
11. Other reactions
11.1. Glycosylation reaction
Glycosylation of a sialyl donor with a thioethyl galactosyl acceptor has been performed in the presence of Bi(OTf)3 used in large excess vs. the sialyl donor (Scheme 65).73 In this case, the desired disaccharide was obtained with nearly complete α-stereoselectivity (α/β = 20
:
1). Activation with other Lewis acids led to decreased stereoselectivities.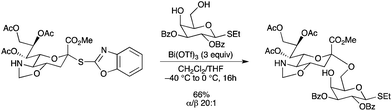 |
| Scheme 65 | |
11.2. Ritter reaction
N-tert-Alkyl and aryl amides have been obtained by a Ritter reaction of various nitriles with tertiary alcohols in the presence of a catalytic amount of Bi(OTf)3 (Scheme 66).74 Presumably under these aqueous conditions, catalysis is effected by triflic acid.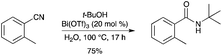 |
| Scheme 66 | |
11.3. Oxa- and thia-Pictet–Spengler reaction
Different isothiochroman or isochroman compounds have been synthesized from phenylethanethiol or phenylethanol and a series of benzaldehydes, in the presence of Bi(OTf)3 (Scheme 67).75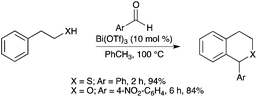 |
| Scheme 67 | |
11.4. [3 + 2] Cycloaddition reaction
In parallel, the use of a nitrile as a nucleophile was also involved in the [3 + 2] cycloaddition of a series of substituted N-tosylaziridines with nitriles promoted by Bi(OTf)3.76 Under these conditions, Bi(OTf)3 appeared to be the best promoter among various Lewis acids and various imidazolines were obtained under mild reaction conditions (Scheme 68). |
| Scheme 68 | |
11.5. Wagner–Meerwein rearrangement
The use of Bi(OTf)3 as a catalyst for the Wagner–Meerwein rearrangement of lupane derivatives with expansion of ring E and formation of an additional O-containing ring was reported.77 This process has also been extended to other terpenes, such as (−)-caryophyllene oxide (Scheme 69). Evidence for a Brønsted acid species being the actual catalyst was provided by the authors. In related studies, Bi(OTf)3 was also used as a catalyst for the direct conversion of corticosteroids into highly functionalized 17-ketosteroids by cleavage of the C17-dihydroxyacetone side chain.78 Participation of an in situ generated Brønsted acid species from Bi(OTf)3 was also pointed out for the opening of oleanolic hydroxy-γ-lactones into the corresponding 12-oxo-28-carboxylic acid derivatives.79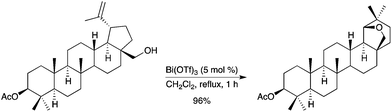 |
| Scheme 69 | |
11.6. Cationic cyclization via halide activation
Bi(OTf)3 was used to catalyze 5-exo-trig cyclization via halide activation.80 Employing 10 mol% Bi(OTf)3 with 4Å molecular sieves resulted in successful cyclization (Scheme 70). This study suggested that Bi(OTf)3 possesses interesting halophilic properties.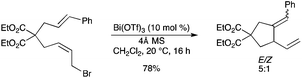 |
| Scheme 70 | |
11.7. Co-catalysis
Cooperative catalytic action of a chiral nucleophilic tertiary amine (the cinchona alkaloid derivative dihydroquinine 2,5-diphenyl-4,6-pyrimidinediyl diether [(DHQ)2PYR]) and Bi(OTf)3, in the presence of 1,2,2,6,6-pentamethylpiperidine (PMP), was proposed in the [2+2]-cycloaddition of sulfenes and aldehydes.81 The reaction of propanesulfonyl chloride with chloral provided the disubstituted β-sulfone with high diastereo- and enantioselectivity (Scheme 71). Bi(OTf)3 provided better yields (47–87%) than In(OTf)3 (28–69%), whereas in terms of enantioselectivity a clear-cut trend is not obvious.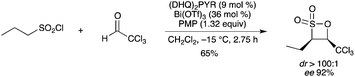 |
| Scheme 71 | |
Bismuth salts have been studied for their ability to activate chiral nickel catalysts in the chemo- and enantioselective hydrovinylation of styrene.82 BiBr3 was very efficient in the activation of the Ni complex, resulting in high conversion (Scheme 72). The Ni catalyst is activated by the BiIII salt, which can abstract the chloride ligand. Substantial increase of the catalytic activity of the nickel complex was also possible using InI3 or InBr3 as activators.
