DOI:
10.1039/C3GC40702K
(Paper)
Green Chem., 2013,
15, 1880-1888
Comparative performance evaluation and systematic screening of solvents in a range of Grignard reactions†
Received 14th April 2013, Accepted 3rd May 2013
First published on 3rd May 2013
Abstract
The solvent effect on the Grignard reaction of benzyl, aryl and heteroaromatic substrates has been systematically evaluated based on reaction efficiency, ease of subsequent work-up, safety and greenness. 2-Methyltetrahydrofuran (2-MeTHF), which can be derived from renewable resources, had at least an equal if not a superior overall process most notably in suppressing the Wurtz coupling by-product from the benzyl Grignard reactions. It is therefore a recommended alternative solvent to Et2O and THF for the preparation of most Grignard reagents and their subsequent reactions.
1 Introduction
The American Chemical Society's Green Chemistry Institute Pharmaceutical Roundtable (GCIPR) was developed in 2005 to encourage the integration of green chemistry and engineering into the pharmaceutical industry and to promote sustainable manufacturing. GCIPR presently has 16 member companies including contract research/manufacturing organizations, generic pharmaceuticals, and related companies. In 2009, GCIPR identified that a preponderance of commonly used organometallic reagents was supplied in solvents lacking greenness from an environmental and process safety perspective. Unfortunately, the solvent used for the organometallic reagent biases a process towards a less green position from the outset of development. Frequently these sub-optimal solvents persist through the development lifecycle all the way through to commercial manufacturing. To address these issues, a GCIPR team was assembled with the objective to catalyse suppliers to provide organometallic reagents in the greenest possible solvent. In 2010, feedback was received from several organometallic reagent suppliers that the strongest impact could be realized by conducting independent research and publishing the findings with head to head performance comparisons between different solvents. Based on this feedback, the GCIPR developed a research grant to systematically evaluate solvents for Grignard reactions. In 2011, the Zhang group at UMass Boston was awarded a grant from GCIPR to carry out this targeted research and development. Preliminary results of these R&D efforts were presented at the 16th Annual Green Chemistry and Engineering Conference.1 Herein we report the full set of results, which includes explorations of benzyl, aryl and heteroaromatic Grignard reactions.1.1 Background of the Grignard reaction
Victor Grignard and Paul Sabatier received the Nobel Prize in 1912 for the discovery of what was later called the Grignard reaction.2 The classic Grignard reaction is an organometallic reaction in which alkyl- or aryl-magnesium halides (Grignard reagents) add to carbonyl groups of aldehydes and ketones to generate a new carbon–carbon bond. In addition to the broad applications on a research scale, Grignard reactions have also been adapted to a large scale and are the pivotal steps in the manufacture of numerous complex molecules. From a pharmaceutical perspective, the Grignard reaction has been employed as a key step in numerous syntheses such as that of tramadol,3 ravuconazole,4 naproxen,5 ibuprofen,6 aprepitant,7 droloxifene,8 and tamoxifen9 (Fig. 1). For each molecule shown in Fig. 1, the bond prepared by the Grignard reaction is highlighted.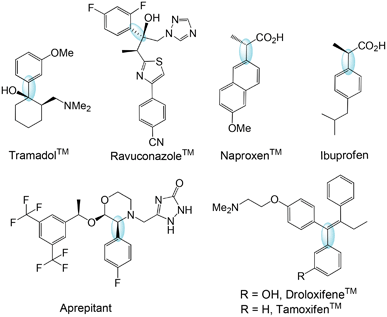 |
| Fig. 1 Pharmaceutical syntheses featuring the Grignard reaction. | |
Despite significant utility, Grignard reactions present challenges particularly on scaling-up. From a safety standpoint, the initiation step is often hard to control, and is strongly exothermic as is the subsequent Grignard reaction. In some instances research scale chemistry has been recently carried out using continuous flow methods to alleviate the exotherm hazards of the Grignard reaction.10 Furthermore, the solvents typically employed such as Et2O and THF have low boiling points, and are capable of forming peroxides, which are both hazardous as well as detrimental to the reaction. In addition, the reactions typically use the reactive Grignard metal, and the product profile is often complicated by the symmetrical homo (Wurtz) coupling by-product and the proteo by-product resulting from direct reduction of the substrate or unfavourable water ingress into the reaction. The amounts of oxygen and moisture intake are also key parameters impacting both the success of the initial reagent formation and subsequent processes of Grignard reactions. Numerous methods have been devised to initiate sluggish Grignard reactions.11 All these derive from the common theme of weakening the passivation layer of magnesium oxide, thereby exposing highly reactive magnesium to the organic halide.
