DOI:
10.1039/C2RA21067C
(Communication)
RSC Adv., 2012,
2, 7666-7668
Highly sensitive fluorescence PET (photo-induced electron transfer) sensor for water based on anthracene–bisboronic acid ester†
Received
29th May 2012
, Accepted 27th June 2012
First published on 27th June 2012
Abstract
Anthracene–bisboronic acid ester was designed and developed as a highly sensitive fluorescence PET sensor for the detection of trace amounts of water in various solvents (polar, less polar, protic and aprotic solvents).
The detection and quantification of water in organic solvents and the atmosphere are of fundamental interest in analytical chemistry and are of major importance for the construction of environmental monitoring systems in industry. Organic fluorescent dyes have been developed as fluorescence sensors for the detection of trace amounts of water in organic solvents.1–5 Recently, Deng and Wang developed a fluorescent-conjugated polymer, poly(3-aminobenzoic acid) (PMBA), as a fluorescent water sensor.6 In most of these fluorescent water sensors, the fluorescent intensity decreases as the amount of water increases in organic solvents, and this feature makes it difficult to detect trace amounts of water. Additionally, the detection and quantification of water based on fluorescence quenching systems depend strongly on the kind of solvent (polar, less polar, protic and aprotic solvents). Thus, the development of highly sensitive fluorescent water sensors based on a new detection principle is required for improving the detection limit of water and extending the range of application to various solvents.
An anthracene–boronic acid system7 was developed by the Shinkai group as a fluorescent PET (photo-induced electron transfer)8 sensor for saccharides. Their anthracene–boronic acid system inspired us to conceive the anthracene–boronic acid ester OM-1 as a new fluorescent PET sensor for water (Scheme 1a).9 In OM-1, boronic ester enhances the Lewis acidity of boron atoms and the solubility of the sensor dye in organic solvents.10–12 The addition of water to organic solvents containing OM-1 causes efficient formation of the fluorescent ionic structure of OM-1a by hydrolysis, resulting in the suppression of PET (occurrence of fluorescence) due to protonation of the amino group. The detection limit (DL) and quantitation limit (QL) were as high as 0.04 and 0.1 wt%, respectively, for both acetonitrile and ethanol (Table 1). However, the DL and QL in less polar organic solvents, such as 1,4-dioxane and THF, were lower than those in polar organic solvents.
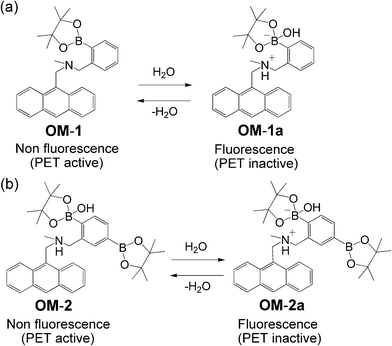 |
| Scheme 1 Proposed mechanisms of fluorescent PET sensors (a) OM-1 and (b) OM-2 for the detection of water in organic solvents. | |
Table 1 DL and QL of OM-2 and of previously reported fluorescent water sensors for water determination in various organic solvents
Sensor |
Solvent |
m
s
|
DL |
QL |
OM-19 |
1,4-Dioxane |
14.0 |
0.2 wt% |
0.7 wt% |
THF |
19.7 |
0.2 wt% |
0.5 wt% |
Acetonitrile |
67.6 |
0.04 wt% |
0.1 wt% |
Ethanol |
106.5 |
0.04 wt% |
0.1 wt% |
OM-2 |
1,4-Dioxane |
183.4 |
0.018 wt% |
0.054 wt% |
THF |
237.4 |
0.014 wt% |
0.042 wt% |
Acetonitrile |
250.5 |
0.013 wt% |
0.040 wt% |
Ethanol |
487.2 |
0.007 wt% |
0.021 wt% |
Ref. 13b
|
Acetonitrile |
34.7 |
0.1 wt% |
0.3 wt% |
Ethanol |
35.3 |
0.1 wt% |
0.3 wt% |
Ref. 2
|
Acetone |
−67.59 |
0.006 wt% |
No data |
Acetonitrile |
−78.91 |
0.002 wt% |
No data |
Ethanol |
−53.83 |
0.008 wt% |
No data |
Ref. 3
|
1,4-Dioxane |
−40.23 |
0.008 wt% |
No data |
Acetonitrile |
−52.10 |
0.006 wt% |
No data |
Ethanol |
−22.42 |
0.015 wt% |
No data |
Ref. 6
|
DMF |
−722.3 |
0.008 wt% |
0.03 wt% |
NMP |
−610.2 |
0.009 wt% |
0.03 wt% |
Acetonitrile |
