DOI:
10.1039/C2RA00892K
(Review Article)
RSC Adv., 2012,
2, 2621-2629
Three-component photoinitiating systems: towards innovative tailor made high performance combinations
Received
14th October 2011
, Accepted 21st December 2011
First published on 10th January 2012
Abstract
Three-component photoinitiating systems of radical and cationic polymerization are reviewed. The involved mechanisms are described. The design of new promising systems for the photopolymerization of low viscosity monomers under air and low intensity light sources (household lamps, LED bulbs, the sun, LEDs, diode lasers) in the 350–700 nm wavelength range suggest novel applications for Radiation Curing, Laser Imaging, optics, holography or medicine areas.
Introduction
Photopolymerization reactions are encountered in a lot a applications in various areas:1 radiation curing (varnishes and paints, coatings, adhesives, graphic arts), microlithography, stereolithography, microelectronics, laser imaging (writing of complex relief structures for the manufacture of microcircuits, direct writing on a printing plate in Computer-To-Plate technology), optics (manufacture of optical elements), holography (holographic recording and information storage), medicine (dentistry, ophthalmic products, drug microencapsulation), nanotechnology (structured materials on the nanoscale size, nanocomposites). Most of them correspond to photoinduced radical or cationic reactions. The photoinitiation step of such reactions requires the presence of a photoinitiating system, PIS, that has to generate radicals or acidic species. The practical efficiency of a given PIS is connected with the amount of light absorbed and its intrinsic reactivity.
Usually, in free radical photopolymerization, FRP, PIS are based on i) one-component systems (eqn 1): Type I PIs (photoinitiators) leading to a homolytic cleavage process from their excited singlet (1PI) or triplet (3PI) states and ii) two-component systems: Type II PIs consisting of either a PI and a hydrogen donor DH (eqn 2) (such as an amine, a thiol or more recently a silane or ligated borane; the reaction can be a pure hydrogen transfer or an electron/proton transfer) or a PI and a photosensitizer, PS, e.g. through energy transfer (eqn 3). In photoinduced cationic photopolymerization (CP), one-and two-component systems are used: PI alone e.g. onium salts On+ (eqn 4) or PI/PS (eqn 5). Two-component systems (radical source RSo/On+) are usually involved in free radical promoted cationic photopolymerization FRPCP (eqn 6).
| PI (hν) → 1,3PI → R• →→→ R–Mn• | (1) |
| PI + DH → PIH• + D• (hν) | (2a) |
| PI + DH → [PI+• DH−•] (hν) and [PI+• DH−•] → PIH• + D• | (2b) |
| PS (hν) → 1,3PS; 3PS + PI → PS + 3PI; 3PI → R• | (3) |
| PI (hν) → 1,3PI → C+ →→→ C–Mn+ | (4) |
| PS (hν) → 1,3PS ; 1,3PS + PI →→ C+ | (5) |
| RSo (hν) →→ R• and R• + On+ → R++ On• | (6) |
In the past thirty years, however, many three (or even four)-component systems have been proposed to achieve higher performances than those obtained for Type I and Type II systems. Typical advantages are i) a better absorption of PIS, ii) an enhanced photochemical and chemical reactivity, iii) a higher practical efficiency (in terms of polymerization rates and conversions), iv) the possibility for either a tunable absorption through the choice of a well deigned class of PI or a selective excitation at a given laser line, v) the promising design of systems less sensitive to oxygen, vi) the development of dual radical/cationic PISs, vii) the proposal of photoinitiators behaving as photocatalysts. Disadvantages might be: i) the presence of more than one or two compounds, ii) the cost and eventual toxicity of some selected chemicals (although this is not only related to three-component systems), iii) the compatibility of the added compounds (e.g. an amine and an iodonium salt), iv) a higher complexity of the photoinitiating system (rather complex chemical mechanisms leading to the formation of the initiating species are usually involved)…
Through selected examples of applications, the present paper will first review the historically proposed three-component systems for different traditional and high-tech application sectors and then the new trends for the development of tailor made systems being able to meet the challenge for new innovative end-uses.
Typical compounds used as the absorbing compounds (and referred hereafter as PI) in three-component systems are depicted in Chart 1.
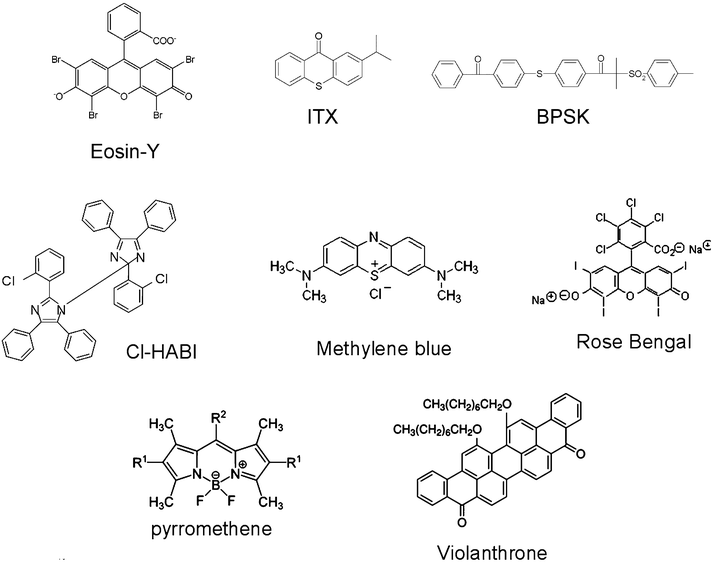 |
| Chart 1 | |
Table 1 gathers some interesting representatives of three-component photoinitiating systems together with examples of applications. The encountered mechanisms will be schematically presented hereafter in a condensed manner; more details are available in the cited relevant literature.
Table 1 Examples of three-component photoinitiating systems and areas where they can be used. See text
Photoinitiating system |
UV Curing |
Imaging, optics, holography, microelectronics, printing plates, Computer-to-Plate, Laser Direct Imaging |
film photopolymerization (coating), a1: drying of printing inks.
laser induced photopolymerization.
adhesives.
paints.
holographic recording.
Computer-to-plate systems.