1.2 Selection of substrates and solvents for Grignard reactions
Because of the broad use in the pharmaceutical and fine chemicals industry, benzyl, aryl and heteroaromatic halides were identified as the representative substrates to systematically evaluate the solvent effect for the Grignard reactions. The reaction solvent is the key component in the formation of the Grignard reagent and reaction. From a process standpoint, the solvent typically represents the largest fraction of the process mass12 and as such its judicious selection for work-up, safety, cost and reaction profile is critical. Numerous Grignard reagents are available commercially, and as previously mentioned the vast majority of these are offered either as THF or Et2O solutions. The use of commercially prepared Grignard solutions provides an advantage for the synthetic chemist in that the hazardous initiation step is removed. However, it causes problems in that the subsequent Grignard reactions are also performed in these solvents, though co-solvents can be introduced for purposes of solubility or reactivity. It has long been recognized that the solvent in which a Grignard reagent is formed has a significant effect on its reactivity due to the formation of potential aggregates with a chelating solvent, and a redistribution of organomagnesium species in the Schlenk equilibrium. THF and in particular Et2O are not optimal solvents for scale-up work due to their potential for peroxide formation, as well as physical hazards such as their low boiling points and flashpoints. An ideal alternative solvent would present an improved reaction profile, and allow for an operationally simpler work-up. From an environmental standpoint, the optimal solvent would also be one with a better safety profile, and ideally available from renewable sources.13 Aycock14 has presented initial data showing the promising nature of 2-MeTHF for Grignard reactions, and Clarke15 has employed Grignard reagents of significantly enhanced concentrations (up to 5 M) in cross-coupling chemistry using this solvent. 2-MeTHF can be derived from a renewable source,16 is more stable to acids and bases than THF, is less prone to peroxide formation, and is immiscible with water thus enabling an easier phase-split for work-up.17 Cyclopentylmethyl ether (CPME), which can be produced directly from cyclopentene, has also been promoted as a potential alternative solvent for Grignard reactions.18 CPME is resistant to peroxide formation, and like 2-MeTHF it is more easily dried than typical ethereal solvents due to formation of an azeotrope with water. Merck recently reported the results of oral toxicity studies for 2-MeTHF and CPME.19 For both these solvents the results were negative for genotoxicity and mutagenicity which should assist in favourable long-term ICH classifications. Although widely promoted as greener solvents, only a handful of Grignard reagents are available in 2-MeTHF or CPME. Despite the initial promising results demonstrated for 2-MeTHF and CPME, to the best of our knowledge, no systematic and unbiased evaluation of solvent performance of a range of solvents has been reported in the literature. For the purpose of comparison, other solvents such as diethoxymethane (DEM),20 toluene, and diglyme are also included in our study (Table 1).
Table 1 Physical property of solvents used for Grignard reactions
| Boiling point (°C) | Flash point (°C) | Dipole moment (D) | Dielectric constant | Solubility in water (g 100 g−1) | Solubility of water in solvent (g 100 g−1) |
---|
Et2O | 35 | −45 | 1.15 | 4.2 | 6.5 | 1.2 |
THF | 66 | −14 | 1.63 | 7.6 | mis | mis |
DEM | 88 | −7 | | | 4.2 | 1.3 |
2-MeTHF | 80 | 11 | 1.38 | 7.0 | 14 | 4.4 |
CPME | 106 | −1 | 1.27 | 4.8 | 1.1 | 0.3 |
Toluene | 111 | 4 | 0.36 | 2.4 | 0.05 | |
Diglyme | 162 | 67 | 1.6 | 7.2 | mis | mis |
2 Results and discussion
The approach used in this study was to evaluate Grignard reactions of the selected substrates in the seven solvents shown in Table 1. The Grignard reagents were generated by reacting the alkyl- or aryl-halides with commercial grade magnesium turnings. The in situ generated Grignard reagents were allowed to react with the electrophile. In the solvent screening reactions, the reaction mixtures were analysed by GC-MS or LC-MS to determine the ratio of the product and by-products. Gram-scale reactions were then conducted under optimized conditions in selected solvents to examine the ease of product isolation and determine the yield. Other than solvents, key parameters such as temperature and activators were also evaluated. It is important to note in these systems that the reaction of the magnesium metal with the aryl or alkyl halide forms the Grignard reagent, and is not classified as the actual Grignard reaction, though both processes are often carried out sequentially and impurities generated from the Grignard reagent formation step are carried into the Grignard reaction.2.1 Benzyl Grignard reactions
Benzyl Grignard reactions are well-known and have been utilized for the synthesis of important pharmaceutical compounds such as propoxyphene21 and several selective norepinephrine inhibitors.22 A major issue associated with benzyl Grignard reagents is the generation of substantial quantities of the undesired Wurtz coupling by-product, especially from the more reactive benzyl bromides. In our experiments, the in situ generated benzyl Grignard reagents from either benzyl bromide or chloride were allowed to react with 2-butanone in different solvents to evaluate the solvent effect (Scheme 1).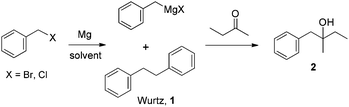 |
| Scheme 1 Benzyl Grignard reaction. | |
A screening using the full range of selected solvents was carried out to determine whether the desired product was observed prior to the optimization of reaction conditions for the subsequent scale-up reaction and product isolation. The reaction mixtures were analysed by GC-MS to determine the ratio of the product and the Wurtz coupling by-product. Using iodine as an activator, only the reactions in Et2O, THF, and 2-MeTHF gave the desired Grignard product 2. GC-MS analysis indicated that the Et2O and 2-MeTHF systems both gave an 80
:
20 ratio of the product to the Wurtz coupling by-product (Table 2, entries 1 and 3). Interestingly, the chemoselectivity is reversed to a 30
:
70 ratio in the THF system (entry 2). Reactions in CPME and DEM gave a small amount of the Grignard product using DIBAl-H in THF as an activator (entries 4 and 5).
Table 2 Solvent screening of benzyl bromide Grignard reaction
Entry | Solvent | Activator | 2 : 1 Ratioa |
---|
Determined by GC-MS. |
---|
1 | Et2O | I2 | 80 : 20 |
2 | THF | I2 | 30 : 70 |
3 | 2-MeTHF | I2 | 80 : 20 |
4 | CPME | DIBAL-H | 35 : 65 |
5 | DEM | DIBAL-H | 20 : 80 |
6 | Toluene | I2 or DIBAL-H | — |
7 | Diglyme | I2 or DIBAL-H | — |
Grignard reaction of benzyl chloride in Et2O or 2-MeTHF improved the product to Wurtz coupling by-product ratio to 90
:
10 (Table 3, entries 1 and 3), but it still remained 30
:
70 for the THF system (entry 2). Reactions in CPME, DEM, toluene, and diglyme afforded some limited amounts of the Grignard product by using DIBAL-H as an activator (entries 4–7).