−275.4 |
0.02 wt% |
0.07 wt% |
Ethanol |
−75.6 |
0.1 wt% |
0.3 wt% |
In order to further improve the sensing abilities of fluorescent water sensors based on PET, we designed and synthesized anthracene–bisboronic acid ester OM-2 (Scheme 1b). In the present study, we demonstrate the highly sensitive detection of trace amounts of water in various solvents based on the PET characteristics of the anthracene–bisboronic acid ester OM-2. We have previously proposed and developed an anthracene–amino acid system13 as a fluorescent water sensor based on PET. In the anthracene–amino acid system, the intramolecular proton transfer of the carboxyl proton to the amino group suppressed PET and thus fluorescence was enhanced by increasing the water content in various organic solvents. It is worth noting here that in various solvents, the DL and QL of an anthracene–amino diacid with two carboxyl groups were higher than those of an anthracene–amino acid with one carboxyl group, although the detailed reasoning is still under debate.13
Anthracene–bisboronic acid ester OM-2 was synthesized by the stepwise synthetic protocol illustrated in Scheme 2. Compound 1 was prepared from 2,5-dibromotoluene with bis(pinacolato)diboron by the Miyaura boration reaction.14 Benzylic bromination of 1 with 1,3-dibromo-5,5-dimethylhydantoin by the use of Lewis acid-catalyzed benzylic bromination by Yamamoto et al. was efficiently achieved to yield compound 2.15 The reaction of 9-(methylaminomethyl)anthracene with 2 in the presence of sodium hydride yielded the fluorescent water sensor OM-2.9,13
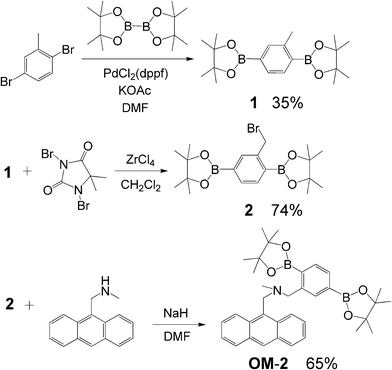 |
| Scheme 2 Synthesis of fluorescent PET sensor OM-2. | |
Absorption and fluorescent spectra of OM-2 were measured in 1,4-dioxane, THF, acetonitrile and ethanol that contained various concentrations of water. As shown in Fig. 1a and 1c for THF and acetonitrile, respectively, the absorption spectra of OM-2 in all four solvents underwent no appreciable changes in intensity and shape upon addition of water. In contrast, the corresponding fluorescence spectra exhibited significant changes in intensity with negligible changes in their spectral shapes (Fig. 1b and 1d). The changes in the fluorescent peak intensity are plotted in Fig. 2a–d against the water fractions of these four organic solvents. In the low water content region below 1.0 wt%, as shown in Fig. 2e, the fluorescent intensities increased dramatically and almost linearly with the increase in the water content for all four solvents, although the linear region of the plot for ethanol is narrow (0.03–0.24 wt%). The fluorescent intensities level off in the water content region greater than 1.0 wt% for all four solvents (Fig. 2a–d). It is particularly worth noting that the plots for 1,4-dioxane, THF and acetonitrile show straight lines passing through the origin, which are required for the practical use of the fluorescent water sensors. As in anthracene–boronic acid ester OM-1, these results show that the fluorescent enhancement of OM-2 with the increase in the water content can be attributed to suppression of PET due to the formation of OM-2a with a fluorescent ionic structure by hydrolysis of OM-2 (Scheme 1b). Consequently, the enhanced fluorescence of OM-2 in anhydrous ethanol may be attributed to the suppression of PET by the hydrogen bonding between the hydroxyl group of ethanol and the amino group of OM-2. To gain insight into the detection and quantification of water in organic solvents, the calibration equations for the determination of water in organic solvents were obtained from Fig. 2e as follows:
| 1,4-Dioxane: F = 183.4[H2O] + 7.8 (R2 = 0.981, [H2O] = 0.04–1.0 wt%) | (1) |
