DOI:
10.1039/C1FO10149H
(Paper)
Food Funct., 2011,
2, 684-696
Intestinal passage of microencapsulated fish oil in rats following oral administration
Received
20th July 2011
, Accepted 20th September 2011
First published on 17th October 2011
Abstract
Fish oil labelled with [14C-linolenin] tracer was orally administered by gavage as an oil–water mixture (free oil) or as an oil-in-water emulsion formulation (microencapsulated oil) to fasted rats. Groups of rats were then given food after gavage or alternatively not fed to examine the effect of food on intestinal passage. Feeding after gavage drives lumenal free oil faster through the gastrointestinal (GI) tract. Microencapsulation slows down the lumenal progression in the GI tract with feeding. Non-feeding also slows down the lumenal progress of free oil in the GI tract but this is not influenced by microencapsulation. Analysis of the relative distribution of the label along the GI tract tissue wall showed that the upper small intestine was the main site of label accumulation in the GI tract. Of the oil that remained in the lumen, there was slight protection against early uptake and metabolism in the stomach and upper GI tract in rats that were either fed or not fed after dosing when microencapsulated oil was administered. Microencapsulation increased the levels of radiolabel in the liver and blood. The primary organ for accumulation of radiolabel for both free and microencapsulated oils in rats that were fed or fasted after dosing was the liver.
Introduction
The bioavailability of ingested fat is dependent on their degree of hydrolysis, which is facilitated by bile acids and specific lipases from the stomach and duodenum, and then the absorption and transport of the triacylglycerol breakdown products into the enterocytes of the digestive system.1 Most fats ingested in foods are present as oil-in-water emulsions. Lipolysis is influenced by many factors, including the physical properties of the fat (e.g. the length of the fatty acid, its degree of unsaturation, melting point and the position of the fatty acid on the triglyceride), the size of the oil droplet, the nature of the interface around the oil droplet and the activity and level of lipases within the various parts of the GI tract.2–7 In healthy subjects, usually 98% of the ingested fats are absorbed.8
Further, the bioavailability of fats as oils depends upon whether they are presented as non-emulsified neat oils or in the presence of food, emulsified oils stabilized by different surface active components, or oils delivered in various food matrices.9–12 The food matrix containing the lipid, other food components, the presence of a meal in the GI tract and the format of delivery can also influence the availability of fats and lipophilic nutrients.9,13,14 This can occur because of changes mediated by the physical properties of the digesta. An example of physically mediated effects is the influence of viscous dietary fibres, which can decrease lipid digestion.4,15 The presence of food can also influence physiological changes that impact on fat digestion. For example, an increasing amount of fat in the diet leads to higher concentrations of gastric lipase.16 Gastric lipase digests fats in the stomach, producing monoacylglycerols and free fatty acids. These breakdown products of fat have surface-active properties and they improve the emulsification of fat, which leads to increased surface area for lipolysis. However, free fatty acids generated during lipolysis by gastric lipase can also inhibit subsequent lipolysis of triaclyglycerols by other mechanisms.3
After lipolysis, the products of fat digestion are absorbed by the enterocytes of the intestinal wall by passive diffusion, re-esterified and combined with apoproteins to form chylomicrons prior to re-circulation in the body.1,7,17 A detailed understanding of the specific mechanisms of lipid absorption is not fully understood, especially how different colloidal forms of lipid digestion products are absorbed by the enterocytes.18 Both GI tract physiology and fed/fasted states are known to affect the absorption of drugs. The difference in absorption in the presence of food has been related to altered secretion of gastric, bile and pancreatic fluids, modified gastric emptying rates and motility of the intestinal tract, and changed blood and lymph flow.19 These factors also affect the absorption of fat digestion products.7,17
The delivery of fish oil is of interest because it has many beneficial health effects, which have been attributed to its high level of long chain n-3 polyunsaturated fatty acids (n-3 PUFA).12,20–24 As the n-3 PUFAs in fish oils are very susceptible to oxidation, encapsulation of these oils within emulsion systems is a strategy that is used for their stabilization against the development of off-flavours and off-odours.25–28 Studies have shown that n-3 PUFA delivered as oil or encapsulated oil in food-grade materials are bioavailable as determined by assessment of plasma fatty acids in humans.29,30
The aim of the present study was to compare the oral delivery (by gavage) of free or microencapsulated fish oil into fasted rats, which were then allowed to feed or were not fed after dosing. This was to establish whether access to food after dosing affects the release of the oil. The effects of the fed and fasted states after dosing on the transit and digestion of oil, spiked with radiolabeled [14C]-trilinolenin, delivered as a free (unencapsulated) or microencapsulated oil, over a period of 14 h post administration was examined. The label recovered in the lumenal contents from different regions of the GI tract over time was measured as an indicator of the rate of passage of [14C]-trilinolenin and/or its metabolites while its bio-distribution into various rat tissues (GI tract tissues, blood and liver) were also determined. Free oil was delivered as a homogeneous mixture of oil in water (unencapsulated oil) while the microencapsulated oil was an emulsion formulation stabilised by a heated mixture of sodium caseinate, glucose and a modified resistant starch.