Table 3 Solvent screening of benzyl chloride Grignard reaction
Entry | Solvent | Activator | 2 : 1 ratioa |
---|
Determined by GC-MS. |
---|
1 | Et2O | I2 | 90 : 10 |
2 | THF | I2 | 30 : 70 |
3 | 2-MeTHF | I2 | 90 : 10 |
4 | CPME | DIBAL-H | 48 : 52 |
5 | DEM | DIBAL-H | 55 : 45 |
6 | Toluene | DIBAL-H | 30 : 70 |
7 | Diglyme | DIBAL-H | 20 : 80 |
The high yield and chemoselectivity in 2-MeTHF and Et2O cannot be explained solely based on polarity, since solvents with higher and lower dielectric constants produce much greater quantities of the Wurtz by-product. Rather the aggregation state of the Grignard reagent is likely a key contributor. Overall, the equivalent performance of 2-MeTHF to Et2O justifies the preferential usage of 2-MeTHF for the benzyl Grignard class of reaction, since Et2O has a much lower boiling point, a lower flashpoint, and a higher propensity for peroxide formation.
After the solvent screening reactions, gram-scale reactions of benzyl chloride were carried out under optimized conditions in the selected solvents for product isolation (Table 4). As expected, excellent yields of the Grignard product were obtained from the reactions in both Et2O (94%) and 2-MeTHF (90%), but due to a significant amount of the Wurtz by-product, the yield was poor in THF (27%). Low product yields were obtained from the CPME (45%) and DEM (45%) systems using DIBAL-H in THF as an activator. We also conducted the reactions in a hybrid solvent system by generating the Grignard reagent in 2-MeTHF and adding the electrophile 2-butanone in CPME or toluene. Compared to the reactions in the single CPME or toluene solvent, which did not afford the Grignard product using I2 as an activator, the hybrid systems gave 60% and 51% product yields for the reactions involving CPME and toluene respectively as the secondary solvents (entries 6 and 7).
Table 4 Benzyl chloride Grignard reaction
Entry | Solvent | Activator | 2a |
---|
Isolated yield calculated based on the electrophile limiting reagent. Solvent for Grignard reagents. Solvent for electrophiles. |
---|
1 | Et2O | I2 | 94% |
2 | THF | I2 | 27% |
3 | 2-MeTHF | I2 | 90% |
4 | CPME | DIBAL-H | 45% |
5 | DEM | DIBAL-H | 45% |
6 | 2-MeTHFb/CPMEc | I2 | 60% |
7 | 2-MeTHFb/Toluenec | I2 | 51% |
Since ethereal benzylmagnesium chloride solutions are available from several commercial sources, we thought it was important to assess the purity of the reagent. Seven benzylmagnesium chloride preparations in THF, 2-MeTHF and Et2O were purchased from five suppliers. The benzyl Grignard solutions obtained from commercial suppliers were analysed by HPLC and titration (Table 5) within one month of the receipt of the samples.23 For all preparations, substantial quantities of impurities were observed by LC analysis and titration confirmed that the strength of these Grignard reagents was consistently 25–50% below the label claim. For the THF systems, the Wurtz by-product 3 was the major observed impurity ranging from 12–33%. In addition, two additional impurities, benzyl alcohol 4 and benzene pentan-1-ol 5, were prominent. Benzyl alcohol 4 is likely formed by the known reaction of the Grignard reagent with oxygen, followed by hydrolysis whereas benzene pentan-1-ol 5 is likely produced from a radical reaction between THF and the Grignard reagent. This impurity was observed in all THF preparations, and as expected not observed in the 2-MeTHF and Et2O preparations. Interestingly, internal preparation of 1 M benzyl magnesium chloride THF solutions reveals only trace amounts (<0.1%) of 2-benzene pentanol after 3 months of ambient storage under nitrogen. These data imply that the commercially purchased benzyl magnesium chloride THF solutions were of significant age and/or exposed to non-ideal storage conditions prior to the receipt of these materials by the customer. Because of these issues, suppliers who produce Grignard reagents are encouraged to establish expiry date specifications and re-test these reagents if they exceed the expiry date. While 2-MeTHF contained much less Wurtz by-product and no benzene pentan-1-ol or related by-product, the amount of benzyl alcohol was relatively high, indicating strong oxygen sensitivity. Overall, these data support the optimal preparation of benzyl Grignard reagents in 2-MeTHF. However, based on the apparent air sensitivity, purchase of commercial solutions of benzyl Grignard reagents is not recommended, and rather in situ preparation with 2-MeTHF and direct use appear the best option.
