DOI:
10.1039/C2RA00968D
(Paper)
RSC Adv., 2012,
2, 3691-3699
Salts of 1-(chloromethyl)-1,1-dimethylhydrazine and ionic liquids†
Received
28th October 2011
, Accepted 1st February 2012
First published on 6th March 2012
Abstract
The reaction of 1,1-dimethylhydrazine with excess dichloromethane led to the formation of the chloride salt of the 1-(chloromethyl)-1,1-dimethylhydrazinium cation ([(CH3)2N(CH2Cl)NH2]Cl, 1). The reaction of 1 with a suitable silver salt provided the nitrate ([(CH3)2N(CH2Cl)NH2][NO3], 2), perchlorate ([(CH3)2N(CH2Cl)NH2][ClO4], 3), azide ([(CH3)2N(CH2Cl)NH2][N3], 4), dicyanamide ([(CH3)2N(CH2Cl)NH2][N(CN)2], 5) and sulphate ([(CH3)2N(CH2Cl)NH2]2[SO4], 6) salts. Compound 6 reacted with barium 5,5′-azobistetrazolate pentahydrate (Ba[N4C–N
N–CN4]·5H2O), barium dipicrate tetrahydrate (Ba[(NO2)3Ph–O]2·4H2O) and barium 5-amino-1H-tetrazolate tetrahydrate (Ba[H2N–CN4]2·4H2O) to form the corresponding metathesis products: [(CH3)2N(CH2Cl)NH2]2[N4C–N
N–CN4] (7), [(CH3)2N(CH2Cl)NH2][(NO2)3Ph–O] (8) and [(CH3)2N(CH2Cl)NH2][H2N–CN4] (9). Compounds 1–9 were characterized by elemental analysis, mass spectrometry, NMR (1H and 13C) and vibrational spectroscopy (infrared and Raman). Additionally, we measured the 15N NMR spectrum of the nitrate salt 2 and identified the solid state structure of compounds 3, 6, 7 and 8 by low temperature X-ray crystallography (3: Triclinic P
, a = 5.983(1) Å, b = 7.502(1) Å, c = 9.335(1) Å; α = 93.86(1)°, β = 101.21(1)°; γ = 91.13(1)°; V = 409.8(1) Å3, 6: Monoclinic C2/c, a = 11.674(2) Å, b = 17.503(3) Å, c = 6.616(1) Å; β = 90.27(1)°; V = 1351.8(4) Å3, 7: Triclinic P
, a = 8.851(1) Å, b = 8.872(1) Å, c = 11.529(1) Å; α = 80.98(1)°, β = 83.47(1)°; γ = 71.37(1)°; V = 845.4(1) Å3 and 8: Monoclinic C2/c, a = 24.168(3) Å, b = 7.375(1) Å, c = 17.062(3) Å; β = 116.19(2)°; V = 1351.8(3) Å3). The solid state structure of barium dipicrate hexahydrate (Ba[(NO2)3Ph–O]2·6H2O) was also elucidated: Triclinic P
, a = 6.641(1) Å, b = 11.588(1) Å, c = 15.033(1) Å; α = 84.64(1)°, β = 80.07(1)°; γ = 86.80(1)°; V = 1133.8(1) Å3. Furthermore, we studied the thermal properties of compounds 1–9 by differential scanning calorimetry (DSC). Salts 2–4, 8 and 9 fall within the category of ionic liquids. Lastly, the energetic salts were subjected to standard sensitivity tests and a software code was used to predict the detonation parameters and specific impulses of the compounds and their mixtures with an oxidizer.
Introduction
Hydrazine and its derivatives, such as monomethylhydrazine (MMH) and 1,1-dimethylhydrazine (UDMH) can ignite spontaneously on contact with an oxidizer, such as nitrogen tetroxide (N2O4), white fuming nitric acid (WFNA) and red fuming nitric acid (RFNA)1–4 to form hypergolic mixtures.5,6 Hypergolic systems are widely used in rocket boosters where the fuel and oxidizer are stored separately and then mixed when the rocket is launched. This allows the rocket to re-ignite and simplifies engine design. However, hydrazine and its derivatives are highly toxic, volatile and they can form explosive mixtures with air.7 In this context, salt-based materials have several advantages over analogous non-ionic molecular compounds, such as higher densities (i.e. higher specific impulses) and lower vapour pressures (i.e. lower toxicities). Recently,8,9 a series of salts based on the 2,2-dimethyltriazanium cation ([(CH3)2N(NH2)2]+) (Scheme 1) were prepared. The anions (A−) of choice included nitrocyanamide ([N(CN)(NO2)]−), dicyanamide ([N(CN)2]−), dinitrocyanmethanide ([C(CN)(NO2)2]−), nitrodicyanmethanide ([C(CN)2(NO2)]−), tricyanmethanide ([C(CN)3]−), nitrate ([NO3]−), 5-amino-1H-tetrazolate ([N4C–NH2]−), 5-nitro-2H-tetrazolate ([N4C–NO2]−) and 5,5′-azobistetrazolate ([N4C–N
N–CN4]2−).
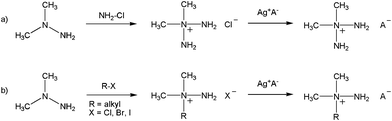 |
| Scheme 1 A general method for the synthesis of a) alkyltriazanium salts8,9 and b) alkylhydrazinium salts.10,11 | |
Later on, the authors of ref. 8 also reported the synthesis of a new family of salts based on the [(CH3)2N(R)NH2]+ cation (R = Me, Et, n-Bu, allyl, propargyl, –CH2CH2OH and –CH2CN) and dicyanamide ([N(CN)2]−) and nitrocyanamide ([N(CN)(NO2)]−) anions.10 From these two studies it could be concluded that both [(CH3)2N(NH2)2]+ and [(CH3)2N(R)NH2]+ cations can be used for the formation of ionic liquids with hypergolic properties.
In 2001, the Sharp group11 reported on the unexpected easy reaction between 1,1-dimethylhydrazine (UDMH) and dichloromethane or 1,2-dichloroethane to form [(CH3)2N(CH2Cl)NH2]Cl (1) and [(CH3)2N(CH2CH2Cl)NH2]Cl, respectively. Our research in the field of hydrazines12 drew our attention to the easy synthesis of 111,13 and we hypothesised whether the [(CH3)2N(CH2Cl)NH2]+ cation might form salts with interesting energetic properties. In this report we present our results on the synthesis, characterization and energetic testing of several energetic salts based on the previously relatively neglected [(CH3)2N(CH2Cl)NH2]+ cation.
Results and discussion
Syntheses
1,1-Dimethylhydrazine (UDMH) was reacted with dichloromethane to form the chloride salt of the 1-(chloromethyl)-1,1-dimethylhydrazinium cation ([(CH3)2N(CH2Cl)NH2]Cl, 1) (Scheme 2) with an improved yield of 75%.11,131 was obtained as a highly crystalline hygroscopic solid that can be stored under nitrogen for extended periods of time. The analogous reaction using monomethylhydrazine (MMH) instead of UDMH does not form the product of addition, rather an acid–base reaction takes place with MMH acting as a base (B) to form [MeNH–NH3]Cl and the corresponding carbene (Scheme 3).
