DOI:
10.1039/C1SC00494H
(Edge Article)
Chem. Sci., 2011,
2, 2402-2406
Vapochromic and semiconducting solids of a bifunctional hydrocarbon†
Received
22nd July 2011
, Accepted 19th August 2011
First published on 15th September 2011
Abstract
This study explores a bifunctional hydrocarbon, which has two non-planar dibenzocycloheptatriene moieties linked by three cumulative C–C double bonds. This flexible conjugated molecule can adopt two conformations, namely, syn and anti, in its crystals. Of particular interest is that the molecule’s solids not only function as p-type semiconductors with field effect mobility of 0.02 cm2 V−1 S−1 but also exhibit vapochromic behavior with high selectivity. Upon selectively trapping specific volatile molecules, such as toluene and m-xylene, in the crystal lattice, the guest-free crystals of the syn conformer change to the clathrate crystals of the anti conformer accompanied by a change of color from red to orange. The unique combination of the two useful functions of this molecule can be attributed to its cycloheptatriene and cumulene moieties, both of which have rarely been used in building blocks for organic functional materials.
Introduction
To equip an organic material with multiple useful functions1 is much more challenging than to connect an organic molecule to multiple functional groups. Here we report a bifunctional hydrocarbon molecule (1 as shown in Fig. 1), which has two non-planar dibenzocycloheptatriene moieties linked by three cumulative C–C double bonds, leading to a bifunctional organic material that exhibits both vapochromic behavior and semiconductor properties in the solid state. Vapochromic materials, which show changes in color and/or emission in response to volatile organic compounds, have potential applications in chemical sensing. 2 A variety of metal complexes have exhibited vapochromic behavior in the solid state because adsorption and desorption of organic volatile molecules typically lead to significant changes in metal–metal interactions,3 or coordination modes.4 On the other hand, vapochromic behaviors in metal-free organic crystals have been rarely explored.5 Organic semiconductors are the key components of flexible, large-area, light-weight and low-cost organic electronics and have experienced tremendous development over the past decade.6 One advantage of organic semiconductors over their inorganic counterparts is that organic semiconductors in principle can be equipped with various functions through molecular design and organic synthesis. The study detailed below shows that highly selective vapochromism and electrical conductivity can be integrated with a flexible non-planar conjugated molecule.
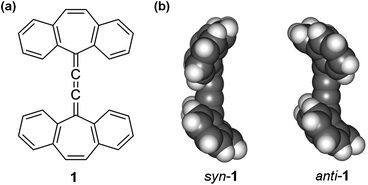 |
| Fig. 1 (a) Molecular structure of 1; (b) crystal structures of syn-1 and anti-1. | |
Results and discussion
Non-planar hydrocarbon 1 features cycloheptatriene and cumulene moieties, both of which have rarely been used as building blocks for organic functional materials.7 Because a cycloheptatriene ring, unlike a benzene ring, is non-planar and flexible, 1 can adopt two stable conformations, namely, syn and anti as found from the crystal structures (shown in Fig. 1b). The two conformers can be converted to each other by flipping the seven-membered rings. As found by calculation at the B3LYP level of density functional theory (DFT),8 the anti conformer is more stable than the syn conformer by 1.2 Kcal mol−1. Compound 1 was synthesized from 5-dibenzosubrenenone following reported procedures.9 It was found that 1 forms red crystals upon crystallization from ethyl acetate, chloroform or hexane, while it forms orange crystals upon slow crystallization from benzene, toluene, m-xylene or mesitylene. Interestingly, fast cooling of solutions of 1 in m-xylene yielded both orange and red crystals. As indicated by the 1H NMR spectra from completely dried crystals,10 the red crystals contain 1 only, while the orange crystals contain both 1 and solvent molecules in a ratio of 3
:
1. X-Ray crystallographic analysis of a red single crystal grown from ethyl acetate revealed that molecules of 1 adopt a syn conformation and form stacks. As indicated by the red arrows in Fig. 2a, in each stack of syn-1, two neighboring molecules have their dibenzocycloheptatriene moieties arranged in a face-to-face mode (the dihedral angle between two stacked benzene rings is 10.75°) with ten intermolecular carbon–carbon contacts within the range of 3.54 to 3.85 Å.11 In addition, edge-to-face interactions with two carbon–carbon contacts of 3.71 Å are found between two neighboring stacks as shown by the blue arrows in Fig. 2a. In contrast, X-ray crystallographic analysis of an orange single crystal grown from m-xylene revealed that 1 adopts an anti conformation and imprisons m-xylene molecules in its network, forming a clathrate of 3(anti-1)·m-xylene.12 As shown in Fig. 2b, each m-xylene (guest) molecule is surrounded by six molecules of anti-1 (host). Only four short carbon–carbon contacts within the range of 3.59 to 3.86 Å are found between two neighboring molecules of anti-1,13 and no face-to-face interactions are observed (shown in Fig. S-1 of ESI†). The orange clathrate crystals of anti-1 do not lose the guest molecules at room temperature under vacuum, but release guest molecules and convert to red crystalline powders of syn-1 when heated at 200 °C for 5 min. Formation of clathrates of anti-1 by trapping guest molecules in its crystal lattice suggests inefficient filling of space with molecules of anti-1 themselves.14
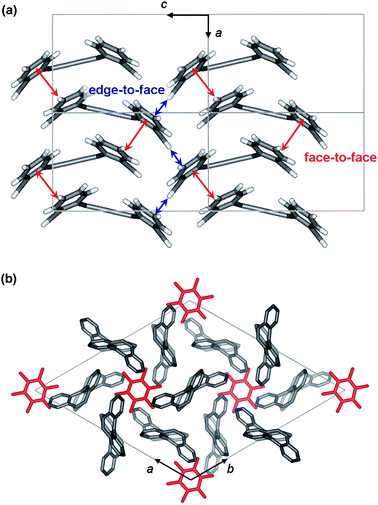 |
| Fig. 2 (a) Molecular packing of syn-1 in the red crystal as viewed along the b axis of the unit cell; (b) molecular packing of 3(anti-1)·m-xylene in the orange crystal as viewed along the c axis of the unit cell with m-xylene shown in red and anti-1 shown in grey (m-xylene molecules are disordered in orientation and all hydrogen atoms are removed for clarification). | |
To understand the different colors of syn-1 and anti-1 in the solid state, UV-vis absorption spectra of 1 were recorded from solids15 and from solution. As shown in Fig. 3, the yellow solution of 1 (a mixture of syn and anti conformers) in CH2Cl2 (5 × 10−5 mol L−1) has the longest wavelength absorption at 432 nm. The red color of syn-1 in the solid state is associated with the longest wavelength absorption at 474 nm, which shifts to the red by 42 nm relative to that measured from the solution of 1. In contrast, the orange color of the 3(anti-1)·m-xylene crystal is associated with the longest wavelength absorption at 454 nm. The large red shift of syn-1 can be attributed to electronic delocalization due to aromatic interactions16 with a large number of short carbon–carbon contacts as found in its crystal structure, while the smaller red shift of anti-1 is in agreement with the absence of face-to-face π-stacking in the crystal of 3(anti-1)·m-xylene. This conclusion is further confirmed by calculation of the frontier molecular orbitals, which excludes the possibility that the different absorptions of syn-1 and 3(anti-1)·m-xylene in the solid state are due to different degrees of π-conjugation in the individual molecules. The highest occupied molecular orbitals (HOMO) and lowest unoccupied molecular orbitals (LUMO) of syn-1 and anti-1 were calculated at the B3LYP level of density functional theory (DFT) with a 6-311G** basis set.17 The calculations indicate that both syn-1 and anti-1 have delocalized electron distributions in their HOMO and LUMO (shown in Fig. S-2 of the ESI†) with almost the same conjugation between the dibenzocycloheptatriene moiety and cumulene double bonds. The HOMO energy levels are calculated as −5.24 eV for syn-1 and −5.19 eV for anti-1; the LUMO energy levels are calculated as −2.12 eV for syn-1 and −2.16 eV for anti-1. The calculated HOMO–LUMO gap of syn-1 is slightly larger than that of anti-1 by 0.09 eV. On the other hand, the observed red color of syn-1 in the solid state is associated with an optical gap (2.62 eV) smaller than that of anti-1 (2.73 eV) in the solid state. Therefore the red-shifted absorption of syn-1 in the solid state should be attributed to the strong π–π interactions between molecules rather than the conformation of an individual molecule.
