Environmental effects of ozone depletion and its interactions with climate change: progress report, 2011
Received
20th October 2011
, Accepted 21st October 2011
First published on 2nd December 2011
Abstract
The parties to the Montreal Protocol are informed by three panels of experts. One of these is the Environmental Effects Assessment Panel (EEAP), which deals with two focal issues. The first focus is the effects of increased UV radiation on human health, animals, plants, biogeochemistry, air quality, and materials. The second focus is on interactions between UV radiation and global climate change and how these may affect humans and the environment. When considering the effects of climate change, it has become clear that processes resulting in changes in stratospheric ozone are more complex than believed previously. As a result of this, human health and environmental problems will be longer-lasting and more regionally variable. Like the other panels, the EEAP produces a detailed report every four years; the most recent was published in 2010 (Photochem. Photobiol. Sci., 2011, 10, 173–300). In the years in between, the EEAP produces less detailed and shorter progress reports, which highlight and assess the significance of developments in key areas of importance to the parties. The next full quadrennial report will be published in 2014–2015.
1. Ozone and changes in biologically active UV radiation reaching the Earth's surface
• Without the Montreal Protocol, erythemally weighted UV radiation‡would have been increased by a factor of 3 or more at all latitudes by 2065, with values of the UV Index (UVI) exceeding 40 at the equator. These high UVI values would have resulted in substantial harmful environmental impacts, while in winter at high latitudes there may have been some beneficial effects (see section 2). In the expected future, following the implementation of the Montreal Protocol, long-term absolute changes in both summer and winter clear-sky UVI will be less than 0.4 outside southern polar latitudes.1
• A recent analysis of total ozone data from multiple satellite instruments (1979–2009), reported that an upward trend in total ozone over Antarctica since the late 1990s is detectable and is presumably due to reduction in CFCs.2 This positive trend was identified after removing the effect of circulation changes on ozone. However, significant uncertainties exist in the stability of the satellite instruments over the long periods considered, the combination of measurements from different satellites, and the isolation of effects from circulation. The latter is particularly difficult as changes in circulation may affect the temperature in the stratosphere and the formation of polar stratospheric clouds that are involved in the depletion of ozone. Further confirmation of these findings and/or longer data sets would be valuable. This finding does not preclude the formation of ozone holes in the future that would result in increased UV radiation levels over the southern high latitudes.
• New modelling studies have further strengthened our understanding of the impacts of ozone depletion on climate-related factors. These impacts include changes in: (a) atmospheric circulation (displacement of the mid-latitude jet),3,4 (b) precipitation (e.g., increases in the southern subtropics during the austral summer),5 and (c) circulation in the ocean (e.g., strengthening of the sub-polar meridional ocean circulation and the Antarctic circumpolar current).5 These changes may have impacts on air quality and ecosystems (see sections 3, 5, and 6).
Unprecedented chemical destruction of stratospheric ozone inside the Arctic polar vortex has been observed in March 2011, identifiable as an Arctic “ozone hole”.
6 For the first time in the observational record, destruction of ozone over the Arctic in the springtime was comparable to that over Antarctica. Unusually long-lasting cold conditions in the lower stratosphere led to destruction of more than 80% of the ozone between 18 and 20 kilometres above sea level. In sharp contrast with normal years when ozone amounts less than 275 DU are rare, in March 2011, over 40% of the vortex area had ozone levels less than that threshold (Fig. 1). Areas of low ozone that may extend to populated areas in the northern mid- and high-latitudes would result in significantly elevated UV irradiances compared with the normal Arctic spring, with consequences for human health and for ecosystems. However, the UV levels in the Arctic should be less extreme compared to Antarctica. The results show that ozone “holes” are possible even with temperatures much milder than those in the Antarctica. It is difficult to predict whether such severe Arctic ozone depletion may be matched or exceeded in the future. However, if it continues to occur, and particularly if it becomes more severe, effects of increased UV on organisms may be exacerbated by reduced protection due to decreases in snow- or ice-cover resulting from global warming.
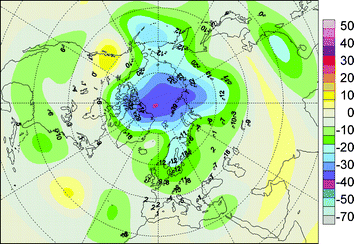 |
| Fig. 1 Percent average reduction in total ozone over the northern hemisphere for March 2011. The highest loss (more than 80%) was observed between 18 and 20 kilometres above sea level over the Arctic. The map was produced by the WMO Northern Hemisphere Ozone Mapping Centre in Thessaloniki, Greece, by combining data from the GOME-2 satellite and Brewer/Dobson ground-based measurements. Reductions were computed relative to 1979–1981 average derived from TOMS total ozone measurements. | |
• Surface UV radiation in the 21st century will be influenced by increases in stratospheric ozone due to reduction in ozone-depleting substances and by changes in ozone and cloudiness induced by increasing concentrations of greenhouse gases. Models suggest that in the first half of the 21st century the recovery of ozone and changes in cloudiness will result in decreases in surface erythemal irradiance by 2–10% at mid-latitudes, and by up to 20% at northern and 50% at southern high latitudes. These decreases are dominated by the projected increases in stratospheric ozone. In the tropics, the projected changes are small (<2%) over this period.7 By comparing model projections between 2100 and 1960, the effects of climate change on surface UV can be estimated, since the ozone depletion started after 1960 and ozone recovery would have been completed by 2100. By the end of the 21st century erythemal irradiance: (a) will remain below 1960 levels due to changes in clouds and greenhouse gas (GHG)-induced ozone transport at mid-latitudes, (b) will be reduced at high latitudes (particularly in the Arctic) by 5–10% due to changes in clouds, and c) will increase in the tropics by between 3 and 8% due to decreases in clouds and ozone, induced by GHGs.
• Modelling the effects of UV radiation by weighting spectral irradiance measurements does not necessarily correspond directly to the actual environmental impacts. Firstly, the shape and orientation of surfaces exposed is important: for example, solar UV radiation incident on a cylindrical surface is typically less than 50% of that on a horizontal surface.8 Secondly, there may be additional uncertainties arising from incomplete knowledge of the wavelength dependence of the effects (i.e., the shape of the weighting functions)9,10 (see sections 2 and 3). Consequently, care must be taken when assessing the environmental impacts of UV changes using measurements of ambient UV irradiance.
2. Human health: effects of solar UV radiation and interactions with climate change
• Melanoma is now more definitively linked with solar UV-B exposure as a risk factor, particularly episodes of severe sunburn; its incidence continues to increase. Recent findings support the evidence that UV-B radiation is critical for the initiation of cutaneous malignant melanoma (CMM).11,12 The risk of melanoma is most closely related to episodes of severe sunburn, often occurring with deliberate over-exposure in fair-skinned individuals. As it is not possible to predict frequency of sunburn in future years, the corresponding impact on the incidence of CMM in relation to changes in solar UV-B radiation is uncertain. Marked increases in incidence of CMM, e.g. a doubling between 1978 and 2007,13 have been noted in several countries,14–16 but not to the same extent in younger age groups in locations with strong and well-established sun protection programmes.17 The relative proportion of thick and thin melanomas has remained relatively constant,18–20 although more of the thick tumours resulted in death.20 A high number of common melanocytic naevi (moles) is a well-recognised risk factor for CMM.21 In a recent study in France, children who practised outdoor sports had more naevi than those who did not,22 while the use of sun protection in young children in Sweden led to the development of fewer naevi.23
• Exposure to solar UV-B radiation causes the non-melanoma skin cancers (NMSCs), basal cell carcinoma (BCC) and squamous cell carcinoma (SCC). Where monitored, the annual incidence rate of these cancers has increased over the past 30 years and is projected to continue to increase, particularly in those aged over 50 years. For example, the annual incidence of BCC increased by 80% between 1999 and 2008 in Santa Catarina (Brazil),24 and of all skin cancers by 305% between 1974 and 2005 in Puerto Rico.25 The lifetime risk of BCC in the Dutch population is estimated to be 1 in 5–6, with no indication of a plateauing in the incidence rate.26 Two recent meta-analyses of all the relevant published studies to date showed that occupational exposure to UV radiation increased the risk of both SCC27 and BCC.28 In contrast, in the Danish population, outdoor workers had a significantly decreased risk of NMSC.29 Although cutaneous malignancies are less frequent in those with deeply pigmented compared with fair skin, they are thought to rank amongst the top 10 malignancies in sub-Saharan Africa with significant under-reporting being likely.30 In terms of world health and public health management, the NMSCs represent a major and increasing burden.
• Exposure to solar UV radiation contributes to the development of Merkel cell carcinoma (MCC), an uncommon but aggressive tumour that occurs on sun-exposed body sites.31 MCC is found most frequently in elderly men, and survival is poorer than for melanoma.32,33 The highest incidence of this malignancy has been reported in Western Australia (age-standardised rate of 0.82 per 100
000) and there is evidence that the incidence rate is increasing.32,33 Solar UV may activate a virus-specific (polyomavirus) tumour antigen found in many MCC, thus providing a possible link between UV radiation and risk of MCC.34 The incidence of MCC may increase in the future as a result of the depletion of the stratospheric ozone layer that has already occurred.
• Common eye diseases resulting from chronic exposure to UV radiation, such as pterygium and cataract, create a large social and financial burden globally. A detailed study of pterygium lesions (an invasive growth of the eye), traditionally classified as degenerative, showed that a significant proportion contained concurrent ocular tumours, including melanomas.35 Epidemiological evidence suggests that conjunctival melanomas (on the external surface of the eye) but not intraocular (internal) melanomas are likely to be induced by exposure to solar UV radiation.36 The molecular changes detected recently in conjunctival melanomas are different from those in intraocular melanomas, further suggesting that they have different causes.36 In a prospective study involving almost 5000 individuals, the risk of developing cortical cataract was significantly higher in subjects using sun-sensitising medications.37 Measurements of irradiance may not provide a good guide to risks of UV exposure to the eye, because they do not take account of the modifications in the received dose due to the facial profile and reflected UV radiation.38 The impact of stratospheric ozone depletion on these eye diseases remains difficult to assess, either quantitatively (for example, uncertainty regarding wavelength-dependence and dose-responses for cataract and pterygium) or even qualitatively (for example, whether there is a definite association between UV exposure and ocular melanomas).
• The potential benefit of vitamin D, produced as a result of exposure to solar UV-B radiation, should be balanced against the harmful outcomes of such exposure, including skin cancers and cataract. High percentages of people, even in sunny climates, are considered vitamin D-insufficient, although inaccuracies in measurement limit the confidence in these findings.39,40 Nevertheless, there is considerable interest in strategies to minimise vitamin D-deficiency. Theoretical calculations of synthesis of vitamin D achieved by solar UV-B irradiation41–43 are now being replaced by what are likely to be more accurate estimates from real-life exposure to the sun. In experiments using irradiation of volunteers with simulated sunlight, it was shown that casual exposure to midday summer sun at mid-latitudes for about 30 min (unshaded sun exposure while dressed in summer clothing, three times weekly) raises production of vitamin D to sufficiently high levels in most fair-skinned individuals.44 Many people can attain similar vitamin D levels in summer with more frequent exposures, and with more extended exposures at the weekends.45 Most studies have shown that vitamin D-sufficiency in the summer does not necessarily guarantee adequate levels in the winter months.45–48 Extended sunbathing in the summer should not be encouraged, and the decrease in vitamin D that occurs in the winter months at mid to high latitudes, due to the lack of solar UV-B irradiation at this time of the year, might be best countered by diet or the judicious use of vitamin D supplements.
• Low levels of exposure to the sun and/or of vitamin D are associated with an increased risk of multiple sclerosis, diabetes and some infectious diseases. Evidence from both animal models49 and epidemiological studies50–53 suggest that lower exposure to the sun and vitamin D-insufficiency are separately important to increasing the risk of developing multiple sclerosis (MS). In contrast, two animal models have shown that the onset of MS and the severity of the disease are diminished by vitamin D-deficiency.54,55 Further uncertainty regarding the importance of vitamin D in modulating the activity of the disease in people with MS is indicated by clinical trials of vitamin D-supplementation, which have not shown an improvement in disease parameters.56 Vitamin D-deficiency is associated with higher disease activity in people with the autoimmune disease, systemic lupus erythematosus (SLE),57,58 including in the newly diagnosed, and is more common in anti-nuclear antibody (ANA)-positive healthy controls (at increased risk of SLE) compared with ANA-negative controls.58 The incidence of diabetes (mainly type 2) is lower in people with active sun-exposure habits59 or higher levels of vitamin D,60 and one study showed an inverse relationship between the prevalence of type 1 diabetes and irradiance of erythemal UV-B in Newfoundland.61
An Australian study showed that the development of food allergies in Australia was more common for those born in the autumn/winter season, possibly due to lower vitamin D-status during early infancy.62 The evidence in relation to asthma and sun exposure and/or vitamin D is weak and contradictory.63,64
Seasonal patterns in the incidence of tuberculosis suggest an increased risk of active disease with lower levels of UV exposure,65 but clinical trials using vitamin D-supplementation in addition to regular antibiotic therapy, indicate a beneficial effect of vitamin D only in those with a specific vitamin D receptor genotype.66 Lower vitamin D status has been associated with an increased risk of upper respiratory tract infections during winter,67 and increased reactivation from latency of the human herpes virus, Epstein Barr virus.68,69
• Although many health benefits have been proposed for vitamin D, some caution is required, particularly in situations where the vitamin D levels are increased through dietary supplements rather than by natural sunlight exposure. In several cases, a U-shaped dose-response curve has been observed, indicating that a low and a high vitamin D status may not be beneficial. This has been shown for prostate cancer,70 tuberculosis,71 premature aging,72 and mortality,73,74 and is illustrated in Fig. 2.