![The synthesis of salts of the [(CH3)2N(CH2Cl)NH2]+ cation.](/image/article/2012/RA/c2ra00968d/c2ra00968d-s2.gif) |
| Scheme 2 The synthesis of salts of the [(CH3)2N(CH2Cl)NH2]+ cation. | |
![A general reaction equation leading to the formation of [MeNH–NH3]Cl. B = monomethylhydrazine (MMH).](/image/article/2012/RA/c2ra00968d/c2ra00968d-s3.gif) |
| Scheme 3 A general reaction equation leading to the formation of [MeNH–NH3]Cl. B = monomethylhydrazine (MMH). | |
Compound 1 was weighed in a nitrogen-filled glove box and reacted with a suitable silver salt in either water or alcohol, leading to the precipitation of silver chloride and the formation of the nitrate ([(CH3)2N(CH2Cl)NH2][NO3], 2), perchlorate ([(CH3)2N(CH2Cl)NH2][ClO4], 3), azide ([(CH3)2N(CH2Cl)NH2][N3], 4), dicyanamide ([(CH3)2N(CH2Cl)NH2][N(CN)2], 5) and sulphate ([(CH3)2N(CH2Cl)NH2]2[SO4], 6) salts (Scheme 1). Subsequently, the sulphate salt (6) was reacted in either water or alcohol with a suitable barium compound to form insoluble barium sulphate and the corresponding 5,5′-azobistetrazolate ([(CH3)2N(CH2Cl)NH2]2[N4C–N
N–CN4], 7), picrate ([(CH3)2N(CH2Cl)NH2][(NO2)3Ph–O], 8) and 5-amino-1H-tetrazolate ([(CH3)2N(CH2Cl)NH2][H2N–CN4], 9) salts. Alternatively, the corresponding silver salts can be used to synthesise compounds 7, 8 and 9. However, due to the high sensitivity of silver 5,5′-azobistetrazolate and silver picrate towards impact and friction, the barium salts are preferred from the standpoint of safety. Compounds 1–6 are colourless materials, whereas 7 and 8 are bright yellow solids. Salts 1–9 are readily soluble in alcohol, acetone (except for 1), water, DMSO or DMF and insoluble in apolar solvents, such as dichloromethane and ether. Lastly, all the materials in this work are stable to hydrolysis.
Vibrational and NMR spectroscopy
Computational details.
Full geometry optimization of the [(CH3)2N(CH2Cl)NH2]+ cation was performed using the density functional B3LYP method14,15 with a 6-311+G(d,p) basis set. The optimized structure was characterized as a true energy minimum on the potential energy surface without imaginary frequencies. For the optimized structure harmonic vibrational frequencies, infrared intensities and Raman scattering activities and NBO analysis were calculated at the same level of theory using the Gaussian 03W package.16 The calculated harmonic frequencies were scaled by a factor of 0.9613 (B3LYP) in accordance with the method of Scott and Radom.17
The harmonic vibrational frequencies computed for the [(CH3)2N(CH2Cl)NH2]+ cation are summarized in the supporting information (SI Table 1†) with the average experimental data. The B3LYP computed values show an overestimation in comparison to the experimental frequencies since anharmonicity was neglected in the real system.18 The frequencies of the [(CH3)2N(CH2Cl)NH2]+ cation observed experimentally in the different compounds were found at fairly consistent values. The Raman spectra were characterized by two bands of strong activity at ca. 2980 cm−1 (C–H symmetrical stretching) and at ca. 740 cm−1 (N–N and C–Cl stretching modes). The IR spectra, however, were dominated by asymmetric and symmetric N–H stretching modes at 3275 and 3180 cm−1, respectively. Additionally, other bands of lower intensity could be assigned as follows (ν = stretching, δ = in-plane bending, γ = out-of-plane bending, ω = in-plane rocking, τ = torsion and s = symmetric): 2880–3050 cm−1 [νs(C–H)], 1430–1620 cm−1 [δ(N–H) + δ(N–H)], 1290–1390 cm−1 [γ(C–H) + γ(N–H)], 970–1250 cm−1 [τ(C–H) + τ(N–H)], 740–970 cm−1 [ω(N–H) + ω(C–H)], ca. 565 cm−1 [νs(N–C)] and <500 cm−1 [ω(C–H) + ω(N–H)].19,20
The structure of the [(CH3)2N(CH2Cl)NH2]+ cation in compounds 1–9 was also characterized by multinuclear (1H, 13C and 15N) NMR spectroscopy in DMSO-d6 (SI Table 2†). The 1H NMR spectra showed the three expected resonances. The methyl group resonated at ca. 3.3 ppm, whereas the –CH2Cl group resonance is shifted to a lower field at ca. 5.4 ppm and the –NH2 group protons were found between ca. 6.2 and 6.7 ppm. In the 13C NMR spectra the methyl groups resonated at ca. 53 ppm and the –CH2Cl carbon atoms at ca. 72 ppm. Lastly, we measured the 15N NMR spectrum (natural abundance) of the [(CH3)2N(CH2Cl)NH2]+ cation for the nitrate salt 2 (Fig. 1). The –N(CH3)2) group resonated at ca. 85 ppm and no coupling was observed whereas the –NH2 group coupled at one bond with its protons (1JN–H = 66.7 Hz) and appeared as a triplet at ca. 116 ppm.
![The 15N NMR spectrum of [(CH3)2N(CH2Cl)NH2][NO3] (2) in DMSO-d6.](/image/article/2012/RA/c2ra00968d/c2ra00968d-f1.gif) |
| Fig. 1 The 15N NMR spectrum of [(CH3)2N(CH2Cl)NH2][NO3] (2) in DMSO-d6. | |
Crystal structures.
The solid state structure of compounds 3, 6, 7, 8 and that of barium dipicrate hexahydrate (BaPic = Ba[(NO2)3Ph–O]2·6H2O) were determined by X-ray crystallography at low temperature. Crystals suitable for these measurements were grown as described in the experimental section, which also contains details concerning the method used for solving the structures. Only the crystal structure of the perchlorate salt 3 will be described here.
To obtain a better understanding of the structure of the [(CH3)2N(CH2Cl)NH2]+ cation, the optimized structure of the [(CH3)2N(CH2Cl)NH2]+ cation in the gas phase was obtained using B3LYP functional analyses with a B3LYP/6-311+G(d,p) basis set.21 A natural bond orbital (NBO) analysis22 was performed on the optimized structure of the [(CH3)2N(CH2Cl)NH2]+ cation (Fig. 2). The optimized gas phase geometry of the [Me2N(CH2Cl)NH2]+ cation is identical to that found in the solid state structure of [(CH3)2N(CH2Cl)NH2]2Cl (1, Fig. 3) but not to that found for [(CH3)2N(CH2Cl)NH2][ClO4] (3, Fig. 4), which forms an intramolecular hydrogen bond (see the discussion further on). According to the NBO analysis, N6 carries a relatively large negative charge (−0.604 e), whereas Cl1 carries a small positive charge (+0.047 e). This charge distribution suggests that N6 can form hydrogen bonds, whereas Cl1 should not. These results are in agreement with the X-ray crystallography studies that show, in contrast to Cl1, that N6 participates in the formation of medium-to-strong hydrogen bonds (SI Table 5†).
![Optimized geometry and charge distribution in the [Me2N(CH2Cl)NH2]+ cation as found with NBO analysis (B3LYP/6-31+G(d,p)).](/image/article/2012/RA/c2ra00968d/c2ra00968d-f2.gif) |
| Fig. 2 Optimized geometry and charge distribution in the [Me2N(CH2Cl)NH2]+ cation as found with NBO analysis (B3LYP/6-31+G(d,p)). | |
![Diamond plot (at 50% probability) of the asymmetric unit of [Me2N(CH2Cl)NH2]Cl (1) with the labelling scheme. The dashed line represents the hydrogen bond between the [(CH3)2N(CH2Cl)NH2]+ cation and the Cl− anion.](/image/article/2012/RA/c2ra00968d/c2ra00968d-f3.gif) |
| Fig. 3 Diamond plot (at 50% probability) of the asymmetric unit of [Me2N(CH2Cl)NH2]Cl (1) with the labelling scheme. The dashed line represents the hydrogen bond between the [(CH3)2N(CH2Cl)NH2]+ cation and the Cl− anion. | |
 (3) with the labelling scheme and the formation of an intramolecular hydrogen bond (dashed line).](/image/article/2012/RA/c2ra00968d/c2ra00968d-f4.gif) |
| Fig. 4 Diamond plot (at 50% probability) of the asymmetric unit of [Me2N(CH2Cl)NH2](ClO4) (3) with the labelling scheme and the formation of an intramolecular hydrogen bond (dashed line). | |
Contrary to the structure of the previously reported chloride salt 1 (Fig. 3),13 the N3–C2 bond in [Me2N(CH2Cl)NH2][ClO4] (3) is twisted so that the chlorine atom forms an intramolecular hydrogen bond (N6⋯Cl1 = 3.117(5) Å) with the graph-set descriptor S(5) (Fig. 4).23,24 This intramolecular hydrogen bond is also found for one of the two crystallographically independent [(CH3)2N(CH2Cl)NH2]+ cations in the crystal structure of [(CH3)2N(CH2Cl)NH2]2[N4C–N
N–CN4] (6) (see X-ray discussion in the supporting information†).