3D photopolymerization.
direct laser imaging in microelectronics.
film photopolymerization in aerated media.
sunlight induced photopolymerization.
photopolymerization under household and LED bulb exposure.
green and red diode laser induced photopolymerization.
|
Benzophenone/CBr4/Ph2I+ |
X a |
|
Isopropyl thioxanthone/CBr4/Ph2I+ |
X a |
|
Benzophenone/amine/maleimide |
X a |
|
Isopropyl thioxanthone/Benzophenone sulfonyl ketone/amine |
X a |
X a |
Methylene Blue/amine/cobalt salt |
X a |
|
Cyanine dye/borate salt/triazine derivative |
X c |
X b |
Carbocyanine dye/borate salt/alkoxypyridinium salt |
|
X b |
Styrylquinolinium dye/borate salt/triazine derivative |
|
X b |
Rose Bengal/Ferrocenium salt/hydroperoxide |
X d |
|
Rose Bengal/phosphine oxide derivative/cobalt complex |
X d |
|
Eosin/amine/diphenyl iodonium salt |
X a |
X e |
Camphorquinone/alcohol/diphenyl iodonium salt |
X a |
|
Ketocoumarin/amine/diphenyl iodonium salt |
|
X ef |
Methylene Blue/amine/diphenyl iodonium salt |
X a |
X |
Merocyanine/amine/bromocompound |
|
X |
Thioxanthene dye/amine/diphenyl iodonium salt |
|
X e |
Fluorone dye/amine/diphenyl iodonium salt |
|
X g |
Rose Bengal/amine/triazine derivative |
X a |
X |
Methylene Blue/benzophenone/amine |
X a |
|
Pyrromethene dye/amine/triazine derivative |
X a |
|
Styrylnaphtothiazole dye/Cl-HABI/thiol |
|
X e |
Julolidine dye/Cl-HABI/thiol |
|
X e |
Diethylamino benzophenone/Cl-HABI/thiol |
|
X h |
Benzophenone/amine/silane |
X i |
|
Benzophenone/amine/borane |
X i |
|
Benzophenone/amine/germane |
X i |
|
Metal carbonyl/silane/hydroperoxide |
X j |
|
Benzophenone/silane/diphenyl iodonium salt |
X i |
|
Thiopyrilium salt/silane/diphenyl iodonium salt |
X ij |
|
Metal carbonyl/silane/diphenyl iodonium salt |
X ij |
|
Ru or Ir complex/silane/diphenyl iodonium salt |
X ik |
|
Violanthrone/silane/diphenyl iodonium salt |
|
X l |
Thirty years of development of three-component systems
In this section, the encountered three-component systems are reviewed as a function of a given application. They are very often used in the design of high speed photopolymers.2–9 The involved mechanisms will be presented. For the sake of clarity, the different components of the initiating system are given in bold in Scheme 1–14.
UV Curing area.
Free radical photopolymerization FRP.
In Type II PIs, the PIH• radical produced in (eqn 2) is known as a terminating radical of the growing polymer chain. As recalled in the well known and rather old (but still valid) mechanism shown in Scheme 1 (see ref. 19 and references therein) where the ketone/amine interaction results in electron transfer and the formation of the two radicals, the use of a third component called the additive and referred as SC (scavenging compound) which is able to scavenge PIH• allows the generation of new initiating radicals and an increase in the polymerization rate or the cure speed.
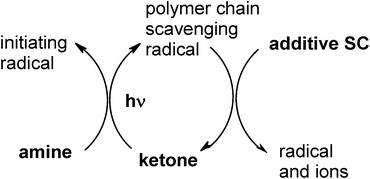 |
| Scheme 1 | |
Scheme 1 is typical of many PIS where the ketone is benzophenone or thioxanthone and the SC additive is a bromo compound (e.g. CBr4, bromo acetophenone or bromo phenylsulfone derivatives) or an iodonium salt (e.g. diphenyl iodonium hexafluorophosphate DPI also noted Ph2I+),10 a maleimide, maleic anhydride or an arylnaphtalimide.11–13 The aminoalkyl radical D• is generated in eqn (2) as well as the tribromomethyl radical when using CBr4 (or the phenyl radical when using an onium salt) formed in eqn (7) as the initiating radicals. A two fold increase of radical polymerization rate (Rp) is noted in acrylate film photopolymerization upon addition of SC to the BP/bromo compound couple.
| PIH• + CBr4 → PI + H+ + CBr3• + Br− | (7a) |
| PIH• + Ph2I+ → PI + H+ + Ph• + PhI | (7b) |
The ketone/ketone/amine combination is another example: in isopropylthioxanthone ITX/benzophenone sulfonylketone BPSK/amine, a predominant ITX/amine electron transfer reaction and a ketyl radical quenching (by BPSK which plays the role of SC) occur as above in Scheme 1 (an energy transfer between ITX and BPSK can contribute to some extent).14 Rates of polymerization of an acrylate matrix are in the ratio 1
:
2
:
15 in the presence of ITX/BPSK, BPSK/MDEA and ITX/BPSK/MDEA, respectively (MDEA stands for methyl diethanolamine).
The dye (methylene blue MB)/amine/cobalt salt (Co acetylacetonate, hexammine cobalt) systems have also been described15 and involve a different mechanism. A redox cycle reaction between the reduced leuco dye, the cobalt salt and O2 allows the oxygen consumption. Photoassisted oxypolymerization of alkyd resins was achieved in the presence of Rose Bengal/phosphine oxide derivative/cobalt complex;16 the mechanism is not really clear.
The polymethine dye/borate salt/1,3,5-triazine derivative TZ system has been proposed for the photocrosslinking of pressure sensitive adhesives.17 It likely works according to Scheme 2. Radicals are formed through electron transfer between the dye and the borate anion, cleavage of the formed boranyl radical RBX3• (which yields a borane BX3 and an initiating alkyl radical R•) and dye radical Dye•/TZ electron transfer (which produces an additional initiating triazinyl radical).
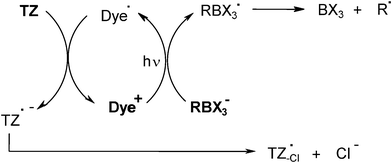 |
| Scheme 2 | |
Systems based on Rose Bengal RB/ferrocenium Fc(+) salt/cumene hydroperoxide allow the curing of maleimide–vinylether and maleimide–allylether formulations.18 The addition of a fourth component to such a system still enhances the efficiency. For example, the RB/ferrocenium salt Fc(+)/hydroperoxide/amine combination is particularly efficient in the curing of thick and heavily pigmented acrylate paints in an industrial UV drying line. Final coating thicknesses of 150–400 μm (depending on the pigment)19 were polymerized. A set of reactions has been identified20,21 (Scheme 3). The primary dye RB/ferrocenium salt interaction upon light excitation leads to the reduced Fc(0) structure which behaves as a 17, 18 or 19 electron species. The subsequent dark reactions of Fc(0) with the amine, the hydroperoxide and oxygen as well as the formation of Fe2+ (and then Fe3+) lead to various initiating radicals and presumably explain the polymerization in the core of the film where almost no light penetrates.