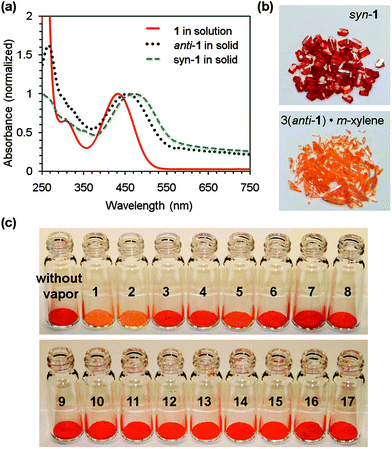 |
| Fig. 3 (a) UV-vis absorption spectra of 1 in CH2Cl2 (5 × 10−5 mol L−1), syn-1 and 3(anti-1)·m-xylene in the solid state; (b) red crystals of syn-1 and orange crystals of 3(anti-1)·m-xylene; (c) crystalline powders of syn-1 after exposure to varied organic vapors for 6 h (1: toluene, 2: m-xylene, 3: p-xylene, 4: o-bromotoluene, 5: bromobenzene, 6: o-dibromobenzene, 7: benzene: 8: mesitylene, 9: ethyl acetate, 10: hexane, 11: THF, 12: acetone, 13: ethanol, 14: chloroform, 15: acetic acid, 16: DMSO, 17: DMF). | |
The different colors of the guest-free crystal of syn-1 and the clathrate crystal of anti-1 suggest that the crystal of syn-1 may respond structurally to guest absorption18 with a change of its color upon exposure to certain organic vapors. To test the vapochromic behaviors, red crystalline powders of syn-1, which were prepared by grinding the crystals of 1 grown from ethyl acetate, were exposed to various organic vapors. As shown in Fig. 3c, the red crystalline powders of syn-1 turned orange after being exposed to saturated vapors of m-xylene for 6 h. The resulting orange powders were completely dried under vacuum and dissolved in CD2Cl2 before characterization by 1H NMR spectroscopy,19 which revealed that 1 and m-xylene existed in the resulting orange powders with a ratio of 3
:
1. Moreover, X-ray diffraction analysis of the resulting orange powders showed the same patterns (shown in Fig. 4) as the crystalline powders of 3(anti-1)·m-xylene, which were prepared by grinding the crystals of 1 grown from m-xylene. These observations indicate that the change of color accompanied a change from the guest-free syn-1 crystal to the clathrate crystal of 3(anti-1)·m-xylene. Upon heating at 200 °C for 5 min, the orange powders of 3(anti-1)·m-xylene returned to the red powders of syn-1 by losing m-xylene guest molecules and changing conformation, as indicated by the 1H NMR spectrum and powder X-ray diffraction pattern.