![Odds of prostate cancer (A) and tuberculosis (B) in relation to concentration of 25-hydroxyvitamin D [25(OH)D] in serum. Bars are 95% confidence intervals. (A) plotted from data in Table III of Tuohimaa et al.70 using the median of the range of 25(OH)D concentration in each category; (B) plotted from data in Table 1 of Nielsen et al.71 Median of reference category; upper and lower categories are taken as 50 nmol L−1 and 150 nmol L−1.](/image/article/2012/PP/c1pp90033a/c1pp90033a-f2.gif) |
| Fig. 2 Odds of prostate cancer (A) and tuberculosis (B) in relation to concentration of 25-hydroxyvitamin D [25(OH)D] in serum. Bars are 95% confidence intervals. (A) plotted from data in Table III of Tuohimaa et al.70 using the median of the range of 25(OH)D concentration in each category; (B) plotted from data in Table 1 of Nielsen et al.71 Median of reference category; upper and lower categories are taken as 50 nmol L−1 and 150 nmol L−1. | |
• Protection against the adverse effects of solar UV-B radiation is necessary when the sunlight is intense. Public health campaigns in several countries aim to inform the public about the risks and the most effective methods of protection but are not available in all regions of high risk. Sunscreens lessen the risk of sunburn and SCC development; recently a trial of regular use of sunscreens in Queensland residents over a four year period reduced the incidence of new primary cutaneous melanomas.75 Improved sun protection and awareness has been suggested as one reason for the decreasing incidence rates of melanoma in children in Australia and Sweden, from a peak in the mid-1990s.76 On the other hand, holidays in sunny climates have become very popular, leading to a high risk of sunburn especially as many people on vacation wish to tan intentionally.77 Measures used for protecting against solar UV need to target such behaviour. While the local UV Index should be informative, there is not widespread understanding of what it means and therefore sun and photoprotection behaviour are not modified according to the UV conditions.78 In addition to staying indoors, seeking shade, covering up, wearing wrap-around sunglasses or UV-absorbing contact lenses79,80 and using conventional sunscreens, more effective sunscreens and alternative strategies for photoprotection are in development.81,82 Topically applied agents that have been tested in human subjects include the antioxidant nicotinamide (one of the group B vitamins),83 and enzymes for repair of DNA.84 Dietary components which may provide systemic photoprotection include green tea extracts, pomegranate juice, and omega-3 polyunsaturated fatty acids.85 Zerumbone, an extract from a Southeast Asian ginger plant, protected against UV-induced cataract in a mouse model.86 These strategies may have the potential to counter some detrimental health effects of stratospheric ozone depletion.
• There is limited new information in the peer-reviewed literature regarding the toxicity of products being proposed as alternatives for ozone-depleting substances. The lack of published information is principally due to two factors. First, many of the products being submitted for approval to regulatory agencies are not new but are re-purposed having received prior approval for another use or in another industrial sector.87,88 For example, a number of the low toxicity replacements for halons used in fire suppression, such as water and detergent-based formulations, have an increasingly wide range of applications.89 Such products are less likely to require additional toxicity information because the submissions focus on changes in exposure and how they might affect previous risk assessments. Secondly, when manufacturers develop new toxicity information, they are required to submit it to regulatory programmes such as SNAP (Significant New Alternatives Policy) but rarely publish it and, indeed, can designate it as confidential business information. The result is that the regulatory agencies can integrate such information into their risk assessments without disclosing the confidential details. For example, when concerns about the reproductive effects of brominated hydrocarbons, such as n-propyl bromide, led to their phase-out as substitutes for the ozone-depleting substances in certain sectors, much of the data upon which the SNAP decisions were based never entered the peer-reviewed literature.90
• Changes in the climate resulting from depletion of stratospheric ozone and global warming might result in a rise in the incidence and prevalence of water-borne and vector-borne infections. One example is that depletion of the ozone layer in the polar stratosphere has led to increased rainfall in the southern subtropics,5 possibly increasing the risks of diarrhoeal diseases, malaria and dengue fever.91–93 However, because of changes in societal behaviour, infrastructure, economic development and disease control, such adverse outcomes are uncertain at the present time.94
3. Terrestrial ecosystems: effects of solar UV radiation and interactions with climate change
• While depletion of stratospheric ozone results in increased UV-B radiation reaching the Earth's surface with well-documented impacts on biota,95–99new studies suggest that this ozone depletion also can influence biological processes by driving large scale climate change events. There is evidence that the Antarctic ozone hole has been responsible for changes in atmospheric circulation in the Southern Hemisphere over the last half century, leading to increased wind speeds around the Antarctic coast100 and altering precipitation in the subtropics.5 In Antarctica, higher wind speeds appear to have caused drying of Antarctic coastal sites, leading to decreasing growth rates in Antarctic mosses,101 as well as increasing salinity and changing diatom communities in East Antarctic lakes.102 In the subtropical regions, ozone-related changes in precipitation may have consequences for water resources and terrestrial ecosystems in the Southern Hemisphere.100
• Evidence continues to demonstrate that solar UV-B radiation is an important regulator of interactions between plants and consumer organisms, such as pests and pathogens. Solar UV-B radiation can induce changes in plant chemistry that affect the abundance and performance of herbivorous insects. For example, attenuation of solar UV-B can increase reproduction of aphids on plants – a response associated with reduced amounts of phenolic compounds in the host plants.103 Similarly, attenuation of UV-B can result in increased herbivory by thrips. This effect is at least partially attributable to changes in the expression of defence responses controlled by the plant hormone jasmonate.104 In certain cases, the metabolic response that provides resistance against a biotic stressor, such as pathogen attack, is different from the one that confers protection against UV-B radiation. Under these circumstances, the acclimation strategy of plants may be directed toward biotic defence, thereby potentially increasing vulnerability to UV-B radiation.105 Abscisic acid, a plant hormone, has been implicated in the mediation of the shifts in defence against biotic and abiotic stress.105,106 These metabolic and sometimes morphological adjustments may also imply an energy cost to the plant in terms of redistribution of resources.99,107,108 Laboratory studies indicate that UV-B radiation can affect the survival and behavioural patterns of predatory mites, which are natural enemies of plant feeders.109,110 Collectively, these studies support the prediction that variations in solar UV-B radiation fluxes may affect trophic relationships in plant canopies in natural and managed ecosystems.
• A major breakthrough has been made in the understanding of the molecular mechanisms that control plant responses to UV-B radiation. As indicated in previous assessments,99 significant advances have been made in recent years in identifying the mechanisms by which plants sense and respond to UV-B radiation. This year, these efforts led to the identification of UVR8 (UV RESISTANCE LOCUS 8) as a UV-B photoreceptor.111 Absorption of UV-B induces instant monomerisation of the photoreceptor and the interaction of UVR8 with the protein COP1, which is a central regulator of light signalling in plants. This study, which is now supported by additional modelling evidence,112 along with further characterisation of the signalling pathway,113 and identification of new functions of UVR8,114 provide a robust molecular framework which will greatly facilitate efforts to understand the mechanisms of plant response to changes in solar UV-B radiation.
• Whereas some doubts have been cast about the accuracy of previous attempts to simulate the effects of ozone depletion on UV levels, a new computational assessment largely validates most experimental approaches that have been used thus far in plant research. This study10 compares potential errors that can occur in the use of UV emitting lamps in field experiments with plants, and points out situations that can lead to substantial errors in experimental designs of UV-lamp experiments.
4. Aquatic ecosystems: effects of solar UV radiation and interactions with climate change
• The mechanisms by which UV-B radiation affects aquatic organisms are now well-known in many cases, but scaling-up to responses of whole ecosystems remains a major challenge. In addition, while there are many laboratory and small-scale field studies of UV effects, there have been no large-scale field investigations in the open ocean. Investigation of the effects of enhanced exposure to UV-B at the ecosystem level is necessary to determine the impacts on aquatic ecosystem services, such as coastal protection, control of erosion, habitat-fishery linkages,115 biodiversity maintenance,116 and other ecosystem functions.117,118 It is unknown whether altered ecosystems with different functional properties might arise as a result of differential sensitivities of species to UV-B radiation.119 The deficiency of large-scale field investigations in the open ocean is due to the vast area to be covered and the lack of instrumentation to do this scale of research in the water column.120–122 As a result, in the open ocean, there has been no substantial experimental work or long-term monitoring other than that using various satellite programs123–125 to provide baseline biological data, and there likely will not be in the near future.126,127
• Increased exposure of aquatic organisms to solar UV-B radiation has the potential to decrease production in the surface layer of the water column. This is because one of the outcomes of global climate change is a decrease in thickness of the upper mixed layer in the water column of lakes and oceans. This may further decrease the amount of available nutrients (and thus photosynthetic production) within the upper mixed layer as it is isolated from the flux of nutrients from deep water.128,129 Degradation of dissolved organic matter by UV-B radiation partially counteracts the lack of (or limitation of) nutrients, and increases biomass and productivity by bacteria and phytoplankton. This in turn also stimulates higher trophic levels and affects biogeochemical cycles. This has been shown not only in oceans,130 but also in some lakes131 and rivers.132 A reduction in photosynthesis due to a decrease in nutrients and/or to an increase in UV-B exposure is likely to result in reduced carbon fixation (see also section 5), and therefore less food for other trophic levels. This may, in turn, have a negative impact on fish stocks.
• Increased penetration of solar UV-B radiation into certain marine organisms may occur because of increased acidification of the upper mixed layer of the ocean which compromises the calcification of these organisms. This is due to the enhanced uptake of carbon dioxide from the atmosphere into the top layers of the ocean. Many phytoplankton, macroalgae (seaweeds) and animals (sea shells, worms, corals, etc.) possess external calcified skeletons, which protect them from environmental impacts including solar UV-B exposure.133 Increased acidification impairs the incorporation of calcium carbonate into the shells.134–136 This decreased protection against UV-B can decrease photosynthesis, thereby reducing carbon fixation.133,137 In addition, acidification can increase the dissolution of calcified structures in the sediments, which will decrease sequestration of carbon dioxide into the ocean.138 Thus, as a result of these two processes, less carbon withdrawn from the atmosphere and less calcification of algal and animal species would decrease the “natural” protection of coasts (i.e., compacting and cementing into durable reef formation), exposing beaches and coastal cities to potential damage.139,140 Combined effects of UV radiation (both UV-A and UV-B) and climate change factors (such as increased carbon dioxide concentration, acidification and temperature) are likely to have complex interactions in aquatic primary producers. Increased temperature can partially counteract the negative effects of UV-B radiation as demonstrated in laboratory experiments and in natural assemblages.141 One pathway appears to be through increasing the activity and gene expression of an enzyme involved in carbon fixation; thus resulting in increased photosynthesis and primary production.142
• Increased export of UV-absorbing coloured dissolved organic matter from terrestrial and wetland ecosystems to inland and coastal oceanic waters and a resultant decrease in UV transparency of the water are predicted to occur as a consequence of extreme precipitation events in some regions143 (see also section 5). Coloured dissolved organic matter (CDOM) is so effective at absorbing UV radiation that it can be considered the ozone of aquatic ecosystems, which reduces the antimicrobial effects of natural solar radiation in surface water used in drinking water supplies.91 Extreme precipitation, wind, and flooding events enhance runoff and flow of rivers to inland and coastal oceanic waters, which in turn increases CDOM, re-suspends sediments, and reduces transparency of these waters to UV radiation.132 When re-suspended sediments are exposed to sunlight they may be an important source of both nutrients 144 and dissolved organic matter (DOM).145 Studies on the Yunnan Plateau and Yangtze River indicate that particulates and phytoplankton, as well as CDOM, are important in controlling penetration of UV in rivers, while CDOM alone is most important in lakes.146 UV exposure of aquatic plants may also alter both nutrient sources and colour of water in lakes.147 Recent advances in optical techniques and fluorescence spectroscopy have enabled better quantification of the types, sources, and processing of CDOM in aquatic ecosystems.148–153 Application of these methods in the future will help us better understand the causes, consequences, and fate of the large increases in DOM that have been observed in many regions and how they influence transparency of aquatic ecosystems to UV.154
• The combined effects of UV-B exposure and elevated temperatures from climate change may alter the species composition of cyanobacteria, which may have significant impacts on the aquatic food webs. In inland waters, increased prevalence of toxic cyanobacteria would compromise the freshwater available for human consumption.155,156 For example, it has been shown that exposure to solar UV radiation combined with increased temperature has a very small or no negative impact on a globally important, potentially toxic species of cyanobacteria. However, reductions in photosynthetic efficiency, and changes to morphology and DNA were observed in other ecologically important cyanobacteria where the effects of solar UV radiation appear to be dependent on temperature.157
• Aquatic vertebrates, such as amphibians and fish, are sensitive to UV radiation, but UV-induced damage may be reduced through behavioural responses and/or deposition of eggs at greater depths. Recent studies have supported the sensitivity of the early life history stages of amphibians to UV radiation, but also the reduction of the potential for UV damage in natural ecosystems. Factors influencing potential damage are the depth at which eggs are deposited, attenuation of UV in the water column, and behavioural responses to UV radiation.158 Molecular tools that consider genotoxicity, together with the use of environmentally realistic exposure to UV and knowledge of the defences of organisms against UV, are essential for assessing the potential impacts of UV radiation on higher trophic levels.159
• Many invertebrates, including zooplankton, have a variety of successful defences that can reduce the negative impacts of elevated UV exposure. However, the interactive effects of UV and other environmental stressors, including nutrient and food availability, as well as temperature, may pose a greater threat than UV radiation alone.160 Zooplankton can detect and behaviourally avoid UV radiation in their daily vertical migration in more transparent lakes and oceans.161 While differences in tolerance to UV among species do not necessarily lead to changes in community structure of zooplankton,162 limitations of nutrients, availability of food, and cooler temperatures may all reduce tolerance to UV in zooplankton and larvae of invertebrates.163–167 UV has a negative effect on calanoid copepods grazing at cooler temperatures.168 Calanoid copepods are small crustaceans that are some of the most abundant metazoans on Earth (Fig. 3). Increased oxidative damage and developmental abnormalities of sea urchin embryos that were observed in field exposures in areas of high natural UV radiation in ozone-depleted regions may be aggravated by the loss of sea ice.169 Recent modelling efforts targeting management strategies for penguins and seals indicate that the increase in UV-B radiation due to ozone thinning over Antarctica may have increased the mortality rate of Antarctic krill by as much as 10%.170 Krill are a primary food source for these marine vertebrates.