Materials and methods
Animals
Eighty male SPF (specific pathogen-free) Sprague-Dawley rats, approximately 8 weeks of age, were obtained from the Animal Resources Centre (ARC) Western Australia. Animals were housed under conventional Animal House conditions in the RDDT Animal Facility and were allowed to acclimatize for a minimum of 6 days. Rats were exposed to a 12
:
12 h light
:
dark cycle, in rooms maintained at 21 ± 2 °C under standard humidity conditions. Animals were fed Rat and Mouse Pellets® (Specialty Feeds, Glen Forrest, Western Australia) and provided waterad libitum. Periodic feed and water quality testing results indicate that there are no contaminants that could affect the study. During the acclimatization period the rats were housed in cages containing fresh bedding changed twice weekly. Rats were identified by permanent pen tail markings and housed 2–3 per cage. Rats were randomized into the different treatment groups prior to the start of dosing. All housing conditions and experimental procedures were conducted under the oversight of the animal ethics committees of CSIRO Food and Nutritional Sciences and RMIT in accordance with the Australian NH&MRC code of practice guidelines, which included power calculations in the experimental design.31
Materials
Fish oil (Hi-DHA 25N Food®) was from Nu-Mega Ingredients (Melbourne, Australia). The radioactive tracer [14C]18
:
3 (trilinolenin [linolenic-1-14C]; 0.1 mCi mL−1; Specific activity: 55 mCi mmol−1) was obtained from American Radiolabeled Chemicals, Inc. (St. Louis, MO, USA). Sodium caseinate (NaCas; Alanate 180®) was from New Zealand Milk Products, Fonterra, Melbourne, Victoria, Australia), high amylose maize starch (Hylon VII) from National Starch, Seven Hills, New South Wales, Australia and glucose from Penford Ltd., Lane Cove, NSW, Australia. For preparation of the modified resistant starch Hylon VII, a suspension of the starch in water was heated, sheared and microfluidised at 80 MPa for three passes using a Microfluidizer M210-EHB (MFIC, Newton MA, USA).32 Materials used for the preparation of samples for scintillation counting (BTS-450R tissue solubiliser, Ready OrganicR, Ready GelR and Ready ProteinR) were from Beckman, Sydney, NSW, Australia.
Preparation of oil formulations
For preparation of free oil (unencapsulated) fish oil preparation, 0.25 mL of the radiolabelled tracer was added to 4.56 g (4.96 mL) of fish oil. The final dose aliquots of fish oil (0.1 mL) plus the radiolabelled tracer (5 μL) were made up to 2 mL in water. A homogenous mixture was made by aspirating 5–10 times with a gavage needle to facilitate dispersion of oil into the water. Each rat received 0.1 mL of unlabelled fish oil and 0.5 μCi (5 μL) of the radiolabelled tracer.
The microencapsulated oil was an oil-in-water emulsion preparation stabilized by a heated mixture of sodium caseinate, glucose and a modified resistant starch. The encapsulant mixture was prepared by heating a mixture containing 78.5% water, 5.3% caseinate, 5.3% glucose and 5.3% modified resistant starch at 90 °C/30 min. The fish oil containing a radioactive tracer was added to the encapsulant mixture for preparation of 21.4% total solids emulsion containing 5.3% oil. Briefly, 0.25 mL of the radiolabelled tracer was added to 4.56 g (4.96 mL) of fish oil and mixed with the encapsulant mixture and made up to a final volume of 100 mL using a Silverson mixer, prior to 2-pass homogenization (50 + 10 MPa) using an Avestin EmulsFlex-C3 homogenizer (Avestin Inc, Ottawa, Ontario, Canada). Test samples of the emulsion containing the microencapsulated fish oil was administered in a volume of 2 mL to rats. This meant that each rat received 0.1 mL of unlabelled fish oil and 0.5 μCi (5 μL) of radiolabelled tracer.
In separate experiments, the radioactivity administered by aliquots of each formulation (unencapsulated and microencapsulated oil) was determined. This direct measurement of radioactivity of the total dose delivered into sample tubes confirmed that the dose of unencapsulated and microencapsulated oil administered was similar.