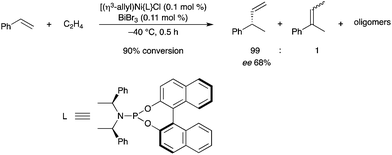 |
| Scheme 72 | |
A variety of 1,4-diazaspiro[2.2]pentanes were converted to the corresponding 1,3-diaminated ketone products via treatment with HOAc, followed by addition of 5 mol% of Bi(OTf)3 (Scheme 73).83 The authors obtained the evidence that the role of Bi(OTf)3 might be to simply generate small amounts of HOTf.
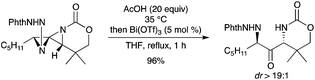 |
| Scheme 73 | |
An efficient one-pot tandem reaction of 2-alkynylbenzaldoximes with isocyanides co-catalyzed by AgOTf and Bi(OTf)3 has been developed, which affords N-(isoquinolin-1-yl)formamides in good to excellent yields (Scheme 74).84 AgOTf was effective for the generation of the intermediate isoquinoline N-oxides, Bi(OTf)3 being a catalyst for the further reaction with the isocyanide.
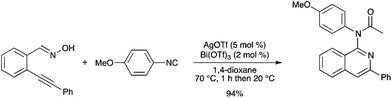 |
| Scheme 74 | |
11.8. Michael addition reaction
Bi(OTf)3-catalyzed Friedel–Crafts alkenylation, followed by air oxidation, allowed the preparation of 2-arylnaphthoquinones. Conjugate addition of dicyclohexylphosphine oxide to the obtained product was also catalyzed by Bi(OTf)3 (Scheme 75).85 Both arylation and phosphorylation reactions could be run in one-pot, however a better overall yield was obtained when isolated 2-arylnaphthoquinone was used. |
| Scheme 75 | |
11.9. Enantioselective cyclization
The synthesis of (2R)-2,5-diaryl-2,3-dihydropyarano[2,3-b]quinolin-4-ones was recently achieved by the reaction of 3-acetyl-4-aryl-carbostyril and an aldehyde, in the presence of Bi(OTf)3 and L-proline (Scheme 76).86 A Bi(OTf)3–L-proline complex is believed to be formed in situ.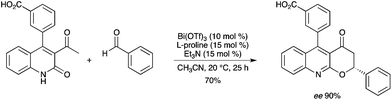 |
| Scheme 76 | |
11.10. Synthesis of furans
Bi(OTf)3 was found to be an effective catalyst for a tandem condensation/cyclization reaction of acyloins and β-diketones or β-keto esters to afford highly substituted furans in good to excellent yields (Scheme 77).87 |
| Scheme 77 | |
11.11. Synthesis of peroxides
Bi(OTf)3 was found to be an efficient and mild catalyst for the synthesis of both 1,1-dihydroperoxides, and symmetrical and unsymmetrical 1,2,4,5-tetraoxanes (Scheme 78).88 TfOH was not as efficient as Bi(OTf)3 as a catalyst for both reactions. |
| Scheme 78 | |
11.12. Preparation of functionalized organoaluminiums
BiCl3 was proved to be efficient for the generation of functionalized arylaluminium halides.89 BiCl3, used as an additive (3 mol%), allowed the direct insertion of aluminium powder in the presence of Me3SiCl (3 mol%) and LiCl (3 equiv.). Several functionalized unsaturated substrates readily underwent an Al insertion with BiCl3 as a catalyst, followed by transmetallation with a Zn salt and Pd-catalyzed cross-coupling, to generate the desired product (Scheme 79). However, the aluminium insertion in the presence of an ester or an amide functional group did not lead to satisfactory yields with BiCl3. Interestingly, Bi did not insert into the aryl iodide under the standard reaction conditions.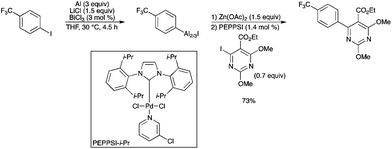 |
| Scheme 79 | |
12. Conclusions and future challenges