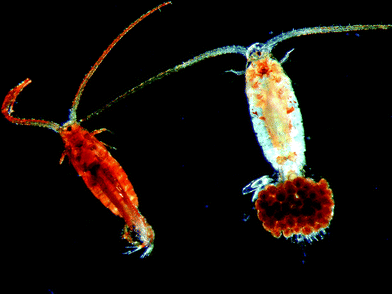 |
| Fig. 3 Calanoid copepods are some of the most abundant multi-cellular animals on Earth. They are the dominant primary consumers in the world's oceans and in many lakes. These small crustacean zooplankton influence water quality and are a critical component of aquatic food webs that may influence survival and recruitment of commercially important fish. Shown here are an adult male (left) and a female freshwater calanoid with eggs (right). Note the brighter colour of the male and the eggs vs. the female due to higher concentrations of UV-protective compounds. (Photo credit: Robert E. Moeller, with permission). | |
5. Biogeochemical cycles: effects of solar UV radiation and interactions with climate change
Interactions among stratospheric ozone changes, solar UV-B radiation, and climate change influence biogeochemical cycles in ways that can alter the balance of carbon dioxide uptake and release from the aquatic and terrestrial ecosystems. As summarised in the following bullets, understanding of the magnitude of biogeochemical responses in relation to these interactions remains very limited, and many mechanisms have yet to be incorporated in models of the global biogeochemical cycles.
• The combined effects of polar stratospheric ozone depletion and climate change are decreasing the capacity of the oceans in the polar regions to take up carbon dioxide from the atmosphere. Depletion of polar stratospheric ozone in conjunction with increasing concentrations of greenhouse gases can lead to enhanced wind-driven up-welling of carbon dioxide-rich deep water,171 which alters the balance of CO2 uptake and release from the oceans, resulting in localised reductions in the net uptake of CO2172 (see sections 1 and 6). Delayed recovery of polar stratospheric ozone due to climate change173 may cause a positive feedback; potentially further increasing concentrations of CO2 in the atmosphere. Future changes remain difficult to quantify due to uncertainties in the timing of recovery in stratospheric ozone172–174 and the challenges of integrating all relevant biological and physico-chemical processes into models.
• Reactions driven by solar UV radiation, coupled with a range of effects caused by climate change, can significantly alter carbon cycling in aquatic and terrestrial ecosystems by changing the nature and biological availability of organic carbon and nitrogen. Climate change is altering precipitation patterns5,175–177 and enhancing the frequency and amplitude of climate extremes.176 These changes in continental hydrology show great geographical variation and modify the run-off of terrestrial organic carbon into aquatic systems. In aquatic systems, solar UV radiation has multiple effects on organic matter, including the enhanced dissolution of particulate organic carbon (POC) and nitrogen.144,145,178 In addition, solar UV can alter the biological availability of organic carbon to heterotrophic bacteria, which in turn, may increase the microbial production of CO2.153,179–181 In terrestrial ecosystems, organic compounds (particularly lignin) in litter (dead plant material) are sensitive to degradation by solar UV radiation. Recent studies have shown that break-down by UV radiation can control the rate of abiotic degradation of plant litter,173 and that photodegradation of lignin also enhances microbial decomposition of plant litter.182–184 The net effect of these UV-induced processes suggests an increased release of CO2 from aquatic and terrestrial systems, potentially contributing to future climate change.
• The combined effects of changes in solar UV-B radiation and climate can reduce biological carbon fixation in aquatic systems and thus modify oceanic uptake of CO2. UV-induced photobleaching of coloured dissolved organic matter (CDOM)179 enhances the exposure of marine organisms to UV-B radiation due to increased penetration of UV in the water column. Negative impacts on photosynthetic marine organisms caused by exposure to solar UV-B radiation (see section 4) reduce biological fixation of CO2, which can result in reduced storage of carbon-containing detritus in deeper layers of water, although the balance of this and other biological and physico-chemical processes acting on carbon storage remains unclear. Photobleaching of CDOM may increase due to stratification (layering185) of the ocean related to climate change and acidification. Climate change may also reduce up-welling and availability of nutrients for phytoplankton,186,187 resulting in further interactions with solar UV radiation on biological fixation of carbon.
• UV-induced carbon emissions to the atmosphere are likely to increase with projected shifts to warmer and drier conditions, such as in the Mediterranean and in western North America; a trend towards increased droughts in the Amazon and East Asia will also likely contribute to the UV-induced carbon emissions. Arid lands currently occupy more than 30% of the terrestrial land surface and are expanding.188 There is increasing evidence that carbon cycling in these ecosystems is strongly linked to UV-induced processes,189–193 although some short-term studies have highlighted the importance of other factors affecting loss of carbon.194,195 Large emissions of carbon dioxide due to photodegradation of organic matter by UV have been shown in a broad range of ecosystems, including arid grasslands,193 deserts, and prairies,190 as well as in models for arid and arctic zones.196 If current predictions of increased aridity for some zones are correct,197,198 the ecological significance of these UV-driven processes for carbon loss from terrestrial ecosystems is expected to increase.
• Exposure to solar UV radiation affects below-ground as well as above-ground processes in terrestrial ecosystems, with consequences for ecosystem carbon balance. In non-arid terrestrial ecosystems, above-ground exposure of plants to solar UV radiation can influence below-ground growth of roots and function of roots, and the microbiology of the soil.199 Exposure to experimental increases in UV-B radiation can affect microbial biomass near the roots and reduce soil-respiration in agricultural systems190,200 and peatlands,201 which can translate into modest increases in carbon in soil and emissions of non-methane volatile organic compounds.202 Thus, it is increasingly clear that the balances between different mechanisms through which solar UV radiation influences the carbon balance of terrestrial ecosystems may be very different between arid and non-arid regions.
• There is increasing understanding of natural sources of nitrous oxide (N2O) from oceanic and terrestrial ecosystems. The oceans account for around 30% of the natural sources of N2O, an important greenhouse gas and important precursor for ozone-depleting gases.199 It has recently been identified that Archaea (a widely distributed class of bacterioplankton) are a major source of this oceanic N2O.203
6. Tropospheric air quality, composition, and processes: effects of solar UV radiation and interactions with climate change
• Depletion of stratospheric ozone affects weather patterns and air quality at ground level. Circulation in the lower atmosphere (and hence meteorological processes) in the southern hemisphere is altered by depletion of stratospheric ozone.5,174 These effects include latitudinal shifts in wind currents (mid-latitudinal jets)5 and increased precipitation (10% relative to the current climatology) in the southern subtropics.5 It is well established that meteorological processes affect air quality. For example, in Melbourne, Australia, ca. 25% of the variance in ground-level ozone can be explained by local meteorological variables such as temperature and wind speed.204 Similar results were reported for the northern hemisphere.205 Changes in stratospheric ozone need to be considered as a long-term modifier of local weather patterns and associated air quality. These effects of stratospheric ozone should be incorporated into models used for predicting future air quality.
The projected recovery of stratospheric ozone has been calculated to offset much of the influence of climate change on meteorological processes during the 21st century in the southern hemisphere. These changes in atmospheric circulation and precipitation174,206 are likely to affect long range transport and removal of pollutants from the troposphere, and therefore the intensity and frequency of occurrence of local pollution events. This will have implications for human health, plants, and environmental processes as discussed above (see sections 2–5). This should be considered in the predictions of future air pollution events.
• Hydroxyl radicals (˙OH) generated by UV-B-induced photo-dissociation of tropospheric ozone, are the main cleansing agents of the troposphere, and are responsible for the removal of many pollutants and some greenhouse gases from the atmosphere. The new measurements of ˙OH bring observations into better agreement with models,207 in particular for the inter-annual variability of global ˙OH amounts. These measurements better account for the global burden of methyl-chloroform and its rate of change due to ˙OH. They also increase confidence in modelled projections of future concentrations of ˙OH under different ozone-recovery and climate-change scenarios.
• Recent evidence suggests that a significant fraction (up to 30%) of hydroxyl radicals in the troposphere can be produced from photo-dissociation of nitrous acid (HONO). Because the photo-dissociation of HONO occurs mostly at UV-A wavelengths, this mechanism of ˙OH production is less sensitive to UV-B radiation (and hence depletion of stratospheric ozone) than previously recognised mechanisms. The sources of HONO have not been completely identified, but at least some may be of biogenic origin from soil microbes and leaves of plants exposed to UV-B199 and may be affected by human activities, such as agriculture.208 This source of ˙OH should be considered in future estimates of air quality.
• Estimates of the human health and environmental impact of air pollution highlight the potentially large impact of the coupled effects of stratospheric ozone depletion and climate change. UV-B radiation is an essential ingredient in the formation of many photochemical pollutants, including ground-level ozone and a large portion of fine particulates. Recent studies confirm the potentially large adverse effects of these pollutants on human health and vegetation. A study using atmospheric modelling has suggested that ground-level ozone was associated with an estimated 0.7 ± 0.3 million deaths/annum and particulate matter (PM2.5) was associated with 3.7 ± 1.0 million deaths per annum.209 These deaths are the equivalent of 6.3 ± 3.0 and 30 ± 7.6 million years of life lost, respectively. Although some controversy exists,210,211 these results show the importance of UV radiation and changing climate in the causation of disease in humans. A meta-analysis of environmental effects of ozone212 showed that statistically significant reductions in number of seeds (16%), number of fruit (9%) and weight of fruit (22%) result from exposures to ambient concentrations of ozone as compared to pre-industrial concentrations (<10 parts per billion (ppb)). Elevated concentrations (70–100 ppb) resulted in greater reductions in yield (27%) and weight of individual seeds (18%). Because of the importance of UV-B radiation in these processes, depletion of stratospheric ozone and interactions with climate change will have indirect but potentially large effects on the health of humans and crops.
• Regulations directed at lowering ground-level ozone will have only a small effect on UV radiation transmitted to the surface. Therefore, quality of air at ground-level can be improved without significant increases in exposures to UV radiation. A new modelling study for the eastern two-thirds of the United States indicates that reductions in the ground-level standard for ozone from 84 to 70 ppb will result in a population-weighted UV radiation increase of 0.19 ±0.06%,213 about an order of magnitude lower than previously published estimates.214
• Aircraft-based observations of the vertical distribution of UV radiation are becoming more widely available and will enable better predictions of the spatial and temporal distribution of pollutants of concern for human and environmental health. These observations215 reveal the vertical structure of UV radiation above and below clouds (actinic flux) and thus provide better evaluation of models of the photochemical processing of air pollutants in the troposphere.
• Interactions between greenhouse gases, resulting from the coupling of stratospheric and tropospheric chemical processes, have been identified. Increases in emissions of nitrous oxide (N2O) lead to a partial decrease in concentrations of methane (CH4). This interaction occurs via the effects of N2O on stratospheric ozone depletion, UV radiation, and abundance of tropospheric ˙OH. The increase of N2O from 260 ppb (pre-industrial times) to present-day 320 ppb has offset ca. 18 ppb of the methane concentration increase.216 Although this offset is only about 1% of the current concentration of CH4, such interactions between the chemical and climatic effects of various long-lived trace gases need to be considered as their concentrations continue to increase.
• New data suggests no significant increased risks from trifluoroacetic acid (TFA) to humans and the environment. Based on recent modelling, emissions of the HCFC and HFC precursors of TFA from east Asia have been adjusted to improve agreement with measured concentrations,217 but estimates of total releases to the global environment remain unchanged. In a previous assessment of releases of these and related compounds that degrade to TFA,218 risks to humans and the environment were assessed as negligible. The new information on regional releases does not change these assessments. Other fluorinated chemicals in commerce,219 which are not substitutes for ozone-depleting substances (ODSs), are degraded in the troposphere with relatively short half-lives (1.1 to 135 days) but are not expected to produce TFA (T. Wallington, personal communication).
7. Materials: effects of solar UV radiation and interactions with climate change
The currently elevated solar UV levels will result in relatively higher rates of degradation of materials exposed outdoors. However, the presently available and new stabiliser systems and strategies for substitution of material are likely able to mitigate the effect and maintain useful lifetimes of materials at present levels. This will, however, add to the lifetime cost of using the improved materials.
• Polymers commonly used in solar cells and optoelectronic devices are degraded by solar UV radiation, affecting their service lifetimes and cost. Semi-conducting polymers such as poly(3-hexylthiophene) (P3HT) and poly(3-octothiophene) (P3OT), which are used in active layers of solar cells as well as the plastics used in enclosures of solar panels, degrade on exposure to solar UV radiation, thus limiting their service life.220 While accelerated test methods specific to these materials are still being formulated,220,221 preliminary results show the light-induced damage in P3HT polymer follows a free-radical process.222 Complete action spectra for photodamage of these over the solar wavelength range are not yet available. However, in P3OT, exposure to blue light efficiently destroys its semi-conductor characteristics and is therefore more damaging compared to exposure to UV.223 Multi-walled carbon nanotubes as well as hindered amine light stabilisers (HALS) that are effective with commodity polymers might also be good stabilizers for the P3HT polymer.224 A better understanding of the photostability of materials used to produce photovoltaic panels will lead to improved technologies for generating clean energy that can help mitigate the effects of climate change and the interactions with ozone depletion.
