Porous cross linked enzyme aggregates (p-CLEAs) of Saccharomyces cerevisiae invertase
Received
8th May 2012
, Accepted 8th June 2012
First published on 13th June 2012
Abstract
In this work, porous cross-linked enzyme aggregates (p-CLEAs) of Saccharomyces cerevisiae invertase are reported for the first time. Porous CLEAs were prepared by adding starch as a pore-making agent in crude invertase solution and co-precipitating with invertase using ammonium sulfate as a precipitating agent. The resultant co-precipitate was cross-linked for 8 h with 20 mM glutaraldehyde and in the end starch was removed by alpha amylase. The highest catalytic efficiency was obtained at 0.4% (w/v) starch. The scanning electron microscopy analysis of invertase p-CLEAs showed a porous structure. The p-CLEAs were shown to have improved internal mass transfer compared to conventional CLEAs.
Introduction
Recently, cross-linked enzyme aggregates (CLEAs) have emerged as a novel and versatile carrier-free immobilization strategy.1 In this strategy, enzyme proteins are firstly aggregated into supermolecular structures induced by appropriate precipitating agents, including inorganic salts,2 organic solvents,3–7 and some nonionic polymers.8,9 Subsequently, the formed aggregates are chemically bonded to each other by a bifunctional reagent, usually glutaraldehyde. Since precipitation from an aqueous medium is often used to purify enzymes, the CLEA methodology could be used for direct purification and immobilization of enzymes from a crude fermentation broth.10,11 The final preparation of CLEAs is able to yield pure protein with high specific enzyme activity and low production cost as no support is needed. CLEAs exhibit improved storage and operational stability towards denaturation by heat, organic solvents, autoproteolysis and are stable towards leaching in aqueous media.12 So far, CLEAs of various enzymes such as penicillin acylases, horseradish peroxidase, lipases, laccases, etc. have been prepared.13–16 However, an improvement in the production of CLEAs is needed since due to a cross-linking reaction the enzyme molecules are packed together in a small volume resulting in quite a small pore size. As a result, one might expect internal mass-transport limitations.17–20 Moreover, the washing period, centrifugation and filtration treatments might lead to an increased formation of clumps due to the low compression resistance of CLEAs,21 which would further hamper the internal mass transport of substrate and thereby reduce global activity. These result in some problems for the broad use of CLEAs.
To overcome this problem, a novel method called porous-CLEAs (p-CLEAs) has recently been developed to increase the porosity of CLEAs of papain.22 It involves the addition of starch as a pore making agent into an enzymatic solution to prepare a co-dissolved homogeneous mixture, co-precipitation of the enzyme and starch and then removal of the starch after glutaraldehyde cross-linking by alpha amylase, because alpha amylase can hydrolyze starch into maltose and glucose which can be washed away easily. Starch was selected as the pore-making agent because the large molecular size of starch is suitable to make large pores in CLEAs sufficient to improve the internal mass transport of the substrate. The present study explores the feasibility of the p-CLEAs method for improving the porosity of CLEAs of Saccharomyces cerevisiae invertase. The prepared p-CLEAs of Saccharomyces cerevisiae invertase were characterized in terms of diffusional limitations, enzyme kinetic parameters and surface morphology.
Experimental
Materials
Corn starch, sucrose and glutaraldehyde (25%) were obtained from Sigma (St. Louis, USA). DNSA (3,5-dinitrosalycyclic acid) was purchased from Merck (Darmstadt, Germany). Other reagents used were of analytical grade and obtained either from Sigma or Merck.
Invertase production
Invertase was obtained from Saccharomyces cerevisiae grown at 30 °C for 48 h in a shaking flask containing: Sucrose, 20 g l−1; Peptone, 5 g l−1; Yeast extract, 3 g l−1; pH 6. The crude invertase solution (5 mg protein/ml) isolated from the fermentation broth by centrifugation at 10
000 × g for 10 min was stored at 4 °C and used directly without further purification.
Assay of invertase
Invertase activity was determined using sucrose (1%, w/v) as a substrate. The reducing sugar produced was measured using 3,5-dinitrosalicylic acid.23 One unit (U) of enzyme activity is defined as the amount of enzyme required to produce 1 μmol of reducing sugar (estimated as glucose) per minute at 40 °C and pH 4.2.
Preparation of CLEAs of invertase
Choice of precipitating agents.