Catalytic transformation using bismuth is clearly an emerging area. The growing amount of activities in the field and the resulting constant need for knowledge developments make this review an essential update in organic synthesis using bismuth catalysis. Synergistic effects with other Lewis acids have also been recently highlighted. The discovery that some bismuth salts could be used as Lewis acids under aqueous conditions finally opened the door to designing catalysts and to broadening the concept of hydrocompatible Lewis acids, which has since been applied to various reaction types. Moreover, the use of bismuth catalysts has definitively contributed to the area of environmentally benign catalysts, known as green catalysts. These are fascinating developments since such green catalysts are now widely appreciated and new reactions and catalysts are being designed and published on a regular basis. The current developments allow us to demonstrate that bismuth chemistry truly is an emerging field. Efficient catalytic transformations using bismuth are definitively high potential processes, which encompass asymmetric catalysis using chiral bismuth(III) complexes as one of their most promising challenges to reach. The expanding activity in the field clearly highlights the growing potential of bismuth catalysts. Research in this area will contribute to meeting some of the remaining challenges of synthetic organic chemistry.Acknowledgements
The author wants to thank the Natural Sciences and Engineering Research Council of Canada (NSERC) and the Centre in Green Chemistry and Catalysis (CGCC) for financial support.Notes and references
- Bismuth-Mediated Organic Reactions, ed. T. Ollevier, Springer-Verlag, Berlin, Heidelberg, 2012 Search PubMed; H. Suzuki and Y. Matano, Organobismuth Chemistry, Elsevier, Amsterdam, 2001 Search PubMed.
- J. M. Bothwell, S. W. Krabbe and R. S. Mohan, Chem. Soc. Rev., 2011, 40, 4649 RSC; J. A. R. Salvador, S. M. Silvestre and R. M. A. Pinto, Molecules, 2011, 16, 2884 CrossRef CAS; R. Mohan, Nat. Chem., 2010, 2, 336 CrossRef; T. Ollevier, E. Nadeau and V. Desyroy, Bismuth(III) trifluoromethanesulfonate, in Electronic Encyclopedia of Reagents for Organic Synthesis, ed. P. L. Fuchs, John Wiley and Sons, Chichester, 2009 CrossRef; J. A. R. Salvador, R. M. A. Pinto and S. M. Silvestre, Mini-Rev. Org. Chem., 2009, 6, 241 CrossRef; R. Hua, Curr. Org. Synth., 2008, 5, 1 CrossRef; H. Gaspard-Iloughmane and C. Le Roux, Bismuth(III) Lewis acids, in Acid Catalysis in Modern Organic Chemistry, ed. H. Yamamoto and K. Ishihara, John Wiley & Sons, New York, NY, USA, 2008, 551–588 CrossRef; V. A. Mulamoottil, Synlett, 2005, 2699 CrossRef; H. Gaspard-Iloughmane and C. Le Roux, Eur. J. Org. Chem., 2004, 2517 CrossRef; S. Antoniotti and E. Duñach, C. R. Chimie, 2004, 7, 679 CrossRef; S. Antoniotti, Synlett, 2003, 1566 CrossRef; N. M. Leonard, L. C. Wieland and R. S. Mohan, Tetrahedron, 2002, 58, 8373 CrossRef; C. Le Roux and J. Dubac, Synlett, 2002, 181 CrossRef; S. Vidal, Synlett, 2001, 1194 CrossRef; H. Suzuki, T. Ikegami and Y. Matano, Synthesis, 1997, 249 CrossRef; M. Postel and E. Duñach, Coord. Chem. Rev., 1996, 155, 127 CrossRef; A. R. Salvador, S. A. C. Figueiredo, R. M. A. Pinto and S. M. Silvestre, Future Med. Chem., 2012, 4, 1495 CrossRef.
- T. Ollevier, J.-E. Bouchard and V. Desyroy, J. Org. Chem., 2008, 73, 331 CrossRef CAS.
