DOI:
10.1039/C3FO30243A
(Paper)
Food Funct., 2013,
4, 770-776
Glucose enhancement of event-related potentials associated with episodic memory and attention
Received
10th September 2012
, Accepted 11th March 2013
First published on 13th March 2013
Abstract
Previous studies have reported that increasing glycaemia by a glucose-containing drink enhances memory functioning. The aim of the present study was to extend this literature by examining the effects of glucose on episodic memory as well as attention processes, and to investigate associated event-related potential (ERP) markers. Fifteen minutes after treatment (25 g glucose or placebo drink), 35 participants performed an old/new recognition memory task and a Stroop colour naming task. Consistent with previous research, when controlling for glucose regulation, cognitive facilitation was observed behaviourally for verbal memory, but there was also a trend towards attentional facilitation. Furthermore, across both domains, it was the most demanding task conditions that exhibited glucose sensitivity. In support of the behavioural results, the analysis of ERPs across treatment groups revealed an enhanced left-parietal old/new effect related to recollection, and also suggested modulation of attentional processes. The results suggest that glucose may facilitate attention as well as memory.
Glucose is a key substrate of the mammalian central nervous system and plays an important role in healthy brain function. Importantly, the brain requires a constant supply of glucose for fuel and stores are limited.1 Experimental work with humans has demonstrated that acutely increasing glucose provision to the brain, by means of a glucose drink, modulates mental alertness and cognition.2–5 Although research has investigated the impact of increasing glucose availability, the precise cognitive abilities that are susceptible to glucose action remain unclear.
At the heart of previous research has been the notion that increasing blood glucose impacts on specific cognitive domains, and verbal memory appears to be particularly sensitive. The dominant theoretical position holds that the administration of glucose benefits tasks which result in high levels of hippocampal activity.5,6 The special status of the medial temporal lobes and hippocampus in glucose-induced memory facilitation may be related to increased acetylcholine release,7 to secondary changes in insulin levels stimulating glucose uptake in the brain, particularly the insulin receptor-rich hippocampus,8 or to increased peripheral glucose redressing the depletion of extracellular glucose levels following demanding memory processes.9 At the cognitive level, memory enhancement has been demonstrated in several populations including adolescents,10 young adults,11,12 older adults,13 and in individuals with Mild Cognitive Impairment14 and dementia.15 For example, Foster and colleagues11 tested young healthy participants on a number of measures of cognitive function, including verbal short- and long-term memory, after the consumption of either a glucose or placebo drink. Across the battery of tests, significant glucose facilitation effects were found only on measures of verbal episodic memory. This notion, that glucose effects are selective for verbal episodic memory, was consolidated in a recent meta-analysis comprising 104 individual effect sizes, which demonstrated much larger glucose facilitation effects for long-term episodic memory (d = 0.91) compared with other cognitive domains (e.g., attention d = 0.13; semantic memory d = 0.05; visual spatial processing d = 0.20).3
Although this ‘hippocampus hypothesis’ has been influential, mapping of the neuro-cognitive processes susceptible to glucose action has benefited from more recent neuroimaging work. For example, the event-related potential (ERP) component related to successful recollection has been labelled the left parietal old/new effect (LP) and comprises a positivity at 400–800 ms after presentation of ‘old’ versus ‘new’ items.16 Investigating the possible modulation of this component by glucose administration, Smith and colleagues17 found evidence for glucose facilitation in the form of an enhanced LP effect, consistent with earlier behavioural work. However, there is also evidence that activity related to attentional processes may exhibit glucose facilitation. Using a three-stimulus version of the classic ‘oddball’ task, Riby and colleagues investigated the possibility that glucose impacts on the neural correlates of memory updating (P3b component) and/or attention (P3a component).18 As expected, glucose moderated the amplitude and latency of the P3b ERP component related to memory, but glucose was also found to interact with attention (i.e., the P3a as well as the earlier P2 component19). Furthermore, in a functional magnetic resonance imaging (fMRI) study, Stone and colleagues examined the impact of 50 g glucose versus a sweetened matched placebo drink on performance of a verbal memory encoding task in schizophrenia patients.20 They showed significantly greater activation in the predicted left medial temporal lobe region after ingestion of glucose, but also observed a trend towards greater activation of prefrontal cortex. This fMRI evidence, along with the previously discussed ERP findings, highlight the importance of determining the extent to which glucose moderates memory as well as other cognitive processes and, in particular, attention.