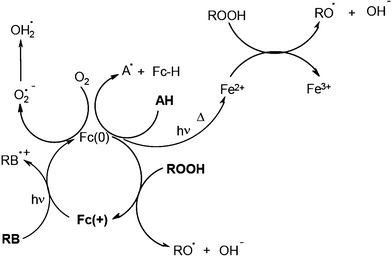 |
| Scheme 3 | |
Free radical promoted cationic photopolymerization FRPCP.
Visible light photosensitive three-component systems (e.g. the xanthenic dye Xan/amine/diphenyl iodonium (DPI) system)22 for FRPCP allows a longer wavelength photosensitivity (compared to the available range in the presence of amine/DPI) and have been currently proposed for a long time. Such a system works according to Scheme 1. The radicals (aminoalkyl and Xan-H•) are formed after electron/proton transfer as in eqn (2b). DPI scavenges the Xan-H• radicals (eqn 8). The same mechanism holds true in benzophenone/dimethylaniline/DPI, thioxanthone fluorene carboxylic acid (or thioxanthone carbazole)/hydrogen donor/DPI,23 dimanganese decacarbonyl/alkyl halide/DPI.24 | Xan-H• + On+ → R++ On• | (8) |
Introducing a photoinitiator in the DPI/alcohol ROH combination leads to an interesting and efficient system for visible light induced FRPCP that also works according to a similar mechanism as in Scheme 1 (eqn 9): The alcohol effects on the propagation step can also contribute to some extent. Suitable PIs are camphorquinone25 or a titanium complex.26
| PI + ROH → PI–H• + R–HOH• (hν) and PI–H• + On+→ PI + H+ + On• | (9) |
Other interesting systems will be described below.
Printing plates, optics, holography, computer-to-plate systems, laser direct imaging
All the following systems are operating in FRP. The ketocoumarin KC/amine (N-phenyl glycine NPG)/DPI system27 was used for the photopolymerization reaction of an acrylate matrix upon Ar+ laser exposure at 488 nm. Photosensitivities of 0.2, 0.9 and 1.2 mJ cm−2 were obtained in the presence of the KC/NPG/DPI, KC/DPI and KC/NPG combinations, respectively. Holograms can also been recorded. The mechanism follows Scheme 1 (with ketone = KC, SC = DPI).
Other systems developed for (laser) printing plates involve Eosin Eo/amine (MDEA)/DPI,28 Eo/α-keto-O-acyloxime derivative (PDO)/DPI,28b methylene blue MB/amine/onium salt,29,30 merocyanine (or safranine)/amine/bromo compound (or onium salt),31,32 thioxanthene dye (TXD)/amine (NPG)/DPI (or bromo compound),33 safranine/amine/DPI,34 fluorone dye/amine/DPI,35 acridinium cation Acr+/dihydropyridine pyH2/Ph2I+.36 For example, photosensitivities of 0.3, 1.4 and 1.8 mJ cm−2 were obtained under exposure of a filtered Xe lamp at 490 nm in the presence of the TXD/NPG/DPI, TXD/NPG and TXD/DPI combinations, respectively. Under Ar+ laser exposure at 488 nm, relative photosensitivities were in a ratio of i) 1/5 > 30 for the Eo/MDEA/DPI, Eo/DPI and Eo/MDEA combinations, respectively and ii) 1/1.6/5 for the Eo/MDEA/PDO, Eo/MDEA and Eo/PDO systems, respectively. Most of these PIS work according to Scheme 1 (ketone = Eosin, TXD, methylene blue, etc; SC = DPI, bromo compound, PDO). In the last mentioned Acr+/pyH2/Ph2I+ system, the mechanism is more complicated (Scheme 4) as the dihydropyridine radical cation pyH2+− yields a dihydropyridyl radical pyH2•; both the acrydinyl radical Acr• and pyH2• are scavenged by DPI; and the phenyls are the initiating radicals.
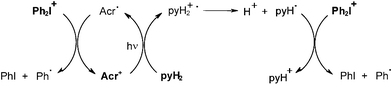 |
| Scheme 4 | |
In dye/amine/triazine derivatives, the mechanism is still basically similar to Scheme 1 (e.g. when using Rose Bengal RB):37,38 Dye-H• and A• formation with Dye-H• quenching by TZ. It becomes, however, more complex due to the additional dye/Tz electron transfer (Scheme 5). Eosin, erythrosine, acriflavine, acridine orange, safranin, phenosafranin or fluorone derivatives37,38 and pyrromethene dyes39 have also been tested. Excellent results were achieved in acrylate photopolymerization under visible lights. The cyanine dye/butyltriphenylborate anion/triazine halogenated derivative40 (see below) also leads to successful polymerization reactions. The mechanism is described by Scheme 2.
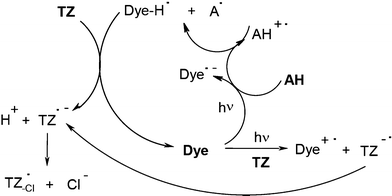 |
| Scheme 5 | |
Dye/ketone/amine combinations (where the dye is crystal violet, phenosafranine, Methylene Blue, thiopyronine and the ketone is acetophenone, benzophenone, thioxanthone, 4,4′-bis-dimethylamino benzophenone) were reported many years ago. The dye/ketone interaction is quite complex and relatively unclear.41
In coumarin C (or ketocoumarin KC)/amine (cyano-N-phenyl glycine CPG)/ferrocenium salt Fc(+) systems, Fc(+) plays a crucial role. The mechanism is also different and rather complex.42,43 It presumably involves (as shown in Scheme 6), a C (or KC)/Fc(+) electron transfer yielding Fc(0), a Fc(0)/amine interaction and dark by-side reactions (as in Scheme 3). Interesting photosensitivities for an acrylate formulation under exposure at 490 nm were achieved: they are in a relative ratio 1/2/30 for the C/CPG/Fc(+), C/Fc(+) and C/NPG combinations, respectively. The ratio becomes 1.4/2.8/14 when using the KC/CPG/Fc(+), KC/Fc(+) and KC/NPG systems.