- S. Kobayashi, T. Ogino, H. Shimizu, S. Ishikawa, T. Hamada and K. Manabe, Org. Lett., 2005, 7, 4729 CrossRef CAS.
- S. Kobayashi and C. Ogawa, Chem.–Eur. J., 2006, 12, 5954 CrossRef CAS.
- R. Qiu, Y. Qiu, S. Yin, X. Xu, S. Luo, C.-T. Au, W.-Y. Wong and S. Shimada, Adv. Synth. Catal., 2010, 352, 153 CrossRef CAS.
- O. A. Attanasi, G. Favi, G. Giorgi, F. Mantellini, V. Karapetyan and P. Langer, Tetrahedron, 2009, 65, 5456 CrossRef CAS.
- X. Zhang, R. Qiu, N. Tan, S. Yin, J. Xia, S. Luo and C.-T. Au, Tetrahedron Lett., 2010, 51, 153 CrossRef CAS.
- N. Tan, S. Yin, Y. Li, R. Qiu, Z. Meng, X. Song, S. Luo, C.-T. Au and W.-Y. Wong, J. Organomet. Chem., 2011, 696, 1579 CrossRef CAS; X. Zhang, S. Yin, R. Qiu, J. Xia, W. Dai, Z. Yu, C.-T. Au and W.-Y. Wong, J. Organomet. Chem., 2009, 694, 3559 CrossRef; R. Qiu, S. Yin, X. Zhang, J. Xia, X. Xu and S. Luo, Chem. Commun., 2009, 4759 RSC; R. Qiu, S. Yin, X. Song, Z. Meng, Y. Qiu, N. Tan, X. Xu, S. Luo, F.-R. Dai, C.-T. Au and W.-Y. Wong, Dalton Trans., 2011, 40, 9482 RSC ; for a review on these bismuth-containing heterocyclic compounds: S. Shimada, Curr. Org. Chem., 2011, 15, 601 CrossRef.
- C. Ogawa, S. Azoulay and S. Kobayashi, Heterocycles, 2005, 66, 201 CrossRef CAS; C. Ogawa, M. Kokubo and S. Kobayashi, Yuki Gosei Kagaku Kyokaishi, 2010, 68, 718 CrossRef.
- S. Répichet, A. Zwick, L. Vendier, C. Le Roux and J. Dubac, Tetrahedron Lett., 2002, 43, 993 CrossRef CAS; T. C. Wabnitz, J.-Q. Yu and J. B. Spencer, Chem.–Eur. J., 2004, 10, 484 CrossRef; T. Ollevier and E. Nadeau, Org. Biomol. Chem., 2007, 5, 3126 Search PubMed.
- H. Qin, N. Yamagiwa, S. Matsunaga and M. Shibasaki, J. Am. Chem. Soc., 2006, 128, 1611 CrossRef CAS.
- H. Qin, N. Yamagiwa, S. Matsunaga and M. Shibasaki, Chem.–Asian J., 2007, 2, 150 CrossRef CAS.
- X. Cheng, Y. Xia, H. Wei, B. Xu, C. Zhang, Y. Li, G. Qian, X. Zhang and W. Li, Eur. J. Org. Chem., 2008, 1929 CrossRef CAS; H. Wei, G. Qian, Y. Xia, K. Li, Y. Li and W. Li, Eur. J. Org. Chem., 2007, 4471 CrossRef.
- F. Mathia and P. Szolcsányi, Org. Biomol. Chem., 2012, 10, 2830 CAS.
- K. Komeyama, Y. Kouya, Y. Ohama and K. Takaki, Chem. Commun., 2011, 47, 5031 RSC.
- H. Kamisaki, T. Nanjo, C. Tsukano and Y. Takemoto, Chem.–Eur. J., 2011, 17, 626 CrossRef CAS.
- K. Komeyama, M. Miyagi and K. Takaki, Heteroat. Chem., 2008, 19, 644 CrossRef CAS.
- A.-L. Girard, T. Enomoto, S. Yokouchi, C. Tsukano and Y. Takemoto, Chem.–Asian J., 2011, 6, 1321 CrossRef CAS.