• Recent studies show that the effectiveness of nanoscale fillers as light stabilisers in plastics varies with the type and chemistry of the nanocomposite. Nanoscale fillers (ultrafine particle additives) are increasingly used in formulations of plastics to obtain superior strength and mechanical properties. These are also expected to act as solar UV-stabilisers. Nanoscale zinc oxide (ZnO)-filled polyurethane coatings on steel225 or in thin films of polyethylene,226 nano-silica filled polyurethane coatings on polyethylene film227 and nano-ZnO or nano-titanium dioxide (TiO2) in clear wood coatings228 all result in improved photostability of the underlying substrate. The coatings retard the degradation of the surfaces from exposure to solar UV radiation. However, in bulk polyethylenes filled with nanofillers of ZnO,229 clay or silica,230 and in polypropylene filled with nanosized calcium carbonate,231 a decrease in photostability has been observed. These data suggest that the efficacy of nanofillers as light stabilisers observed in coatings or thin films cannot be generalised to include all bulk polymer nanocomposite systems.
• Wood–plastic composites with a polyethylene surface layer protect the material from UV degradation. Better technologies that control surface discoloration of wood following exposure to solar UV will increase the service life of these products. Recent work shows that bark extractives (compounds extractible by solvents) incorporated into surface coating formulations act as environmentally friendly stabilisers, and retard the UV-induced discoloration of wood.232 A hydrophobic polyethylene surface cap layer on wood reduces the loss of natural extractives that act as antioxidants in wood/polyethylene composites.233 As with surface wax treatment234 or oxide-filled coatings235 that stabilise wood against discoloration and reduction of strength, the cap layer also limits intrusion of water into the wood that otherwise promotes biodegradation.
• A recent model that reliably predicts the surface temperatures reached by different materials exposed to solar radiation outdoors can be used to better interpret weathering data.236 Improved modelling of the weathering of materials is critical for designing better light-stabiliser technologies. Rates of UV-induced damage processes in materials increase with temperature221 and will therefore also be affected by climate change. Using the surface temperatures of plastics exposed to solar radiation in place of the ambient air temperatures in weathering studies results in more accurate estimates of the photoreaction rates.
Acknowledgements
The Environmental Effects Assessment Panel gratefully acknowledges the Swiss Federal Office for the Environment (FOEN), International Affairs Division, for sponsorship of the report-writing meeting of the Panel held in Basel in August–September 2011. Funding for authors' travel was provided by: UNEP (PJA, ATA, CLB, WH, MI, HHR, MS); Bundesministerium für Umwelt, Naturschutz und Reaktorsicherheit (DPH); DEFRA (MN, NDP); NZ International Technical Input Programme (JFB, RLM); NSF DEB #0734277 & NSF DGE IGERT #0903560 (CEW); University of Wollongong & Wiley Blackwell Publishers (SR); U.S. Global Change Research Program (ALA, MC, DJE, JL, SM, CEW, RCW); EC Integrated Project SCOUT-O3, contract 505390-GOCE-CT-2004 (AFB).
References
- P. A. Newman and R. McKenzie, UV impacts avoided by the Montreal Protocol, Photochem. Photobiol. Sci., 2011, 10, 1152–1160 CAS.
- M. Salby, E. Titova and L. Deschamps, Rebound of Antarctic ozone, Geophys. Res. Lett., 2011, 38, L09702 CrossRef.
- S. B. Feldstein, Subtropical Rainfall and the Antarctic Ozone Hole, Science, 2011, 332, 925–926 CrossRef CAS.
- S.-W. Son, E. P. Gerber, J. Perlwitz, L. M. Polvani, N. Gillett, K.-H. Seo, V. Eyring, T. G. Shepherd, D. Waugh, H. Akiyoshi, J. Austin, A. Baumgaertner, S. Bekki, P. Braesicke, C. Brühl, N. Butchart, M. Chipperfield, D. Cugnet, M. Dameris, S. Dhomse, S. Frith, H. Garny, R. Garcia, S. C. Hardiman, P. Jöckel, J.-F. Lamarque, E. Mancini, M. Marchand, M. Michou, T. Nakamura, O. Morgenstern, G. Pitari, D. A. Plummer, J. Pyle, E. Rozanov, J. F. Scinocca, K. Shibata, D. Smale, H. Teyssèdre, W. Tian and Y. Yamashita, The impact of stratospheric ozone on the Southern Hemisphere circulation change: A multimodel assessment, J. Geophys. Res. Atmos., 2010, 115, D00M07 CrossRef.
- S. M. Kang, L. M. Polvani, J. C. Fyfe and M. Sigmond, Impact of polar ozone depletion on subtropical precipitation, Science, 2011, 332, 951–954 CrossRef CAS.
- G. L. Manney, M. L. Santee, M. Rex, N. J. Livesey, M. C. Pitts, P. Veefkind, E. R. Nash, I. Wohltmann, R. Lehmann, L. Froidevaux, L. R. Poole, M. R. Schoeberl, D. P. Haffner, J. Davies, V. Dorokhov, H. Gernandt, B. Johnson, R. Kivi, E. Kyro, N. Larsen, P. F. Levelt, A. Makshtas, C. T. McElroy, H. Nakajima, M. C. Parrondo, D. W. Tarasick, P. von der Gathen, K. A. Walker and N. S. Zinoviev, Unprecedented Arctic ozone loss in 2011, Nature, 2011, 478, 469–475 CrossRef CAS.
- A. F. Bais, K. Tourpali, A. Kazantzidis, H. Akiyoshi, S. Bekki, P. Braesicke, M. P. Chipperfield, M. Dameris, V. Eyring, H. Garny, D. Iachetti, P. Jöckel, A. Kubin, U. Langematz, E. Mancini, M. Michou, O. Morgenstern, T. Nakamura, P. A. Newman, G. Pitari, D. A. Plummer, E. Rozanov, T. G. Shepherd, K. Shibata, W. Tian and Y. Yamashita, Projections of UV radiation changes in the 21st century: impact of ozone recovery and cloud effects, Atmos. Chem. Phys. Discuss., 2011, 11, 10769–10797 CrossRef.
- S. J Pope and D. E. Godar, Solar UV geometric conversion factors: Horizontal plane to cylinder model dagger, Photochem. Photobiol., 2010, 86, 457–466 CrossRef CAS.
- M. Norval, L. O. Björn and F. R. de Gruijl, Is the action spectrum for the UV-induced production of previtamin D3 in human skin correct?, Photochem. Photobiol. Sci., 2010, 9, 11–17 CAS.
- T. Kotilainen, A. Lindfors, R. Tegelberg and P. J. Aphalo, How realistically does outdoor UV-B supplementation with lamps reflect ozone depletion: An assessment of enhancement errors, Photochem. Photobiol., 2011, 87, 174–183 CrossRef CAS.
- M. R. Zaidi, S. Davis, F. P. Noonan, C. Graff-Cherry, T. S. Hawley, R. L. Walker, L. Feigenbaum, E. Fuchs, L. Lyakh, H. A. Young, T. J. Hornyak, H. Arnheiter, G. Trinchieri, P. S. Meltzer, E. C. De Fabo and G. Merlino, Interferon-gamma links ultraviolet radiation to melanomagenesis in mice, Nature, 2011, 469, 548–553 CrossRef CAS.
- D. L. Mitchell, A. A. Fernandez, R. S. Nairn, R. Garcia, L. Paniker, D. Trono, H. D. Thames and I. Gimenez-Conti, Ultraviolet A does not induce melanomas in a Xiphophorus hybrid fish model, Proc. Natl. Acad. Sci. U. S. A., 2010, 107, 9329–9334 CrossRef CAS.
- N. Fuglede, U. Brinck-Claussen, I. Deltour, E. Boesen, S. Dalton and C. Johansen, Incidence of cutaneous malignant melanoma in Denmark, 1978–2007, Br. J. Dermatol., 2011, 165, 349–353 CrossRef CAS.
- S. Wallingford, R. Alston, J. Birch and A. Green, Increases in invasive melanoma in England, 1979–2006, by anatomic site, Br. J. Dermatol., 2011, 165, 859–864 CrossRef CAS.
- A. Klit, J. Drejoe and K. Drzewiecki, Trends in the incidence of malignant melanoma in Denmark 1978–2007. Incidence on the island of Bornholm compared with the whole country incidence in Denmark, Dan. Med. Bull., 2011, 58, A4229 Search PubMed.
- E. Cicarma, A. Juzeniene, A. C. Porojnicu, O. S. Bruland and J. Moan, Latitude gradient for melanoma incidence by anatomic site and gender in Norway 1966–2007, J. Photochem. Photobiol., B, 2010, 101, 174–178 CrossRef CAS.
- R. M. Lucas, An epidemiological perspective of ultraviolet exposure-public health concerns, Eye Contact Lens Sci. Clin. Pract., 2011, 37, 168–175 CrossRef.
- L. M. Hollestein, S. A. van den Akker, T. Nijsten, H. E. Karim-Kos, J. W. Coebergh and E. de Vries, Trends of cutaneous melanoma in The Netherlands: increasing incidence rates among all Breslow thickness categories and rising mortality rates since 1989, Ann. Oncol., 2011 DOI:10.1093/annonc/mdr128.
- P. Baade, X. Meng, D. Youlden, J. Aitken and P. Youl, Time trends and latitudinal differences in melanoma thickness distribution in Australia, 1990–2006, Int. J. Cancer, 2011, 130, 170–178 CrossRef.
- V. D. Criscione and M. A. Weinstock, Melanoma thickness trends in the United States, 1988–2006, J. Invest. Dermatol., 2009, 130, 793–797 CrossRef.
- J. Bauer and C. Garbe, Acquired melanocytic nevi as risk factor for melanoma development. A comprehensive review of epidemiological data, Pigm. Cell Res., 2003, 16, 297–306 CrossRef.
- E. Mahe, A. Beauchet, M. de Paula Correa, S. Godin-Beekmann, M. Haeffelin, S. Bruant, F. Fay-Chatelard, F. Jegou, P. Saiag and P. Aegerter, Outdoor sports and risk of UV-related skin lesions in children: evaluation of risks, and prevention, Br. J. Dermatol., 2011, 165, 360–367 CrossRef CAS.
- M. A. Karlsson, C. F. Wahlgren, K. Wiklund and Y. Rodvall, Parental sun-protective regimens and prevalence of common melanocytic naevi among 7-year-old children in Sweden: changes over a 5-year period, Br. J. Dermatol., 2011, 164, 830–837 CrossRef CAS.
- G. Custodio, L. H. Locks, M. F. Coan, C. O. Goncalves, D. J. Trevisol and F. S. Trevisol, Epidemiology of basal cell carcinomas in Tubarao, Santa Catarina (SC), Brazil between 1999 and 2008, An. Bras. Dermatol., 2010, 85, 819–826 CrossRef.
- E. M. De La Torre-Lugo, L. D. Figueroa, J. L. Sanchez, A. Morales-Burgos and D. Conde, Skin cancer in Puerto Rico: a multiannual incidence comparative study, Puerto Rico Health Sci. J., 2010, 29, 312–316 Search PubMed.
- S. C. Flohil, S. Koljenovic, E. R. de Haas, L. I. Overbeek, E. de Vries and T. Nijsten, Cumulative risks and rates of subsequent basal cell carcinomas in the Netherlands, Br. J. Dermatol., 2011, 165, 874–881 CrossRef CAS.
- J. Schmitt, A. Seidler, T. L. Diepgen and A. Bauer, Occupational ultraviolet light exposure increases the risk for the development of cutaneous squamous cell carcinoma: a systematic review and meta-analysis, Br. J. Dermatol., 2011, 164, 291–307 CrossRef CAS.
- A. Bauer, T. Diepgen and J. Schmitt, Is occupational solar UV-irradiation a relevant risk factor for basal cell carcinoma? A systematic review and meta-analysis of the epidemiologic literature, Br. J. Dermatol., 2011, 165, 612–625 CAS.
- L. Kenborg, A. D. Jorgensen, E. Budtz-Jorgensen, L. E. Knudsen and J. Hansen, Occupational exposure to the sun and risk of skin and lip cancer among male wage earners in Denmark: a population-based case-control study, Cancer, Causes Control, 2010, 21, 1347–1355 CrossRef.
- P. M. Nthumba, P. C. Cavadas and L. Landin, Primary cutaneous malignancies in sub-Saharan Africa, Ann. Plast. Surg., 2011, 66, 313–320 CrossRef CAS.
- K. G. Paulson, J. G. Iyer and P. Nghiem, Asymmetric lateral distribution of melanoma and Merkel cell carcinoma in the United States, J. Am. Acad. Dermatol., 2011, 65, 35–39 CrossRef.
- J. Girschik, K. Thorn, T. W. Beer, P. J. Heenan and L. Fritschi, Merkel Cell carcinoma in Western Australia: A population-based study of incidence and survival, Br. J. Dermatol., 2011, 165, 1051–1057 CrossRef CAS.
- S. Kuwamoto, Recent advances in the biology of Merkel cell carcinoma, Hum. Pathol., 2011, 42, 1063–1077 CrossRef CAS.
- A. Mogha, A. Fautrel, N. Mouchet, N. Guo, S. Corre, H. Adamski, E. Watier, L. Misery and M. D. Galibert, Merkel cell polyomavirus small T antigen mRNA level is increased following in vivo UV-radiation, PLoS One, 2010, 5, e11423 Search PubMed.
- J. Chui, M. T. Coroneo, L. T. Tat, R. Crouch, D. Wakefield and N. Di Girolamo, Ophthalmic pterygium: a stem cell disorder with premalignant features, Am. J. Pathol., 2011, 178, 817–827 CrossRef.
- D. N. Hu and S. A. McCormick, Progress in the studies of etiology, epidemiology and pathogenesis of ocular melanomas, Yan Ke Xue Bao, 2011, 26, 18–22 Search PubMed.
- B. E. Klein, K. E. Lee, L. G. Danforth, T. M. Schaich, K. J. Cruickshanks and R. Klein, Selected sun-sensitizing medications and incident cataract, Arch. Ophthalmol., 2010, 128, 959–963 CrossRef.
- H. Sasaki, Y. Sakamoto, C. Schnider, N. Fujita, N. Hatsusaka, D. H. Sliney and K. Sasaki, UV-B Exposure to the Eye Depending on Solar Altitude, Eye Contact Lens Sci. Clin. Pract., 2011, 37, 191–195 CrossRef.