| THF: F = 237.4[H2O] + 4.2 (R2 = 0.982, [H2O] = 0.01–0.83 wt%) | (2) |
| Acetonitrile: F = 250.5[H2O] + 3.7 (R2 = 0.9958, [H2O] = 0.02–0.82 wt%) | (3) |
| Ethanol: F = 487.2[H2O] + 108.4 (R2 = 1, [H2O] = 0.03–0.24 wt%) | (4) |
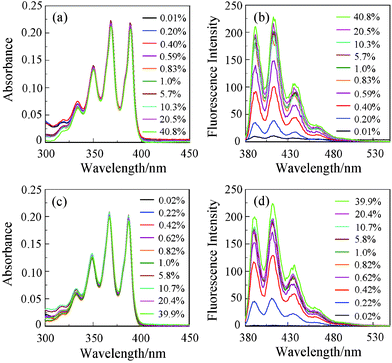 |
| Fig. 1 (a) Absorption and (b) fluorescence spectra (λex = 366 nm) of OM-2 (c = 2.0 × 10−5 M) in THF-containing water (0.01–40.8 wt%). (c) Absorption and (d) fluorescent spectra (λex = 366 nm) of OM-2 (c = 2.0 × 10−5 M) in acetonitrile-containing water (0.02–39.9 wt%). | |
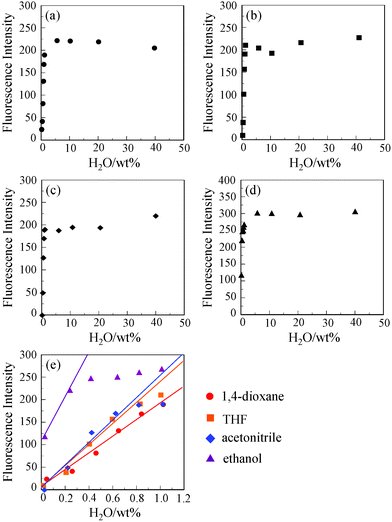 |
| Fig. 2 Fluorescence peak intensity of OM-2 at around 415 nm (λex = 366 nm) as a function of water content in (a) 1,4-dioxane, (b) THF, (c) acetonitrile and (d) ethanol. (e) Fluorescence peak intensity of OM-2 in 1,4-dioxane, THF, acetonitrile and ethanol in a low water-content region below 1.0 wt%. | |
eqn (1)–(4) show that the slope (ms) for OM-2 becomes steeper in the following order: 1,4-dioxane (ms = 183.4) < THF (ms = 237.4) < acetonitrile (ms = 250.5) < ethanol (ms = 487.2). The ms values for OM-2 in all four solvents are much larger than those of OM-1 and anthracene–amino diacid (ref. 13b in Table 1). Moreover, the ms values for OM-2 in acetonitrile, as a polar solvent, and ethanol, as a protic solvent, are larger than those of the reported fluorescent water sensors based on organic fluorescent dyes (ref. 2 and 3 in Table 1) and fluorescent-conjugated polymer (ref. 6 in Table 1).
We estimated DL and QL based on the following equations: DL = 3.3σ/msl and QL = 10σ/msl, where is the standard deviation of a blank sample and msl is the slope of the calibration curve in the region with a low water content (below 1.0 wt%), respectively (Table 1). The DL and QL of OM-2 are, respectively, 0.018 and 0.054 wt% for 1,4-dioxane, 0.014 and 0.042 wt% for THF, 0.013 and 0.040 wt% for acetonitrile and 0.007 and 0.021 wt% for ethanol, which are superior to those of OM-1 and anthracene–amino diacid (ref. 13b in Table 1) and are equivalent to or superior to those of the reported fluorescent water sensors based on fluorescent quenching systems (ref. 2, 3 and 6 in Table 1). These results show that anthracene–bisboronic acid ester OM-2 acts as a highly sensitive fluorescent PET sensor for the detection of trace amounts of water in polar, less polar, protic and aprotic solvents, although the response range (below 1.0 wt%) for this fluorescent enhancement system based on PET is narrow compared to the fluorescence quenching system (below 10 wt%) shown in Table 1.
In conclusion, we have designed and synthesized anthracene–bisboronic acid ester OM-2 to develop a highly sensitive fluorescence PET sensor for the detection of trace amounts of water. Fluorescent enhancement was observed with increasing water content in various solvents (polar, less polar, protic and aprotic solvents), which was attributed to the suppression of PET by the formation of fluorescent ionic structure OM-2a by hydrolysis. The DL and QL of OM-2 in various solvents are higher than those of anthracene–boronic acid ester OM-1 and are equivalent to or much higher than those of the reported fluorescent water sensors based on fluorescent quenching systems. Thus, we demonstrate that the fluorescent enhancement system based on PET is useful for the detection and quantification of trace amounts of water in various solvents.
Acknowledgements
This work was supported by Grants-in-Aid for Scientific Research (B) (23350097) (C) (24550225) from the Japan Society for the Promotion of Science (JSPS) and by a research grant from the Center for Collaborative Research & Community Cooperation, Hiroshima University.
References
- M. Bai and W. R. Seiz, Talanta, 1994, 41, 993 CrossRef CAS.