Chilled organic solvents (ethanol, n-propanol, t-butanol and acetonitrile, 5 ml each), saturated ammonium sulfate solution (5 ml) were added dropwise separately to samples of crude invertase solution (1 ml) with shaking and kept for 30 min at 4 °C for complete precipitation of the enzyme and then centrifuged for 10 min at 10
000 × g. The supernatant was discarded and the precipitate was redissolved in 0.1 M sodium acetate buffer, pH 4.2. The invertase activity was then checked.
Cross linking of enzyme precipitate
Saturated ammonium sulfate solution (5 ml) was added to crude invertase solution (1 ml) in capped centrifuge tubes. After keeping the mixture for 30 min at 4 °C for complete precipitation of enzyme, varying amounts of glutaraldehyde were added. The tubes were shaken continuously during this addition. The mixture was kept at 30 °C with varying cross linking times with constant shaking at 150 rpm. At the end of each cross linking reaction time, the suspension was centrifuged at 10
000 × g for 10 min. The supernatant was collected and checked for invertase activity. The pellets were washed three times with 0.1 M sodium acetate buffer, pH 4.2 to remove unreacted glutaraldehyde. The final enzyme preparation was kept in the same buffer (1 ml) at 4 °C. The activity recovery of invertase in CLEAs was determined using the following equation:
Preparation of porous CLEAs (p-CLEAs) of invertase
Porous CLEAs (p-CLEAs) of invertase were prepared by a general CLEA preparation procedure but starch was added as a pore making agent into the enzyme solution before precipitation.22 Saturated ammonium sulfate solution (5 ml) was added to the homogeneous mixture of 1 ml crude invertase solution and 0.3 ml gelatinized starch (0.4%, w/v) in capped centrifuge tubes. After keeping the mixture for 30 min at 4 °C for co-precipitation of the enzyme and starch, glutaraldehyde was added to give a final concentration of 20 mM. The tubes were shaken continuously during this addition. The mixture was kept at 30 °C for 8 h with constant shaking at 150 rpm. The supernatant was decanted and the pellets were washed three times with 0.1 M sodium acetate buffer, pH 4.2 to remove unreacted glutaraldehyde. To this final enzyme preparation, 1 mL of alpha amylase was added and the system was incubated at 37 °C. I2–KI indicator (contained 1% I2 and 2% KI) was added to the p-CLEAs sample so as to detect whether starch was completely hydrolyzed. When the color of p-CLEAs did not change into blue or reddish-brown, it indicated that starch was totally hydrolyzed.24 Then the insoluble p-CLEAs were washed with distilled water three times and finally resuspended in 0.1 M sodium acetate buffer, pH 4.2 and stored at 4 °C.
Effect of starch concentration on catalytic efficiency of p-CLEAs
Gelatinized starch (0.3 ml) prepared with different concentrations of corn starch (0.1–0.5%, w/v) was used during the preparation of p-CLEAs. The resulting p-CLEAs were used for inversion of sucrose at 40 °C, pH 4.2 and the amount of glucose produced within 10 min was determined using DNSA23 with the repeated experiment in the absence of p-CLEAs as the blank value. The catalytic efficiency of p-CLEAs for sucrose inversion was determined using the following equation:
SEM analysis
Surface morphology of the CLEAs and p-CLEAs was examined using an electron microscope. Scanning electron micrographs (SEMs) of CLEAs and p-CLEAs were obtained on a JEOL JSM-6360 (Germany) scanning electron microscope (SEM) operated at 5 kV. Samples were dried by rinsing with anhydrous acetone, placed on a sample holder, coated with platinum before being scanned under vacuum.
Determination of kinetic parameters
Kinetic parameters of free invertase, CLEAs and p-CLEAs of invertase were estimated by measuring initial reaction rates using different sucrose concentrations in the range of 2–440 mM at pH 4.2 and temperature 40 °C. Km, Vmax values of free invertase, CLEAs and p-CLEAs of invertase were calculated from nonlinear regression fitting of the initial reaction rates corresponding to different sucrose concentrations with Graph Pad Prism software.
Determination of diffusional limitations
Initial reaction rates were determined at 40 °C and pH 4.2 using different sucrose concentrations (2 mM, 5 mM, 25 mM, 300 mM, 600 mM and 800 mM) for free, CLEA and p-CLEA forms of invertase. Effectiveness factor (η) for each form of invertase was determined using the following equation25
where, Vimm and Vfree are the rates of the reaction catalyzed by the same enzyme concentrations with immobilized and free enzyme.