Table 5 Commercial benzyl magnesium chloride impurity analysis

|
---|
Supplier | Solvent | Label purity (M) | Titrated purity (M) | HPLC purity (%) | 3 (%) | 4 (%) | 5 (%) |
---|
A (Batch1) | THF | 2.0 | 1.2 | 58.6 | 24.5 | 6.5 | 9.8 |
A (Batch1) | THF | 2.0 | 1.1 | 44.4 | 33.1 | 16.1 | 5.95 |
A (Batch1) | THF | 2.0 | 1.1 | 46.8 | 33.0 | 13.2 | 5.9 |
B | THF | 1.5 | 0.75 | 52.3 | 16.5 | 14.7 | 15.1 |
C | THF | 1.5 | 0.91 | 76.5 | 12.3 | 1.6 | 5.7 |
D | Et2O | 1.0 | 0.75 | 62.2 | 14.1 | 20.8 | 0.0 |
E | 2-MeTHF | 1.0 | 0.65 | 76.6 | 6.6 | 15.1 | 0.0 |
2.2.1 Aryl Grignard reactions for benzyl alcohol. The use of aryl Grignard reagents in organic chemistry is also well established in the literature, and has been exploited in the synthesis of a number of important pharmaceutical compounds such as naproxen,5 emend (aprepitant),7 droloxifene,8 and tamoxifen.9 It is another important transformation we have selected for the investigation of the solvent effect. In a similar manner to that described above, the reaction of the in situ prepared Grignard reagent of 3-bromoanisole with 2-butanone in seven different solvent systems was evaluated (Scheme 2).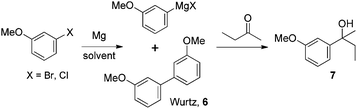 |
| Scheme 2 Anisole Grignard reaction. | |
The results summarized in Table 6 indicate that the reaction proceeded well in Et2O, THF or 2-MeTHF using iodine as the initiator and heating the reaction to 45 °C (entries 1–3). For CPME, DEM, toluene, and diglyme solvent systems, no reaction was observed even when the reaction was heated to 60 °C. Utilizing DIBAL-H in THF as the initiator, it was observed that an almost identical yield could be obtained to that with iodine when the reaction was run in 2-MeTHF (entries 3 and 4). It was also found that acceptable yields of the desired product could be realized using solvents such as CPME, DEM, and diglyme albeit at an elevated temperature (entries 5–7). At this juncture, it became critical to determine whether this is an effect which could be attributed purely to the nature of the initiator, or a more subtle co-solvent effect given that we had utilized the commercially available DIBAL-H in THF solution. Switching to the alternative preparation of the DIBAL-H in toluene (entry 8), and hence eliminating the ethereal co-solvent, we observed a significant drop in yield demonstrating again the benefit of employing such a solvent. However, clearly the judicious selection of the initiator is important as well given that no reaction is observed for solvents such as CPME when iodine was utilized.
Table 6 Grignard reaction of anisole bromide
Entry | Solvent | Tempa (°C) | Activator | 7b (%) |
---|
For generating Grignard reagents. Isolated yield. In toluene. |
---|
1 | Et2O | 25–35 | I2 | 79 |
2 | THF | 25–45 | I2 | 68 |
3 | 2-MeTHF | 25–45 | I2 | 75 |
4 | 2-MeTHF | 25–45 | DIBAL-H | 72 |
5 | CPME | 60 | DIBAL-H | 72 |
6 | DEM | 60 | DIBAL-H | 60 |
7 | Diglyme | 60 | DIBAL-H | 70 |
8 | CPME | 60 | DIBAL-Hc | 30 |
Overall the results show equivalent performance of 2-MeTHF to THF and Et2O for the aryl Grignard chemistry. Given the other advantages associated with the employment of 2-MeTHF as a solvent one should make this the preferred choice for the aryl Grignard reaction class. CPME and DEM have been promoted as new green solvent alternatives, and have been demonstrated in certain cases to be effective solvents for Grignard chemistry. In the current evaluation of aryl Grignard reactions though, these solvents are shown to be significantly inferior to Et2O, THF and 2-MeTHF. The observed differences in reactivity here cannot be simply explained in terms of polarity, but instead reflect complex solvent perturbations of the Schlenk equilibrium leading to changes in aggregation state of the reactive magnesium species. Glymes have also been promoted as solvents or additives for Grignard reactions,24 though on inspection, the inferior results obtained using diglyme are not difficult to rationalize. The reaction would be perceived to be favoured in terms of both the polarity and the donating effect of the solvent. However, one would expect the actual rate of the reaction to be slow due to the greater amount of energy required to displace the strongly coordinating solvent from the reaction intermediates. This is reflected to some degree in the elevated temperature required to promote this reaction, and suggests that although the reactions may be slow, the lowered reactivity potentially offers the opportunity to obtain higher selectivity using such solvents.
Experiments were also conducted in a series of solvents to examine the potential to use 3-chloroanisole to generate the Grignard reagent. Reactions were carried out in Et2O, THF and 2-MeTHF using either iodine or DIBAL-H as an initiator in a range of temperatures, though under none of the examined reaction conditions was the desired product observed. Knochel's method to generate the aryl Grignard reagent was also evaluated using 3-bromoanisole as the substrate (Scheme 3).25 Due to the ready commercial availability of the requisite Grignard reagent in THF, this was the solvent used for evaluation. As shown in Scheme 3, the reaction proceeds smoothly to provide a similar yield to that obtained above (Table 6, entry 3). However, this method does possess several advantages such as (1) no initiator is required, (2) the reactions proceed at ambient temperature, and most notably (3) only a trace amount of the Wurtz coupling product was observed. It is important to note that this reagent is also commercially available in 2-MeTHF.
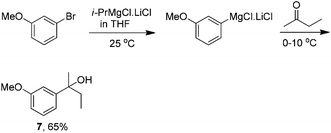 |
| Scheme 3 Knochel's method for bromoanisole Grignard reaction. | |
2.2.2 Aryl Grignard reactions for benzyl tramadol. To further examine the solvent effect for aryl Grignard reactions, our attention was turned to the synthesis of tramadol,3 which is a well-known analgesic for the treatment of pain, and an important model compound in opioid research. Tramadol is marketed as a racemic mixture with the final step of the synthesis featuring a Grignard reaction of (3-methoxy)phenylmagnesium bromide with racemic 2-((dimethylamino)methyl) cyclohexanone to afford tramadol as a mixture of diastereomers in an 80
:
20 ratio in favour of the desired cis isomer. The desired compound is then obtained by recrystallization as its hydrochloride salt.3 Not only will this study enable a further comparison of the ability of the solvents screened to promote the Grignard reaction, but it will also allow us to examine whether the solvent has any influence on the diastereomeric ratio obtained from the reaction. Previous studies have demonstrated that it is possible to increase the amount of the cis-8 by addition of an inorganic lithium salt and use of DEM as a co-solvent,26 though we felt it would be instructive to learn if any variations were observed in different reaction solvents (Scheme 4).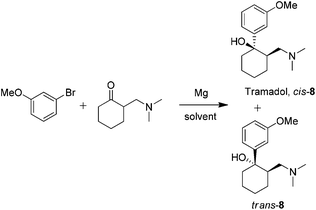 |
| Scheme 4 Grignard reaction for tramadol. | |
Small-scale reactions for solvent screening were carried out to determine whether the desired product was observed prior to optimization of the most promising conditions for scale-up, product isolation, and determination of the diastereomeric ratio. The results obtained were similar to our earlier findings with Et2O, THF and 2-MeTHF standing out for further evaluation. For the gram-scale reactions, mild heating was applied to push the reactions to completion (Table 7, entries 1–3). The yield with 2-MeTHF was slightly higher than the other two solvents possibly reflecting a more facile work-up and isolation. Examining the diastereomeric ratio for the reactions in 2-MeTHF (83
:
17) and THF (80
:
20) indicated a slight enhancement in the formation of the desired cis-8 in 2-MeTHF. In agreement with the previous exploration of aryl Grignards, it was also possible to get relatively good yields of the desired products in CPME or DEM solvents at slightly elevated temperature (60 °C) using DIBAL-H in THF as the initiator.