The objectives of this study were as follows: (1) to establish the extent to which glucose facilitation is specific to verbal episodic memory function, specifically, whether or not attention may also exhibit a benefit; (2) to determine whether task difficulty plays a role in the glucose modulation of episodic memory; and (3) to assess the effects of glucose consumption on known neural correlates of episodic memory and attentional processing.
Methods
In order to determine the specificity of the glucose facilitation effect, performance of an old/new item recognition task21 and a Stroop colour naming task was assessed following consumption of either a glucose-containing drink or a placebo. Additionally, ERP data were collected to investigate the extent to which the associated components of interest, based on the previous literature, were modulated by glucose consumption. The ERPs of interest were the LP, as discussed above, and the central-frontal negativity (typically 350–500 ms post-stimulus, peaking at around 410–450 ms). The latter component has previously shown to be related to conflict monitoring during incongruent colour-word stimuli in the Stroop task, and is believed to be generated from anterior cingulate cortex.22–26
Participants
Thirty-nine young adults participated. The data of two participants were discarded due to equipment error and a further two due to insufficient available trials to create the ERP waveforms. The remaining 35 participants included 14 males and 21 females, aged 18–35 years (M = 22.17 years; SD = 5.97), who were randomly assigned to either the placebo or glucose condition (placebo n = 17, glucose n = 18). Five participants were left-handed (placebo = 2, glucose = 3). There were no differences between the treatment groups in mean age, baseline blood glucose level, or estimated IQ (National Adult Reading Test;27 all p > 0.05, see Table 1). No participants were diabetic. All participants were paid a small honorarium.
Table 1 Means (with standard deviations) for demographic and baseline blood glucose variables across treatment condition
|
Age |
NART-Estimated IQ |
Baseline blood glucose concentration |
Placebo |
22.94 (6.38) |
110.00 (3.98) |
5.66 (0.51) |
Glucose |
21.44 (5.64) |
107.11 (5.71) |
5.79 (1.01) |
Tasks
Episodic memory (item recognition) task.
A short practice phase was followed by two experimental study-test cycles. In the first study phase, participants were presented with 72 items, 36 words and 36 line drawings, in a randomised order. Each stimulus was presented for 750 ms followed by a fixation character (+) for 500 ms. In the test phase that followed, 108 words were presented, 72 of which depicted the items presented in the ‘old’ words and pictures that had previously been studied, and 36 of which were ‘new’ items (i.e., not previously studied). Each test trial comprised a blank screen (500 ms) followed by the stimulus (500 ms) and a blank screen (1500 ms). Participants responded by pressing the ‘old’ key if they thought the item had been viewed in the study phase and the ‘new’ key if they thought it had not been viewed. The second and final study-test cycle then followed in the same way. The resulting data took the form of item recognition accuracy related to old items (initially presented either as words or pictures) and new items (never previously presented). Across the two blocks there were a total of 216 test trials, with 72 trials for each of the three stimulus types (old words, old pictures, new items).
Attention (Stroop) task.
After a practice session, four experimental blocks were administered in a randomised order. Two of the experimental blocks involved presenting the words ‘red’, ‘green’, ‘blue’, and ‘yellow’ in either red, green, blue or yellow font colour. The font colour was therefore either ‘congruent’ or ‘incongruent’ with the presented word. These two blocks varied by task, with participants being asked either to read the word or name the font colour. The two remaining blocks comprised control conditions for each task version, in which participants either read the colour words presented in black font, or named the font colour of a row of ampersand symbols. There were 96 trials in each of the two experimental blocks (i.e., 48 trials for each of the congruent and incongruent conditions) and 48 trials in each control block. On each trial the stimulus appeared for 500 ms, followed by a blank screen for 2750 ms.