Results and discussion
Preparation of CLEAs of invertase
Choice of precipitating agents.
In order to capture enzyme activity in the ultimate product CLEA, it is necessary that all the enzyme activity is precipitated to a significant level. Several types of protein precipitants, including organic solvents such as ethanol, n-propanol, t-butanol and acetonitrile, inorganic salt like ammonium sulfate were investigated for evaluating their abilities of precipitating invertase. Fig. 1 shows that out of the five precipitants tried, ammonium sulfate worked best for precipitating all the invertase activity completely. Therefore, ammonium sulfate was selected as the best precipitating agent for preparing the invertase CLEAs in subsequent investigations.
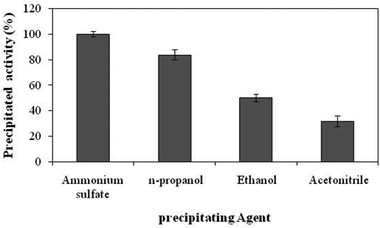 |
| Fig. 1 Precipitation of invertase with different precipitating agents. The experiments were done in triplicate and the error bar represents the percentage error in each set of readings. | |
Cross linking of the enzyme precipitate
The next step in the preparation of CLEAs is cross linking of the precipitated enzyme. Glutaraldehyde is a commonly used cross linking reagent. If too little cross-linker and cross linking time are used the enzyme molecules may still be too flexible while too much cross-linker and cross linking time can result in a loss of the minimum flexibility needed for the activity of the enzyme.26 So the concentration of cross linking agent and time of cross linking reaction must be optimized to recover maximum precipitated enzyme activity in CLEAs. As shown in Fig. 2, the activity recovery of invertase in CLEAs was found to increase with an increase in glutaraldehyde concentration and cross linking time. At glutaraldehyde concentrations of 20 mM and 8 h cross linking time the 100% activity of invertase was recovered in CLEAs. Invertase activity was detected in each supernatant collected after cross linking using glutaraldehyde concentration and cross linking time less than 20 mM and 8 h, respectively. This could be because of insufficient cross linking at lower glutaraldehyde concentrations and cross linking times.
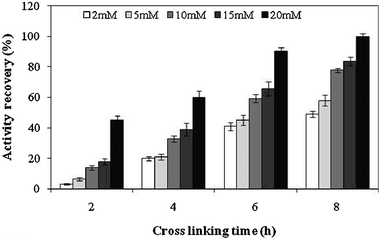 |
| Fig. 2 Effect of glutaraldehyde concentration and cross linking time on the activity recovery of invertase in CLEAs. The experiments were done in triplicate and the error bar represents the percentage error in each set of readings. | |
Effect of starch concentration on catalytic efficiency of p-CLEAs
Starch was selected as the pore-making agent during preparation of p-CLEAs. It occupies the inner spaces of co-precipitate which could form pores after removal of starch.22 Therefore; the porosity in CLEAs can be controlled by varying the starch concentration. The effect of concentration of starch on the pore formation in CLEAs is shown in Fig. 3. The catalytic efficiency of p-CLEAs of invertase was increased with increasing the concentration of starch and the highest catalytic efficiency was obtained at 0.4% (w/v) starch. Further increases in starch concentration to 0.5% did not increase the catalytic efficiency. So, 0.4% (w/v) starch was considered as the optimal starch concentration required for formation of maximum porosity in invertase CLEAs.
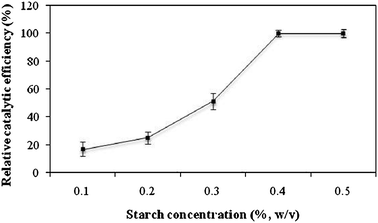 |
| Fig. 3 Effect of starch concentration on catalytic efficiency. The experiments were done in triplicate and the error bar represents the percentage error in each set of readings. | |
SEM analysis
The morphologies of CLEAs and p-CLEAs were characterized by SEM. Fig. 4a illustrated the structure of CLEAs which was prepared by the conventional method. The surface of CLEAs was quite smooth and non porous resulting in serious mass transfer limitations for substrates and limited the substrate to enter the inner CLEAs. On the contrary, an uneven and porous surface was found in p-CLEAs due to the role of starch as a pore-making agent as illustrated in Fig. 4b. Compared with conventional CLEAs, these pores not only decreased the mass transfer limitations but also increased the catalytic specific surface of CLEA particles.