Table 7 Grignard reaction for tramadol
Entry | Solvent | Tempa (°C) | Activator | Yieldb (%) | 8cis : transc |
---|
For generating Grignard reagents. Isolated yield. Determined by LC. |
---|
1 | Et2O | 25–35 | I2 | 70 | 80 : 20 |
2 | THF | 25–45 | I2 | 67 | 80 : 20 |
3 | 2-MeTHF | 25–45 | I2 | 78 | 83 : 17 |
4 | CPME | 60 | DIBAL-H | 66 | 80 : 20 |
5 | DEM | 60 | DIBAL-H | 52 | 85 : 15 |
2.3 Heteroaromatic Grignard reactions
Heteroaromatic systems are of paramount importance in the pharmaceutical industry and it is important to assess the effect of solvents on the Grignard reaction performed on such substrates. 3-Methyl thiophene was selected as the substrate for deprotonation and subsequent functionalization through the Grignard reaction. Several studies have been reported on this system involving Li or Mg-based deprotonations as well as transmetallation approaches.27 The conditions including temperature and additives have been shown to have a dramatic effect on both the regioselectivity and the subsequent reactivity of the intermediate organometallic species. Workers at Amgen have recently reported a practical, robust method to access metallothiophenes, and their utilization in the regioselective synthesis of 2,4-disubstituted thiophenes.28 Under the reported optimized conditions, treatment of a slight excess of 3-methylthiophene with i-PrMgCl (1.1 equiv.) and 2,2,6,6-tetramethylpiperidine (TMP-H) (0.13 equiv.) in THF at 66 °C followed by trapping of the intermediate species in excess solvent at reduced temperature led to moderate to good yields of the desired thiophenes with excellent regioselectivity. We felt that this study serves as an excellent starting point to probe this chemistry in a variety of solvents paying particular attention to both the overall yield and the regioselectivity of the initial deprotonation. Shown in Scheme 5 is the deprotonation and trapping steps being performed in the solvent under investigation. It is important to note however that the i-PrMgCl is in itself supplied as a 2 M THF solution, and given this, there is always an ethereal solvent present under the reaction conditions. However, the solvent ratio in the deprotonation is approximately 1
:
1 THF–screening solvent, and in addition the electrophile (DMF or benzaldehyde) in the trapping step is introduced as a solution in the screening solvent.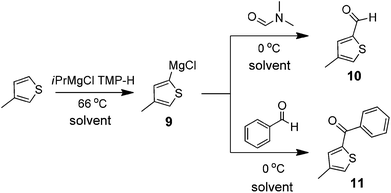 |
| Scheme 5 Grignard reaction of 3-methyl thiophene. | |
As it has been previously documented, several by-products such as Wurtz coupling or the proteo derivative can arise from the intermediate Grignard species. In addition, it is possible to obtain regioisomeric species arising from deprotonation and trapping at the 2-position of the thiophene. Experiments were conducted evaluating two electrophiles (DMF and benzaldehyde). The results obtained by trapping with DMF under Bouvealt formylation24 conditions to provide the aldehyde are summarized in Table 8. All the solvents investigated performed well to give a high yield of product 10 in a regioselective fashion. It was noticed that 2-MeTHF does provide a slightly higher yield of the desired product (entry 3). Taking into account the other advantages of employing this solvent, such as ease of work-up, and the fact that as noted previously, i-PrMgCl is also available as a solution in this solvent, this should be made the solvent of choice for conducting this type of chemistry. In a similar manner, the reaction was conducted using benzaldehyde as the electrophile, and the results are also summarized in Table 8. Once again, all the solvents performed well in this reaction providing the desired product 11 in good yield, and in a regioselective fashion. 2-MeTHF was noted to slightly outperform the other solvents evaluated in the chemistry.
Table 8 Reaction of 9 with DMF or benzaldehyde
Entry | Solvent | 10a (%) | 11a (%) |
---|
Isolated yield. |
---|
1 | Et2O | 67 | 78 |
2 | THF | 67 | 80 |
3 | 2-MeTHF | 72 | 82 |
4 | CPME | 60 | 70 |
5 | DEM | 54 | 65 |
6 | Toluene | 50 | 65 |
7 | Diglyme | — | 68 |
Of additional interest in this chemistry was the ability to tune the reaction conditions to also obtain the corresponding ketone through a Mg-mediated Oppenauer oxidation of the intermediate alcohol. This has been reported by Knochel,29 and it is believed that the role of LiCl in the system is crucial in promoting this reaction. The LiCl is proposed to solubilise the intermediate alkoxide in the reaction solvent as well as activate the carbonyl function of benzaldehyde by acting as a Lewis acid.