- H. Qin, N. Yamagiwa, S. Matsunaga and M. Shibasaki, Angew. Chem., Int. Ed., 2007, 46, 409 CrossRef CAS.
- Z.-P. Zhan, W.-Z. Yang, R.-F. Yang, J.-L. Yu, J.-P. Li and H.-J. Liu, Chem. Commun., 2006, 3352 RSC.
- K. Csatayová, S. G. Davies, J. A. Lee, K. B. Ling, P. M. Roberts, A. J. Russell and J. E. Thomson, Org. Lett., 2010, 12, 3152 CrossRef.
- W. Rao, P. Kothandaraman, C. B. Koh and P. W. H. Chan, Adv. Synth. Catal., 2010, 352, 2521 CrossRef CAS.
- N. Kawai, R. Abe, M. Matsuda and J. Uenishi, J. Org. Chem., 2011, 76, 2102 CrossRef CAS; N. Kawai, R. Abe and J. Uenishi, Tetrahedron Lett., 2009, 50, 6580 CrossRef.
- L. Wang and R. Fan, Org. Lett., 2012, 14, 3596 CrossRef CAS.
- P. W. Anzalone, A. R. Baru, E. M. Danielson, P. D. Hayes, M. P. Nguyen, A. F. Panico, R. C. Smith and R. S. Mohan, J. Org. Chem., 2005, 70, 2091 CrossRef CAS ; for seminal studies with other Lewis acids, see: I. E. Markó, A. Mekhalfia, D. J. Bayson and H. Adams, J. Org. Chem., 1992, 57, 2211 CrossRef.
- P. A. Evans and W. J. Andrews, Tetrahedron Lett., 2005, 46, 5625 CrossRef CAS; P. A. Evans, J. Cui, S. J. Gharpure, A. Polosukhin and H.-R. Zhang, J. Am. Chem. Soc., 2003, 125, 14702 CrossRef; P. A. Evans, J. Cui, S. J. Gharpure and R. J. Hinkle, J. Am. Chem. Soc., 2003, 125, 11456 CrossRef; P. A. Evans, J. Cui and S. J. Gharpure, Org. Lett., 2003, 5, 3883 CrossRef.
- P. Mochirian, F. Godin, I. Katsoulis, I. Fontaine, J.-F. Brazeau and Y. Guindon, J. Org. Chem., 2011, 76, 7654 CrossRef CAS.
- Y. Lian and R. J. Hinkle, J. Org. Chem., 2006, 71, 7071 CrossRef CAS.
- K. A. Bhatia, K. J. Eash, N. M. Leonard, M. C. Oswald and R. S. Mohan, Tetrahedron Lett., 2001, 42, 8129 CrossRef CAS.
- R. F. Lambert, R. J. Hinkle, S. E. Ammann, Y. Lian, J. Liu, S. E. Lewis and R. D. Pike, J. Org. Chem., 2011, 76, 9269 CrossRef CAS.
- R. J. Hinkle, Y. Lian, L. C. Speight, H. E. Stevenson, M. M. Sprachman, L. A. Katkish and M. C. Mattern, Tetrahedron, 2009, 65, 6834 CrossRef CAS.
- B. Leroy and I. E. Markó, Org. Lett., 2002, 4, 47 CrossRef CAS; B. Leroy and I. E. Markó, Tetrahedron Lett., 2001, 42, 8685 CrossRef.
- G. Sabitha, M. Bhikshapathi, S. Nayak, J. S. Yadav, R. Ravi and A. C. Kunwar, Tetrahedron Lett., 2008, 49, 5727 CrossRef CAS.
- P. A. Evans, A. Grisin and M. J. Lawler, J. Am. Chem. Soc., 2012, 134, 2856 CrossRef CAS.
- P. A. Evans and W. J. Andrews, Angew. Chem., Int. Ed., 2008, 47, 5426 CrossRef CAS.
- B. Kelly, J. M. Allen, R. E. Tundel and T. H. Lambert, Org. Lett., 2009, 11, 1381 CrossRef CAS.
- A. D. Fotiadou and A. L. Zografos, Org. Lett., 2011, 13, 4592 CrossRef CAS.