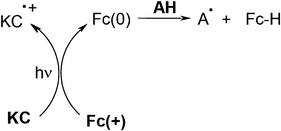 |
| Scheme 6 | |
An efficient four-component system (Eosin Eo/titanocene derivative Ti/triazine derivative TZ/CBr4) has also been proposed without any mechanistic support.44 Photosensitivities under Ar+ laser exposure at 488 nm were in a relative ratio 1/2.2/3.3/4.4 for the Eo/Ti/TZ/CBr4, Eo/Ti/TZ, Ti/TZ/CBr4 and Ti/TZ combinations, respectively.
A somewhat qualitatively close mechanism (similar to Scheme 2) occurs in a variety of dye borate salt ion pairs in the presence of an additive (whose chemical nature has an influence on the involved reactions). Many structures have been synthesized (see reviews in ref. 45 and 46). Improvements of the photosensitive formulations are continuously provided as exemplified in the carbocyanine/butyltriphenylborate RBX3−/methyl picolinium salt,47 styrylbenzothiazolinium derivative/butyltriphenylborate/alkoxypyridinium salt,48 styrylquinolium iodide/borate/N-alkoxypyridinium salt,49 cyanine dye/borate salt/heteroaromatic thiol systems.50 Primary radicals (R• and dye•) are formed from the borate moiety and the dye, respectively, as in Scheme 2: the dye•/additive (e.g. the picolinium or the alkoxypyridinium salt instead of the triazine derivative TZ) interaction leads to the production of additional radicals through electron transfer.
Photosensitizer PS/hexabisimidazole derivative (e.g. Cl-HABI abbreviated by L2)/additive systems were largely employed in the imaging technology area4 for Computer-To-Plate (CTP) printing plates, holographic recording, laser direct writing and patterning in microelectronics. Efficient PSs for the decomposition of Cl-HABI derivatives under exposure at 488 or 514 nm (Ar+ laser), 568 nm (Kr+ laser) or 632.8 nm (HeNe laser line) were based on julolidine derivatives JAW51 or diethylamino styryl naphto thiazole derivatives NAS.52,53 For example, lophyl radicals L• are generated through JAW/Cl-HABI electron transfer. A further L•/thiol RSH interaction leads to the initiating thiyl radical RS• (Scheme 7).
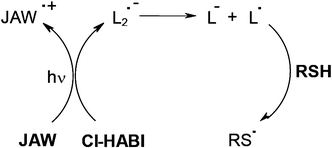 |
| Scheme 7 | |
For laser direct imaging LDI applications in the near UV/visible range (i and j lines of Hg lamps, blue LEDs, diode lasers), PS was preferably a ketone (e.g. diethyl amino benzophenone EAB), a coumarin or a ketocoumarin. The mechanisms were extensively described in refs. 54–56 and examples of photosensitivity at 365 nm are 110, 160 and 200 mJ cm−1 using Coumarin 1/Cl-HABI/thiol, 3,3′-carbonyl-bis-7-diethylamino coumarin/Cl-HABI/thiol and Cl-HABI/thiol, respectively. In EAB/Cl-HABI/thiol, the basic encountered reactions are similar to those shown in Scheme 7. In addition, regeneration of EAB occurs and new lophyls are created (Scheme 8).
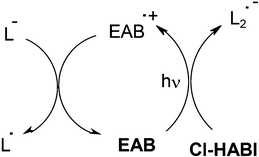 |
| Scheme 8 | |
Compared to EAB/NPG, the three-component EAB/NPG/DPI photoinitiating system leads to a 3-fold increase of RP in an acrylate formulation.57 The driving reactions observed in the EAB/Cl-HABI/amine AH system correspond to those of Scheme 7, 8 already shown and Scheme 9 where an additional usual EAB/amine interaction concomitantly occurs (then, a scavenging of the ketyl radicals by Cl-HABI is observed; new lophyls are formed).
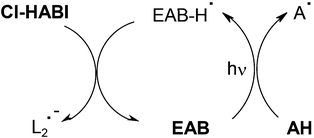 |
| Scheme 9 | |
Addition of Cl-HABI in the four-component combination (EAB/NPG/Cl-HABI/DPI) was also proposed. It still increases RP by ∼20%. In addition to the reactions of Scheme 7, 8 and 9, other reactions have been identified such as a competitive EAB/DPI electron transfer that generates phenyl radicals.
Design of tailor-made systems based on a new radical chemistry
Most of the above-mentioned systems are working in laminate, in highly viscous matrices and upon relatively high energy light sources. In recent years, significant progresses have been made for the design of three-component systems for FRP and FRPCP using low viscosity monomers in aerated media and under low light intensity exposure. This has opened up new doors for possible novel applications. These new systems require the presence of a silane (e.g. tris-(trimethylsilyl)silane TTMSS) or a germane derivative R′3Ge–H. Selected examples are the following.
Oxygen accelerated radical photopolymerization.
In FRP experiments, compared to benzophenone BP/amine, a significant enhanced efficiency for the photopolymerization of trimethylolpropane triacrylate TMPTA under air is noted when using the BP/amine/silane system.58 Similar behaviors are also encountered for other PIs based on isopropylthioxanthone, camphorquinone or Eosin-Y. This is due to the additional production of highly efficient initiating silyl radicals (Scheme 10) and to their ability to decrease the oxygen inhibition: the silyls consume oxygen and scavenge the peroxyls thereby leading to new initiating silyls. Germanes and boranes behave as silanes.
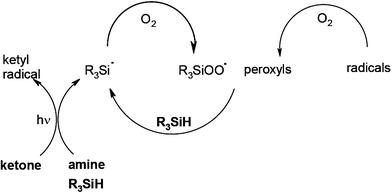 |
| Scheme 10 | |
Therefore, oxygen plays a positive role in FRP. The better ability of silanes compared to amines to overcome the oxygen inhibition is shown in Fig. 1 (curve b vs. curve c).
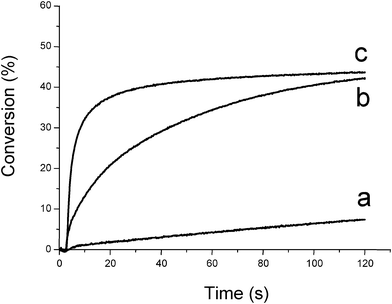 |
| Fig. 1 Conversion vs. time curves for the photopolymerization of trimethylolpropane triacrylate TMPTA under air as a function of the photoinitiator and additive (Type II system). (a) CQ (3% w/w); (b) CQ/EDB (3%/3% w/w); (c) CQ/TTMSS (3%/3% w/w); (diode laser at 473 nm) sample thickness = 20 μm. | |
Hydroperoxide accelerated radical photopolymerization.