3 Experimental section
General procedure for the Grignard reactions of benzyl halides with 2-butanone
Magnesium turnings (2.33 g, 9.7 mmol) and iodine (0.57 g, 0.45 mmol) were placed in a dry, nitrogen flushed 50 mL flask equipped with a magnetic stirrer, a thermometer and a septum. The flask was warmed to 40 °C for 5 min to partially vaporize the iodine. To the reaction mixture was added 1 mL of 2-MeTHF and then cooled to 0 °C. A continuous stream of dry nitrogen was passed via a septum to avoid moisture. A portion of 10% of a benzyl chloride solution (11.56 g, 9.01 mmol in 10 mL of 2-MeTHF) was added in a dropwise fashion. The exothermic reaction was observed and the temperature increased to 10–15 °C. The remaining benzyl chloride solution was added slowly over 40 min by maintaining the temperature below 10 °C. A grey suspension of the Grignard reagent was generated after the complete addition of benzyl chloride. The formation of a Grignard complex was confirmed by extracting an aliquot and diluting in methanol for GC-MS analysis. The Grignard complex was stirred at 0 °C for 30 min and then a solution of 2-butanone (3.24 g, 4.5 mmol in 8 mL of 2-MeTHF) was added slowly maintaining the temperature below 5 °C. The reaction mixture was stirred at room temperature for 4 h and then poured into a beaker containing 10 g of NH4Cl in 80 mL of ice water. The mixture was extracted with ether (3 × 30 mL) and the combined organic layers were dried over Na2SO4 and concentrated in vacuo. The resulted crude product was purified by flash chromatography over silica gel eluting with 7
:
3 hexane–ethyl acetate to yield product 2 as a colorless oil (6.7 g, 90%).30 The isolation of the product was carried out to determine an accurate yield.General procedure for the Grignard reaction of 3-bromoanisole with 2-butanone
Magnesium turnings (2.33 g, 9.7 mmol) and iodine (0.57 g, 0.45 mmol) were placed in a dry, nitrogen flushed 50 mL flask equipped with a magnetic stirrer, a thermometer and a septum. The flask was warmed to 40 °C for 5 min to partially vaporize the iodine. A continuous stream of dry nitrogen was passed via a septum to avoid moisture. To the flask, 10% of 3-bromoanisole solution (16.88 g, 9.07 mmol in 10 mL of 2-MeTHF) was slowly added at room temperature. An exothermic reaction was observed and the temperature increased to 45 °C. The remaining 3-bromoanisole solution was added slowly over 40 min while maintaining the temperature around 45 °C. The Grignard reagent was generated as a pale yellow solution after the complete addition of 3-bromoanisole. The formation of a Grignard complex was confirmed by extracting an aliquot and diluting in methanol for GC-MS analysis. The Grignard complex was stirred for 30 min at 25 °C and then cooled to 0 °C. A solution of 2-butanone (5 g, 6.9 mmol in 6 mL of 2-MeTHF) was added slowly maintaining the temperature below 0 °C. The reaction mixture was stirred at room temperature for 4 h and then poured into a beaker containing 10 g of NH4Cl in 80 mL of ice water. The mixture was extracted with ether (3 × 30 mL) and the combined organic layers were dried over Na2SO4 and concentrated in vacuo. The resulting crude product was purified by flash chromatography over silica gel eluting with 7
:
3 hexane–ethyl acetate to yield product 7 as a colorless oil (9.4 g, 75%).31Knochel's method for the Grignard reaction of 3-bromoanisole with 2-butanone
A solution of i-PrMgCl·LiCl (1.33 M in THF, 2.6 mL, 2.6 mmol) was charged to a dry 10 mL round bottom flask equipped with a magnetic stirrer and a septum under a nitrogen atmosphere. The 3-bromoanisole (482 mg, 2.6 mmol) was added to a single portion. The reaction mixture was stirred at room temperature for 8 h. The conversion to the Grignard complex was checked by GC-MS. The reaction mixture was cooled to 0 °C and a solution of 2-butanone (205 mg, 2.86 mmol in 1 mL of THF) was added slowly maintaining the temperature below 5 °C. After the reaction mixture was stirred at room temperature for about 4 h, it was poured into a beaker containing 0.5 g of NH4Cl in 12 mL of ice water. After extracting with ether (3 × 5 mL), the combined organic layers were dried over Na2SO4 and concentrated in vacuo to give product 7 as a colorless oil (280 mg, 60%).General procedure for the Grignard reaction of tramadol
Magnesium turnings (250 mg, 1.04 mmol) and iodine (61 mg, 0.048 mmol) were placed in a dry, nitrogen flushed 25 mL flask equipped with a magnetic stirrer, a thermometer and a septum. The flask was warmed to 40 °C for 5 min to partially vaporize the iodine. A continuous stream of dry nitrogen was passed via a septum to avoid moisture. 10% of a 3-bromoanisole solution (1.805 g, 0.97 mmol in 1 mL of 2-MeTHF) was slowly added at room temperature. An exothermic reaction was observed and the temperature increased to 45 °C. The remainder of 3-bromoanisole solution was added in a dropwise fashion over 10 min maintaining the temperature at 45 °C. The Grignard reagent was generated as a pale yellow solution. The formation of the Grignard complex was confirmed by extracting an aliquot and diluting in methanol for GC-MS analysis. The Grignard complex was stirred for an additional 30 min at 25 °C and then cooled to 0 °C. A solution of 2-dimethylamino methyl cyclohexanone (1.151 g, 0.7 mmol, in 1 mL of 2-MeTHF) was added slowly maintaining the temperature below 5 °C. The reaction mixture was stirred at room temperature for 4 h and then poured into a beaker containing 1.5 g of NH4Cl in 30 mL of ice water. The mixture was extracted with ether (3 × 5 mL) and the combined organic layers were dried over Na2SO4 and concentrated in vacuo. The crude product was dissolved in 5 mL of toluene and cooled in an ice bath. The pH was adjusted to 0 by addition of 6 N HCl. Two layers were obtained with an aqueous layer containing the hydrochloride salt of tramadol. To the aqueous phase was added 10 mL of EtOAc and then NH4OH to reach pH 9. The aqueous layer was extracted with ether (3 × 5 mL). The combined organic layers were dried over Na2SO4 and concentrated in vacuo to give product 8 as a brown-colored oil (1.4 g, 78%)3 with a diastereomeric ratio of 83