 |
| Fig. 4 The SEM images of (a) CLEAs and (b) p-CLEAs. The starch concentration in preparing p-CLEAs was 0.4% (w/v). | |
Determination of kinetic parameters
Kinetic parameters of free, CLEA and p-CLEA forms of invertase were determined by measuring initial reaction rates for each form with varying amounts of starch. For free, CLEA and p-CLEA forms Michaelis–Menten kinetic behavior was observed. As shown in Table 1, for free and p-CLEA forms of invertase, Km and Vmax were about same. On the other hand, higher Km and lower Vmax were observed for the CLEA form as compared to that of free and p-CLEA forms of invertase. This could be possibly explained on the basis that the enzyme active sites in CLEA have an accessibility problem for substrate due to internal mass transfer constraints because of less porosity. This, of course, gets reflected in lowered Vmax/Km of CLEA forms of invertase.
Table 1 Kinetic parameters of free, CLEA and p-CLEA forms of invertase.
Form of invertase |
V
max (mM min−1) |
K
m (mM) |
V
max/Km (min−1) |
Free |
526 ± 2.6 |
10.80 ± 0.44 |
48.70 ± 1.74 |
CLEAs |
440 ± 2.1 |
17.50 ± 0.29 |
25.14 ± 0.30 |
p-CLEAs |
524 ± 2.8 |
11.00 ± 0.20 |
47.63 ± 0.61 |
Determination of diffusional limitations
For Michaelis–Menten enzyme kinetics, the extent of mass-transfer is usually expressed by effectiveness factor.25 The data in Table 2 show reduced effectiveness factors for CLEAs compared to free and p-CLEAs. This clearly reveals internal mass transfer limitations in CLEAs due to lesser porosity compared to p-CLEAs. At higher substrate concentrations, effectiveness factors for the CLEAs were improved. This could be due to increased substrate diffusion in CLEAs at high substrate concentrations.
Table 2 Effectiveness factor (η) for free, CLEA and p-CLEA forms of invertase
Form of invertase |
Concentration of sucrose |
2 mM |
5 mM |
25 mM |
300 mM |
600 mM |
800 mM |
Velocity (mM min−1) |
η
|
Velocity (mM min−1) |
η
|
Velocity (mM min−1) |
η
|
Velocity (mM min−1) |
η
|
Velocity (mM min−1) |
η
|
Velocity (mM min−1) |
η
|
Free |
0.72 ± 0.03 |
1 ± 0.04 |
2.8 ± 0.2 |
1 ± 0.07 |
18 ± 1.2 |
1 ± 0.06 |
227 ± 2.6 |
1 ± 0.01 |
526 ± 2.9 |
1 ± 0.005 |
526 ± 3.1 |
1 ± 0.005 |
CLEA |
0.11 ± 0.05 |
0.15 ± 0.06 |
0.90 ± 0.1 |
0.32 ± 0.02 |
10 ± 0.8 |
0.55 ± 0.01 |
178 ± 1.0 |
0.78 ± 0.01 |
439 ± 1.4 |
0.83 ± 0.002 |
440 ± 2.7 |
0.83 ± 0.006 |
p-CLEA |
0.30 ± 0.04 |
0.41 ± 0.04 |
2.0 ± 0.2 |
0.71 ± 0.03 |
16 ± 0.6 |
0.88 ± 0.02 |
222 ± 3.0 |
0.97 ± 0.01 |
523 ± 2.8 |
0.99 ± 0.004 |
523 ± 2.2 |
0.99 ± 0.002 |
Conclusion
The p-CLEAs of Saccharomyces cerevisiae invertase were obtained by co-precipitation with starch and then removal of the starch by alpha amylase. The morphology characterization results indicate that the p-CLEAs exhibit uneven and porous surfaces when compared with conventional CLEAs which resulted in improved internal mass transfer of sucrose into p-CLEAs. The convenient preparation process and the excellent porosity of p-CLEAs reported in this paper confirm the feasibility of recently developed methods of preparation of p-CLEAs and provide efficient solutions to the drawbacks of conventional CLEAs in biocatalysis.
Acknowledgements
We gratefully thank to Kolhapur Institute of Technology’s College of Engineering for financial support. Also, we wish to thank the Department of Physics, Shivaji University, Kolhapur, India for help with the SEM analysis.
Notes and references
- L. Q. Cao, F. van Rantwijk and R. A. Sheldon, Org. Lett., 2000, 2, 1361–1364 CrossRef CAS.