- J. K. Lai, R. M. Lucas, E. Banks and A. L. Ponsonby, Variability in vitamin D assays impairs clinical assessment of vitamin D status, Intern. Med. J., 2011 DOI:10.1111/j.1445-5994.2011.02471.x.
- M. Nowak, S. L. Harrison, P. G. Buettner, M. Kimlin, D. Porter, L. Kennedy and R. Speare, Vitamin D status of adults from tropical Australia determined using two different laboratory assays: Implications for public health messages, Photochem. Photobiol., 2011, 87, 935–943 CrossRef CAS.
- J. C. Dowdy, R. M. Sayre and M. F. Holick, Holick's rule and vitamin D from sunlight, J. Steroid Biochem. Mol. Biol., 2010, 121, 328–330 CrossRef CAS.
- R. L. McKenzie, P. J. Aucamp, A. F. Bais, L. O. Bjorn, M. Ilyas and S. Madronich, Ozone depletion and climate change: impacts on UV radiation, Photochem. Photobiol. Sci., 2011, 10, 182–198 CAS.
- B. L. Diffey, Modelling the seasonal variation of vitamin D due to sun exposure, Br. J. Dermatol., 2010, 162, 1342–1348 CrossRef CAS.
- L. E. Rhodes, A. R. Webb, H. I. Fraser, R. Kift, M. T. Durkin, D. Allan, S. J. O'Brien, A. Vail and J. L. Berry, Recommended summer sunlight exposure levels can produce sufficient (> or = 20 ng ml(-1)) but not the proposed optimal (> or = 32 ng ml(-1)) 25(OH)D levels at UK latitudes, J. Invest. Dermatol., 2010, 130, 1411–1418 CrossRef CAS.
- A. R. Webb, R. Kift, M. T. Durkin, S. J. O'Brien, A. Vail, J. L. Berry and L. E. Rhodes, The role of sunlight exposure in determining the vitamin D status of the U.K. white adult population, Br. J. Dermatol., 2010, 163, 1050–1055 CrossRef CAS.
- K. Nakamura, M. Nashimoto and M. Yamamoto, Summer/winter differences in the serum 25-hydroxyvitamin D3 and parathyroid hormone levels of Japanese women, Int. J. Biometeorol., 2000, 44, 186–189 CrossRef CAS.
- E. Thieden, P. A. Philipsen, J. Heydenreich and H. C. Wulf, Vitamin D level in summer and winter related to measured UVR exposure and behavior, Photochem. Photobiol., 2009, 85, 1480–1484 CrossRef CAS.
- H. E. Hanwell, R. Vieth, D. E. Cole, A. Scillitani, S. Modoni, V. Frusciante, G. Ritrovato, I. Chiodini, S. Minisola and V. Carnevale, Sun exposure questionnaire predicts circulating 25-hydroxyvitamin D concentrations in Caucasian hospital workers in southern Italy, J. Steroid Biochem. Mol. Biol., 2010, 121, 334–337 CrossRef CAS.
- B. R. Becklund, K. S. Severson, S. V. Vang and H. F. Deluca, UV radiation suppresses experimental autoimmune encephalomyelitis independent of vitamin D production, Proc. Natl. Acad. Sci. U. S. A., 2010, 107, 6418–6423 CrossRef CAS.
- R. M. Lucas, A. L. Ponsonby, K. Dear, P. C. Valery, M. P. Pender, B. V. Taylor, T. J. Kilpatrick, T. Dwyer, A. Coulthard, C. Chapman, I. von der Mei, D. Williams and A. J. McMichael, Sun exposure and vitamin D are independent risk factors for CNS demyelination, Neurology, 2011, 76, 540–548 CrossRef CAS.
- S. M. Orton, L. Wald, C. Confavreux, S. Vukusic, J. P. Krohn, S. V. Ramagopalan, B. M. Herrera, A. D. Sadovnick and G. C. Ebers, Association of UV radiation with multiple sclerosis prevalence and sex ratio in France, Neurology, 2011, 76, 425–431 CrossRef.
- S. V. Ramagopalan, A. E. Handel, G. Giovannoni, S. Rutherford Siegel, G. C. Ebers and G. Chaplin, Relationship of UV exposure to prevalence of multiple sclerosis in England, Neurology, 2011, 76, 1410–1414 CrossRef CAS.
- S. Sloka, C. Silva, W. Pryse-Phillips, S. Patten, L. Metz and V. W. Yong, A quantitative analysis of suspected environmental causes of MS, Can. J. Neurol. Sci., 2011, 38, 98–105 Search PubMed.
- H. F. Deluca and L. A. Plum, Vitamin D deficiency diminishes the severity and delays onset of experimental autoimmune encephalomyelitis, Arch. Biochem. Biophys., 2011, 513, 140–143 CrossRef CAS.
- D. A. Fernandes de Abreu, E. C. Ibrahim, J. Boucraut, M. Khrestchatisky and F. Feron, Severity of experimental autoimmune encephalomyelitis is unexpectedly reduced in mice born to vitamin D-deficient mothers, J. Steroid Biochem. Mol. Biol., 2010, 121, 250–253 CrossRef CAS.
- M. Stein, Y. Liu, O. Gray, J. Baker, S. Kolbe, M. Ditchfield, G. Egan, P. Mitchell, L. Harrison, H. Butzkeuven and T. Kilpatrick, A randomized trial of high-dose vitamin D2 in relapsing remitting multiple sclerosis, Neurology, 2011, 77, 1611–1618 CrossRef CAS.
- Z. S. Bonakdar, L. Jahanshahifar, F. Jahanshahifar and A. Gholamrezaei, Vitamin D deficiency and its association with disease
activity in new cases of systemic lupus erythematosus, Lupus, 2011, 20, 1155–1160 CrossRef CAS.
- L. L. Ritterhouse, S. R. Crowe, T. B. Niewold, D. L. Kamen, S. R. Macwana, V. C. Roberts, A. B. Dedeke, J. B. Harley, R. H. Scofield, J. M. Guthridge and J. A. James, Vitamin D deficiency is associated with an increased autoimmune response in healthy individuals and in patients with systemic lupus erythematosus, Ann. Rheum. Dis., 2011, 70, 1569–1574 CrossRef CAS.
- P. G. Lindqvist, H. Olsson and M. Landin-Olsson, Are active sun exposure habits related to lowering risk of type 2 diabetes mellitus in women, a prospective cohort study?, Diabetes Res. Clin. Pract., 2010, 90, 109–114 CrossRef.
- C. Gagnon, Z. X. Lu, D. J. Magliano, D. W. Dunstan, J. E. Shaw, P. Z. Zimmet, K. Sikaris, N. Grantham, P. R. Ebeling and R. M. Daly, Serum 25-hydroxyvitamin D, calcium intake, and risk of type 2 diabetes after 5 years: results from a national, population-based prospective study (the Australian Diabetes, Obesity and Lifestyle study), Diabetes Care, 2011, 34, 1133–1138 CrossRef CAS.
- S. Sloka, M. Grant and L. A. Newhook, The geospatial relation between UV solar radiation and type 1 diabetes in Newfoundland, Acta Diabetol. Lat., 2010, 47, 73–78 CrossRef.
- R. J. Mullins, S. Clark, C. Katelaris, V. Smith, G. Solley and C. A. Camargo Jr, Season of birth and childhood food allergy in Australia, Pediatr. Allergy Immu., 2011, 22, 583–589 CrossRef.
- A. M. Hughes, R. M. Lucas, A. L. Ponsonby, C. Chapman, A. Coulthard, K. Dear, T. Dwyer, T. J. Kilpatrick, A. J. McMichael, M. P. Pender, B. V. Taylor, P. Valery, I. A. van der Mei and D. Williams, The role of latitude, ultraviolet radiation exposure and vitamin D in childhood asthma and hayfever: an Australian multicenter study, Pediatr. Allergy Immunol., 2011, 22, 327–333 CrossRef.
- G. Krstic, Asthma prevalence associated with geographical latitude and regional insolation in the United States of America and Australia, PLoS One, 2011, 6, e18492 CAS.
- A. Fares, Seasonality of tuberculosis, J. Global Infect. Dis., 2011, 3, 46–55 CrossRef.
- A. R. Martineau, P. M. Timms, G. H. Bothamley, Y. Hanifa, K. Islam, A. P. Claxton, G. E. Packe, J. C. Moore-Gillon, M. Darmalingam, R. N. Davidson, H. J. Milburn, L. V. Baker, R. D. Barker, N. J. Woodward, T. R. Venton, K. E. Barnes, C. J. Mullett, A. K. Coussens, C. M. Rutterford, C. A. Mein, G. R. Davies, R. J. Wilkinson, V. Nikolayevskyy, F. A. Drobniewski, S. M. Eldridge and C. J. Griffiths, High-dose vitamin D(3) during intensive-phase antimicrobial treatment of pulmonary tuberculosis: a double-blind randomised controlled trial, Lancet, 2011, 377, 242–250 CrossRef CAS.
- J. R. Sabetta, P. DePetrillo, R. J. Cipriani, J. Smardin, L. A. Burns and M. L. Landry, Serum 25-hydroxyvitamin D and the incidence of acute viral respiratory tract infections in healthy adults, PLoS One, 2010, 5, e11088 Search PubMed.
- R. M. Lucas, A. L. Ponsonby, K. Dear, P. Valery, M. P. Pender, J. M. Burrows, S. R. Burrows, C. Chapman, A. Coulthard, D. E. Dwyer, T. Dwyer, T. Kilpatrick, M. L. Lay, A. J. McMichael, B. V. Taylor, I. A. van der Mei and D. Williams, Current and past Epstein-Barr virus infection in risk of initial CNS demyelination, Neurology, 2011, 77, 371–379 CrossRef CAS.
- S. R. Zwart, S. K. Mehta, R. Ploutz-Snyder, Y. Bourbeau, J. P. Locke, D. L. Pierson and S. M. Smith, Response to vitamin D supplementation during Antarctic winter is related to BMI, and supplementation can mitigate Epstein-Barr Virus reactivation, J. Nutr., 2011, 141, 692–697 CrossRef CAS.
- P. Tuohimaa, L. Tenkanen, M. Ahonen, S. Lumme, E. Jellum, G. Hallmans, P. Stattin, S. Harvei, T. Hakulinen, T. Luostarinen, J. Dillner, M. Lehtinen and M. Hakama, Both high and low levels of blood vitamin D are associated with a higher prostate cancer risk: a longitudinal, nested case-control study in the Nordic countries, Int. J. Cancer, 2004, 108, 104–108 CrossRef CAS.
- N. O. Nielsen, T. Skifte, M. Andersson, J. Wohlfahrt, B. Soborg, A. Koch, M. Melbye and K. Ladefoged, Both high and low serum vitamin D concentrations are associated with tuberculosis: A case-control study in Greenland, Br. J. Nutr., 2010, 104, 1487–1491 CrossRef CAS.
- P. Tuohimaa, Vitamin D and aging, J. Steroid Biochem. Mol. Biol., 2009, 114, 78–84 CrossRef CAS.
- D. M. Freedman, A. C. Looker, C. C. Abnet, M. S. Linet and B. I. Graubard, Serum 25-hydroxyvitamin D and cancer mortality in the NHANES III study (1988–2006), Cancer Res., 2010, 70, 8587–8597 CrossRef CAS.
- M. L. Melamed, E. D. Michos, W. Post and B. Astor, 25-hydroxyvitamin D levels and the risk of mortality in the general population, Arch. Intern. Med., 2008, 168, 1629–1637 CrossRef.
- A. C. Green, G. M. Williams, V. Logan and G. M. Strutton, Reduced melanoma after regular sunscreen use: randomized trial follow-up, J. Clin. Oncol., 2010, 29, 257–263 CrossRef.
- P. D. Baade, A. C. Green, B. M. Smithers and J. F. Aitken, Trends in melanoma incidence among children: possible influence of sun-protection programs, Expert Rev. Anti-Infect. Ther., 2011, 11, 661–664 CrossRef.
- B. Koster, C. Thorgaard, A. Philip and I. H. Clemmensen, Vacations to sunny destinations, sunburn, and intention to tan: a cross-sectional study in Denmark, 2007–2009, Scand. J. Public Health, 2011, 39, 64–69 CrossRef.
- J. Morris, T. Laing-Morton, P. Marno and A. Curnow, An investigation into the awareness and understanding of the ultraviolet index forecasts in the South West of England, Photochem. Photobiol. Sci., 2011, 10, 103–108 CAS.
- D. H. Sliney, Intraocular and crystalline lens protection from ultraviolet damage, Eye Contact Lens Sci. Clin. Pract., 2011, 37, 250–258 CrossRef.
- J. E. Roberts, Ultraviolet radiation as a risk factor for cataract and macular degeneration, Eye Contact Lens Sci. Clin. Pract., 2011, 37, 246–249 CrossRef.
- F. Afaq, Natural agents: cellular and molecular mechanisms of photoprotection, Arch. Biochem. Biophys., 2011, 508, 144–151 CrossRef CAS.
- D. R. Sambandan and D. Ratner, Sunscreens: an overview and update, J. Am. Acad. Dermatol., 2011, 64, 748–758 CrossRef CAS.
- G. Sivapirabu, E. Yiasemides, G. M. Halliday, J. Park and D. L. Damian, Topical nicotinamide modulates cellular energy metabolism and provides broad-spectrum protection against ultraviolet radiation-induced immunosuppression in humans, Br. J. Dermatol., 2009, 161, 1357–1364 CrossRef CAS.
- A. Hofer, F. J. Legat, A. Gruber-Wackernagel, F. Quehenberger and P. Wolf, Topical liposomal DNA-repair enzymes in polymorphic light eruption, Photochem. Photobiol. Sci., 2011, 10, 1118–1128 CAS.
- S. M. Pilkington, R. E. Watson, A. Nicolaou and L. E. Rhodes, Omega-3 polyunsaturated fatty acids: photoprotective macronutrients, Exp. Dermatol., 2011, 20, 537–543 CrossRef CAS.