The metal carbonyl/silane/cumene hydroperoxide CuOOH59 systems are also interesting. This led to an important improvement compared to the use of the previously proposed metal carbonyl/alkyl halides two-component systems.2 Metal centered radicals are generated (Met•) under light irradiation. They obey to the mechanism described in (eqn 10). The Met• radicals decompose the hydroperoxides. Further reactions with the silane leads to the formation of new polymerization initiating species. These systems are also worthwhile for photopolymerization in aerated conditions: i) all peroxides or hydroperoxides present in the medium are decomposed ii) new silyls are generated (as in Scheme 10) and iii) better polymerization profiles are obtained (Fig. 2). | PI → Met• (hν) and Met• + CuOOH → → CuO• | (10a) |
| CuO• + R′3X–H → R′3X• + CuO–H | (10b) |
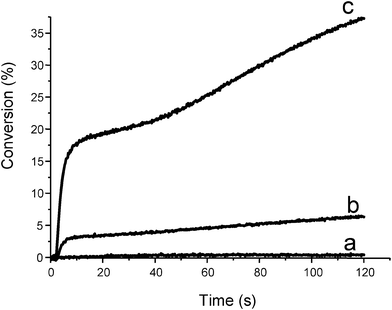 |
| Fig. 2 Photopolymerization profiles of trimethylolpropane triacrylate under air upon a Xe–Hg lamp irradiation (λ > 310 nm) in the presence of (a) Cp2Mo2(CO)6 (1% w/w); (b) Cp2Mo2(CO)6/CumOOH (1%/3% w/w); (c) Cp2Mo2(CO)6/CumOOH/TTMSS (1%/3%/3% w/w). | |
Long wavelength cationic photopolymerization under air.
Long wavelength photopolymerization under air becomes possible using PI/silane/DPI.60 Moreover, the low shell stability of the formulations in the presence of the above mentioned PI/amine/DPI systems compared to PI/silane/DPI is overcome. The suitable selection of PI obviously allows to easily tune the absorption from the near UV to the visible wavelength range and leads in any case to an active silyl radical R3Si• and then to the same initiating silylium R3Si+ cation as in eqn (6) where RSo stands for the PI/silane couple.
The interest of the PI/silane/DPI combination is supported by the following two points. First, in the two-component systems based on ketone/DPI (originally proposed a long time ago), a different structure R+ is generated when changing PI;61 this leads to a different reactivity for the epoxy ring opening or vinyl ether addition reactions. On the opposite, the same cationic structure R3Si+ is formed here using the PI/silane/DPI three-component system and whatever PI. Second, the presence of the silane also ensures a positive role of oxygen in FRPCP and allows a fast curing of an epoxide film under air (which cannot be attained when using the various PI/DPI systems as the formed radicals were quenched by O2).
Examples are illustrated by the various following systems: ketone/silane/DPI where the ketone is camphorquinone CQ, ITX, Eosin62 or acridinedione,63 ketone (= CQ, ITX…)/germane/DPI,64 thiopyrilium salt/silane (or disilane)/DPI,65,66 metal carbonyl compound (centred on Mn, Re, Fe, Cr, Mo, Ru)/silane/DPI,67,68 decatungstate/silane/DPI.69 The overall qualitative mechanism observed in ketone/R3SiH/Ph2I+ depicted in Scheme 11 can be easily understood from all the above mentioned considerations.
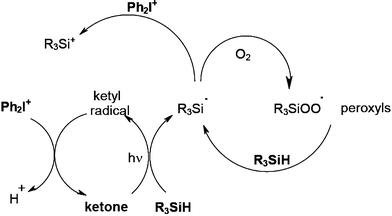 |
| Scheme 11 | |
Dual systems for simultaneous radical/cationic photopolymerization.
Other recently developed efficient cationic photoinitiating systems for FRPCP involve the organometallic chemistry. They are based on Ru(bpy)32+,70 Ru(phenanthroline)32+,71 Ir(ligand)372–74 in combination with a silane and DPI. The Ru or Ir complex/DPI interaction yields a phenyl radical which abstracts a hydrogen from a silane and generates a silyl. Then, the silyl reacts with DPI to form R3Si+ or the oxidized form of the complex to re-generate the starting complex (Scheme 12). These systems behave as dual radical/cationic PIs: both radicals and cations are produced. The manufacture of interpenetrated networks IPN might be feasible (in an acrylate/epoxide formulation, simultaneous conversions of ∼65% and > 50% for the double bond and epoxy ring content were reached upon a one step exposure to a visible light).
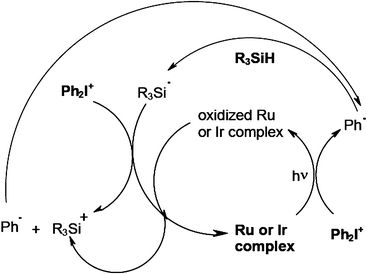 |
| Scheme 12 | |
Photoinitiators behaving as photocatalysts.
In Scheme 12, it appears that the Ru or Ir complexes are re-generated and as a consequence can be considered photocatalysts, the PI becoming a photoinitiator catalyst PIC. This is still a unique feature of particular three-component systems. Other systems involving pure organic PICs (anthracene derivatives) in an oxidation cycle as in Scheme 12, organometallic compounds (Ru complex/amine/phenacyl halide) or coloured structures (eosin/amine/phenacyl halide) working through a reduction cycle (Scheme 13) becomes to appear in radical photopolymerization.
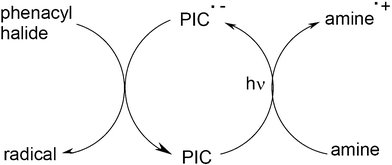 |
| Scheme 13 | |
Radical and cationic sunlight curing.
Several systems allow an efficient curing under soft conditions under air: sunlight, household fluorescent or LED lamp exposure. Using the Ru(phenanthroline)32+/TTMSS/Ph2I+ initiating system, a very efficient polymerization was observed under sunlight irradiation (Fig. 3). The formation of the polyether network is well evidenced by a new band at 1080 cm−1.