- B. S. Aytar and U. Bakir, Process Biochem., 2008, 43, 125–131 CrossRef CAS.
- L. F. Zhao, L. Y. Zheng, G. Gao, F. Ha and S. G. Cao, J. Mol. Catal. B: Enzym., 2008, 54, 7–12 CrossRef CAS.
- L. M. van Langen, R. P. Selassa, F. van Rantwijk and R. A. Sheldon, Org. Lett., 2005, 7, 327–329 CrossRef CAS.
- C. Roberge, D. Amos, D. Pollard and P. Devine, J. Mol. Catal. B: Enzym., 2009, 56, 41–45 CrossRef CAS.
- F. L. Cabirol, P. L. Tan, B. Tay, S. Cheng, U. Hanefeld and R. A. Sheldon, Adv. Synth. Catal., 2008, 350, 2329–2338 CrossRef CAS.
- L. Cao, L. M. van Langen, F. van Rantwijk and R. A. Sheldon, J. Mol. Catal. B: Enzym., 2001, 11, 665–670 CrossRef CAS.
- K. Sangeetha and T. E. Abraham, Int. J. Biol. Macromol., 2008, 43, 314–319 CrossRef CAS.
- B. K. Vaidya, S. S. Kuwar, S. B. Golegaonkar and S. N. Nene, J. Mol. Catal. B: Enzym., 2012, 74, 184–191 CrossRef CAS.
- C. Mateo, J. M. Palomo, L. M. van Langen, F. van Rantwijk and R. A. Sheldon, Biotechnol. Bioeng., 2004, 86, 273–276 CrossRef CAS.
- R. A. Sheldon, Adv. Synth. Catal., 2007, 349, 1289–1307 CrossRef CAS.
- R. A. Sheldon, Org. Process Res. Dev., 2011, 15, 213–223 CrossRef CAS.
- L. M. Van Langen, N. H. P. Oosthoek, F. van Rantwijk and R. A. Sheldon, Adv. Synth. Catal., 2003, 345, 797–801 CrossRef CAS.
- F. Šuleka, D. P. Fernández, Ž. Knez, M. Habulin and R. A. Sheldon, Process Biochem., 2011, 46, 765–769 CrossRef.
- F. Kartala, M. H. A. Janssenb, F. Hollmannc, R. A. Sheldon and A. Kilinc, J. Mol. Catal. B: Enzym., 2011, 71, 85–89 CrossRef.
- I. Matijos
te, I. W. C. E. Arends, S. de Vries and R. A. Sheldon, J. Mol. Catal. B: Enzym., 2010, 62, 142–148 CrossRef.
- R. Schoevaart, M. W. Wolbers, M. Golubovic, M. Ottens, A. P. G. Kieboom, F. van Rantwijk, L. A. M. van der Wielen and R. A. Sheldon, Biotechnol. Bioeng., 2004, 87, 754–762 CrossRef CAS.
- C. Garcia-Galan, A. Berenguer-Murcia, R. Fernandez-Lafuente and R. C. Rodrigues, Adv. Synth. Catal., 2011, 353, 2885–2904 CrossRef CAS.
- R. A. Sheldon, Appl. Microbiol. Biotechnol., 2011, 92, 467–477 CrossRef CAS.
- L. Cao, L. van Langen and R. A. Sheldon, Curr. Opin. Biotechnol., 2003, 14, 387–394 CrossRef CAS.
- S. Montoro-García, F. Gil-Ortiz, J. Navarro-Fernández, V. Rubio, F. García-Carmona and Á. Sánchez-Ferrer, Bioresour. Technol., 2010, 101, 331–336 CrossRef.
- M. Wang, C. Jia, W. Qi, Q. Yu, X. Peng, R. Su and Z. He, Bioresour. Technol., 2011, 102, 3541–3545 CrossRef CAS.
- G. L. Miller, Anal. Chem., 1959, 31, 426–428 CrossRef CAS.
- O. Kazuo, K. Hidemi and A. Takashi, Plant and Cell Physiology, 1980, 21, 201–204 Search PubMed.
- W. Tischer and V. Kasche, Trends Biotechnol., 1999, 17, 326–335 CrossRef CAS.
- R. A. Sheldon, I. Matijos
te, I. W. C. E. Arends and S. de Vries, J. Mol. Catal. B: Enzym., 2010, 62, 142–148 CrossRef.
|
This journal is © The Royal Society of Chemistry 2012 |
Click here to see how this site uses Cookies. View our privacy policy here.