Procedure
Participants attended the lab on one occasion between 9 am and 3 pm and, after giving informed consent, completed a compliance questionnaire confirming that they had not consumed anything other than water in the preceding 2 hours. At this point a small blood sample was taken from the fingertip (via a standard pinprick device; Freestyle Freedom, Abbott Diabetes Care, Inc.) to measure baseline blood glucose level. The 62-channel electroencephalogram (EEG) cap was then applied. Approximately 40 minutes into the session participants were given either glucose (25 g) or placebo (37.5 mg saccharin) products (administered double-blind), ingested via a sugar-free orange squash drink (Robinsons, Britvic PLC). To control for sweetness and ‘mouthfeel’ the glucose drink comprised 30 ml squash diluted with 200 ml water, while the placebo drink comprised 45 ml squash diluted with 200 ml water (note that similar matching of drinks has been successfully used before28). A delay of 15 minutes was ensured before the first task was administered and, during this delay period, the NART and a nutrition questionnaire were both completed (with the latter to assess eating habits as part of an ongoing project). The episodic memory and attention tasks were then administered, in a counter-balanced order, via E-Prime (Psychology Software Tools, Inc.). Blood glucose level was monitored immediately following completion of each task (at approximately 40 and 65 minutes post-drink). Fig. 1 displays a timeline of the session.
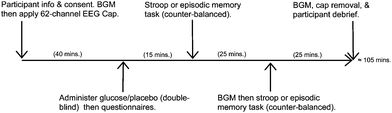 |
| Fig. 1 Timeline of session. NB: BGM = blood glucose monitoring. | |
EEG acquisition
A 62-channel EEG cap featuring sintered silver–silver chloride electrodes (Compumedics Neuromedical Supplies) arranged in the extended international 10–20 system was used.29 The montage included 8 midline sites (FPZ; FZ; FCZ; CZ; CPZ; PZ; POZ; OZ), 27 sites over the left hemisphere (FP1; AF3; F1; F3; F5; F7; FC1; FC3; FC5; FT7; C1; C3; C5; T7; CP1; CP3; CP5; TP7; P1; P3; P5; P7; PO3; PO5; PO7 O1; CB1), and 27 sites over the right hemisphere (FP2; AF4; F1; F4; F6; F8; FC2; FC4; FC6; FT8; C2; C4; C6; T8; CP2; CP4; CP6; TP8; P2; P4; P6; P8; PO4; PO6; PO8 O2; CB2). Two further electrodes were applied to the left and right mastoids to form a linked reference for each electrode. The EEG signals were digitised at a rate of 1000 per second. To monitor eye movements and blinking, two electrodes were placed above and below the left eye to record the vertical-electrooculogram, while two electrodes were placed on each temple to record the horizontal-electrooculogram. Inter-electrode impedance levels were kept below 5 kΩ. The EEGs were obtained using Synamps2 amplifiers and Acquire 4.3 software, while offline analysis was carried out using Edit 4.3 (Compumedics Neuroscan). Epochs were created for each task (episodic memory = −200 to 1500 ms; Stroop −200 to 1200 ms) which were baseline corrected to −100 ms pre-stimulus. To remove disturbances in the waveforms due to eye movements, blinking, and other anomalies, automatic occular artefact reduction was carried out followed by automatic artefact rejection for any waveform reaching ±75 μV. Manual inspection was then carried out to ensure no artefacts remained. The data were band-pass-filtered at 0.5–30 Hz and smoothed over 21 points. A minimum of 16 correct trials were then used to create the ERPs. Based on previous research, ERP analyses were focused on the P3 electrode (500–800 ms time window) during the episodic memory task, and FCz electrode (350–500 ms) during the attention task.
Statistical analysis
The data were analysed using the software package SPSS Statistics 19 (IBM). Analysis of Variance (ANOVA) was used with the accepted significance level of 0.05. The greenhouse-geisser method was used to correct for any statistical deviations that occurred. As individual differences in glucose regulation can influence the enhancing properties of glucose,2 the behavioural analyses included a measure of regulation (i.e., mid-session blood glucose minus baseline28) as a covariate, where this was found to interact with the results. All reported pairwise comparisons employed Bonferroni correction.
Results
Glycaemic response
Blood glucose concentration was constant across the session in the placebo condition, while the expected increase from baseline to mid-session in the glucose condition was observed. From mid-session, levels in the glucose condition began to return to baseline (see Fig. 2). To confirm that blood glucose levels were manipulated between treatment groups, an ANOVA was carried out on these data across time (baseline, 40 min post-drink, 65 min post-drink) and between the two groups (placebo, glucose). The analysis confirmed that the glucose manipulation had been successful, revealing main effects of time, F(1.7,54.6) = 6.10, p = 0.006, ηp2 = 0.16, treatment condition, F(1,33) = 15.75, p < 0.001, ηp2 = 0.32, and the interaction, F(1.7,54.6) = 9.03, p = 0.001, ηp2 = 0.22.