- P. Rubenbauer, E. Herdtweck, T. Strassner and T. Bach, Angew. Chem., Int. Ed., 2008, 47, 10106 CrossRef CAS.
- Z. Li, B. Plancq and T. Ollevier, Chem.–Eur. J., 2012, 18, 3144 CrossRef CAS.
- M. Rueping, B. J. Nachtsheim and A. Kuenkel, Org. Lett., 2007, 9, 825 CrossRef CAS.
- M. Rueping, B. J. Nachtsheim and E. Sugiono, Synlett, 2010, 1549 CrossRef CAS.
- M. Rueping, B. J. Nachtsheim and W. Ieawsuwan, Adv. Synth. Catal., 2006, 348, 1033 CrossRef CAS.
- S. K. De and R. A. Gibbs, Tetrahedron Lett., 2005, 46, 8345 CrossRef CAS.
- P. Rubenbauer and T. Bach, Tetrahedron Lett., 2008, 49, 1305 CrossRef CAS.
- T. Ollevier and Z. Li, Adv. Synth. Catal., 2009, 351, 3251 CrossRef CAS; T. Ollevier and Z. Li, Org. Biomol. Chem., 2006, 4, 4440 Search PubMed.
- S. Kobayashi, T. Kitanosono and M. Ueno, Synlett, 2010, 2033 CrossRef CAS.
- K. E. Judd and L. Caggiano, Org. Biomol. Chem., 2011, 9, 5201 CAS.
- T. Ollevier and T. M. Mwene-Mbeja, Synthesis, 2006, 3963 CrossRef CAS.
- H. Sun, R. Hua, S. Chen and Y. Yin, Adv. Synth. Catal., 2006, 348, 1919 CrossRef CAS.
- S. T.-C. Eey and M. J. Lear, Org. Lett., 2010, 12, 5510 CrossRef CAS.
- S. Ghosh, L. K. Kinthada, S. Bhunia and A. Bisai, Chem. Commun., 2012, 48, 10132 RSC.
- K. Komeyama, T. Yamada, R. Igawa and K. Takaki, Chem. Commun., 2012, 48, 6372 RSC; K. Komeyama, R. Igawa, T. Morimoto and K. Takaki, Chem. Lett., 2009, 38, 724 CrossRef CAS.
- M. Rueping, B. J. Nachtsheim and T. Scheidt, Org. Lett., 2006, 8, 3717 CrossRef CAS.
- H.-B. Sun, B. Li, R. Hua and Y. Yin, Eur. J. Org. Chem., 2006, 4231 CrossRef CAS.
- M. Rueping, B. J. Nachtsheim and A. Kuenkel, Synlett, 2007, 1391 CrossRef CAS.
- B. Cacciuttolo, S. Poulain-Martini and E. Duñach, Eur. J. Org. Chem., 2011, 3710 CrossRef CAS.
- G. Lemière, B. Cacciuttolo, E. Belhassen and E. Duñach, Org. Lett., 2012, 14, 2750 CrossRef.
- K. Komeyama, K. Takahashi and K. Takaki, Chem. Lett., 2008, 37, 602 CrossRef CAS.
- K. Komeyama, K. Takahashi and K. Takaki, Org. Lett., 2008, 10, 5119 CrossRef CAS.
- K. Komeyama, M. Miyagi and K. Takaki, Chem. Lett., 2009, 38, 224 CrossRef CAS.
- K. Komeyama, N. Saigo, M. Miyagi and K. Takaki, Angew. Chem., Int. Ed., 2009, 48, 9875 CrossRef CAS.
- X.-C. Wang, R.-L. Yan, M.-J. Zhong and Y.-M. Liang, J. Org. Chem., 2012, 77, 2064 CrossRef CAS.
- Z. Wang and S. Fang, Eur. J. Org. Chem., 2009, 5505 CrossRef CAS.
- J. Godeau, S. Olivero, S. Antoniotti and E. Duñach, Org. Lett., 2011, 13, 3320 CrossRef CAS; J. Godeau, F. Fontaine-Vive, S. Antoniotti and E. Duñach, Chem.–Eur. J., 2012, 18, 16815 CrossRef.