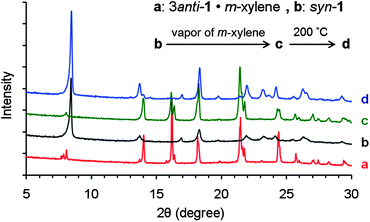 |
| Fig. 4
X-Ray diffraction patterns from powders of (a) 3(anti-1)·m-xylene; (b) syn-1; (c) b after exposure to saturated vapor of m-xylene for 6 h; (d) c after heating at 200 °C for 5 min. | |
The selectivity of vapochromic behavior was then tested by exposing the red crystalline powders of syn-1 to a variety of saturated organic vapors for 6 h. As shown in Fig. 3c, only the powders that were exposed to m-xylene and toluene turned orange, while those exposed to the other organic vapors did not exhibit an apparent color change. When the exposure time was prolonged to 5 days, the powders that were exposed to bromobenzene, o-bromotoluene and o-dibromobenzene also turned orange as shown in Fig. S-4 of the ESI†. In comparison, exposing powders of syn-1 to p-xylene, benzene and mesitylene for 5 days also led to a color change, but the change was less obvious. The 1H NMR spectra of the completely dried powders19 showed that p-xylene, benzene and mesitylene were trapped in the crystals, however, with the ratio of guest to 1 smaller than 1/3. X-Ray diffraction patterns from these powders showed a new set of peaks almost identical to those from 3(anti-1)·m-xylene besides the peaks for syn-1. These results indicate that the prolonged exposure of syn-1 solids to p-xylene, benzene and mesitylene led only to incomplete conversion to the corresponding clathrates. On the other hand, exposure for 5 days to the saturated vapors of non-aromatic organic solvents, including ethyl acetate, hexane, THF, acetone, ethanol, chloroform, acetic acid, DMSO and DMF, did not lead to a visible color change of the syn-1 crystalline powders. These volatile molecules were not trapped in the crystals of syn-1, as found from 1H NMR spectra of the completely dried red powders and the powder X-ray diffraction patterns.
The above results indicate that whether a volatile molecule can be trapped in the crystal lattice of anti-1 as a guest is determined by its shape and size. Although the crystal structure of 3(anti-1)·m-xylene, particularly the disordered arrangement of m-xylene molecules, suggests that benzene and substituted benzenes that are not larger than hexamethylbenzene can be included by anti-1 in the crystals, the observed highly selective vapochromism for toluene and m-xylene, particularly the discrimination between the m- and p-isomers of xylene, indicate that small structural differences may play an important role in the inclusion process. Another possible reason for the different time of response is the vapor pressure of the organic volatiles, and the slower vapochromic response of syn-1 to bromobenzene, o-bromotoluene and o-dibromobenzene may be related to their lower saturated vapor pressures.20 However, the slow vapochromic response to benzene, which has the highest saturated vapor pressure among all the aromatic guests tested in this study,20 suggests that vapor pressure does not play an important role in determining how efficiently a volatile guest molecule is trapped by the crystal lattice of anti-1. Because the red color of syn-1 in the solid state arises from the red shift of absorption due to strong π–π interactions, the mechanism involved in the selective vapochromism of 1 can be concluded as the guest-induced change in π–π interactions,21,22 which is accompanied by the conformational change of the host molecules. Conversion of the syn conformer to the more stable anti conformer is possibly a driving force for this vapochromic process.
The studies on molecular packing and electronic structure indicate that syn-1 self-assembles into stacks with intermolecular π-orbital overlap, and has a delocalized HOMO with an energy level accessible for charge injection.23 These findings suggest that syn-1 can function as a p-type organic semiconductor24 although both cumulene and cycloheptatriene have rarely been used as building blocks for organic semiconductors.25 Therefore the semiconductor properties of cumulene 1 were tested in an organic thin film transistor (OTFT) structure. Thin films of 1 were deposited by thermal evaporation onto silicon wafers, and the devices had vacuum-deposited gold as top-contact source and drain electrodes and highly doped Si as a gate electrode. A 300nm-thick layer of SiO2 that was pre-treated with a self-assembled monolayer of octadecyltrimethoxysilane (OTMS)26 functioned as dielectrics. The vacuum-deposited film of 1 exhibited a UV-vis absorption spectrum (shown in Fig. S-12 of the ESI†) almost identical to