- B. Y. Chen, D. P. Lin, K. C. Su, Y. L. Chen, C. Y. Wu, M. C. Teng, Y. T. Tsai, C. Y. Sun, S. R. Wang and H. H. Chang, Dietary zerumbone prevents against ultraviolet B-induced cataractogenesis in the mouse, Mol. Vis., 2011, 17, 723–730 CAS.
- SNAP, Summary of substitute refrigerants listed in SNAP Notice 25. Significant New Alternatives Policy Program, Environmental Protection Agency, http://www.epa.gov/ozone/snap/refrigerants/Notice25SubstituteRefrigerants.pdf, accessed 2011.
- SNAP, Summary of Substitute Foam Blowing Agents Listed in SNAP Notice 25, Significant New Alternatives Policy Program, Environmental Protection Agency, http://www.epa.gov/ozone/snap/foams/Notice25SubstituteFoams.pdf, accessed 2011.
- SNAP, Halon Substitutes Under SNAP as of January 2, 2009, Significant New Alternatives Policy Program, Environmental Protection Agency, http://www.epa.gov/ozone/snap/fire/halon.pdf, accessed 2011.
- P. Nordly, K. S. Korsholm, E. A. Pedersen, T. S. Khilji, H. Franzyk, L. Jorgensen, H. M. Nielsen, E. M. Agger and C. Foged, Incorporation of a synthetic mycobacterial monomycoloyl glycerol analogue stabilizes dimethyldioctadecylammonium liposomes and potentiates their adjuvant effect in vivo, Eur. J. Pharm. Biopharm., 2011, 77, 89–98 CrossRef CAS.
- C. E. Williamson and K. C. Rose, Environmental science. When UV meets fresh water, Science, 2010, 329, 637–639 CrossRef CAS.
- Y. H. Hsieh and C. W. Chen, Turning points, reproduction number, and impact of climatological events for multi-wave dengue outbreaks, Trop. Med. Int. Health, 2009, 14, 628–638 CrossRef.
- P. E. Sheffield and P. J. Landrigan, Global climate change and children's health: threats and strategies for prevention, Environ. Health Perspect., 2010, 119, 291–298 CrossRef.
- P. W. Gething, D. L. Smith, A. P. Patil, A. J. Tatem, R. W. Snow and S. I. Hay, Climate change and the global malaria recession, Nature, 2010, 465, 342–345 CrossRef CAS.
- P. S. Searles, S. D. Flint and M. M. Caldwell, A meta analysis of plant field studies simulating stratospheric ozone depletion, Oecologia, 2001, 127, 1–10 CrossRef.
- C. L. Ballaré, C. M. Rousseaux, P. S. Searles, J. G. Zaller, C. V. Giordano, M. T. Robson, M. M. Caldwell, O. E. Sala and A. L. Scopel, Impacts of solar ultraviolet-B radiation on terrestrial ecosystems of Tierra del Fuego (southern Argentina). An overview of recent progress, J. Photochem. Photobiol., B, 2001, 62, 67–77 CrossRef.
- K. K. Newsham and S. A. Robinson, Responses of plants in polar regions to UVB exposure: a meta-analysis, Global Change Biol., 2009, 15, 2574–2589 CrossRef.
- F. R. Li, S. L. Peng, B. M. Chen and Y. P. Hou, A meta-analysis of the responses of woody and herbaceous plants to elevated ultraviolet-B radiation, Acta Oecol., 2010, 36, 1–9 CrossRef.
- C. L. Ballaré, M. M. Caldwell, S. D. Flint, S. A. Robinson and J. F. Bornman, Effects of solar ultraviolet radiation on terrestrial ecosystems. Patterns, mechanisms, and interactions with climate change, Photochem. Photobiol. Sci., 2011, 10, 226–241 Search PubMed.
- J. Perlwitz, S. Pawson, R. L. Fogt, J. E. Nielsen and W. D. Neff, Impact of stratospheric ozone hole recovery on Antarctic climate, Geophys. Res. Lett., 2008, 35, L08714, DOI:10.1029/2008GL033317.
- L. J. Clarke, S. A. Robinson, Q. Hua, D. J. Ayre and D. Fink, Radiocarbon bomb spike reveals biological effects of Antarctic climate change, Glob. Change Biol., 2011 DOI:10.1111/j.1365-2486.2011.02560.x.
- D. A. Hodgson, D. Roberts, A. McMinn, E. Verleyen, B. Terry, C. Corbett and W. Vyverman, Recent rapid salinity rise in three East Antarctic lakes, J. Paleolimnol., 2006, 36, 385–406 CrossRef.
- F. Kuhlmann and C. Müller, UV-B impact on aphid performance mediated by plant quality and plant changes induced by aphids, Plant Biol., 2010, 12, 676–684 CAS.
- P. V. Demkura, G. Abdala, I. T. Baldwin and C. L. Ballaré, Jasmonate-dependent and -independent pathways mediate specific effects of solar ultraviolet B radiation on leaf phenolics and antiherbivore
defense, Plant Physiol., 2009, 152, 1084–1095 CrossRef.
- M. Fujita, Y. Fujita, Y. Noutoshi, F. Takahashi, Y. Narusaka, K. Yamaguchi-Shinozaki and K. Shinozaki, Crosstalk between abiotic and biotic stress responses: a current view from the points of convergence in the stress signaling networks, Curr. Opin. Plant Biol., 2006, 9, 436–442 CrossRef.
- F. Y. Cao, K. Yoshioka and D. Desveaux, The roles of ABA in plant-pathogen interactions, J. Plant Res., 2011, 124, 489–499 CrossRef CAS.
- K. R. S. Snell, T. Kokubun, H. Griffiths, P. Convey, D. A. Hodgson and K. K. Newsham, Quantifying the metabolic cost to an Antarctic liverwort of responding to an abrupt increase in UVB radiation exposure, Global Change Biol., 2009, 15, 2563–2573 CrossRef.
- R. W. Hofmann and B. D. Campbell, Response of Trifolium repens to UV-B radiation: Morphological links to plant productivity and water availability, Plant Biol., 2011, 13, 896–901 CrossRef CAS.
- A. Onzo, M. W. Sabelis and R. Hanna, Effects of ultraviolet radiation on predatory mites and the role of refuges in plant structures, Environ. Entomol., 2010, 39, 695–701 CrossRef.
- Y. Sakai and M. Osakabe, Spectrum-specific damage and solar ultraviolet radiation avoidance in the two-spotted spider mite, Photochem. Photobiol., 2010, 86, 925–932 CrossRef CAS.
- L. Rizzini, J. J. Favory, C. Cloix, D. Faggionato, A. O'Hara, E. Kaiserli, R. Baumeister, E. Schäfer, F. Nagy, G. I. Jenkins and R. Ulm, Perception of UV-B by the Arabidopsis UVR8 protein, Science, 2011, 332, 103–106 CrossRef CAS.
- M. Wu, E. Grahn, L. A. Eriksson and A. Strid, Computational evidence for the role of Arabidopsis thaliana UVR8 as UV-B photoreceptor and identification of its chromophore amino acids, J. Chem. Inf. Model., 2011, 51, 1287–1295 CrossRef CAS.
- H. Gruber, M. Heijde, W. Heller, A. Albert, H. K. Seidlitz and R. Ulm, Negative feedback regulation of UV-B-induced photomorphogenesis and stress acclimation in Arabidopsis, Proc. Natl. Acad. Sci. U. S. A., 2010, 107, 20132–20137 CrossRef CAS.
- B. Fehér, L. Kozma-Bognár, É. Kevei, A. Hajdu, M. Binkert, S. J. Davis, E. Schäfer, R. Ulm and F. Nagy, Functional interaction of the circadian clock and UV RESISTANCE LOCUS 8-controlled UV-B signaling pathways in Arabidopsis thaliana, Plant J., 2011, 67, 37–48 CrossRef.
- E. B. Barbier, S. D. Hacker, C. Kennedy, E. W. Koch, A. C. Stier and B. R. Silliman, The value of estuarine and coastal ecosystem services, Ecol. Monogr., 2011, 81, 169–193 CrossRef.
-
A. Fischlin and G. F. Midgley, Ecosystems, their properties, goods and services, in Contribution of Working Group II to the Fourth Assessment Report of the Intergovernmental Panel on Climate Change, 2007 ed., M. L. Parry, O. F. Canziani, J. P. Palutikof, P. J. van der Linden and C. E. Hanson, Cambridge University Press, Cambridge, United Kingdom and New York, NY, USA, 2007, pp. 212–272 Search PubMed.
-
C. H. Sekercioglu, Chapter 3. Ecosystem functions and services, in Conservation Biology for All ed.: N. S. Sodhi and P. R. Ehrlich, Oxford University Press, Oxford, 2010, pp. 45–73 Search PubMed.
-
R. Haines-Young and M. Potschin, The Links Between Biodiversity, Ecosystem Services and Human Well-being, in Ecosystem Ecology: A New Synthesis, ed., D. G. Raffaelli and C. L. J. Frid, Cambridge University Press, 2010, pp. 210–239 Search PubMed.
- J. M. Montoya and D. Raffaelli, Climate change, biotic interactions and ecosystem services, Philos. Trans. R. Soc. London, Ser. B, 2010, 365, 2013–2018 CrossRef.
- D.-P. Häder, Does enhanced solar UV-B radiation affect marine primary producers in their natural habitats?, Photochem. Photobiol., 2011, 87, 263–266 CrossRef.
- H. W. Ducklow, S. C. Doney and D. K. Steinberg, Contributions of long-term research and time-series observations
to marine ecology and biogeochemistry, Annu. Rev. Mar. Sci., 2009, 1, 279–302 CrossRef.
- G. Li, K. Gao and G. Gao, Differential impacts of solar UV radiation on photosynthetic carbon fixation from the coastal to offshore surface waters in the South China Sea, Photochem. Photobiol., 2011, 87, 329–334 CrossRef CAS.
- Group on Earth Observations, What is GEOSS?: The global earth observation system of systems, Report No., Geneva, Switzerland, 2011, http://www.earthobservations.org/geoss.shtml.
- T. Westberry, M. J. Behrenfeld, D. A. Siegel and E. Boss, Carbon-based primary productivity modeling with vertically resolved photoacclimation, Global Biogeochem. Cycles, 2008, 22, GB2024 CrossRef.
- V. S. Saba, M. A. M. Friedrichs, D. Antoine, R. A. Armstrong, I. Asanuma, M. J. Behrenfeld, A. M. Ciotti, M. Dowell, N. Hoepffner, K. J. W. Hyde, J. Ishizaka, T. Kameda, J. Marr, F. Mélin, A. Morel, J. O'Reilly, M. Scard, W. O. Smith Jr, T. J. Smyt, S. Tan, J. Uitz, K. Waters and T. K. Westberry, An evaluation of ocean color model estimates of marine primary productivity in coastal and pelagic regions across the globe, Biogeosciences, 2011, 8, 489–503 CrossRef CAS.
-
J. E. Keister, D. L. Pascual, J. L. Clasen, K. N. Hopfensperger, N. Kelly, J. K. Llopiz, S. M. Moseman and L. E. Petes, Climate and anthropogenic change in aquatic environments: a cross ecosystem perspective, in Eco-DAS VIII Symposium Proceedings, Chapter 1 (Ed.: P. F. Kemp), American Society of Limnology and Oceanography, Inc., University of Hawai'i, 2010, pp. 1–16 Search PubMed.
- C. L. Johnson, J. A. Runge, K. A. Curtis, E. G. Durbin, J. A. Hare, L. S. Incze, J. S. Link, G. D. Melvin, T. D. O'Brien and L. Van Guelpen, Biodiversity and ecosystem function in the Gulf of Maine: Pattern and role of zooplankton and pelagic nekton, PLoS One, 2011, 6, e16491 CAS.
- R. Bertoni, W. Jeffrey, M. Pujo-Pay, L. Oriol, P. Conan and F. Joux, Influence of water mixing on the inhibitory effect of UV radiation on primary and bacterial production in Mediterranean coastal water, Aquat. Sci., 2011, 73, 377–387 CrossRef.
- J. Raven, M. Giordano, J. Beardall and S. Maberly, Algal and aquatic plant carbon concentrating mechanisms in relation to environmental change, Photosynth. Res., 2011, 109, 281–296 CrossRef CAS.
- A. V. Vähätalo, H. Aarnos, L. Hoikkala and R. Lignell, Photochemical transformation of terrestrial dissolved organic matter supports hetero- and autotrophic production in coastal waters, Mar. Ecol.: Prog. Ser., 2011, 423, 1–14 CrossRef.
- P. Hörtnagl, M. Pérez and R. Sommaruga, Contrasting effects of ultraviolet radiation on the growth efficiency of freshwater bacteria, Aquat. Ecol., 2010, 45, 125–136 CrossRef.
- Y. Zhang, Y. Yin, E. Zhang, G. Zhu, M. Liu, L. Feng, B. Qin and X. Liu, Spectral attenuation of ultraviolet and visible radiation in lakes in the Yunnan Plateau, and the middle and lower reaches of the Yangtze River, China, Photochem. Photobiol. Sci., 2011, 10, 469–482 CAS.
- K. Xu, K. Gao, V. E. Villafañe and E. W. Helbling, Photosynthetic responses of Emiliania huxleyi to UV radiation and elevated temperature: roles of calcified coccoliths, Biogeosciences, 2011, 8, 1441–1452 CrossRef CAS.
- S. Milius, Acidification may halve coral class of 2050, Science News, 2010, 178, 10 Search PubMed.
- L. Morello, Can corals adapt to climate change and ocean acidification?, Sci. Am., 2010 Search PubMed , February 23, 2010.
- H. M. Welladsen, P. C. Southgate and K. Heimann, The effects of exposure to near-future levels of ocean acidification on shell characteristics of Pinctada fucata (Bivalvia: Pteriidae), Mollusc. Res., 2010, 30, 125–130 Search PubMed.