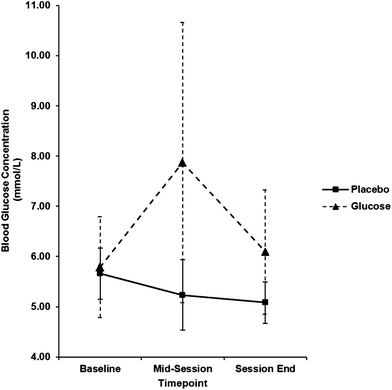 |
| Fig. 2 Blood glucose measurements across the session for each experimental group. Error bars represent standard deviation. | |
Behavioural data
Episodic memory.
Memory retrieval accuracy was analysed in a 2 (treatment group: placebo, glucose) × 3 (item type: old words, old pictures, new items) ANCOVA. This revealed a main effect of item type, F(2,64) = 14.46, MSE = 145.58 p < 0.001, ηp2 = 0.31. Pairwise comparisons revealed that accuracy was better for both new items (M = 77.11, SE = 2.31) and old pictures (M = 70.35, SE = 2.72) compared with old words (M = 56.12, SE = 2.03; both p < 0.001). An interaction between treatment group and item type highlighted that glucose facilitation occurred for the more difficult condition of words only, and that, conversely, performance with new items may suffer due to glucose administration (see Table 2), F(2,64) = 4.14, MSE = 145.58, p = 0.02, ηp2 = 0.12. Notably, an interaction was found between stimulus type and the covariate of glucose regulation, F(2,64) = 7.01, MSE = 145.58, p = 0.002, ηp2 = 0.18, further highlighting the involvement of glucose in performance of this task.
Table 2 Behavioural measures of episodic memory and attention across treatment group, (adjusted to control for glucose regulation)a
|
Episodic memory (old/new recognition task) |
Attention (Stroop colour naming task) |
Old words |
Old pictures |
New items |
Control |
Congruent |
Incongruent |
N.B. The dependent measures were accuracy in the episodic memory task, and response time (ms) for the attention task.
|
Placebo |
51.25 (3.19) |
71.62 (4.29) |
81.99 (3.64) |
779.50 (49.49) |
866.78 (61.94) |
1025.83 (74.47) |
Glucose |
60.98 (3.09) |
69.08 (4.15) |
72.24 (3.52) |
801.00 (46.21) |
814.60 (57.84) |
902.85 (69.54) |
Stroop task.
As is normally expected with the Stroop task, accuracy scores were generally high (Placebo: control M = 93.26, SD = 11.77, congruent M = 93.18, SD = 13.57, incongruent M = 75.47, SD = 35.07; glucose: control M = 93.67, SD = 11.42, congruent M = 94.17, SD = 12.08, incongruent M = 88.94, SD = 14.02). The most sensitive Stroop measure of response time (RT) during the colour naming task was analysed using a 2 (treatment group: placebo, glucose) × 3 (congruency: control, congruent, incongruent) ANOVA. This revealed a main effect of congruency, F(1.4,45.9) = 26.52, MSE = 14
123, p < 0.001, ηp2 = 0.45, with pairwise comparisons confirming that RT in the incongruent condition (M = 964 ms, SE = 46) was slower than RT in both the congruent (M = 841 ms, SE = 38) and control conditions (M = 791 ms, SE = 31; both p < 0.001). There was no reliable interaction between congruency and glucose condition, F(1.4,46.0) = 2.66, MSE = 14
123, p = 0.10, ηp2 = 0.08. Interestingly, however, although regulation did not result in any significant effects, when it was included as a covariate, the interaction between congruency and glucose condition trended towards significance, F(1.4,44.5) = 3.10, MSE = 14
294, p = 0.07, ηp2 = 0.09. Indeed, the pattern of adjusted means (i.e., taking regulation into account) do suggest specific glucose facilitation for the predicted, most difficult, incongruent condition. That is, RTs across treatment group are similar for control stimuli, only slightly quicker in the glucose condition for congruent stimuli, but substantially quicker in the glucose condition for the incongruent stimuli (see Table 2).
Event-related potential data
Episodic memory.