that of syn-1 in the solid state. The X-ray diffraction pattern of the film (shown in Fig. S-13 of the ESI†) exhibited one main peak at 2θ = 8.43° (d-spacing: 10.48 Å) with small peaks at 2θ = 16.91° (d-spacing: 5.24 Å) and 2θ = 25.45° (d-spacing: 3.50 Å). These three peaks exactly correspond to the (020), (040) and (060) diffractions derived from the single crystal structure of syn-1. These observations suggest that molecules of 1 adopt the syn conformation in the vacuum deposited film. It was found that 1 functioned as a p-type semiconductor with field effect mobility in the range of 0.01–0.03 cm2 V−1 S−1, as measured in the saturation regime from 96 channels of 18 devices in air. The average mobility measured from these channels was 0.022 cm2 V−1 S−1. Shown in Fig. 5 are the typical transfer I–V curves for the transistor of 1, from which a field-effect mobility of 0.03 cm2 V−1s−1 was measured using the equation: IDS = (μWCi/2L)(VGS − VT)2 and Ci of 11 nF cm−2 for 300 nm SiO2. The on/off ratio of the drain current obtained between 0 and −50 V gate bias is larger than 3 × 105. The successful application of 1 as an organic semiconductor in OTFTs suggests that cycloheptatriene and cumulene moieties can be used as useful building blocks for organic semiconductors. The unique combination of vapochromic behavior and semiconducting properties suggests that the OTFT device performance of 1 may respond to certain organic vapors. It was found that when the OTFTs of 1 were exposed to a saturated vapor of m-xylene for about 10 min, both the drain current (measured at VGS = −50 V and VGS = −50 V) and the field effect mobility decreased by one order of magnitude, as shown in Fig. S-15 of the ESI†. However, the mechanism involved in the change of drain current has not been understood, mainly because the vapor-induced changes of film crystallinity and morphology are complicated by another process known as vapor-annealing, which has been widely used for controlling the film morphology of organic semiconductors.27
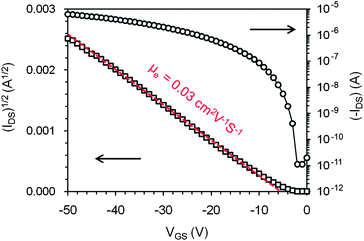 |
| Fig. 5 Drain current (IDS) versus gate voltage (VGS) with drain voltage (VDS) of −50 V for an OTFT of 1 with the active channel of W = 1 mm and L = 50 μm. | |
Conclusions
In summary, the above studies have revealed that the bifunctional hydrocarbon 1 exhibits both vapochromic behavior with high selectivity and p-type semiconductor properties in the solid state. Such a unique combination of two useful functions can be attributed to two building blocks that are rarely used in organic functional materials, particularly organic semiconductors. The embedded cycloheptatriene rings make 1 non-planar and flexible, allowing it to adopt anti and syn conformations in the solid state. The cumulative C–C double bonds link the two dibenzocycloheptatriene moieties with sufficient conjugation to form a delocalized HOMO of suitable energy level for a p-type organic semiconductor. The selective vapochromism of 1 involves a mechanism that specific guest molecules induce a change of π–π interactions accompanying a change from the syn conformer to the more stable anti conformer. As the first vapochromic organic semiconductor, 1 can in principle transduce a molecular inclusion event into electric signals. This suggests a new strategy to construct OTFT-based sensors28 for chemical vapors with unique semiconducting molecules that can trap analyte molecules within the crystal lattice.
Acknowledgements
We thank Ms Hoi Shan Chan (the Chinese University of Hong Kong) for the single crystal crystallography. This work was supported by grants from the Research Grants Council of Hong Kong (project number: GRF402011 and CUHK2/CRF/08).
Notes and references
- Hybrid solids consisting of the conducting layers of organic donor molecules and the magnetic layers of inorganic anions have been reported as bifunctional organic materials. For examples, see:
(a) M. Kurmoo, A. W. Graham, P. Day, S. J. Coles, M. B. Hursthouse, J. L. Caulfield, J. Singleton, F. L. Pratt, W. Hayes, L. Ducasse and P. Guionneau, J. Am. Chem. Soc., 1995, 117, 12209–12217 CrossRef CAS;
(b) E. Coronado, J. R. Galán-Mascarós, C. J. Gómez-García and V. N. Laukhin, Nature, 2000, 408, 447–449 CrossRef CAS;
(c) H. Fujiwara, H. Kobayashi, E. Fujiwara and A. Kobayashi, J. Am. Chem. Soc., 2002, 124, 6816–6817 CrossRef CAS;
(d) N. D. Kushch, E. B. Yagubskii, M. V. Kartsovnik, L. I. Buravov, A. D. Dubrovskii, A. N. Chekhlov and W. Biberacher, J. Am. Chem. Soc., 2008, 130, 7238–7240 CrossRef CAS.