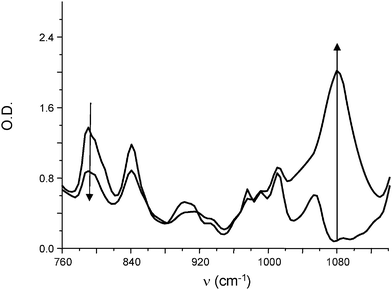 |
| Fig. 3 IR spectra recorded during the photopolymerization of (3,4-epoxycyclohexane)methyl 3,4-epoxycyclohexylcarboxylate in the presence of Ru(phenanthroline)32+/TTMSS/Ph2I+ (0.2%/3%/2% w/w). Sunlight irradiation (5 min). | |
In situ incorporation of Ir or Ru luminescent complexes in a photopolymerized matrix.
Due to the specific reactions in Ir complex/silane/DPI, the in situ incorporation of the Ir complex during the course of photopolymerization is readily observed: this could be of interest for the design of photoluminescent materials in OLED applications74 (the Ir complexes are well known as triplet emitters).
Green and red laser light induced cationic photopolymerization.
New systems based on violanthrone Vi (or ruthenium salt Ru, iridium salt Ir, Methylene Blue) derivative/silane/DPI allows a polychromatic light or laser diode irradiation.75 The mechanism is described by Scheme 12. These systems could be helpful for applications in optics (e.g. for holographic recording or the manufacture of optical elements) under green (488 and 514 nm) or red laser line (635 nm) excitation using cationic matrices that have the noticeable advantage of presenting a lower shrinkage than radical formulations (Fig. 4); other can work at 785 nm.
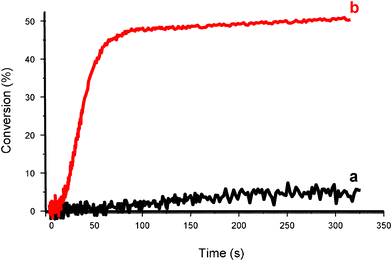 |
| Fig. 4 Polymerization profiles of (3,4-epoxycyclohexane)methyl 3,4-epoxycyclohexylcarboxylate under air upon a red diode laser irradiation (635 nm) in the presence of (a) Violanthrone-79/Ph2I+ (0.2%/2% w/w); (b) Violanthrone-79/Ph2I+/TTMSS (0.2%/2%/3% w/w). | |
Large UV/visible wavelength range photosensitized polymerization.
The Vi/Ru/silane/DPI four-component PIS still enhances the attained performance under a polychromatic irradiation. Both Vi and Ru absorbs the light thereby ensuring i) a quite good matching between the PIS absorption spectrum and the light source emission spectrum and ii) a more complete recovery of the UV/visible incident photons of the light source. In addition to the processes encountered in the Vi/silane/DPI and Ru/silane/DPI systems (same mechanism as in Scheme 12), particular Vi/Ru interactions (Scheme 14) lead to interesting new sources of phenyl radicals (through a primary Vi/Ru electron transfer) and excited violanthrone molecules (through Vi/Ru energy transfer).
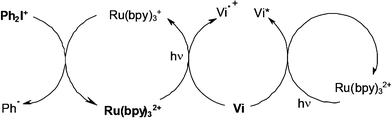 |
| Scheme 14 | |
Conclusions
The present paper clearly demonstrates that none of the attained photopolymerization efficiencies could have been obtained using any two-component systems instead of the three-component PIS. This outlines the huge improvement brought by all the reported strategies during more than thirty years aiming at developing more and more efficient systems through a careful selection of suitable interactions between the three compounds. Today, the recent input of the silyl (or germyl) chemistry should allow a decisive step for the design of high performance photopolymerizable materials in FRP and FRPCP which would be able to operate in severe experimental conditions (aerated media, exposure to sunlight and household fluorescent lamps, LED bulbs or diode lasers, low viscosity matrices, low film thicknesses) as well as under polychromatic UV and visible light Hg sources (or Xe, Hg–Xe, doped lamps), LED devices or diode laser arrays. In our opinion, however, there is still a place for other innovative chemistries involving multi-component systems. All these last developments should initiate novel possible applications for the future.
References
-
(a)
J. P. Fouassier, Photoinitiation, Photopolymerization, Photocuring, Hanser, Münich, 1995 Search PubMed;
(b)
J. P. Fouassier and J. Lalevée, Photoinitiators for Polymer Synthesis: Scope, Reactivity and Efficiency, Wiley-VCH., Weinheim, to be publ Search PubMed;
(c)
D. C. Neckers, UV and EB at the Millenium, Sita Technology, London, 1999 Search PubMed;
(d)
Photoinitiated Polymerization, ed. K. D. Belfied, J. V. Crivello, ACS Symposium series 847, Washington DC, 2003 Search PubMed;
(e)
R. Schwalm, UV coatings: basics, recent developments and new applications, Elsevier, Oxford, UK, 2007 Search PubMed;
(f)
S. Davidson, Exploring the Science, Technology and Application of UV and EB Curing, Sita Technology Ltd, London, 1999 Search PubMed;
(g)
Lasers in Chemistry, M. Lackner Ed., Wiley-VCH., Weinheim, 2008 Search PubMed;
(h)
Handbook of Vinyl Polymers, M.K. Mishra, Y. Yagci, ed., CRC Press, 2009 Search PubMed;
(i)
K. Dietliker, A Compilation of Photoinitiators Commercially Available for UV Today, Sita Technology Ltd, Edinburgh, London, 2002 Search PubMed;
(j)
Photochemistry and Photophysics of Polymer Materials, N.S. Allen, Eds, Wiley, USA, 2010 Search PubMed;
(k)
Photochemistry and UV Curing: new trends, J.P. Fouassier, ed., Research Signpost, Trivandrum India, 2006 Search PubMed;
(l)
Basics of Photopolymerization Reactions, J.P. Fouassier, X. Allonas, ed., Research Signpost, Trivandrum India, 2010 Search PubMed;
(m)
W. Schnabel, Polymer and Light, Wiley-VCH, Weinheim, 2007 Search PubMed.
-
A. F. Cunningham and V. Desobry, in Radiation Curing in Polymer Science and Technology, ed. J. P. Fouassier, J. F. Rabek ed., Elsevier, Barking (UK), vol. 2, 323–374, 1993.
-
D. F. Eaton, in Advances in Photochemistry, ed. D. H. Hammond, G. S. Gollnick Eds, Wiley, NY, 13, 427–487 1986.
- B. M. Monroe and G. C. Weed, Chem. Rev., 1993, 93, 435–448 CrossRef CAS.
- T. Urano, E. Ohno, K. Sakamoto, M. Wada, S. Ito, N. Ono, S. Suzuki and T. Yamaoka, Imaging Sci. J., 2000, 48, 147–152 CAS.