- S. Chen and K. Gao, Solar ultraviolet radiation and CO2-induced ocean acidification interacts to influence the photosynthetic performance of the red tide alga Phaeocystis globosa (Prymnesiophyceae), Hydrobiologia, 2011, 675, 105–117 CrossRef CAS.
- T. Hassenkam, A. Johnsson, K. Bechgaard and S. L. S. Stipp, Tracking single coccolith dissolution with picogram resolution and implications for CO2 sequestration and ocean acidification, Proc. Natl. Acad. Sci. U. S. A., 2011, 108, 8571–8576 CrossRef CAS.
- J Kleypas, R. Buddemeier and J.-P. Gattuso, The future of coral reefs in an age of global change, Int. J. Earth Sci., 2001, 90, 426–437 CrossRef CAS.
- R. A. Feely, C. L. Sabine, K. Lee, W. Berelson, J. Kleypas, V. J. Fabry and F. J. Millero, Impact of anthropogenic CO2 on the CaCO3 system in the oceans, Science, 2004, 305, 362–366 CrossRef CAS.
- M. Thyssen, G. Ferreyra, S. Moreau, I. Schloss, M. Denis and S. Demers, The combined effect of ultraviolet B radiation and temperature increase on phytoplankton dynamics and cell cycle using pulse shape recording flow cytometry, J. Exp. Mar. Biol. Ecol., 2011, 406, 95–107 CrossRef CAS.
- E. W. Helbling, A. G. J. Buma, P. Boelen, H. J. van der Strate and M. V. Fiorda, Giordanino and V. E. Villafañe, Increase in Rubisco activity and gene expression, due to elevated temperature, partially counteracts UVR-induced photoinhibition in the marine diatom Thalassiossira weissflogii, Limnol. Oceanogr., 2011, 56, 1330–1342 CrossRef CAS.
-
T. R. Karl, J. M. Melillo and T. C. Peterson, Global climate change impacts in the United States, Cambridge University Press, 2009 Search PubMed.
- M. W. Southwell, R. J. Kieber, R. N. Mead, G. B. Avery and S. A. Skrabal, Effects of sunlight on the production of dissolved organic and inorganic nutrients from resuspended sediments, Biogeochemistry, 2009, 98, 115–126 CrossRef.
- G. C. Shank, A. Evans, Y. Yamashita and R. Jaffe, Solar radiation-enhanced dissolution of particulate organic matter from coastal marine sediments, Limnol. Oceanogr., 2011, 56, 577–588 CrossRef CAS.
- Y. Zhang, Y. Yin, X. Liu, Z. Shi, L. Feng, M. Liu, G. Zhu, Z. Gong and B. Qin, Spatial-seasonal dynamics of chromophoric dissolved organic matter in Lake Taihu, a large eutrophic, shallow lake in China, Org. Geochem., 2011, 42, 510–519 CrossRef CAS.
- M. Bastidas Navarro and B. Modenutti, UVR induce optical changes and phosphorous release of lake water and macrophyte leachates in shallow Andean lakes, J. Limnol., 2010, 69, 112–119 Search PubMed.
- L. Bracchini, A. M. Dattilo, V. Hull, S. A. Loiselle, L. Nannicini, M. P. Picchi, M. Ricci, C. Santinelli, A. Seritti, A. Tognazzi and C. Rossi, Spatial and seasonal changes in optical properties of autochthonous and allochthonous chromophoric dissolved organic matter in a stratified mountain lake, Photochem. Photobiol. Sci., 2010, 9, 304–314 CAS.
- L. Bracchini, A. Tognazzi, A. M. Dattilo, F. Decembrini, C. Rossi and S. A. Loiselle, Sensitivity analysis of CDOM spectral slope in artificial and natural samples: an application in the central eastern Mediterranean Basin, Aquat. Sci., 2010, 72, 485–498 CrossRef CAS.
- J. B. Fellman, E. Hood and R. G. M. Spencer, Fluorescence spectroscopy opens new windows into dissolved organic matter dynamics in freshwater ecosystems: A review, Limnol. Oceanogr., 2010, 55, 2452–2462 CrossRef CAS.
- P. Q. Fu, K. M. G. Mostofa, F. C. Wu, C. Q. Liu, W. Li, H. Q. Liao, L. Y. Wang, J. Wang and Y. Mei, Excitation-emission matrix characterization of dissolved organic matter sources in two eutrophic lakes (Southwestern China Plateau), Geochem. J., 2010, 44, 99–112 CAS.
- R. C. Sandford, R. Bol and P. J. Worsfold, In situ determination of dissolved organic
carbon in freshwaters using a reagentless UV sensor, J. Environ. Monit., 2010, 12, 1678–1683 RSC.
- B. M. Stephens and E. C. Minor, DOM characteristics along the continuum from river to receiving basin: a comparison of freshwater and saline transects, Aquat. Sci., 2010, 72, 403–417 CrossRef CAS.
- J. Zhang, J. Hundson, R. Neal, J. Sereda, T. Clair, M. Turner, D. Jeffries, P. Dillon, L. Molot, K. Somers and R. Hesslein, Long-term patterns of dissolved organic carbon in lakes across eastern Canada: Evidence of a pronounced climate effect, Limnol. Oceanogr., 2010, 55, 30–42 CrossRef CAS.
- H. W. Paerl and J. Huisman, Climate change: a catalyst for global expansion of harmful cyanobacterial blooms, Environ. Microbiol. Rep., 2009, 1, 27–37 CrossRef CAS.
- H. W. Paerl and J. Huisman, Blooms like it hot, Science, 2008, 320, 57–58 CrossRef CAS.
- M. V. Fiorda Giordanino, S. M. Strauch, V. E. Villafañe and E. W. Helbling, Influence of temperature and UVR on photosynthesis and morphology of four species of cyanobacteria, J. Photochem. Photobiol., B, 2011, 103, 68–77 CrossRef.
- R. D. Calfee, E. E. Little, C. A. Pearl and R. L. Hoffman, Effects of simulated solar UVB radiation on early developmental stages of the northwestern salamander (Ambystoma gracile) from three lakes, J. Herpetol., 2010, 44, 572–580 CrossRef.
- H. U. Dahms and J. S. Lee, UV radiation in marine ectotherms: Molecular effects and responses, Aquat. Toxicol., 2010, 97, 3–14 CrossRef CAS.
- M. Rautio and B. Tartarotti, UV radiation and freshwater zooplankton: damage, protection and recovery, Freshwater Rev., 2010, 3, 105–131 Search PubMed.
- C. E. Williamson, J. M. Fischer, S. M. Bollens, E. P. Overholt and J. K. Breckenridge, Toward a more comprehensive theory of zooplankton diel vertical migration: Integrating ultraviolet radiation and water transparency into the biotic paradigm, Limnol. Oceanogr., 2011, 56, 1603–1623 CrossRef.
- S. Hylander and L.-A. Hansson, Vertical migration mitigates UV effects on zooplankton community composition, J. Plankton Res., 2010, 32, 971–980 CrossRef CAS.
- D. M. Martynova and A. V. Gordeeva, Light-dependent behavior of abundant zooplankton species in the White Sea, J. Plankton Res., 2010, 32, 441–456 CrossRef.
- P. Hortnagl, M. T. Perez, M. Zeder and R. Sommaruga, The bacterial community composition of the surface microlayer in a high mountain lake, FEMS Microbiol. Ecol., 2010, 73, 458–467 Search PubMed.
- R. D. Hernández, Moresino and E. W. Helbling, Combined effects of UVR and temperature on the survival of crab larvae (Zoea I) from Patagonia: The role of UV-absorbing compounds, Mar. Drugs, 2010, 8, 1681–1698 CrossRef.
- M. S. Souza, B. E. Modenutti, P. Carrillo, M. Villar-Argaiz, J. M. Medina-Sánchez, F. Bullejos and E. G. Balseiro, Stoichiometric dietary constraints influence the response of copepods to ultraviolet radiation-induced oxidative stress, Limnol. Oceanogr., 2010, 55, 1024–1032 CrossRef CAS.
- M. S. Souza, E. Balseiro, C. Laspoumaderes and B. Modenutti, Effect of ultraviolet radiation on acetylcholinesterase activity in freshwater copepods, Photochem. Photobiol., 2010, 86, 367–373 CrossRef CAS.
- C. E. Williamson, C. Salm, S. L. Cooke and J. E. Saros, How do UV radiation, temperature, and zooplankton influence the dynamics of alpine phytoplankton communities?, Hydrobiologia, 2010, 648, 73–81 CrossRef.
- K. N. Lister, M. D. Lamare and D. J. Burritt, Sea ice protects the embryos of the Antarctic sea urchin Sterechinus neumayeri from oxidative damage due to naturally enhanced levels of UV-B radiation, J. Exp. Biol., 2010, 213, 1967–1975 CrossRef CAS.
- M. Mangel, K. Richerson, K. A. Cresswell and J. R. Wiedenmann, Modelling the effects of UV radiation on the survival of Antarctic krill (Euphausia superba Dana) in the face of limited data, Ecol. Modell., 2010, 221, 2095–2101 CrossRef.
- A. Lenton, F. Codron, L. Bopp, N. Metzl, P. Cadule, A. Tagliabue and J. Le Sommer, Stratospheric ozone depletion reduces ocean carbon uptake and enhances ocean acidification, Geophys. Res. Lett., 2009, 36, L12606 CrossRef.
- J. M. Arblaster, G. A. Meehl and D. J. Karoly, Future climate change in the Southern Hemisphere: Competing effects of ozone and greenhouse gases, Geophys. Res. Lett., 2011, 38 CrossRef CAS.
- J. Austin, H. Struthers, J. Scinocca, D. A. Plummer, H. Akiyoshi, A. J. G. Baumgaertner, S. Bekki, G. E. Bodeker, P. Braesicke, C. Bruehl, N. Butchart, M. P. Chipperfield, D. Cugnet, M. Dameris, S. Dhomse, S. Frith, H. Garny, A. Gettelman, S. C. Hardiman, P. Joeckel, D. Kinnison, A. Kubin, J. F. Lamarque, U. Langematz, E. Mancini, M. Marchand, M. Michou, O. Morgenstern, T. Nakamura, J. E. Nielsen, G. Pitari, J. Pyle, E. Rozanov, T. G. Shepherd, K. Shibata, D. Smale, H. Teyssedre and Y. Yamashita, Chemistry-climate model simulations of spring Antarctic ozone, J. Geophys. Res. Atmos., 2010, 115, D00M11, DOI:10.1029/2009JD013577.
- L. M. Polvani, M. Previdi and C. Deser, Large cancellation, due to ozone recovery, of future Southern Hemisphere atmospheric circulation trends, Geophys. Res. Lett., 2011, 38, L04707 CrossRef.
- R. P. Allan, Human influence on rainfall, Nature, 2011, 470, 344–345 CrossRef CAS.
- S. K. Min, X. B. Zhang, F. W. Zwiers and G. C. Hegerl, Human contribution to more-intense precipitation extremes, Nature, 2011, 470, 378–381 CrossRef CAS.
- P. Pall, T. Aina, D. A. Stone, P. A. Stott, T. Nozawa, A. G. J. Hilberts, D. Lohmann and M. R. Allen, Anthropogenic greenhouse gas contribution to flood risk in England and Wales in autumn 2000, Nature, 2011, 470, 382–385 CrossRef CAS.
- M. L. Estapa and L. M. Mayer, Photooxidation of particulate organic matter, carbon/oxygen stoichiometry, and related photoreactions, Mar. Chem., 2010, 122, 138–147 CrossRef CAS.
- G. C. Shank and A. Evans, Distribution and photoreactivity of chromophoric dissolved organic matter in northern Gulf of Mexico shelf waters, Cont. Shelf Res., 2011, 31, 1128–1139 CrossRef.
- A. Stubbins, C. S. Law, G. Uher and R. C. Upstill-Goddard, Carbon monoxide apparent quantum yields and photoproduction in the Tyne estuary, Biogeosciences, 2011, 8, 703–713 CrossRef CAS.
- M. T. M. Tzortziou, P. J. Neale, J. P. Megonigal, C. L. Pow and M. Butterworth, Spatial gradients in dissolved carbon due to tidal marsh outwelling into a Chesapeake Bay estuary, Mar. Ecol.: Prog. Ser., 2011, 426, 41–56 CrossRef CAS.
- M. E. Gallo, A. Porras-Alfaro, K. J. Odenbach and R. L. Sinsabaugh, Photoacceleration of plant litter decomposition in an arid environment, Soil Biol. Biochem., 2009, 41, 1433–1441 CrossRef CAS.
- J. Frouz, T. Cajthaml and O. Mudrák, The effect of lignin photodegradation on decomposability of Calamagrostis epigeios grass litter, Biodegradation, 2011, 22, 1247–1254 CrossRef CAS.
- B. Foereid, J. Bellarby, W. Meier-Augenstein and H. Kemp, Does light exposure make plant litter more degradable?, Plant Soil, 2010, 333, 275–285 CrossRef CAS.
- E. J. D'Sa and S. F. DiMarco, Seasonal variability and controls on chromophoric dissolved organic matter in a large river-dominated coastal margin, Limnol. Oceanogr., 2009, 54, 2233–2242 CrossRef.
- D. G. Boyce, M. R. Lewis and B. Worm, Global phytoplankton decline over the past century, Nature, 2010, 466, 591–596 CrossRef CAS.
- W. G. Sunda and S. A. Huntsman, Interactive effects of light and temperature on iron limitation in a marine diatom: Implications for marine productivity and carbon cycling, Limnol. Oceanogr., 2011, 56, 1475–1488 CrossRef CAS.