- E. Takahashi, H. Takaya and T. Naota, Chem. Eur. J., 2010, 16, 4793–4802 CAS.
-
(a) C. L. Exstrom, J. R. Sowa, Jr., C. A. Daws, D. Janzen, K. R. Mann, G. A. Moore and F. F. Stewart, Chem. Mater., 1995, 7, 15–17 CrossRef CAS;
(b) C. E. Buss, C. E. Anderson, M. K. Pomije, C. M. Lutz, D. Britton and K. R. Mann, J. Am. Chem. Soc., 1998, 120, 7783–7790 CrossRef CAS;
(c) C. E. Buss and K. R. Mann, J. Am. Chem. Soc., 2002, 124, 1031–1039 CrossRef CAS;
(d) M. Kato, A. Omura, A. Toshikawa, S. Kishi and Y. Sugimoto, Angew. Chem. Int. Ed., 2002, 41, 3183–3185 CrossRef CAS;
(e) L. J. Grove, J. M. Rennekamp, H. Jude and W. B. Connick, J. Am. Chem. Soc., 2004, 126, 1594–1595 CrossRef CAS;
(f) T. J. Wadas, Q.-M. Wang, Y.-J. Kim, C. Flaschenreim, T. N. Blanton and R. Eisenberg, J. Am. Chem. Soc., 2004, 126, 16841–16849 CrossRef CAS;
(g) M. A. Mansour, W. B. Connick, R. J. Lachicotte, H. J. Gysling and R. Eisenberg, J. Am. Chem. Soc., 1998, 120, 1329–1330 CrossRef CAS;
(h) M. A. Rawashdeh-Omary, M. A. Omary, J. P. Fackler, Jr., R. Galassi, B. R. Pietroni and A. Burini, J. Am. Chem. Soc., 2001, 123, 9689–9691 CrossRef CAS;
(i) E. J. Fernández, J. M. López-de-Luzuriaga, M. Monge, M. E. Olmos, J. Pérez, A. Laguna, A. A. Mohamed and J. P. Fackler, Jr., J. Am. Chem. Soc., 2003, 125, 2022–2023 CrossRef;
(j) J. Lefebvre, R. J. Batchelor and D. B. Leznoff, J. Am. Chem. Soc., 2004, 126, 16117–16125 CrossRef CAS;
(k) C. E. Strasser and V. J. Catalano, J. Am. Chem. Soc., 2010, 132, 10009–10011 CrossRef CAS.
-
(a) L. G. Beauvais, M. P. Shores and J. R. Long, J. Am. Chem. Soc., 2000, 122, 2763–2772 CrossRef CAS;
(b) E. J. Fernández, J. M. López-de-Luzuriaga, M. Monge, M. E. Olmos, R. C. Puelles, A. Laguna, A. A. Mohamed and J. P. Fackler, Jr., Inorg. Chem., 2008, 47, 8069–8076 CrossRef.
- To the best of our knowledge, there are only two reports on vapochromic metal-free organic crystals. See:
(a)
ref. 2
;
(b) C. Dou, L. Han, S. Zhao, H. Zhang and Y. Wang, J. Phys. Chem. Lett., 2011, 2, 666–670 CrossRef CAS.
-
(a) S. R. Forrest, Nature, 2004, 428, 911–918 CrossRef CAS;
(b)
Organic Electronics: Materials, Manufacturing and Applications, ed. H. Klauk, WIley-VCH, Weinheim, 2006 Search PubMed;
(c)
Organic Photovoltaics: Materials, Device Physics and Manufacturing Techonologies, ed. C. Brabec, U. Scherf and V. Dyakonov, WIley-VCH, Weinheim, 2008 Search PubMed.