- T. Urano, E. Hino, H. Ito, M. Shimizu and T. Yamaoka, Polym. Adv. Technol., 1998, 9(12), 825–830 CrossRef CAS.
- T. Urano, H. Ito and T. Yamaoka, Polym. Adv. Technol., 1999, 10, 321–328 CrossRef CAS.
- M. Harada, M. Kawabata and Y. Takimoto, J. Photopolym. Sci. and Tech., 1989, 2, 199 CAS.
- J. P. Fouassier, Recent Res. Devel. in Polymer Science, 2000, 4, 131–145 CAS.
- J. P. Fouassier, A. Erddalane, F. Morlet-Savary, I. Sumiyoshi, M. Harada and M. Kawabata, Macromolecules, 1994, 27, 3349–3356 CrossRef CAS.
- T. B. Cavitt, B. Phillips, C. E. Hoyle, B. Pan, S. B. Hait, K. Viswanathan and E. S. Joensson, J. Polym. Sci., Part A: Polym. Chem., 2004, 42, 4009–4015 CrossRef CAS.
- T. B. Cavitt, C. E. Hoyle, V. Kalyanaraman and S. Jonsson, Polymer, 2004, 45(4), 1119–1123 CrossRef CAS.
- M. E. Mullings, C. D. Walker, A. McDonough and T. B. Cavitt, J. Coat. Technol. Res., 2007, 4, 255–261 CrossRef CAS.
- J. P. Fouassier, X. Allonas, J. Lalevée and M. Visconti, J. Polym. Sci., Part A: Polym. Chem., 2000, 38, 4531–4541 CrossRef CAS.
- J. Yang and D. C. Neckers, J. Polym. Sci., Part A: Polym. Chem., 2004, 42, 3836–3841 CrossRef CAS.
- G. Ye, F. Courtecuisse, X. Allonas, C. Ley, C. Crouxte-Barghorn and P. Raja, Prog. Org Coat., 2011 Search PubMed , under press..
- J. Kabatc, Z. Czech and A. Kowalczyk, J. Photochem. Photobiol., A, 2011, 219, 16–25 CrossRef CAS.
- D. Burget, C. Mallein and J. P. Fouassier, Polymer, 2003, 44, 7671–7678 CrossRef CAS.
- L. Catilaz, J. P. Fouassier and P. Navergoni, J. Radiation Curing., 1995, 21, 10–12 Search PubMed.
- D. Burget and J. P. Fouassier, J. Chem. Soc., Faraday Trans., 1998, 94, 1849–1854 RSC.
- L. Catilaz-Simonin and J. P. Fouassier, J. Appl. Polym. Sci., 2001, 79, 1911–1923 CrossRef CAS.
- Y. Bi and D. C. Neckers, Macromolecules, 1994, 27, 3683–3693 CrossRef CAS.
- G. Yilmaz, S. Beyazit and Y. Yagci, J. Polym. Sci., Part A: Polym. Chem., 2011, 49, 1591–1596 CrossRef CAS.
- Y. Yagci and H. Hepuzer, Macromolecules, 1999, 32, 6367–6370 CrossRef CAS.
- J. V. Crivello, J. Polym. Sci., Part A: Polym. Chem., 2009, 47, 866–875 CrossRef CAS.
- J. V. Crivello, J. Macromol. Sci., Part A: Pure Appl. Chem., 2009, 46, 474–483 CrossRef CAS.
- J. P. Fouassier, D. Ruhlmann, Y. Takimoto, M. Harada and M. Kawabata, J. Polym. Sci., Part A: Polym. Chem., 1993, 31, 2245–2248 CrossRef CAS.
-
(a) J. P. Fouassier and E. Chesneau, Makromol. Chem., 1991, 192, 1307–1315 CrossRef CAS;
(b) J. P. Fouassier and E. Chesneau, Angew. Makromol. Chem., 1985, 135, 41–64 CrossRef;
(c) J. P. Fouassier, E. Chesneau and M. Le Baccon, Makromol. Chem. Rapid Commun., 1988, 9, 223–227 CrossRef CAS.
- K. S. Padon and A. B. Scranton, J. Polym. Sci., Part A: Polym. Chem., 2000, 38, 3336–3346 CrossRef CAS.
- K. Kim and A. Scranton, J. Polym. Sci., Part A: Polym. Chem., 2004, 42, 5863–5871 CrossRef.
- M. L. Gomez, V. Avila, H. A. Montejano and C. M. Previtali, Polymer, 2003, 44, 2875–2881 CrossRef CAS.
- M. L. Gomez, H. A. Montejano, M. del Valle Bohorquez and C. M. Previtali, J. Polym. Sci., Part A: Polym. Chem., 2004, 42, 4916–4920 CrossRef CAS.
- A. Erddalane, J. P. Fouassier, F. Morlet-Savary and Y. Takimoto, J. Polym. Sci., Part A: Polym. Chem., 1996, 34, 633–642 CrossRef CAS.
- M. L. Gomez, C. M. Previtali and H. A. Montejano, Polymer, 2007, 48, 2355–2361 CrossRef CAS.
- S. Hassoons and D. C. Neckers, J. Phys. Chem., 1995, 99, 9416–9421 CrossRef.
- H. J. Timpe, S. Ulrich, C. Decker and J. P. Fouassier, Eur. Polym. J., 1994, 30, 1301–1307 CrossRef CAS.
- C. Grotzinger, D. Burget, P. Jacques and J. P. Fouassier, Macromol. Chem. Phys., 2001, 202, 3513–3522 CrossRef CAS.
- C. Grotzinger, D. Burget, P. Jacques and J. P. Fouassier, Polymer, 2003, 44, 3671–3677 CrossRef CAS.
- O. I. Tarzi, X. Allonas, C. Ley and J. P. Fouassier, J. Polym. Sci., Part A: Polym. Chem., 2010, 48, 2594–2603 CrossRef CAS.
- J. Kabatc, M. Zasada and J. Paczkowski, J. Polym. Sci., Part A: Polym. Chem., 2007, 45, 3626–3636 CrossRef CAS.
- S. Jockusch, H. J. Timpe, W. Schnabel and N. J. Turro, J. Phys. Chem. A, 1997, 101, 440–445 CrossRef CAS.
- J. P. Fouassier, F. Morlet-Savary, K. Yamashita and S. Imahashi, J. Appl. Polym. Sci., 1996, 62, 1877–1885 CrossRef CAS.