- C.-G. Niu, A.-L. Guan, G.-M. Zeng, Y.-G. Liu and Z.-W. Li, Anal. Chim. Acta, 2006, 577, 264 CrossRef CAS.
- C.-G. Niu, P.-Z. Qin, G.-M. Zeng, X.-Q. Gui and A.-L. Guan, Anal. Bioanal. Chem., 2007, 387, 1067 CrossRef CAS.
- D. Citterio, K. Minamihashi, Y. Kuniyoshi, H. Hisamoto, S. Sasaki and K. Suzuki, Anal. Chem., 2001, 73, 5339 CrossRef CAS.
- H. Mishra, V. Misra, M. S. Mehata, T. C. Pant and H. B. Tripathi, J. Phys. Chem. A, 2004, 108, 2346 CrossRef CAS.
- Q. Deng, Y. Li, J. Wu, Y. Liu, G. Fang, S. Wang and Y. Zhang, Chem. Commun., 2012, 48, 3009 RSC.
-
(a) T. D. James, K. R. A. S. Sandanayake and S. Shinkai, Nature, 1995, 374, 345 CrossRef CAS;
(b) T. D. James, K. R. A. S. Sandanayake and S. Shinkai, J. Chem. Soc., Chem. Commun., 1994, 477 RSC;
(c) T. D. James, K. R. A. S. Sandanayake, R. Iguchi and S. Shinkai, J. Am. Chem. Soc., 1995, 117, 8982 CrossRef CAS.
-
(a) A. P. de Silva and S. A. de Silva, J. Chem. Soc., Chem. Commun., 1986, 1709 RSC;
(b) A. P. de Silva, H. Q. N. Gunaratne, T. Gunnlaugsson, A. J. M. Huxley, C. P. McCoy, J. T. Rademacher and T. E. Rice, Chem. Rev., 1997, 97, 1515 CrossRef CAS;
(c) A. P. de Silva, T. P. Vance, M. E. S. West and G. D. Wright, Org. Biomol. Chem., 2008, 6, 2468 RSC;
(d) A. P. de Silva, T. S. Moody and G. D. Wright, Analyst, 2009, 134, 2385 RSC.
- Y. Ooyama, A. Matsugasako, K. Oka, T. Nagano, M. Sumomogi, K. Komaguchi, I. Imae and Y. Harima, Chem. Commun., 2011, 47, 4448 RSC.
-
A. Cotton, G. Wilkinson, Advanced Inorganic Chemistry: a Comprehensive Text, Wiley, New York, 19804th edn. Search PubMed.
-
(a) G. Springsteen, S. Deeter, X. Gao and B. Wang, Tetrahedron, 2002, 58, 5291 CrossRef CAS;
(b) G. Springsteen and B. Wang, Chem. Commun., 2001, 1608 RSC;
(c) S. Franzen, W. Ni and B. Wang, J. Phys. Chem. B, 2003, 107, 12942 CrossRef CAS;
(d) W. Ni, G. Kaur, G. Springsteen, B. Wang and S. Franzen, Bioorg. Chem., 2004, 32, 571 CrossRef CAS.
-
(a) J. P. Lorand and J. O. Edwards, J. Org. Chem., 1959, 24, 769 CrossRef CAS;
(b) T. Mizuno, T. Fukumatsu, M. Takeuchi and S. Shinkai, J. Chem. Soc., Perkin Trans. 1, 2000, 407 RSC;
(c) N. DiCesare and J. R. Lakowicz, J. Phys. Chem. A, 2001, 105, 6834 CrossRef CAS;
(d) N. DiCesare and J. R. Lakowicz, Org. Lett., 2001, 3, 3891 CrossRef CAS.
-
(a) Y. Ooyama, M. Sumomogi, T. Nagano, K. Kushimoto, K. Komaguchi, I. Imae and Y. Harima, Org. Biomol. Chem., 2011, 9, 1314 RSC;
(b) Y. Ooyama, A. Matsugasako, T. Nagano, K. Oka, K. Kushimoto, K. Komaguchi, I. Imae and Y. Harima, J. Photochem. Photobiol., A, 2011, 222, 52 CrossRef CAS.
- T. Ishiyama, M. Murata, M. Miyaura and A. Suzuki, J. Am. Chem. Soc., 1993, 115, 11018 CrossRef CAS.
- K. Shibatomi, Y. Zhang and H. Yamamoto, Chem. Asian J., 2008, 3, 1581 CrossRef CAS.
Footnote |
† Electronic supplementary information (ESI) available: Details of experimental procedures, preparation and characterization of compounds 1, 2, and OM-2. See DOI: 10.1039/c2ra21067c/ |
|
This journal is © The Royal Society of Chemistry 2012 |