The cation in [Me2N(CH2Cl)NH2][ClO4] (3) forms two classical hydrogen bonds to the anion, which result in the formation of infinite zig-zag chains running parallel to the a-axis (Fig. 5). These chains take the graph-set descriptor C2,2(6) and are analogous to the C2,2(4) motifs found in the crystal structure of [Me2N(CH2Cl)NH2]Cl (1).13
 (3, view along the c-axis).](/image/article/2012/RA/c2ra00968d/c2ra00968d-f5.gif) |
| Fig. 5 The formation of zig-zag chains along the a-axis via hydrogen-bonding (dashed lines) in the crystal structure of [Me2N(CH2Cl)NH2](ClO4) (3, view along the c-axis). | |
Thermodynamic and energetic properties
Electronic energy calculations.
The electronic energies of the [(CH3)2N(CH2Cl)NH2]+ cation and all the energetic anions in this study were calculated using the Gaussian 03 W suite of programs.10 Calculations were performed using Møller–Plesset perturbation theory truncated at the second order (MP2)25 and the values were used unscaled., The correlation consistent polarized valence double-zeta basis set cc-pVDZ was used for all the atoms in all the calculations.26,27 The method used here was previously described in detail.28
The sensitivities towards impact, friction and electrostatic discharge of the salts of the [(CH3)2N(CH2Cl)NH2]+ cation in this study were tested using BAM procedures (Table 1).29–32 None of the compounds tested positive towards impact (BAM drop-hammer sensitivity: >40 J) and friction (BAM friction tester sensitivity: >360 N) nor when spraying sparks across a small sample of the materials using a Tesla coil (ESD testing). Also, no decomposition reaction was observed when grinding the compounds vigorously in a ceramic mortar. Therefore, the salts of the [(CH3)2N(CH2Cl)NH2]+ cation in this study are safe for transport according to international standards.32 In comparison to commonly used energetic materials, e.g. TNT or RDX = 1,3,5-trinitroperhydro-1,3,5-triazine,33 the compounds described in this report are less sensitive towards classical stimuli and they are also less sensitive than other salts based on the same anions.34,35
Table 1 Computed performance (using the EXPLO5 computer code) and sensitivity towards impact, friction, electrostatic discharge and thermal shock of energetic salts of the [(CH3)2N(CH2Cl)NH2]+ cation
|
T
ex (K)a |
V
0 (L kg−1)b |
P
det (kbar)c |
D (m s−1)d |
I
sp (s)e |
Impact (J) f |
Friction (N) f |
ESD (±)g |
Thermal Shock h |
Temperature of the explosion gases.
Volume of the explosion gases.
Detonation pressure (detonation run with 1% TFNA = trifluoro-trinitro-azahexane).
Detonation velocity (detonation run with 1% TFNA = trifluoro-trinitro-azahexane).
Specific impulse (isobaric combustion for the pure compound at a chamber pressure = 60.0 bar).
Impact and friction sensitivity values according to BAM methods (see ref. 29–32).
Rough sensitivity to electrostatic discharge using a Tesla coil (∼20 kV), + sensitive, − insensitive.
Response of fast heating in the “flame test”.
|
2
|
3672 |
794 |
190 |
7395 |
193 |
>40 |
>360 |
— |
Burns |
3
|
5190 |
749 |
279 |
8160 |
225 |
>40 |
>360 |
— |
Deflagrates |
4
|
2524 |
757 |
187 |
7120 |
196 |
>40 |
>360 |
— |
Burns |
5
|
3005 |
675 |
145 |
6518 |
189 |
>40 |
>360 |
— |
Burns |
7
|
3632 |
725 |
240 |
7926 |
223 |
>40 |
>360 |
— |
Burns |
8
|
3671 |
654 |
203 |
7278 |
181 |
>40 |
>360 |
— |
Burns |
9
|
2415 |
746 |
189 |
7250 |
201 |
>40 |
>360 |
— |
Burns |
The electronic energies of the [(CH3)2N(CH2Cl)NH2]+ cation and the anions of salts 2, 3, 4, 5, 7, 8 and 9 were obtained from quantum chemical calculations (MP2). These values were used to predict the formation energies of salts 2, 3, 4, 5, 7, 8 and 9 (Table 2). The predicted formation energies of compounds 2, 3, 4, 5, 7, 8 and 9 have positive values and are lowest for the carbon-richer picrate salt (8, ΔU°f = 195 kJ kg−1) and highest for the nitrogen-richer 5,5′-azotetrazolate salt (7, ΔUf = 2866 kJ kg−1). These values are between those of TNT33 and of the highly endothermic bis(hydrazinium) 5,5′-azotetrazolate (ΔU°f = +3700 kJ kg−1).36
Table 2 Physical and chemical properties of energetic salts of the [(CH3)2N(CH2Cl)NH2]+ cation
|
2
|
3
|
4
|
5
|
7
|
8
|
9
|
Formula |
C3H10N3ClO3 |
C3H10N2Cl2O4 |
C3H10N5Cl |
C5H10N5Cl |
C8H20N14Cl2 |
C9H12N5ClO7 |
C4H12ClN7 |
Phase transition temperature.
Decomposition point (DSC onsets) from measurement with β = 5°C min−1.
Oxygen balance according to ref. 46.
Density from pycnometer (2, 4 and 5) or X-ray (3, 7 and 8) measurements.
Lattice enthalpy.
Computed (MP2)35 energy of formation.
Computed (MP2) enthalpy of formation.