- For very limited examples of organic functional materials containing cycloheptatriene as building blocks, see:
(a) W. C. Chen, Y. W. Lee and C. T. Chen, Org. Lett., 2010, 12, 1472–1475 CrossRef CAS;
(b) Y. Wei, S. Samori, S. Tojo, M. Fujitsuka, J. S. Lin, C. T. Chen and T. Majima, J. Am. Chem. Soc., 2009, 131, 6698–6707 CrossRef CAS.
-
(a) A. D. Becke, J. Chem. Phys., 1993, 98, 5648–5652 CrossRef CAS;
(b) B. Miehlich, A. Savin, H. Stoll and H. Preuss, Chem. Phys. Lett., 1989, 157, 200–206 CrossRef CAS;
(c) C. Lee, W. Yang and G. Parr, Phys. Rev. B, 1988, 37, 785–789 CrossRef CAS.
- B. J. Dahl and N. S. Mills, J. Am. Chem. Soc., 2008, 130, 10179–10186 CrossRef CAS.
- The crystals were completely dried under vacuum at room temperature and dissolved in CD2Cl2 before characterization by 1H NMR spectroscopy.
- Intermolecular carbon–carbon contacts within the range of 3.5 to 3.92 Å were considered as short contacts between neighboring pentacene molecules in crystals, see: T. Siegrist, C. Kloc, J. H. Schön, B. Ballogg, R. C. Haddon, S. Berg and G. A. Thomas, Angew. Chem., Int. Ed., 2001, 40, 1732–1736 CrossRef CAS.
-
Clathrates are also known as lattice inclusion compounds. For reviews, see:
(a)
J. W. Steed and J. L. Atwood, Supramolecular Chemistry, WIley, Chichester, 2000, ch. 5 Search PubMed;
(b) R. Bishop, Chem. Soc. Rev., 1996, 25, 311–320 RSC;
(c) F. Toda, Pure Appl. Chem., 2001, 73, 1137–1145 CrossRef CAS.
- Three of the short contacts are between a benzene ring of one molecule and the central cumulene carbon atoms of its neighbor, and one short contact is between two nearest benzene rings.
-
M. J. Hardie, in Frontiers in Crystal Engineering, ed. E. R. T. Tiekink and J. J. Vittal, WIley, Chichester, 2006, pp. 135–155 Search PubMed.
- To measure UV-vis absorption spectra from the solids, a quartz plate was coated with fine crystalline powders of syn-1 and 3(anti-1)·m-xylene, which were grown from ethyl acetate and m-xylene respectively.
-
(a) U. Rohr, P. Schilichting, A. Böhm, M. Gross, K. Meerholz, C. Bräuchle and K. Müllen, Angew. Chem., Int. Ed., 1998, 37, 1434–1437 CrossRef CAS;
(b) Q. Miao, X. Chi, S. Xiao, R. Zeiss, M. Lefenfeld, C. Kloc, M. L. Steigerwald, T. Siegrist and C. Nuckolls, J. Am. Chem. Soc., 2006, 128, 1340–1345 CrossRef CAS;
(c) S.-H. Lim, T. G. Bjorklund, F. C. Spano and C. J. Bardeen, Phys. Rev. Lett., 2004, 92, 107402 CrossRef.
-
(a) G. A. Petersson and M. A. Al-Laham, J. Chem. Phys., 1991, 94, 6081–6090 CrossRef CAS;
(b) G. A. Petersson, A. Bennett, T. G. Tensfeldt, M. A. Al-Laham, W. A. Shirley and J. Mantzaris, J. Chem. Phys., 1988, 89, 2193–2218 CrossRef CAS.
- J. T. A. Jones, D. Holden, T. Mitra, T. Hasell, D. J. Adams, K. E. Jelfs, A. Trewin, D. J. Willock, G. M. Day, J. Bacsa, A. Steiner and A. I. Cooper, Angew. Chem. Int. Ed., 2011, 50, 749–753 CrossRef CAS.