:
17 (cis
:
trans) as determined by LC-MS.Grignard reaction of 3-methyl thiophene with DMF
A solution of 3-methyl thiophene (1.47 g, 15 mmol) in 6 mL of THF was charged into a 50 mL round bottom flask equipped with a thermometer and a stirrer under a slow stream of nitrogen. To the solution was added 2,2,6,6-tetramethylpiperidine (0.252 mL, 1.5 mmol) in a single portion followed by addition of iso-propylmagnesium chloride in THF (2.00 M, 6.33 mL, 12.70 mmol) over 10 min keeping the temperature below 30 °C. The mixture was heated at reflux for 24 h. After cooling the Mg–thiophene complex to 0 °C, DMF (1.6 mL, 21 mmol) in 7.2 mL of THF was added over 40 min. After being stirred at 0 °C for a further 30 min, the reaction mixture was quenched with 5.6 mL of water followed by addition of 6 N HCl to adjust the pH to 2. The aqueous layer was extracted with methyl tert-butyl ether (MTBE) and the crude compound purified by flash chromatography over silica gel eluting with 7
:
3 hexane–MTBE to give product 10 as a pale yellow colored oil (1.7 g, 67%).27Grignard reaction of 3-methyl thiophene with benzaldehyde
A solution of 3-methyl thiophene (1.47 g, 15 mmol) in 6 mL of THF was charged into a 50 mL round flask equipped with a thermometer and a stirrer under a slow stream of nitrogen. To the solution was added 2,2,6,6-tetramethylpiperidine (0.252 mL, 1.5 mmol) in a single portion followed by the addition of iso-propylmagnesium chloride in THF (2.0 M, 6.33 mL, 12.70 mmol) over 10 min keeping the temperature under 30 °C. The mixture was heated at reflux for 24 h. After cooling the Mg–thiophene complex to 0 °C, benzaldehyde (2.1 mL, 21 mmol) in 7.2 mL of THF was added over 40 min. After being stirred at 0 °C for another 20 h, the reaction mixture was quenched with 5.6 mL of water followed by addition of 6 N HCl to adjust the pH to 2. The aqueous layer was extracted with methyl tert-butyl ether (MTBE) and the crude compound was purified by flash chromatography over silica gel eluting with 7
:
3 hexane–MTBE to give product 11 as a brown colored oil (3.4 g, 80%).274 Conclusions
In this study, we performed the systematic study focusing on the solvent effect on a series of Grignard-type reactions. In addition, we have looked at both the effects of the initiators employed, and the reaction conditions in these systems. In the reactions that we have studied, 2-MeTHF has consistently either out-performed or performed in an equivalent manner to typical reaction solvents such as Et2O and THF. Given this, 2-MeTHF should be the solvent of choice for such reactions from both safety and environmental standpoints. In addition, we have demonstrated that the judicious selection of the initiator including its preparation (such as DIBAL-H in THF) enables solvents such as CPME, DEM and diglyme to be employed in Grignard couplings. Finally, hybrid solvent systems consisting of 2-MeTHF with toluene, CPME and diglyme have the potential to broaden the scope of solvent systems for the Grignard reaction. Utilization of such systems could have a dramatic effect in terms of facilitating the work-up, and isolation of the desired products from these reactions. Based on the data presented, organometallic reagent suppliers are encouraged to collect data to support manufacture and storage of Grignard reagents in greener solvents such as 2-MeTHF.Acknowledgements
WZ thanks ACS Green Chemistry Institute Pharmaceutical Roundtable for research grant support.Notes and references
- A. Kadam, M. Nguyen, W. Zhang, M. Kopach, P. Richardson, F. Gallou and Z.-K. Wan, The 16th Green Chemistry & Engineering Conference, Washington DC, 2012.
- V. Grignard, Ann. Chim., 1901, 24, 433 CAS.
- K. Flick and E. Frankus, U.S. Patent, 3,652,589, 1972 Search PubMed.
- J. Pesti, C.-K. Chen, L. Spangler, A. J. DelMonte, S. Benoit, D. Berglund, J. Bien, P. Brodfuehrer, Y. Chan, E. Corbett, C. Costello, P. DeMena, R. P. Discordia, W. Doubleday, Z. N. Gao, J. Gingras, O. Haas, D. Kacsur, C. J. Lai, S. Leung, M. Miller, J. Muslehiddinoglu, N. Nguyen, J. Qiu, M. Olzog, E. Reiff, D. Thoraval, M. Totleben, D. Vanyo, P. Vemishetti, J. Wasylak and C. K. Wei, Org. Process Res. Dev., 2009, 13, 716 CrossRef CAS.
- P. J. Harrington and E. Lodewijk, Org. Process Res. Dev., 1997, 1, 72 CrossRef CAS.
- Syntex Corp., US Patent, 4,144, 397, 1979 Search PubMed.
- A. P. G. Holton, US Patent, 4,515,811, 1985 Search PubMed.
- K. M. G. Brands, S. W. Krska, T. Rosner, K. M. Conrad, E. G. Corley, M. Kaba, R. D. Larsen, R. A. Reamer, Y. Sun and F.-R. Tsay, Org. Process Res. Dev., 2006, 10, 109 CrossRef CAS.
-
(a) H. Schickaneder, R. Loser and H. Grill, US Patent, 5, 047, 431, 1991 Search PubMed;
(b) P. C. Ruenitz, J. R. Bagley and C. M. Mockler, J. Med. Chem., 1982, 25, 1056 CrossRef CAS;
(c) A. B. Foster, M. Jatman, O. T. Leung, R. McCague, G. Leclercq and N. Devleeschouwer, J. Med. Chem., 1985, 28, 1491 CrossRef CAS.