|
MW (g mol−1) |
171.58 |
209.03 |
151.60 |
175.62 |
383.24 |
337.67 |
193.64 |
T
m/Tg (°C)a |
−9.7 |
52.0 |
17.2 |
— |
— |
77 |
64 |
T
d (°C)b |
161 |
180 |
147 |
188 |
172 |
208 |
165 |
Ω (%)c |
−70 |
−46 |
−111 |
−132 |
−104 |
−78 |
−111 |
ρ/g cm−3 d |
1.474 |
1.694 |
1.418 |
1.308 |
1.521 |
1.644 |
1.521 |
ΔHL/kJ mol−1 e |
514 |
507 |
525 |
496 |
771 |
444 |
502 |
ΔU°f/kJ kg−1 f |
566 |
1557 |
2376 |
2041 |
2866 |
195 |
2317 |
ΔH°f/kJ kg−1 g |
507 |
1512 |
2261 |
1985 |
2809 |
170 |
2202 |
The performance of new materials has been the centre of attention of recent investigations.37,38 Using the EXPLO539 computer software, it might be possible to calculate certain performance and detonation parameters of interest for compounds based on C, H, N, O, Al, Cl, Si and F. The program is based on Becker–Kistiakowsky–Wilson (BKWN) rules40 and uses the following values for the BKWN equation of state: α = 0.5, β = 0.176, κ = 14.71 and θ = 6620. The formation energies (ΔU°f) of the salts of the [(CH3)2N(CH2Cl)NH2]+ cation were back-calculated on the basis of the combustion equations of the compounds (see below), Hess's Law, the known standard heats of formation for dioxygen, carbon dioxide, water, dinitrogen and hydrogen chloride.41 Using the EXPLO5 computer code.39 these values were subsequently used in conjunction with the molecular formula and density of the compounds (either from the X-ray or from the pycnometer measurements) to predict the detonation performance parameters (pressure and velocity) and the specific impulse for each compound. The densities of the salts of the [(CH3)2N(CH2Cl)NH2]+ cation in this study varied from 1.308 (compound 5) to 1.694 (compound 3) g cm−3 and were higher than those of salts of the [(CH3)3NNH2]+ cation. For example, dicyanamide salt 3 (ρ = 1.308 g cm−3) was significantly denser than the analogous salt of the [(CH3)3NNH2]+ cation (ρ = 1.10 g cm−3).10
2: [(CH3)2N(CH2Cl)NH2][NO3] (l) + 3.75 O2 → 3 CO2 (g) + 4.5 H2O (l) + HCl (g) + 1.5 N2 (g)
3: [(CH3)2N(CH2Cl)NH2][ClO4] (s) + 3 O2 → 3 CO2 (g) + 4 H2O (l) + 2 HCl (g) + N2 (g)
4: [(CH3)2N(CH2Cl)NH2][N3] (s) + 5.25 O2 → 3 CO2 (g) + 4.5 H2O (l) + HCl (g) + 2.5 N2 (g)
5: [(CH3)2N(CH2Cl)NH2][N(CN)2] (l) + 7.25 O2 → 5 CO2 (g) + 4.5 H2O (l) + HCl (g) + 2.5 N2 (g)
7: [(CH3)2N(CH2Cl)NH2]2[N4C–N
N–CN4] (s) + 12.5 O2 → 8 CO2 (g) + 9 H2O (l) + 2 HCl (g) + 7 N2 (g)
8: [(CH3)2N(CH2Cl)NH2][(NO2)3Ph–O] (s) + 8.25 O2 → 9 CO2 (g) + 5.5 H2O (l) + HCl (g) + 2.5 N2 (g)
9: [(CH3)2N(CH2Cl)NH2][H2N–CN4] (s) + 6.75 O2 → 4 CO2 (g) + 5.5 H2O (l) + HCl (g) + 3.5 N2 (g)
The detonation parameters (i.e. detonation pressure and velocity) of compounds 2, 3, 4, 5, 7, 8 and 9 varied from P = 145 to 279 kbar and from D = 6518 to 8160 m s−1. Note that the formation energies were predicted using MP2-calculated electronic energies thus the calculated detonation parameters tended to be an overestimation.42 Whatever the case, the detonation velocities of compounds 2, 3, 6 and 7 were higher than that of TNT (D = 7171 m s−1) and the detonation velocities of the perchlorate (3, D = 8160 m s−1) and the 5,5′-azotetrazolate (7, D = 7926 m s−1) salts were expected to be comparable to that of nitroglycerine (D = 7714 m s−1).33 In comparison to analogous salts of the [(CH3)3NNH2]+ cation, the salts of the [(CH3)2N(CH2Cl)NH2]+ cation in this work had higher detonation parameters. For example, the dicyanamide salt 5 (Pdet = 145 kbar and D = 6518 m s−1) was expected to be more energetic than the dicyanamide salt of the [(CH3)3NNH2]+ cation (Pdet = 108 kbar and D = 6317 m s−1).10 On the other hand, the predicted specific impulses (Isp) varied between 181 s (8) and 225 s (3), higher than for recently reported salts based on the guanylurea cation43 and comparable to those of 1,1-dimethylhydrazine energetic salts.10
It is known that the performance of an energetic material is dependent on the compound's density, heat of formation and oxygen balance and that it reaches a maximum value when the oxygen balance is neutral.44 The densities of all the compounds in this work were calculated using the X-ray data of experimental measurements using a pycnometer. The compounds had moderate density values ranging from 1.308 (5) to 1.694 (3) g cm−3, which were, however, higher than those of recently reported polycyano-anion-based45 and 1,1-dimethylhydrazine energetic salts.10 The oxygen balances (Ω) of all the salts in this work were negative and varied between −46% (3) and −132% (5), in the range between commonly used RDX (Ω = −22%) and recently reported hypergolic ionic liquids, such as trimethylhydrazinium dicyanamide (Ω = −176%).10 Due to the negative oxygen balance (Ω) of compounds 2, 3, 4, 5, 7, 8 and 9, the performance parameters of mixtures of the compounds with an oxidizer, namely AN = ammonium nitrate (Ω = +20%) and ADN = ammonium dinitramide (Ω = +26%) were also calculated in order to increase the performance of the mixture in comparison to the stand-alone materials (SI Tables 7 and 8†). Formulations of compounds 2, 3, 4, 5, 7, 8 and 9 with AN or ADN are predicted to have higher detonation pressures and detonation velocities than isolated compounds. Mixtures with ADN are predicted to perform particularly well with detonation velocities comparable to pure RDX (D = 8750 m s−1).33 Also, formulations with AN and in particular with ADN are expected to have higher specific impulses than stand-alone compounds 2, 3, 4, 5, 7, 8 and 9, and those calculated for AN (Isp = 159 s) and ADN (Isp = 199 s).
Additionally, the melting points/glass transition temperatures and thermal stability of all the salts in this work were assessed by differential scanning calorimetry (DSC) measurements. The DSC measurements were carried out on small samples of the salts of the [(CH3)2N(CH2Cl)NH2]+ cation at a heating rate of β = 5°C min−1. Salts 2–4, 8 and 9 are ionic liquids (m.p. < 100 °C). Salts 2, 4 and 5 are room temperature ionic liquids. On the other side, the thermal stabilities of the compounds vary from moderate (4: 147 °C) to high (8: 208 °C). We are currently investigating the hypergolic properties of mixtures of the compounds in this study with WFNA in view to developing a possible energetic application. These results will be the subject of a future publication.
In addition to the DSC analysis, the response to fast heating under non-confinement conditions was tested by placing a small sample of the materials under a Bunsen burner flame. This resulted in normal burning for all the compounds except for the better oxygen-balance perchlorate salt (3), which presented a burning-to-deflagration transition. Under similar test conditions, commonly used energetic materials, such as TNT and RDX burn and deflagrate, respectively.
Experimental section
Safety note
Most of the compounds handled and/or synthesized in this work contain energetic counter anions. Although preliminary testing shows decreased sensitivity of the materials in this study, they should nevertheless be regarded as energetic and their synthesis should be carried out only by experienced personnel familiar with the applicable safety procedures. The synthesis and handling of highly sensitive silver azide must be carried out with particular care and with suitable protection measures, such as wearing Kevlar gloves and jacket, conductive shoes, ear and face protection, and plastic equipment.
Synthesis
[(CH3)2N(CH2Cl)NH2]NO3 (2).
Neat silver nitrate (1.018 g, 5.99 mmol) was added portion-wise to a colourless solution of 1 (0.869 g, 5.99 mmol) in ethanol (25 mL) producing the immediate precipitation of a white powder. The reaction mixture was stirred for 4 h in darkness and the insoluble silver chloride was filtered and washed with fresh ethanol. The ethanol filtrates were combined and the solvent was stripped at room temperature under high vacuum to give a colourless oil (0.966 g, 94%). No further purification was necessary. ρ (17.6 °C, pycnometer) = 1.474 g mL−1; C3H10N3O3Cl (MW = 171.58 g mol−1, calc./found): C 21.00/20.78, H 5.87/5.74, N 24.49/24.13; DSC (5 °C min−1): −9.7 (Tg), >161 °C (dec.); m/z (+c ESI, H2O): 109.0 (71, [Cat]), 280.0 (100, [Cat2A]), 450.9 (62, [Cat3A2]), 623.9 (67, [Cat4A3]), 794.7 (51, [Cat5A4]), 965.8 (45, [Cat6A5]); m/z (–c ESI, H2O): 233.0 (44, [CatA2]), 403.8 (50, [Cat2A3]), 576.7 (48, [Cat3A4]), 747.7 (100, [Cat4A5]), 918.6 (75, [Cat5A6]); 1H NMR (DMSO-d6, 400.18 MHz, TMS) δ (ppm): 3.31 (6 H, s, –CH3), 5.34 (2 H, s, –CH2Cl), 6.22 (2 H, s(br), –NH2); 13C 1H NMR (DMSO-d6, 100.52 MHz, TMS) δ (ppm): 71.57 (1 C, –CH2Cl), 53.15 (2 C, N–CH3); 14N NMR (DMSO-d6, 40.51 MHz, NH3) δ (ppm): +377.2 (s, NO3−); 15N NMR (DMSO-d6, 40.51 MHz, NH3) δ (ppm): +377.5 (s, NO3−), +115.6 (1 N, t, 1JN–H = 66.7 Hz, –NH2), +84.6 (1 N, s, –N(CH3)2); Raman ṽ/cm−1 (rel. int.): 3289(3), 3166(6), 3044(46), 2979(100), 2882(14), 1448(15), 1048(46), 1009(4), 968(9), 888(5), 809(3), 744(53), 711(4), 528(3), 480(3), 440(2), 356(6), 245(3); IR v˜/cm−1 (golden gate, rel. int.): 3267(m) 3160(m) 3041(m) 2981(w) 2383(w) 2079(w) 1750(w) 1629(m) 1472(m) 1313(s) 1091(m) 1042(m) 1002(w) 956(m) 893(m) 827(m) 804(m) 743(m) 709(w) 570(w).