The parietal memory ERP component was analysed using a 2 (treatment group: placebo, glucose) × 3 (item type: new, old words, old pictures) × 2 (hemisphere: left, right) ANCOVA. This revealed main effects of item type, F(1.5,46.5) = 12.77, MSE = 4.26, p < 0.001, ηp2 = 0.29, and hemisphere, F(1,32) = 4.03, MSE = 1.08, p = 0.05, ηp2 = 0.11. As would be predicted, the adjusted means demonstrated increased positivity in the left (M = 1.31, SE = 0.36) relative to the right hemisphere (M = 0.96, SE = 0.33). Furthermore, simple contrasts also demonstrated increased positivity for both old words (M = 1.47, SE = 0.44) and pictures (M = 1.43, SE = 0.38) relative to new items (M = 0.50, SE = 0.31; both p < 0.001). Critically, however, the analysis also revealed an interaction between item type and treatment group, F(2,64) = 5.07, MSE = 3.10, p = 0.009, ηp2 = 0.14. Across treatment group, the ERPs related to old pictures (placebo: M = 1.63, SE = 0.59; glucose: M = 1.23, SE = 0.57) were more similar than for old words (placebo: M = 0.96, SE = 0.69; glucose: M = 1.97, SE = 0.66) and new items (placebo: M = 1.10, SE = 0.49; glucose: M = −0.10, SE = 0.48). This reflects an enhanced parietal effect for the most difficult condition of words, when glucose has been ingested (see Fig. 3). The analysis also revealed an interaction between item type and hemisphere, F(2,64) = 4.40, MSE = 0.13, p = 0.02, ηp2 = 0.12; more positivity was apparent on the left than the right for the more easily recognised pictures (left: M = 1.73, SE = 0.41; right: M = 1.13, SE = 0.36), confirming the presence and location of the left parietal effect, while less differentiation existed for the words (left: M = 1.57, SE = 0.45; right: M = 1.37, SE = 0.44) and the new items (left: M = 0.63, SE = 0.34; right: M = 0.37, SE = 0.31). Finally, blood glucose regulation (the covariate) significantly interacted with the effect of item type, F(2,64) = 12.45, MSE = 3.10, p < 0.001, ηp2 = 0.28, further highlighting that the availability of glucose has differential impact, depending upon item type. All other effects were non-significant (all ps > 0.49).
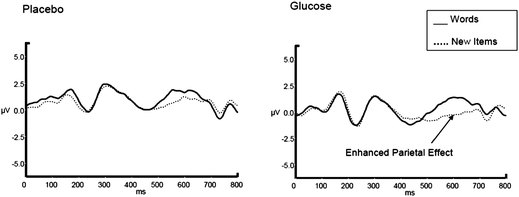 |
| Fig. 3 Grand average ERPs for old words versus new items across placebo and glucose conditions at selected P3 electrode. | |
Attention.
The Stroop ERPs were analysed in a 2 (treatment group: placebo, glucose) × 2 (congruency: congruent, incongruent) × 3 (site: frontal, frontal-central, central) ANOVA. A main effect of site demonstrated greater negativity towards the front of the scalp, F(1.3,41.7) = 56.61, MSE = 4.23, p < 0.001, ηp2 = 0.64, with contrasts confirming lower amplitude from the frontal site compared with the frontal-central site (p < 0.001), and from the frontal-central site compared with the central site (p < 0.001; Fz: M = 1.14, SE = 0.33; FCz: M = 2.52, SE = 0.47; Cz: M = 4.17, SE = 0.54). The analysis also revealed an interaction between treatment group and congruency, F(1,32) = 8.04, MSE = 4.08, p = 0.008, ηp2 = 0.20. Fig. 4 illustrates that the expected frontal-central negativity effect was observed in the placebo condition (congruent: M = 3.51, SE = 0.63; incongruent: M = 3.08, SE = 0.67). In contrast, in the glucose condition, a more negative-going ERP was observed for congruent compared to incongruent stimuli (congruent: M = 1.34, SE = 0.59; incongruent: M = 2.52, SE = 0.63). From Fig. 4, it can be seen that the mean amplitudes of ERPs across treatment group appear lower with glucose than in the placebo condition. However, this was only reliably so for the congruent condition, t(33) = 2.55, p = 0.02. All other effects were non-significant (all p > 0.12). All observations are listed in Table 3.