- J. P. Fouassier, F. Morlet-Savary, K. Yamashita and S. Imahashi, Polymer, 1997, 38, 1415–1421 CrossRef CAS.
- C. Decker and B. Elzaouk, J. Appl. Polym. Sci., 1997, 65, 833–844 CrossRef CAS.
-
B. Jedrzejewska, in Basics of Photopolymerization Reactions, ed. J. P. Fouassier, X. Allonas, Research Signpost, Trivandrum India, vol.1, 115–134, 2010.
-
J. Kabatc, in Basics of Photopolymerization Reactions, ed. J. P. Fouassier, X. Allonas, Research Signpost, Trivandrum India, vol.1, 135–160, 2010.
- J. Kabatc and J. Paczkowski, J. Polym. Sci., Part A: Polym. Chem., 2009, 47, 576–588 CrossRef CAS.
- B. Jedrzejewska, Z. Rafinski and J. Paczkowski, Polimery, 2009, 54, 417–420 CAS.
- J. Kabatc and J. Paczkowski, Journal of Applied Polymer Science, 2010, 117, 2669–2675 CAS.
- J. Kabatc, Polymer, 2010, 51, 5028–5036 CrossRef CAS.
- A. D. liu, A. D. Trifunac and V. Krongrauz, Chem. Phys. Lett., 1992, 198, 200–205 CrossRef.
- T. Urano, Y. Tsurutani, M. Ishikawa and H. Itoh, J. Photopolym. Sci. Technol., 2000, 13, 83–88 CrossRef CAS.
- T. Urano, J. Photopolym. Sci. Technol., 2003, 16, 129–156 CrossRef CAS.
- X. Allonas, J. P. Fouassier, M. Kaji and S. Murakami, Photochem. Photobiol. Sci., 2003, 2, 224 CAS.
- X. Allonas, J. P. Fouassier, M. Kaji, M. Miyasaka and T. Hidaka, Polymer, 2001, 42, 7627–7634 CrossRef CAS.
- X. Allonas, J. P. Fouassier, H. Obeid, M. Kaji and Y. Ichihashi, J. Photopolym. Sci. Technol., 2004, 17, 35–40 CrossRef CAS.
- X. Allonas, J. P. Fouassier, M. Kaji and Y. Murakami, Photochem. Photobiol. Sci., 2003, 2, 224–229 CAS.
- M. El Roz, J. Lalevée, X. Allonas and J. P. Fouassier, Macromolecules, 2009, 42, 8725–8732 CrossRef CAS.
- J. Lalevée, M. A. Tehfe, D. Gigmes, N. Blanchard and J. P. Fouassier, Macromolecules, 2010, 43, 6608–6615 CrossRef.
- J. Lalevée, M. A. Tehfe, F. Morlet-Savary, B. Graff, X. Allonas and J. P. Fouassier, Prog. Org. Coat., 2011, 70, 23–31 CrossRef.
-
(a) A. Ledwith, Makromol. Chem., 1979, 3, 398–405 CrossRef;
(b) W. Schnabel, A. Bottcher, K. Hasebe, G. Hizal, Y. Yagci and P. Stellberg, Polymer, 1991, 32, 2289–2295 CrossRef;
(c) Y. Yagci and A. Ledwith, J. Polym. Sci., Part A: Polym. Chem., 1988, 26, 1911–1918 CrossRef CAS;
(d) Y. Yagci and W. Schnabel, Makromol. Chem. Rapid Commun., 1987, 8, 209–215 CrossRef CAS;
(e) C. Dursun, M. Degirmenci, Y. Yagci, S. Jockusch and N. J. Turro, Polymer, 2003, 44, 7389–7396 CrossRef CAS;
(f) Y. Yagci, M. Sangermano and G. Rizza, Macromolecules, 2008, 41, 7268–7270 CrossRef CAS.
- J. Lalevée, M. El Roz, X. Allonas and J. P. Fouassier, J. Polym. Sci., Part A: Polym. Chem., 2008, 46, 2008–2014 CrossRef.
- M. A. Tehfe, F. Morlet-Savary, J. Lalevée, F. Louerat, B. Graff, X. Allonas and J. P. Fouassier, Eur. Polym. J., 2010, 46, 2138–2144 CrossRef CAS.
- J. Lalevée, A. Dirani, M. El Roz, X. Allonas and J. P. Fouassier, J. Polym. Sci., Part A: Polym. Chem., 2008, 46, 3042–3047 CrossRef.
- J. Lalevée, M. El Roz, M. Tehfe, X. Allonas and J. P. Fouassier, Macromolecules, 2009, 42(22), 8669–8674 CrossRef.
- M. El Roz, F. Morlet-Savary, J. Lalevée, X. Allonas and J. P. Fouassier, J. Polym. Sci., Part A: Polym. Chem., 2008, 46, 7369–7375 CrossRef CAS.
- J. Lalevée, M. A. Tehfe, D. Gigmes, N. Blanchard and J. P. Fouassier, Macromolecules, 2010, 43, 6608–6615 CrossRef.
- M. A. Tehfe, J. Lalevée, D. Gigmes and J. P. Fouassier, J. Polym. Sci., Part A: Polym. Chem., 2010, 48, 1830–1837 CrossRef CAS.
- J. Lalevée, N. Blanchard, M. A. Tehfe and J. P. Fouassier, Macromol. Rapid Commun., 2011, 32, 838–843 CrossRef.
- J. Lalevée, N. Blanchard, M. A. Tehfe, A. C. Chany, F. Morlet-Savary and J. P. Fouassier, Macromolecules, 2010, 43, 10191–10195 CrossRef.
- J. Lalevée, N. Blanchard, M. A. Tehfe, F. Morlet-Savary and J. P. Fouassier, Polym. Chem., 2011, 2, 1986–1991 RSC.
- J. Lalevée and J. P. Fouassier, Polym. Chem., 2011, 2, 1107–1113 RSC.
- J. Lalevée, M. Peter, F. Dumur, D. Gigmes, N. Blanchard, M. A. Tehfe and J. P. Fouassier, Chem. Eur. J., 2011, 17, 15027–15031 CrossRef.
- J. Lalevée, N. Blanchard, M. A. Tehfe, M. Peter, F. Morlet-Savary and J. P. Fouassier, Polym. Bull., 2012, 68, 341–347 CrossRef.
- M. A. Tehfe, J. Lalevée, F. Morlet-Savary, B. Graff and J. P. Fouassier, Macromolecules, 2011, 44, 8374–8379 CrossRef CAS.
|
This journal is © The Royal Society of Chemistry 2012 |