[(CH3)2N(CH2Cl)NH2]ClO4 (3).
1 (0.135 g, 0.93 mmol) and anhydrous silver perchlorate (previously weighed in a glove box, 0.193 g, 0.93 mmol) were loaded in a Schlenk flask, the air was pumped out and the flask was filled with nitrogen. The two solids were taken up in ethanol (10 mL) and the resulting suspension was stirred at room temperature in darkness for 1 h 15 min. At this point, the insoluble materials were filtered and washed with fresh ethanol. Stripping of the solvent under reduced pressure left a colourless crystalline pure solid behind (0.183 g, 94%). Single crystals of the compound suitable for X-ray analysis formed during slow evaporation of a methanolic solution. ρ (X-ray) = 1.694 g cm−3; C3H10Cl2N2O4 (MW = 209.03 g mol−1, calc./found): C 17.24/17.09, H 4.82/4.62, N 13.40/13.11; DSC (5 °C min−1): 52.0 °C (m.p.), >180 °C (dec.); m/z (+c ESI): 60.0 (6), 109.0 (80, [Cat]+), 111.1 (23), 253.0 (15, [Cat2Cl]+), 255.0 (5), 316.9 (100), 318.9 (95, [Cat2A]+), 320.9(26), 526.6 (6, [Cat3A2]+), 528.6 (2); m/z (–c ESI): 83.0 (22), 99.0 (100, [A]−), 101.0 (44), 221.0 (12, [A2Na]−), 222.9 (7), 280.1 (20), 306.9 (55, [A2Cat]−), 308.8 (46), 516.6 (34, [A3Cat2]−), 518.6 (22); 1H NMR (DMSO-d6, 400.18 MHz, TMS) δ (ppm): 3.30 (6 H, s, –CH3), 5.33 (2 H, s, –CH2Cl), 6.21 (2 H, s(br), –NH2); 13C 1H NMR (DMSO-d6, 100.52 MHz, TMS) δ (ppm): 71.60 (1 C, –CH2Cl), 53.18 (2 C, N–CH3); 35Cl NMR (DMSO-d6, 49.03 MHz, NaCl) δ (ppm): 1008.0 (ClO4−); Raman v˜/cm−1 (rel. int.): 3337(5), 3281(7), 3209(5), 3048(12), 2983(61), 2882(46), 2849(2), 1457(6), 1293(3), 1200(2), 1136(2), 933(100), 893(6), 805(2), 745(16), 625(11), 528(4), 460(11), 388(1), 351(4), 240(2); IR v˜/cm−1 (golden gate, rel. int.): 3328(m) 3281(w) 3200(w) 3060(w) 3039(w) 2981(w) 2923(w) 2512(w) 2167(w) 2027(w) 1618(w) 1471(m) 1458(m) 1428(w) 1411(w) 1383(w) 1329(w) 1294(w) 1248(w) 1189(w) 1054(s) 996(m) 947(m) 933(m) 890(m) 801(m) 744(m) 619(s) 560(w).
[(CH3)2N(CH2Cl)NH2][N3] (4).
Dry silver azide (0.370 g, 2.47 mmol) was suspended in distilled water (10 mL) and 1-(chloromethyl)-1,1-dimethylhydrazinium chloride (0.298 g, 2.05 mmol) was added in one portion. The reaction mixture was stirred for 4 h in darkness. At this point the insoluble excess silver azide and the silver chloride were filtered, washed with water and the solvent was stripped at room temperature under high vacuum to obtain the title compound as a pale yellow oil (0.280 g, 90%). ρ (17.6 °C, pycnometer) = 1.418 g mL−1; C3H10N5Cl (MW = 151.60 g mol−1, calc./found): C 23.77/23.49, H 6.65/6.24, N 46.20/45.91; DSC (5 °C min−1): 17.2 (m.p.), >147 °C (dec.); m/z (+c ESI, H2O): 109.0 (100, [Cat]), 260.0 (87, [Cat2A]), 411.6 (42, [Cat3A2]), 563.2 (11, [Cat4A3]), 714.7 (1, [Cat5A4]), m/z (–c ESI, H2O): 193.2 (100, [CatA2]−), 344.4 (22, [Cat2A3]−), 496.0 (8, [Cat3A4]−); 1H NMR (DMSO-d6, 400.18 MHz, TMS) δ (ppm): 3.33 (6 H, s, –CH3), 5.42 (2 H, s, –CH2Cl), 6.42 (2 H, s(br), –NH2); 13C 1H NMR (DMSO-d6, 100.52 MHz, TMS) δ (ppm): 72.09 (1 C, –CH2Cl), 53.43 (2 C, N–CH3); 14N NMR (DMSO-d6, 40.51 MHz, NH3) δ (ppm): +249 (1 N, NNN), +105 (1 N, NNN), +85 (1 N, –N(CH3)2); Raman ṽ/cm−1 (rel. int.): 3029(86), 2964(100), 1762(11), 1635(12), 1570(9), 1449(15), 1326(11), 1042(7), 968(6), 890(9), 805(7), 741(89), 473(26), 440(22), 366(21), 242(16); IR v˜/cm−1 (golden gate, rel. int.): 3226(m) 3103(m) 3029(m) 2010(s) 1715(w) 1631(m) 1470(m) 1343(w) 1301(w) 1240(w) 1202(w) 1093(m) 1003(w) 957(m) 894(m) 804(m) 743(w) 630(w) 609(w) 577(w) 530(w) 504(w).
[(CH3)2N(CH2Cl)NH2][(N(CN)2] (5).
1 (0.435 g, 3.00 mmol) and silver dicyanamide (0.626 g, 3.60 mmol, 1.20 eq) were reacted in distilled water (10 mL) for 7 h under the exclusion of light. The insoluble silver chloride was then filtered and the solvent was evaporated at 40 °C using an oil vacuum pump, leaving behind a colourless liquid (0.480 g, 91%). ρ (17.6 °C, pycnometer) = 1.308 g cm−3; C5H10ClN5 (MW = 175.62 g mol−1, calc./found): C 34.20/33.84, H 5.74/5.61, N 39.88/39.64; DSC (5 °C min−1): >188 °C (dec.); m/z (+c ESI): 109.0 (100, [Cat]+), 283.9 (72, [Cat2A]+), 459.8 (20, [Cat3A2]+), 635.8 (15, [Cat4A3]+), 810.8 (9, [Cat5A4]+); m/z (–c ESI): 240.9 (18, [A2Cat]−), 415.8 (100, [A3Cat2]−), 590.7 (58, [A4Cat3]−); 1H NMR (DMSO-d6, 400.18 MHz, TMS) δ (ppm): 3.29 (6 H, s, –CH3), 5.31 (2 H, s, –CH2Cl), 6.19 (2 H, s(br), –NH2); 13C 1H NMR (DMSO-d6, 100.52 MHz, TMS) δ (ppm): 119.11 (2 C, –CN), 72.01 (1 C, –CH2Cl), 53.37 (2 C, N–CH3); Raman v˜/cm−1 (rel. int.): 3151(2), 3040(11), 2974(26), 2222(6), 2199(100), 2138(3), 1446(3), 1302(2), 1044(2), 958(2), 907(5), 808(2), 743(17), 666(7), 527(3), 440(4), 355(6), 185(54); IR v˜/cm−1 (golden gate, rel. int.): 3507(w) 3250(w) 3139(w) 3034(w) 2973(w) 2238(m) 2197(m) 2127(s) 1627(w) 1470(m) 1315(m) 1240(w) 1201(w) 1092(w) 1003(w) 956(m) 896(m) 805(m) 743(w) 662(w) 522(m) 471(w) 459(w).