Table 3 List of the main observations with statistical values. * denotes blood glucose regulation entered as a covariate
Observation |
Statistical values |
Episodic memory – behavioural (accuracy)
|
*Treatmentplacebo,glucose × item typeold words,old pictures,new items |
Item type |
F = 14.46, p < 0.001, ηp2 = 0.31 |
Treatment × item type |
F = 4.14, p = 0.02, ηp2 = 0.12 |
Item type × regulation |
F = 7.01, p = 0.002, ηp2 = 0.18 |
|
Episodic memory – ERPs
|
*Treatmentplacebo,glucose × item typeold words,old pictures,new items × hemisphereleft,right |
Item type |
F = 12.77, p < 0.001, ηp2 = 0.29 |
Hemisphere |
F = 4.03, p = 0.05, ηp2 = 0.11 |
Item type × treatment |
F = 5.07, p = 0.009, ηp2 = 0.14 |
Item type × hemisphere |
F = 4.40, p = 0.02, ηp2 = 0.12 |
Item type × regulation |
F = 12.45, p < 0.001, ηp2 = 0.28 |
|
Stroop task – behavioural (RT)
|
Treatmentplacebo,glucose × congruencycontrol,congruent,incongruent |
Congruency |
F = 26.52, p < 0.001, ηp2 = 0.45 |
Treatment × congruency |
F = 2.66, p = 0.10, ηp2 = 0.08 |
*Treatment × congruency |
F = 3.10, p = 0.07, ηp2 = 0.09 |
|
Stroop task – ERPs
|
Treatmentplacebo,glucose × congruencycongruent,incongruent × sitefrontal,frontal-central,central |
Site |
F = 56.61, p < 0.001, ηp2 = 0.64 |
Treatment × congruency |
F = 8.04, p = 0.008, ηp2 = 0.20 |
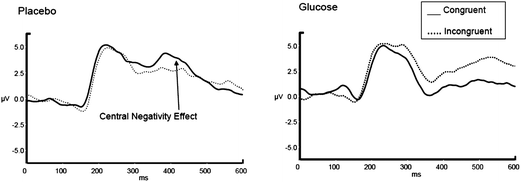 |
| Fig. 4 Grand average ERPs for congruent versus incongruent items during the Stroop colour naming task, across placebo and glucose conditions at selected FCz electrode. | |
Discussion
The purpose of the present study was to determine whether a 25 g dose of glucose would enhance performance of episodic memory and attention tasks, and whether glucose would modulate related ERP markers. Although the ingestion of glucose has been shown to impact reliably on tasks associated with high levels of hippocampal activity, imaging work from our lab (ERPs18) and elsewhere (fMRI20) have indicated that frontal lobe mechanisms may also be susceptible to increased glucose availability.
Consistent with the ‘hippocampus hypothesis’,5 when controlling for glucose regulation, accuracy during the episodic memory recognition task increased following glucose ingestion. This finding also supports earlier investigations directly comparing episodic retrieval of verbal compared to visuo-spatial information, with the presently observed benefit specific to items initially encoded as words rather than pictures.11 Using accuracy in the placebo condition as evidence of task difficulty, memory for words was more demanding than pictures. Thus, in the more difficult word condition, additional glucose resource was beneficial. This supports previous claims that task difficulty is a critical variable, for example in the context of working memory tasks (serial subtraction30), and episodic memory tasks performed under single- versus dual-task conditions.31 Elsewhere, it has been found that difficulty manipulations have not given rise to increased facilitation during memory retrieval32 and therefore a systematic investigation of task difficulty, stimulus, and task type is warranted. An interesting observation, and possibly another avenue for further inquiry, is the apparent difference across treatment for the correct rejection of new words. The correct rejection of new items during memory paradigms largely relies on the familiarity of the material rather than rich episodic information (recollection). The lack of glucose induced memory enhancement for correct rejections is consistent with earlier work examining the distinction between recollection and familiarity processes.6 Importantly, the implications for real word memory is highlighted by similar studies using other substance (e.g., caffeine) that have demonstrated more accurate memories accompanied by more false memories using the Deese–Roediger–McDermott (DRM) paradigm.33
More equivocal are the effects of glucose ingestion on Stroop task performance; a classic measure of frontal-executive function, more specifically, conflict detection, monitoring, and resolution. Potentially supportive of the ‘task difficulty’ hypothesis, a non-significant trend for glucose modulation of Stroop performance was observed, again when controlling for regulation. Although we need to be cautious when interpreting numerical differences (placebo = 1026 ms; glucose = 903 ms), the enhancement may take place under the most demanding task condition, that is, during the colour naming task when the text colour and the word are incongruent. On the basis of the behavioural data, then, we may speculate that cognitive performance is not restricted to episodic memory tasks, and may in fact be observed across multiple domains. Across both episodic memory and attentional performance, however, an important issue to be considered is how the behavioural glucose effects are moderated by individual differences in a person's ability to effectively use the additional glucose resource provided. “The full potential of glucose as a memory enhancer may only be realised after taking into account individual differences in glucose regulation…” (4, p. 83). Indeed, the optimal dose required to produce facilitation needs to take into account varying levels of glucose regulation efficiency.34
In addition to the behavioural data, however, the present study was aimed at investigating known ERP components related to episodic memory and attentional function. Recollection has consistently been found to elicit an ERP component called the left-parietal old/new effect (LP). As expected, verbal episodic memory performance was facilitated by glucose, and is indexed by the enhanced LP effect illustrated in Fig. 3. One caveat is that, due to the anterograde glucose administration procedure presently employed, however, it is impossible to assess whether specifically encoding, consolidation, or retrieval is facilitated during task performance. Although anterograde procedures tend to be employed in the literature, Sünram-Lea and colleagues demonstrated glucose memory facilitation when treatment was given before or after the learning phase of an episodic memory task, suggesting enhancement at retrieval.35 This is clearly an important area for future investigation.