- F. Grau, A. Heumann and E. Duñach, Angew. Chem., Int. Ed., 2006, 45, 7285 CrossRef CAS.
- F. Ono, Y. Ishikura, Y. Tada, M. Endo and T. Sato, Synlett, 2008, 2365 CAS.
- J. A. R. Salvador and S. M. Silvestre, Tetrahedron Lett., 2005, 46, 2581 CrossRef CAS.
- Y. Bonvin, E. Callens, I. Larrosa, D. A. Henderson, J. Oldham, A. J. Burton and A. G. M. Barrett, Org. Lett., 2005, 7, 4549 CrossRef CAS; E. Callens, A. J. Burton, A. J. P. White and A. G. M. Barrett, Tetrahedron Lett., 2008, 49, 3709 CrossRef.
- J.-M. Vatèle, Synlett, 2008, 1785 CrossRef; J.-M. Vatèle, Tetrahedron, 2010, 66, 904 CrossRef.
- B. Barnych and J.-M. Vatèle, Synlett, 2011, 2048 CAS.
- X.-F. Bai, F. Ye, L.-S. Zheng, G.-Q. Lai, C.-G. Xia and L.-W. Xu, Chem. Commun., 2012, 48, 8592 RSC.
- B. N. Harris, P. P. Patel, C. P. Gobble, M. J. Stark and C. De Meo, Eur. J. Org. Chem., 2011, 4023 CrossRef CAS.
- E. Callens, A. J. Burton and A. G. M. Barrett, Tetrahedron Lett., 2006, 47, 8699 CrossRef CAS.
- C. Lherbet, D. Soupaya, C. Baudoin-Dehoux, C. André, C. Blonski and P. Hoffmann, Tetrahedron Lett., 2008, 49, 5449 CrossRef CAS.
- X. Li, X. Yang, H. Chang, Y. Li, B. Ni and W. Wei, Eur. J. Org. Chem., 2011, 3122 CrossRef.
- J. A. R. Salvador, R. M. A. Pinto, R. C. Santos, C. Le Roux, A. Matos Beja and J. A. Paixão, Org. Biomol. Chem., 2009, 7, 508 CAS.
- R. M. A. Pinto, J. A. R. Salvador, C. Le Roux and J. A. Paixão, J. Org. Chem., 2009, 74, 8488 CrossRef CAS.
- J. A. R. Salvador, V. M. Moreira, R. M. A. Pinto, A. S. Leal and C. Le Roux, Adv. Synth. Catal., 2011, 353, 2637 CrossRef CAS.
- R. Hayashi and G. R. Cook, Tetrahedron Lett., 2008, 49, 3888 CrossRef CAS.
- F. M. Koch and R. Peters, Chem.–Eur. J., 2011, 17, 3679 CrossRef CAS; F. M. Koch and R. Peters, Angew. Chem., Int. Ed., 2007, 46, 2685 CrossRef.
- N. Lassauque, G. Franciò and W. Leitner, Eur. J. Org. Chem., 2009, 3199 CrossRef CAS.
- C. D. Weatherly, J. W. Rigoli and J. M. Schomaker, Org. Lett., 2012, 14, 1704 CrossRef CAS.
- Z. Chen, X. Yu, M. Su, X. Yang and J. Wu, Adv. Synth. Catal., 2009, 351, 2702 CrossRef CAS.
- O. M. Demchuk, K. Kielar and K. M. Pietrusiewicz, Pure Appl. Chem., 2011, 83, 633 CrossRef CAS.
- N. Mahajan, S. Koul and T. K. Razdan, J. Heterocycl. Chem., 2011, 48, 1302 CrossRef CAS.
- K. Komeyama, Y. Ohama and K. Takaki, Chem. Lett., 2011, 40, 1103 CrossRef CAS.
- K. V. Sashidhara, S. R. Avula, L. R. Singh and G. R. Palnati, Tetrahedron Lett., 2012, 53, 4880 CrossRef CAS.
- T. Blümke, Y.-H. Chen, Z. Peng and P. Knochel, Nat. Chem., 2010, 2, 313 CrossRef.
|
This journal is © The Royal Society of Chemistry 2013 |
Click here to see how this site uses Cookies. View our privacy policy here.