[(CH3)2N(CH2Cl)NH2]2[N4C–N
N–CN4] (7).
Method 1 (using silver 5,5′-azotetrazolate): Sodium 5,5′-azotetrazolate pentahydrate (0.337 g, 1.12 mmol) was dissolved in distilled water (5 mL) in a plastic beaker and reacted with two equivalents of silver nitrate (0.381 g, 2.24 mmol) producing the precipitation of the highly sensitive orange powder of silver 5,5′-azotetrazolate. The reaction mixture was stirred for ca. 15 min. in darkness and the solvent was then decanted, the silver salt was washed with a small amount of distilled water (2 times) and the water was eliminated by washing with methanol (2 times). Then, the orange solid was redissolved in fresh methanol (5 mL) and added to a solution of 1 (0.325 g, 2.24 mmol) in the same solvent (5 mL). Immediate precipitation of silver chloride took place and the reaction mixture was stirred in darkness. After 1.5 h, the insoluble materials were filtered, washed with fresh methanol and the solvent was rotavaporated, leaving behind the title compound as a yellow powder, which was purified by ether diffusion into a methanolic solution of the compound (0.401 g, 93%).
Method 2 (using barium 5,5′-azotetrazolate): This is a safer procedure, which avoids the use of highly sensitive silver 5,5′-azotetrazolate that can be used as an alternative: Barium 5,5′-azotetrazolate pentahydrate (0.627 g, 1.19 mmol) was suspended in distilled water (10 mL) and reacted with an equivalent amount of bis[1-(chloromethyl)-1,1-dimethylhydrazinium] sulphate (0.373 g, 1.19 mmol). The reaction mixture was stirred for 1.5 h at room temperature and the insoluble materials were filtered through a plug of Celite (previously washed with distilled water), washed with water and the filtrate was rotavaporated to dryness providing the crude material (0.442 g, 97%). Purification proceeded by ether diffusion into a methanolic solution of the compound. This procedure afforded X-ray quality single crystals. ρ (X-ray) = 1.521 g cm−3; C8H20N14Cl2 (MW = 383.24 g mol−1, calc./found): C 25.07/25.38, H 5.26/5.02, N 51.17/50.84; DSC (5 °C min−1): >172 °C (dec.); m/z (+c ESI, H2O): 109.0 (52, [Cat]), 492.9 (100, [Cat3A]), 876.2 (32, [Cat5A2]), 1259.5 (14, [Cat7A3]); m/z (–c ESI): 164.8 (18, [A2− + H+]−), 272.8 (11, [CatA]−), 655.2 (54, [Cat3A2]−), 1039.3 (100, [Cat5A3]−); 1H NMR (DMSO-d6, 400.18 MHz, TMS) δ (ppm): 3.41 (6 H, s, –CH3), 5.57 (2 H, s, –CH2Cl), 6.59 (2 H, s(br), –NH2); 13C 1H NMR (DMSO-d6, 100.52 MHz, TMS) δ (ppm): 173.19 (2 C, [C2N10]2−), 72.08 (1 C, –CH2Cl), 53.51 (2 C, N–CH3); Raman v˜/cm−1 (rel. int.): 3036(3), 2971(2), 2882(1), 2421(1), 1476(52), 1402(14), 1371(100), 1342(3), 1296(1), 1183(3), 1152(1), 1072(12), 1050(17), 918(8), 887(2), 811(2), 741(3), 371(1), 320(1); IR v˜/cm−1 (golden gate, rel. int.): 3276(m) 3197(m) 3075(m) 3029(m) 2322(w) 2194(w) 2037(w) 1958(w) 1656(w) 1466(m) 1435(w) 1419(w) 1406(w) 1378(m) 1347(w) 1336(w) 1303(w) 1244(w) 1180(w) 1165(w) 1149(w) 1123(w) 1095(w) 1067(w) 1050(w) 1034(w) 1025(w) 1006(w) 981(w) 947(w) 922(w) 890(s) 803(s) 772(m) 742(w) 720(s) 666(w) 596(w) 583(w) 554(m) 531(m) 509(m) 494(w) 487(w) 471(w).
[(CH3)2N(CH2Cl)NH2][(NO2)3Ph–O] (8).
Barium picrate hexahydrate (1.401 g, 2.00 mmol) was dissolved in distilled water (20 mL) and reacted with 6 (0.630 g, 2.00 mmol) for ca. 2 h. After this period the insoluble materials were filtered through a plug of Celite, which was washed with distilled water and the filtrates were rotavaporated to dryness leaving behind the crude product. Recrystallization from hot water yielded X-ray quality single crystals of the compound (0.572 g, 85%). ρ (X-ray) = 1.644 g cm−3; C9H12N5ClO7 (MW = 337.67 g mol−1, calc./found): C 32.01/31.94, H 3.58/3.42, N 20.74/30.43; DSC (5 °C min−1): 77.4 °C (m.p.), 208 °C (dec.); m/z (+c ESI): 109.0 (22, [Cat]+), 446.2 (57, [Cat2A]+), 783.4 (100, [Cat3A2]+), 1120.6 (6, [Cat4A3]+), 1457.8 (1, [Cat5A4]+); m/z (–c ESI): 228.1 (100, [A]−), 478.9 (10, [A2Na]−), 564.7 (18, [A2Cat]−), 729.7 (29, [A3Na2]−); 1H NMR (DMSO-d6, 400.18 MHz, TMS) δ (ppm): 3.33 (6 H, s, –CH3), 5.40 (2 H, s, –CH2Cl), 6.32 (2 H, s(br), –NH2), 8.59 (2 H, s, Ar–H); 13C 1H NMR (DMSO-d6, 100.52 MHz, TMS) δ (ppm): 160.83 (1 C, C1), 141.78 (2 C, C2), 125.22 (2 C, C3), 124.28 (1 C, C4), 72.27 (1 C, –CH2Cl), 53.56 (2 C, N–CH3); Raman v˜/cm−1 (rel. int.): 3059(3), 2994(4), 2979(2), 1608(2), 1560(13), 1493(6), 1431(1), 1366(54), 1345(67), 1334(75), 1311(100), 1298(87), 1253(41), 1165(9), 1079(6), 1006(2), 945(9), 911(4), 823(36), 792(4), 742(3), 710(2), 547(2), 499(2), 452(1), 371(3), 334(8), 295(4), 202(3); IR v˜/cm−1 (golden gate, rel. int.): 3299(w) 3132(w) 3100(w) 3078(w) 3057(w) 1634(m) 1609(m) 1564(m) 1553(m) 1509(m) 1485(m) 1427(m) 1365(m) 1333(s) 1311(s) 1261(s) 1164(m) 1117(w) 1079(m) 1004(w) 966(w) 935(w) 922(m) 912(m) 839(w) 820(w) 787(s) 743(m) 706(s) 636(w) 610(w) 573(w) 560(w) 514(w) 476(w).
[(CH3)2N(CH2Cl)NH2][H2N–CN4] (9).