- M. Jung, M. Reichstein, P. Ciais, S. I. Seneviratne, J. Sheffield, M. L. Goulden, G. Bonan, A. Cescatti, J. Q. Chen, R. de Jeu, A. J. Dolman, W. Eugster, D. Gerten, D. Gianelle, N. Gobron, J. Heinke, J. Kimball, B. E. Law, L. Montagnani, Q. Z. Mu, B. Mueller, K. Oleson, D. Papale, A. D. Richardson, O. Roupsard, S. Running, E. Tomelleri, N. Viovy, U. Weber, C. Williams, E. Wood, S. Zaehle and K. Zhang, Recent decline in the global land evapotranspiration trend due to limited moisture supply, Nature, 2010, 467, 951–954 CrossRef CAS.
- A. T. Austin, Has water limited our imagination for aridland biogeochemistry?, Trends Ecol. Evol., 2011, 26, 229–235 CrossRef.
- L. A. Brandt, J. Y. King, S. E. Hobbie, D. G. Milchunas and R. L. Sinsabaugh, The Role of Photodegradation in Surface Litter Decomposition Across a Grassland Ecosystem Precipitation Gradient, Ecosystems, 2010, 13, 765–781 CrossRef CAS.
- I. Dirks, Y. Navon, D. Kanas, R. Dumbur and J. M. Grunzweig, Atmospheric water vapor as driver of litter decomposition in Mediterranean shrubland and grassland during rainless seasons, Global Change Biol., 2010, 16, 2799–2812 CrossRef.
- X. J. Feng, K. M. Hills, A. J. Simpson, J. K. Whalen and M. J. Simpson, The role of biodegradation and photo-oxidation in the transformation of terrigenous organic matter, Org. Geochem., 2011, 42, 262–274 CrossRef CAS.
- S. Rutledge, D. I. Campbell, D. Baldocchi and L. A. Schipper, Photodegradation leads to increased carbon dioxide losses from terrestrial organic matter, Glob. Change Biol., 2010, 16, 3065–3074 Search PubMed.
- S. M. Uselman, K. A. Snyder, R. R. Blank and T. J. Jones, UVB exposure does not accelerate rates of litter decomposition in a semi-arid riparian ecosystem, Soil Biol. Biochem., 2011, 43, 1254–1265 CrossRef CAS.
- M. U. F. Kirschbaum, S. M. Lambie and H. Zhou, No UV enhancement of litter decomposition observed on dry samples under controlled laboratory conditions, Soil Biol. Biochem., 2011, 43, 1300–1307 CrossRef CAS.
- B. Foereid, M. J. Rivero, O. Primo and I. Ortiz, Modelling photodegradation in the global carbon cycle, Soil Biol. Biochem., 2011, 43, 1383–1386 CrossRef CAS.
- K.-S. Choi, S.-B. Oh, H.-R. Byun, R. H. Kripalani and D.-W. Kim, Possible linkage between East Asian summer drought and North Pacific Oscillation, Theor. Appl. Climatol., 2010, 103, 81–93 CrossRef.
- S. L. Lewis, P. M. Brando, O. L. Phillips, G. M. F. van der Heijden and D. Nepstad, The 2010 Amazon Drought, Science, 2011, 331, 554–554 CrossRef CAS.
- R. G. Zepp, D. J. Erickson III, N. D. Paul and B. Sulzberger, Effects of solar UV radiation and climate change on biogeochemical cycling: interactions and feedbacks, Photochem. Photobiol. Sci., 2011, 10, 261–279 CAS.
- S. T. Chen, Z. H. Hu, H. M. Li, Y. H. Ji and Y. P. Yang, Effects of elevated UV-B radiation on ecosystem and soil respiration in a winter wheat farmland, Eur. J. Soil Biol., 2011, 47, 16–23 CrossRef CAS.
- J. K. Haapala, S. K. Morsky, S. Saarnio, R. Rinnan, H. Suokanerva, E. Kyro, K. Latola, P. J. Martikanen, T. Holopainen and J. Silvola, Carbon dioxide balance of a fen ecosystem in northern Finland under elevated UV-B radiation, Global Change Biol., 2009, 15, 943–954 CrossRef.
- P. Faubert, P. Tiiva, A. Rinnan, J. Rasanen, J. K. Holopainen, T. Holopainen, E. Kyro and R. Rinnan, Non-methane biogenic volatile organic compound emissions from a subarctic peatland under enhanced UV-B radiation, Ecosystems, 2010, 13, 860–873 CrossRef CAS.
- A. E. Santoro, C. Buchwald, M. R. McIlvin and K. L. Casciotti, Isotopic Signature of N(2)O Produced by Marine Ammonia-Oxidizing Archaea, Science, 2011, 333, 1282–1285 CrossRef CAS.
- J. L. Pearce, J. Beringer, N. Nicholls, R. J. Hyndman and N. J. Tapper, Quantifying the influence of local meteorology on air quality using generalized additive models, Atmos. Environ., 2011, 45, 1328–1336 CrossRef CAS.
- S. Beaver and A. Palazoglu, Influence of synoptic and mesoscale meteorology on ozone pollution potential for San Joaquin Valley of California, Atmos. Environ., 2009, 43, 1779–1788 CrossRef CAS.
- A. Y. Karpechko, N. P. Gillett, L. J. Gray and M. Dall'Amico, Influence of ozone recovery and greenhouse gas increases on Southern Hemisphere circulation, J. Geophys. Res., 2010, 115, D22117 CrossRef.
- S. A. Montzka, M. Krol, E. Dlugokencky, B. Hall, P. Jockel and J. Lelieveld, Small interannual variability of global atmospheric hydroxyl, Science, 2011, 331, 67–69 CrossRef CAS.
- H. Su, Y. Cheng, R. Oswald, T. Behrendt, I. Trebs, F. X. Meixner, M. O. Andreae, P. Cheng, Y. Zhang and U. Pöschl, Soil nitrite as a source of atmospheric HONO and OH radicals, Science, 2011, 333, 1616–1618 CrossRef CAS.
- S. C. Anenberg, L. W. Horowitz, D. Q. Tong and J. J. West, An estimate of the global burden of anthropogenic ozone and fine particulate matter on premature human mortality using atmospheric modeling, Environ. Health Perspect., 2010, 118, 1189–1195 CrossRef CAS.
- R. L. Prueitt and R. L. Goodman, The global burden of ozone on respiratory mortality: No clear evidence for association, Environ. Health Perspect., 2011, 119, a158 CrossRef.
- S. C. Anenberg, J. J. West, L. W. Horowitz and D. Q. Tong, The global burden of air pollution on mortality: Anenberg et al. respond, Environ. Health Perspect., 2011, 119, a158–159 CrossRef.
- C. P. Leisner and E. A. Ainsworth, Quantifying the effects of ozone on plant reproductive growth and development, Global Change Biol., 2011 DOI:10.1111/j.1365-2486.2011.02535.x.
- S. Madronich, M. Wagner and P. Groth, Influence of tropospheric ozone control on exposure to ultraviolet radiation at the surface, Environ. Sci. Technol., 2011, 45, 6919–6923 CAS.
- R. Lutter and C. Woltz, UV-B screening by tropospheric ozone: Implications for the national ambient air quality standard, Environ. Sci. Technol., 1997, 31, 142–146 CrossRef.
- G. G. Palancar, R. E. Shetter, S. R. Hall, B. M. Toselli and S. Madronich, Ultraviolet actinic flux in clear and cloudy atmospheres: model calculations and aircraft-based measurements, Atmos. Chem. Phys., 2011, 11, 5457–5469 CrossRef CAS.
- M. J. Prather and J. Hsu, Coupling of nitrous oxide and methane by global atmospheric chemistry, Science, 2010, 330, 952–954 CrossRef CAS.
- A. Stohl, J. Kim, S. Li, S. O'Doherty, J. Muhle, P. K. Salameh, T. Saito, M. K. Vollmer, D. Wan, R. F. Weiss, B. Yao, Y. Yokouchi and L. X. Zhou, Hydrochlorofluorocarbon and hydrofluorocarbon emissions in East Asia determined by inverse modeling, Atmos. Chem. Phys., 2010, 10, 3545–3560 CrossRef CAS.
- X. Tang, S. R. Wilson, K. R. Solomon, M. Shao and S. Madronich, Changes in tropospheric composition and air quality due to stratospheric ozone depletion and interactions with changes in climate, Photochem. Photobiol. Sci., 2011, 10, 280–291 CAS.
- T. J. Wallington and M. D. Hurley, Atmospheric chemistry of hexafluorocyclobutene, octafluorocyclopentene, and hexafluoro-1,3-butadiene, Chem. Phys. Lett., 2011, 507, 19–23 CrossRef CAS.
- S. H. Kim, I. W. Hwang, Y. Jin, S. Song, J. Moon, H. Suh and K. Lee, Photodegradation of P3HT-A systematic study of environmental factors, Chem. Mater., 2011, 23, 145–154 CrossRef.
- O. Haillant, Accelerated weathering testing principles to estimate the service life of organic PV modules, Sol. Energy Mater. Sol. Cells, 2011, 95, 1284–1292 CrossRef CAS.
- H. Hintz, H. J. Egelhaaf, L. Luer, J. Hauch, H. Peisert and T. Chasse, Degradation and stabilization of poly(3-hexylthiophene) thin films for photovoltaic applications, Polym. Bull., 2011, 66, 211–222 CrossRef.
- E. Lopez-Elvira, E. Escasain, A. Baro, J. P. Colchero and E. Alacios-Lidon, Wavelength dependence of nanoscale photodegradation in poly(3-octylthiophene) thin films, Polym. Deg. Stab., 2011, 96, 1279–1285 CrossRef CAS.
- G. Giammarco, T. Stefano and L. Marinella, Degradation of semiconducting polymers by concentrated sunlight, Sol. Energy Mater. Sol. Cells, 2011, 95, 1308–1314 CrossRef.
- M. Rashvard, Z. Ranjbar and S. Rastegar, Nano-Zinc oxide as a UV-stabilizer for aromatic polyurethane coatings, Prog. Org. Coat., 2011, 70, 376–382 CrossRef.
- S. Cheng, Z. Peng, X. Wang and X. Cheng, The effect of nano ZnO on corona aging and photo aging in low-density polyethylene, Trans. Elec. Electron. Eng., 2011, 6, 7–13 CrossRef.
- C. C. Yern and Y. I. Idris, Weathering effect on PE coated with thin layer of PU/nanosilica composite, Adv. Mater. Sci. Technol., 2011, 181–182, 697–701 Search PubMed.
- V. C. Miela, R. Bernard and B. Pterre, Enhancing the performance of exterior waterborne coatings for wood by inorganic nanosized UV absorbers, Prog. Org. Coat., 2010, 69, 432–441 CrossRef.
- R. Yang, P. A. Christensen, T. A. Egerton and J. R. White, Degradation products formed during UV-exposure of polyethylene-ZnO nanocomposites, Polym. Deg. Stab., 2011, 95, 1533–1541 CrossRef.
- I. Grigoriadou, K. Paraskevopoulos, M. K. Chrissafis and E. Pavlidou, Effect of different nanoparticles on HDPE UV stability, Polym. Deg. Stab., 2011, 96, 151–163 CrossRef CAS.
- M. Morreale, N. T. Dintcheva and F. P. La Mantia, The role of filler type in the photo-oxidation behavior of micro-and nano-filled polypropylene, Polym. Int., 2011, 60, 1107–1116 CrossRef CAS.
- S. Sudeshna, K. Duygu, B. Yaman and P. Andre, Enhancing exterior durability of jack pine photo-stabilization of acrylic polyurethane coating using bark extract. Part 1: Effect of UV on color change and ATR-FTIR analysis, Prog. Org. Coat., 2011, 70, 376–382 CrossRef.
- L. M. Matuana, S. Jin and N. M. Stark, Ultraviolet weathering of HDPE/wood flour composites coextruded with a clear HDPE cap laver, Polym. Deg. Stab., 2011, 96, 97–106 CrossRef CAS.
- B. Lesar, M. Pavlic, M. S. Petric, A. s. Kapin and M. Humar, Wax treatment of wood slows photodegradation, Polym. Deg. Stab., 2011, 96, 1271–1278 CrossRef CAS.
- N. Pattamasattyasonthi, K. Chaochanchaikul, V. Rosarpitak and N. Sombatsompop, Effect of UV weathering and a CeO2 based coating layer on the mechanical and structural changes of wood/PVC composite, J. Vinyl Addit. Technol., 2011, 17, 9–16 CrossRef.
- P. Bijl, A. Heikkia, S. Syrjala, A. Aarva and A. Poikonen, Modelling of sample surface temperature
in an outdoor weathering test, Polym. Test., 2011, 30, 485–492 CrossRef CAS.
Footnotes |
† This article may be cited as: United Nations Environment Programme, Environmental Effects Assessment Panel, Environmental effects of ozone depletion and its interactions with climate change: progress report, 2011, Photochem. Photobiol. Sci., 2012, 11, DOI: 10.1039/c1pp90033a. List of contributing authors in alphabetical order: Anthony L. Andrady, Pieter J. Aucamp, Amy T. Austin, Alkiviadis F. Bais, Carlos L. Ballaré, Lars Olof Björn, Janet F. Bornman (Co-Chair), Martyn Caldwell, Anthony P. Cullen, David J. Erickson, Frank R. de Gruijl, Donat-P. Häder, Walter Helbling, Mohammad Ilyas, Janice Longstreth, Robyn Lucas, Richard L. McKenzie, Sasha Madronich, Mary Norval, Nigel D. Paul (Co-Chair), Halim Hamid Redhwi, Sharon Robinson, Min Shao, Keith R. Solomon (Secretary), Barbara Sulzberger, Yukio Takizawa, Xiaoyan Tang (Co-Chair), Ayako Torikai, Jan C. van der Leun, Craig E. Williamson, Stephen R. Wilson, Robert C. Worrest and Richard G. Zepp. |
‡ For ease of reading we will sometimes abbreviate the term “UV radiation” to “UV”. The term “UV irradiance” means the measured quantity of UV radiation (usually in units of W m−2). |
|
This journal is © The Royal Society of Chemistry and Owner Societies 2012 |
Click here to see how this site uses Cookies. View our privacy policy here.