As previous work demonstrated a frontal-central negativity for incongruent compared with congruent stimuli 300–500 ms post-stimulus in the Stroop task,22–26 we sought to investigate whether or not this ERP component would be modulated by glucose ingestion. In terms of the functional significance, this component is thought to index executive processes and, in particular, conflict monitoring by anterior cingulate cortex. Considering Fig. 4, in the placebo condition it can be seen that we have replicated this work with a negative-going ERP for incongruent compared to congruent trials in the 300–500 ms time window at the FCz electrode. While there is clear modulation of this ERP component in response to glucose ingestion, the functional meaning of the sustained positivity for incongruent compared to congruent trials with glucose ingestion is less clear. Previous ERP research has demonstrated the presence of a further component during the Stroop task, namely a later positivity for incongruent versus congruent trials over the fronto-central area, 450–550 ms post-stimulus, which may be related to ACC implementing cognitive control.24 In our data it may be the case that glucose influences the implementation of cognitive control, particularly as the behavioural data exhibited a trend towards faster response times with glucose; however, further work, which specifically targets this component, is clearly justified.
In summary, we have demonstrated that, consistent with earlier behavioural work, glucose enhances the neuro-cognitive processes related to verbal episodic memory (i.e., the left parietal effect related to recollection). Importantly, consistent with the data from the episodic memory task, there was limited evidence of enhancement within the attentional task as well, in the most difficult condition. The Stroop ERPs also exhibited modulation, but further research will be required to investigate the late positivity observed for glucose ERPs, and to assess the reliability of glucose modulation of behaviour.
Acknowledgements
This work was supported by a one year Research and Knowledge Transfer Grant awarded to Dr Riby from Glasgow Caledonian University. We thank Jennifer McLaughlin for her assistance in the drinks procedure.
References
-
V. Mark and F. G. Rose, Hypoglycaemia, Blackwell Scientific, Oxford, 1981 Search PubMed.
- C. Messier, Eur. J. Pharmacol., 2004, 490, 33–57 CrossRef CAS.
- L. M. Riby, Brain Impair., 2004, 5, 145–165 CrossRef.
-
L. M. Riby and D. M. Riby, in Ageing, Cognition, and Neuroscience, ed. S. Ballesteros, UNED, Varia, Madrid, 2006, pp. 79–92 Search PubMed.
- M. A. Smith, J. A. M. van Eekelen and J. K. Foster, Neurosci. Biobehav. Rev., 2011, 35, 770–783 CrossRef CAS.
- S.-I. Sünram-Lea, S. A. Dewhurst and J. K. Foster, Biol. Psychol., 2008, 77, 69–75 CrossRef.
- M. E. Ragozzino, K. E. Unick and P. E. Gold, Proc. Natl. Acad. Sci. U. S. A., 1996, 9, 4693–4698 CrossRef.
- S. Craft, J. Newcomer, S. Kanne, S. Dagogo-Jack, P. Cryer, Y. Sheline, J. Luby, A. Dagogo-Jack and A. Alderson, Neurobiol. Aging, 1996, 17, 123–130 CrossRef CAS.