Barium 5-amino-1H-tetrazolate tetrahydrate (0.762 g, 2.02 mmol) was reacted with 6 (0.637 g, 2.02 mmol) in distilled water (5 mL). The reaction mixture was stirred for ca. 30 min at room temperature and the insoluble barium sulphate was filtered through a plug of Celite. After washing the insoluble material the filtrates were combined and rotavaporated to dryness to obtain a pale yellow solid (0.733 g, 94%). ρ (17.6 °C, pycnometer) = 1.521 g cm−3; C4H12ClN7 (MW = 193.64 g mol−1, calc./found): C 24.81/24.54, H 6.25/6.40, N 50.63/50.24; DSC (5 °C min−1): 64.2 °C (m.p.), >165 °C (dec.); m/z (+c ESI): 109.0 (100, [Cat]+), 217.9 (25, [2Cat+–H+]+), 253.0 (64, [2Cat+ + Cl−]+), 301.9 (45, [2Cat+ + A−]+); m/z (–c ESI): 84.0 (100, [A]−); 1H NMR (DMSO-d6, 400.18 MHz, TMS) δ (ppm): 3.37 (6 H, s, –CH3), 5.52 (2 H, s, –CH2Cl), 6.53 (4 H, s(br), –NH2); 13C 1H NMR (DMSO-d6, 100.52 MHz, TMS) δ (ppm): 156.89 (1 C, C–NH2), 71.93 (1 C, –CH2Cl), 53.34 (2 C, N–CH3); Raman v˜/cm−1 (rel. int.): 3036(25), 2971(98), 2831(17), 2431(2), 1643(4), 1518(3), 1444(9), 1270(5), 1215(6), 1126(4), 1058(12), 971(4), 893(5), 806(4), 744(100), 527(3), 470(4), 435(7), 355(7), 240(2), 217(2); IR v˜/cm−1 (golden gate, rel. int.): 3115(m) 3031(m) 2965(w) 2760(w) 2159(vw) 2022(vw) 1951(vw) 1637(s) 1515(m) 1470(m) 1369(w) 1266(w) 1096(m) 1062(m) 1002(w) 957(m) 892(m) 802(m) 742(m) 716(w) 676(w) 651(w) 609(w) 597(w) 559(w).
Reaction of methylhydrazine with dichloromethane (formation of [MeNH–NH3]Cl)
98% methylhydrazine (0.50 mL, 9.31 mmol) was added to dichloromethane (5.0 mL, 78.12 mmol) under a stream of nitrogen. The resulting solution was then stirred for ca. 44 h resulting in the formation of insoluble droplets over the dichloromethane surface. The solvent was then decanted under a stream of nitrogen resulting in the formation of a white solid, which was washed with fresh dichloromethane and dried under high vacuum. The NMR and the melting point of the resulting compound to the same as that of methylhydrazinium chloride (0.632 g, 82%). DSC (5 °C min−1): 72 °C (m.p.). ca. 175 °C (slow dec.), 245 °C (rash dec.); 1H NMR (DMSO-d6, 400.18 MHz, TMS) δ (ppm): 2.56 (3 H, s, –CH3), 7.48 (4 H, s(br), NH); 13C 1H NMR (DMSO-d6, 100.52 MHz, TMS) δ (ppm): 37.05 (N–CH3); 35Cl NMR (DMSO-d6, 49.03 MHz, NaCl) δ (ppm): 70.0 (Cl−).
Conclusion
Salts of the [(CH3)2N(CH2Cl)NH2]+ cation containing energetic anions (i.e., nitrate, perchlorate, azide, dicyanamide, 5,5′-azobistetrazolate, picrate and 5-amino-1H-tetrazolate) were synthesized by metathesis reactions starting either from [(CH3)2N(CH2Cl)NH2]Cl (1) or [(CH3)2N(CH2Cl)NH2]2[SO4] (6). The new compounds were characterized by spectroscopic and analytical methods and the solid state structure of the [(CH3)2N(CH2Cl)NH2]+ cation was solved by low temperature X-ray diffraction analysis. The structures of compounds 3, 6, 7, 8 and Ba[(NO2)3Ph–O]2·6H2O were fully described with particular attention being paid to the hydrogen-bonding networks (graph-set analysis). In addition, standard BAM tests were used to determine the energetic properties of the compounds and the thermal stabilities of the materials were assessed by differential scanning calorimetry (DSC). All of the salts described in this report were insensitive to impact, friction and electrostatic discharge and salts 2–4, 8 and 9 fall within the category of ionic liquids (ILs, i.e. m.p. < 100 °C). Lastly, the detonation parameters and specific impulses of the compounds and oxygen-balanced formulations with ammonium nitrate and ammonium dinitramide were predicted using the EXPLO5 software. Further work will focus on the design of systems containing the ILs presented in this work and strong oxidizers such as white fuming nitric acid (WFNA) in view to determining the potential hypergolic properties of these mixtures. The results of these studies will be subject to a future publication.
Acknowledgements
We are grateful to Centre National de la Recherche Scientifique and the University Claude Bernard of Lyon for their financial support. The authors would also like to thank Mr. Philippe Larochette for his help with proof-reading the manuscript.
References
-
(a) C. Nonnenberg, I. Frank and T. M. Klapötke, Angew. Chem., 2004, 116, 4686–4689 CrossRef;
(b)
Angew. Chem. Int. Ed., 2004, 43, 4586–4589 Search PubMed.
- L. Catoire, N. Chaumeix and C. Paillard, J. Propul. Power, 2004, 20, 87–92 Search PubMed.
- L. Catoire, N. Chaumeix, S. Pichon and C. Paillard, J. Propul. Power, 2006, 22, 120–126 Search PubMed.
- A. Osmont, L. Catoire, T. M. Klapötke, G. L. Vaghjiani and M. T. Swihart, Propellants, Explos., Pyrotech., 2008, 33, 209–212 Search PubMed.
-
(a) M. Tanaka, W. Daimon and I. Kimura, J. Propul. Power, 1985, 1, 314–316 Search PubMed;
(b) W. Daimon, Y. Gotoh and I. Kimura, J. Propul. Power, 1991, 7, 946–952 Search PubMed.
- S. Pichon, L. Catoire, N. Chaumeix and C. Paillard, J. Propul. Power, 2005, 21, 1057–1061 Search PubMed.
- International Conference on Green Propellant for Space Propulsion, 20–22 June 2001, Noordwijk, The Netherlands: http://esamultimedia.esa.int/conferences/01a05/index.html..
-
(a) H. Gao, Y.-H. Joo, B. Twamley, Z. Zhou and J. M. Shreeve, Angew. Chem., 2009, 121, 2830–2833 CrossRef;
(b)
Angew. Chem. Int. Ed., 2009, 48, 2792–2795 Search PubMed.
-
V. Forquet, C. Darwich, C. Miró Sabaté and H. Delalu, New Trends in Research of Energetic Materials, Proceedings of the Seminar, 13th, Pardubice, Czech Republic, 2010 Search PubMed.
- Y. Zhang, H. Gao, Y. Guo, Y. H. Joo and J. M. Shreeve, Chem.–Eur. J., 2010, 16, 3114–3120 CrossRef CAS.
- A. Xia and P. R. Sharp, Inorg. Chem., 2001, 40(16), 4016–4021 CrossRef CAS.
-
(a)
H. Delalu, D. C. Colas, J. Berthet and P. Leurent, (Isochem, Fr.; Centre National De La Recherche Scientifique CNRS; Universite Claude Bernard Lyon I), 2005, FR 2864081;
(b) C. Darwich, M. Elkhatib, G. Steinhauser and H. Delalu, Kinet. Catal., 2009, 50(1), 103–110 Search PubMed;
(c) V. Goutelle, V. Pasquet, J. Stephan, A. J. Bougrine and H. Delalu, Int. J. Chem. Kinet., 2009, 41(6), 382–393 Search PubMed;
(d) J. Molinet, V. Pasquet, A. J. Bougrine and H. Delalu, Kinet. Catal., 2009, 50(5), 715–724 Search PubMed.
- Z. Janas, L. B. Jerzykiewicz, R. L. Richards and P. Sobota, Inorg. Chim. Acta, 2003, 350, 379–386 Search PubMed.
- A. D. Becke, J. Chem. Phys., 1993, 98, 5648 CrossRef CAS.
- C. Lee, W. Yang and R. G. Parr, Phys. Rev., 1988, B37, 785.
- Gaussian: 03W Program, Gaussian Inc., Wallingford, CT, 2004.
- A. P. Scott and L. Radom, J. Phys. Chem., 1996, 100, 16502 CrossRef CAS.