- E. Riva, S. Gagliardib, M. Martinelli, D. Vigo, D. Passarella and A. Rencurosib, Tetrahedron, 2010, 66, 3242 CrossRef CAS.
- U. Tilstam and H. Weinmann, Org. Process Res. Dev., 2002, 6, 906 CrossRef CAS.
- C. Jiminez-Gonzales, C. S. Ponder, Q. B. Broxterman and J. B. Manley, Org. Process Res. Dev., 2011, 15, 912 CrossRef.
- For reviews on green solvents, see:
(a) C. Estévez, in Sustainable Solutions for Modern Economies, ed. R. Höfer, RSC Green Chemistry Series, Cambridge, 2009, vol. 4, pp. 407–424 Search PubMed;
(b) C. Capello, U. Fischer and K. Hungerbühler, Green Chem., 2007, 9, 927 RSC;
(c) S. W. Breeden, J. H. Clark, D. J. Macquarrie and J. Sherwood, in Green Techniques for Organic Synthesis and Medicinal Chemistry, ed. W. Zhang and B. W. Cue, Wiley, Chichester, 2012, pp. 243–262 Search PubMed.
- D. Aycock, Org. Process Res. Dev., 2007, 11, 156 CrossRef CAS.
- M. l. Clarke and E. J. Milton, Green Chem., 2010, 12, 381 RSC.
- For sucrose-based 2-MeTHF, see:
(a) S. Frenzel, S. Peters, T. Rose and M. Kunz, in Sustainable Solutions for Modern Economies, ed. R. Höfer, RSC Green Chemistry Series, Cambridge, 2009, vol. 4, pp. 264–299 Search PubMed;
(b) B. Comanita, 2-MeTHF for Greener Processes, http://www.pennakem.com/pdfs/MeTHFPennGreenChemistry.pdf.
- V. Pace, P. Hoyos, L. Castoldi, P. D. de Maria and A. R. Alcantara, ChemSusChem, 2012, 5, 1369 CrossRef CAS.
-
(a) K. Watanabe, N. Yamagiwa and Y. Torisawa, Org. Process Res. Dev., 2007, 11, 251 CrossRef CAS;
(b) K. Oshima, S. Kanemura, A. Kondoh and H. Yorimitsu, Synthesis, 2008, 2659 Search PubMed.
- J. P. Scott, V. Antonucci, J. Coleman, J. B. Ferry, N. Johnson, M. Mathe and J. Xu, Org. Process Res. Dev., 2011, 15, 939 CrossRef.
- M. T. Coleman, Chim. Oggi (Chem. Today), 2009, 27, 19 Search PubMed.
-
(a) A. Pohland, U.S Patent, 2,728,779, 1955, Eli Lilly and Company Search PubMed;
(b) A. Pohland, L. R. Peters and H. R. Sullivan, J. Org. Chem., 1963, 28, 2483 CrossRef CAS.
- Eli Lilly and Company, WO, 2005/20975 A2 Search PubMed.
- The Grignard solutions were analysed by HPLC after anhydrous methanol addition activity correlated to observed toluene. The unquenched Grignard solutions were also titrated by Knochel's method: P. Knochel and A. Krasovskiy, Synthesis, 2006, 890 CrossRef.
-
(a) D. Stoye, in Ullmann's Encyclopedia of Industrial Chemistry, 2000, onlinelibrary.wiley.com/doi/10.1002/14356007.a24_437/pdf Search PubMed;
(b) H. Zong, H. Huang, J. Liu, G. Bian and L. Song, J. Org. Chem., 2012, 77, 4645 CrossRef CAS.
- A. Krasovskiy and P. Knochel, Angew. Chem., Int. Ed., 2004, 43, 3333 CrossRef CAS.
- M. Finkam and B. Aktereis, U.S. Patent, 6,469,213, 2002 Search PubMed.
- S. M. Asselin, M. M. Bio, N. F. Langille and K. Y. Ngai, Org. Process Res. Dev., 2010, 14, 1427 CrossRef CAS.
- M. L. Bouveault, Bull. Soc. Chim. Fr., 1904, 31, 1306 Search PubMed.
- R. J. Kloetzing, A. Krasovskiy and P. Knochel, Chem.–Eur. J., 2007, 13, 215 CrossRef CAS.
-
(a) S. Coles, J. M. Mellor, A. H. El-Sagheer, E. M. Salem and R. N. Metwallyc, Tetrahedron, 2000, 56, 10057 CrossRef CAS;
(b) E. Díez-Barra, A. D. Hoz, A. Sánchez-Migallón, P. Sánchez-Verdú, G. Bram, A. Loupy, M. Pedoussaut and P. Pigeon, Synth. Commun., 1989, 19, 293 CrossRef;
(c) D. Abenhaim, G. Boireau, C. Bernardon, A. Deberly and C. Germain, Tetrahedron Lett., 1976, 17, 993 CrossRef;
(d) A. Khalaf, J. Indian Chem. Soc., 2010, 5, 595 Search PubMed.
-
(a) J. Hartsel, D. T. Craft, Q. H. Chen, M. Ma and P. R. Carlier, J. Org. Chem., 2012, 77, 3127 CrossRef CAS;
(b) M. Hatano, O. Ito, S. Suzuki and K. Ishihara, J. Org. Chem., 2010, 75, 5008 CrossRef CAS;
(c) C. A. Musser and H. Richey, J. Org. Chem., 2000, 65, 7750 CrossRef CAS.
Footnote |
† Electronic supplementary information (ESI) available. See DOI: 10.1039/c3gc40702k |
|
This journal is © The Royal Society of Chemistry 2013 |
Click here to see how this site uses Cookies. View our privacy policy here.