- For 1H NMR spectra, see the ESI†.
- In order of decreasing value, the saturated vapor pressures of benzene, toluene, p-xylene, m-xylene, bromobenzene, mesitylene, o-bromotoluene and o-dibromobenzene at 25 °C are 100.92, 27.71, 7.94, 7.61, 4.12, 2.32, 1.2 and 0.13 mmHg respectively. (The values of saturated vapor pressures are cited from http://www.chemspider.com).
- Several metal complexes are also reported to (partially) owe their vapochromic behaviors to changes of π–π interactions in response to organic vapors. For examples, see:
(a) W. Lu, M. C. W. Chan, K.-K. Cheung and C.-M. Che, Organometallics, 2001, 20, 2477–2486 CrossRef CAS;
(b) Z. Liu, Z. Bian, J. Bian, Z. Li, D. Nie and C. Huang, Inorg. Chem., 2008, 47, 8025–8030 CrossRef CAS;
(c) A. Kobayashi, M.-a. Dosen, M. Chang, K. Nakajima, S.-i. Noro and M. Kato, J. Am. Chem. Soc., 2010, 132, 15286–15298 CrossRef CAS.
- The vapochromic metal-free organic crystals reported in ref. 2 involve guest-induced change of π-donor/acceptor interactions.
- The cyclic voltammogram (CV) of 1 (shown in the ESI†) in CH2Cl2 exhibits one pseudo-reversible oxidation wave with a half-wave oxidation potential vs.ferrocenium/ferrocene of 0.42 V, from which the HOMO energy level of 1 is estimated as −5.22 eV, in agreement with the calculated value.
- H. E. Katz, Z. Bao and S. L. Gilat, Acc. Chem. Res., 2001, 34, 359–369 CrossRef CAS.
- To the best of our knowledge, there are no reported examples for organic semiconductors containing cumulene moieties, and there is only one precedent for organic semiconductors containing cycloheptatriene subunits. See: ref. 7b.
- Y. Ito, A. A. Virkar, S. Mannsfeld, J. H. Oh, M. Toney, A. Locklin and Z. Bao, J. Am. Chem. Soc., 2009, 131, 9396–9404 CrossRef CAS.
-
(a) A. Amassian, V. A. Pozdin, R. Li, M. D-Smilgies and G. G. Malliaras, J. Mater. Chem., 2010, 20, 2623–2629 RSC;
(b) K. C. Dickey, J. E. Anthony and Y.-L. Loo, Adv. Mater, 2006, 18, 1721–1726 CrossRef CAS.
- For reviews on chemical sensors based on organic thin film transistors, see:
(a) M. E. Roberts, A. N. Sokolov and Z. Bao, J. Mater. Chem., 2009, 19, 3351–3363 RSC;
(b) T. Someya, A. Dodabalapur, J. Huang, K. C. See and H. Katz, Adv. Mater., 2010, 22, 3799–3811 CrossRef CAS;
(c) J. T. Mabeck and G. G. Malliaras, Anal. Bioanal. Chem., 2006, 384, 343–353 CrossRef CAS;
(d) J. Locklin and Z. Bao, Anal. Bioanal. Chem., 2006, 384, 336–342 CrossRef CAS;
(e) L. Wang, D. Fine, D. Sharma, L. Torsi and A. Dodabalapur, Anal. Bioanal. Chem., 2006, 384, 310–321 CrossRef CAS.
Footnote |
† Electronic supplementary information (ESI) available: Electronic structures of 1, fabrication and characterization of thin film transistors, experimental details for vapochromism of 1, and crystallographic information files for syn-1 (CCDC 823690) and 3(anti-1)·m-xylene (CCDC 823689). For ESI and crystallographic data in CIF or other electronic format see DOI: 10.1039/c1sc00494h |
|
This journal is © The Royal Society of Chemistry 2011 |
Click here to see how this site uses Cookies. View our privacy policy here.