- P. Mani, H. Umamaheswari, B. D. Joshua and N. Sundaraganesan, THEOCHEM, 2008, 863, 44–49 CrossRef CAS.
-
(a) K. Karaghiosoff, T. M. Klapötke, P. Mayer, C. Miró Sabaté, A. Penger and J. M. Welch, Inorg. Chem., 2008, 47, 1007–1019 CrossRef CAS;
(b) J. C. Gálvez-Ruiz, G. Holl, K. Karaghiosoff, T. M. Klapötke, K. Loehnwitz, P. Mayer, H. Nöth, K. Polborn, C. J. Rohbogner, M. Suter and J. J. Weigand, Inorg. Chem., 2005, 44, 4237 CrossRef CAS.
-
N. B. Colthup, L. H. Daly and S. E. Wiberley, in Introduction to Infrared and Raman Spectroscopy, Academic Press, Boston, USA, 1990 Search PubMed.
-
R. G. Parr, W. Yang, in Density Functional Theory of Atoms and Molecules, Oxford University Press, New York, USA, 1989 Search PubMed.
-
E. D. Glendening, A. E. Reed, J. E. Carpenter and F. Weinhold, NBO Version 3.1, as Implemented in Gaussian 03, Revision, D.01, Gaussian, Inc., Wallingford, CT, 2004 Search PubMed.
-
(a) M. C. Etter and J. C. MacDonald, Acta Crystallogr., Sect. B: Struct. Sci., 1990, B46, 256–262 CrossRef CAS;
(b) J. Bernstein, R. E. Davis, L. Shimoni and N. L. Chang, Angew. Chem., 1995, 107, 1689–1708;
(c)
Angew. Chem. Int. Ed. Eng.
1995
34
1555–1573
Search PubMed.
- RPLUTO, Cambridge Crystallographic Data Centre, Cambridge, UK, 2000, http://www.ccdc.cam.ac.uk/suport/documentation/rpluto/TOC.html..
- J. A. Pople, R. Seeger and R. Krishnan, Int. J. Quantum Chem., 1977, 11, 149 Search PubMed.
- A. K. Rick, T. H. Dunning and J. H. Robert, J. Chem. Phys., 1992, 96, 6796 CrossRef CAS.
- A. P. Kirk, E. W. David and T. H. Dunning, J. Chem. Phys., 1994, 100, 7410 CrossRef CAS.
- C. Darwich, T. M. Klapötke and C. Miró Sabaté, Chem.–Eur. J., 2008, 14, 5756 CrossRef CAS and references therein..
-
http://www.bam.de;
http://www.reichel-partner.de
.
-
(a) NATO: standardization agreement (STANAG) on explosives, impact sensitivity tests, no. 4489, Ed. 1, Sept. 17, 1999;
(b) NATO: standardization agreement (STANAG) on explosive, friction sensitivity tests, no. 4487, Ed. 1, Aug. 22, 2002.
- WIWEB-Standardarbeitsanweisung: 4-5.1.03, Ermittlung der Explosionsgefährlich oder der Reibeempfindlichkeit mit dem Reibeapparat, Nov. 8, 2002.
- Impact: Insensitive >40 J, less sensitive ≥35 J, sensitive ≥4 J, very sensitive ≤3 J; friction: Insensitive >360 N, less sensitive = 360 N, sensitive <360 N a. >80 N, very sensitive ≤80 N, extreme sensitive ≤10 N. According to the UN Recommendations on the Transport of Dangerous Goods (+) indicates: not safe for transport.
-
J. Köhler and R. Meyer, in Explosivstoffe, Wiley-VCH, Weinheim, Germany, 1998, 9th edn Search PubMed.
- M. Hiskey, A. Hammerl, G. Holl, T. M. Klapötke, K. Polborn, J. Stierstorfer and J. J. Weigand, Chem. Mater., 2005, 17, 3784–3793 CrossRef CAS.
- T. M. Klapötke, C. Miró Sabaté and J. Stierstorfer, Z. Anorg. Allg. Chem., 2008, 11, 1867–1874 CrossRef.
- A. Hammerl, T. M. Klapötke, H. Nöth, M. Warchhold, G. Holl and M. Kaiser, Inorg. Chem., 2001, 40, 3570 CrossRef CAS ; DE Pat. 014 044, 2004.
-
(a) H. Gao, C. Ye, O. D. Gupta, J. C. Xiao, M. A. Hiskey, B. Twamley and J. M. Shreeve, Chem.–Eur. J., 2007, 13, 3853 CrossRef CAS;
(b) Z. Zeng, H. Gao, B. Twamley and J. M. Shreeve, J. Mater. Chem., 2007, 17, 3819 RSC;
(c) C. Ye, H. Gao, B. Twamley and J. M. Shreeve, New J. Chem., 2008, 32, 317 RSC;
(d) Y. H. Joo, B. Twamley, S. Garg and J. M. Shreeve, Angew. Chem., Int. Ed., 2008, 47, 6236 CrossRef CAS.
-
(a) T. M. Klapötke and J. Stierstorfer, Helv. Chim. Acta, 2007, 90, 2132 CrossRef;
(b) G. Geisberger, T. M. Klapötke and J. Stierstorfer, Eur. J. Inorg. Chem., 2007, 4743 CrossRef CAS;
(c) T. M. Klapötke and C. Miró Sabaté, Z. Anorg. Allg. Chem., 2007, 633, 2671 CrossRef;
(d) M. Göbel and T. M. Klapötke, Acta Crystallogr., Sect. C: Cryst. Struct. Commun., 2007, C63, o562 Search PubMed;
(e) T. M. Klapötke, H. Radies and J. Stierstorfer, Z. Naturforsch., 2007, 62b, 1343 Search PubMed;
(f) T. M. Klapötke and C. Miró Sabaté, Chem. Mater., 2008, 20, 3629 CrossRef;
(g) M. Göbel and T. M. Klapötke, Acta Crystallogr., Sect. C: Cryst. Struct. Commun., 2008, C64, o58 Search PubMed.
-
(a) M. Sućeska, Propellants, Explos., Pyrotech., 1991, 16, 197 CrossRef CAS;
(b) M. Sućeska, Propellants, Explos., Pyrotech., 1999, 24, 280 CrossRef CAS.
-
J. Akhavan, in The Chemistry of Explosives, RSC Paperbacks, Cambridge, UK, 2004, 2nd edn Search PubMed.
-
P. J. Linstrom and W. G. Mallard, NIST Chemistry WebBook, NIST Standard Reference Database Number 69, National Institute of Standards and Technology, Gaithersburg, MD, June 2005, 20899, http://webbook.nist.gov. Search PubMed.
- H. Gao, C. Ye, C. M. Piekarski and J. M. Shreeve, J. Phys. Chem., 2007, C111, 10718 Search PubMed.
- T. M. Klapötke and C. Miró Sabaté, Z. Anorg. Allg. Chem., 2010, 636, 163–175 Search PubMed.
- A. K. Sikder and N. Sikder, J. Hazard. Mater., 2004, A112, 1 CrossRef.
- H. Gao, Z. Zeng, B. Twamley and J. M. Shreeve, Chem.–Eur. J., 2008, 14, 1282–1290 CrossRef CAS.
- Oxygen: balance for a compound with the formula CxHyOzClt: Ω (%) = −1600/MW (2x + (y − t)/2 − z); MW = molecular weight.
Footnote |
† Electronic supplementary information (ESI) available: complete cyrstal structure discussion, table of computed IR and Raman frequencies, NMR resonances table, X-ray tables, tables for formulations of compounds 2, 3, 4, 5, 7, 8 and 9 with AN and ADN, general method and synthesis of the starting materials. Additionally, the cif files of 3, 6, 7, 8 and Ba[(NO2)3Ph–O]2·6H2O have been deposited with the Cambridge Crystallographic Data Centre (CCDC). CCDC reference numbers 808540–808544. For ESI and crystallographic data in CIF or other electronic format see DOI: 10.1039/c2ra00968d |
|
This journal is © The Royal Society of Chemistry 2012 |