- E. C. McNay and P. E. Gold, J. Gerontol., Ser. A, 2001, 56, B66–B71 CrossRef CAS.
- M. A. Smith and J. K. Foster, Biol. Psychol., 2008, 79, 209–215 CrossRef.
- J. K. Foster, P. G. Lidder and S.-I. Sünram, Psychopharmacology, 1998, 137, 259–270 CrossRef CAS.
- L. M. Riby, A. S. Law, J. Mclaughlin and J. Murray, Nutr. Res., 2011, 31, 370–377 CrossRef CAS.
- L. M. Riby, A. Meikle and C. Glover, Age Ageing, 2004, 33, 483–487 CrossRef.
- L. M. Riby, A. Marriott, R. Bullock, J. Hancock, J. Smallwood and J. McLaughlin, Eur. J. Clin. Nutr., 2009, 63, 566–571 CrossRef CAS.
- C. A. Manning, M. E. Ragozzino and P. E. Gold, Neurobiol. Aging, 1993, 14, 523–528 CrossRef CAS.
- M. D. Rugg and T. Curran, Trends Cognit. Sci., 2007, 11, 251–257 CrossRef.
- M. A. Smith, L. M. Riby, S.-I. Sünram-Lea, J. A. van Eekelen and J. K. Foster, Psychopharmacology, 2009, 205, 11–20 CrossRef CAS.
- L. M. Riby, S.-I. Sünram-Lea, C. Graham, J. Foster, T. Cooper, C. Moodie and V. P. Gunn, J. Psychopharmacol., 2008, 22, 486–492 CrossRef CAS.
- E. Barron, L. M. Riby, J. Greer and J. Smallwood, Psychol. Neurosci., 2011, 22, 596–601 Search PubMed.
- W. S. Stone, H. W. Thermenos, S. I. Tarbox, R. A. Poldrack and L. J. Seidman, Neurobiol. Learn. Mem., 2005, 83, 54–64 CrossRef CAS.
- L. M. Riby, J. Smallwood and V. Gunn, Psychol. Rep., 2008, 102, 805–818 CrossRef.
- S. Hanslmayr, B. Pastötter, K. Bäuml, S. Gruber, M. Wimber and W. Klimesch, J. Cognit. Neurosci., 2008, 20, 215–225 CrossRef.
- M. Liotti, M. G. Woldorff, R. Perez and H. S. Mayberg, Neuropsychologia, 2000, 38, 701–711 CrossRef CAS.
- J. Markela-Lerenc, N. Ille, S. Kaiser, P. Fiedler, C. Mundt and M. Weisbrod, Cognit. Brain Res., 2004, 18, 278–287 CrossRef.
- C. M. Tillman and S. Wiens, Psychophysiology, 2011, 48, 1405–1411 CrossRef.
- R. West, Neuropsychologia, 2003, 41, 1122–1135 CrossRef.
-
H. E. Nelson, The National Adult Reading Test (NART): test manual, NFER Nelson, Windsor, 1982 Search PubMed.
- A. Meikle, L. M. Riby and B. Stollery, Hum. Psychopharmacol. Clin. Exp., 2004, 19, 523–535 CrossRef CAS.
- H. H. Jasper, Electroencephalogr. Clin. Neurophysiol., 1958, 10, 371–373 Search PubMed.
- D. O. Kennedy and A. B. Scholey, Psychopharmacology, 2000, 149, 63–71 CrossRef CAS.
- S.-I. Sünram-Lea, J. K. Foster, P. Durlach and C. Perez, Psychopharmacology, 2002, 160, 387–397 CrossRef.
- L. M. Riby, H. McMurtrie, J. Smallwood, C. Ballantyne, A. Meikle and E. Smith, Br. J. Nutr., 2006, 95, 414–420 CrossRef CAS.
- S. Capek and R. K. Guenther, Psychol. Rep., 2009, 104, 787–795 CrossRef.
- C. A. Manning, M. W. Parsons, E. Cotter and P. E. Gold, Psychobiology, 1997, 25, 103–108 CAS.
- S.-I. Sünram-Lea, J. K. Foster, P. Durlach and C. Perez, Behav. Brain Res., 2002, 134, 505–516 CrossRef.
Footnote |
† This work was conducted when Louise Brown was at Glasgow Caledonian University. |
|
This journal is © The Royal Society of Chemistry 2013 |
Click here to see how this site uses Cookies. View our privacy policy here.