Particle sizing
On a separate occasion, an emulsion formulation of unlabelled fish oil in a heated mixture of sodium caseinate, glucose and a modified resistant starch was prepared in the same way for particle sizing. The emulsion sample was diluted and the particle size distribution was measured using a Malvern Masterziser 2000 (Malvern Instruments Ltd., Worcestershire, UK). The differential refractive index used was 1.092 (1.456 for oil and 1.333 for water). Duplicate measurements were carried out. The distribution was bimodal with a sub-population of particles having a diameter (D4,3, volume %) around 0.3–0.4 μm and a shoulder at 1 μm corresponding to the oil droplets and another sub-population around 9–10 μm of starch particles. The data for the particle sizing had similar features as that obtained previously for the formulation with the same ratio of the encapsulant (heated mixture of casein, modified resistant starch and glucose) and oil homogenized at 35 + 10MPa using a Rannie lab-scale homogeniser.33
Study design and rat treatment
A total of 80 rats, in groups of 20 were used for the 4 treatment groups over the experimental times (0, 4, 9 and 14 h) with n = 5 rats at each time point. All rats in all time point groups were dosed within a 2 h period. The treatments comprised two test samples, unencapsulated (free) fish oil and microencapsulated fish oil given by oral gavage in a volume of 2 mL. These were tested in animals that were either fed or not fed after dosing to establish whether access to food after dosing affects the time course and intestinal passage of the oil. All the rats in this study (i.e. both the groups of rats which were either i) fed or ii) not fed after dosing) were fasted for 24 h before dosing; but all animals were allowed free access to supplemented drinking water containing 2.5% glucose, 0.5% NaCl and 0.005% KCl (w/v). To maintain comparability, all rats in this study received supplemented water during the fasting period, after dosing, and during the test, including those that were fed. Rats that were fed were allowed free access to water and food throughout the test period until they were euthanized.
Tissue sampling and analysis
At 0, 4, 9 and 14 h following treatment, rats were euthanized by asphyxia with carbon dioxide. A blood sample was taken immediately by cardiac puncture, and liver samples were collected. GI tract lumenal contents were obtained by flushing each GI tract segment with saline and then freezing the washings for subsequent analysis of radioactivity. Lumenal washings were taken from the stomach, upper small intestine, lower small intestine, caecum and colon. The GI tract washings were counted to estimate the total amount of radioactivity. Faecal pellets found in the colon were removed and analysed separately and results added to the total radioactivity for colon (jointly referred to as colon lumen contents). This represents the total amount of unabsorbed oil and/or its metabolites.
Tissue samples collected for determination of radioactivity included the stomach, upper and lower small intestine, caecum, colon and liver. Blood and faeces were also collected for determination of radioactivity. The liver tissue, faeces and colon pellets and different GI tissues and lumenal contents were weighed and these samples were dissolved overnight in tissue solubiliser prior to scintillation counting.
Preparation of samples for analysis of radioactivity
All samples for analysis of radioactivity were prepared according to the Beckman Coulter sample preparation guide for biological tissues or sample preparation guide for biological fluids, as shown below. Each sample was subjected to liquid scintillation counting for determination of radioactivity. The calculation for total radioactivity in the blood was based on total blood volume being 5% in the whole animal. The procedures used have been described in detail previously.34
Tissue samples.
Samples (100 mg) and 0.5 mL BTS-450R tissue solubiliser were mixed and left overnight. Where necessary, 30% H2O2 solution was then titrated to decolorize the solubilised tissue. Glacial acetic acid (35 μL) was added to eliminate chemiluminescence and Ready OrganicR (5 mL) was added to each sample for liquid scintillation counting.
Blood.
Blood (0.15 mL) was mixed with 0.35 mL mixture of BTS-450/iso-propanol (1
:
2 v/v) and incubated for 1 h at 40 °C. A 30% solution of H2O2 (∼0.25 mL) was added to decolorize the mixture. The mixture was incubated for 15 min at room temperature and then for 30 min at 40 °C. Glacial acetic acid (35 μL) and Ready GelR (5 mL) was then added for liquid scintillation counting.
Faeces.
All faeces were rehydrated (0.05 mL water/10 mg faeces) and allowed to sit for 1 h prior to addition of 0.5 mL of BTS-450. The mixture was incubated 1–2 h at 40 °C. A 0.25 mL aliquot of iso-propanol was added and mixed prior to addition of 0.1 mL 30% hydrogen peroxide. This was allowed to stand for 10 min at room temperature and then incubated for 2 h at 40 °C. This mixture was diluted with 2.5 mL of deionized water. Glacial acetic acid (35 μL) and Ready Gel R (5 mL) was then added for liquid scintillation counting.
Washings obtained by flushing segments of the GI tract.
The flushings were aliquotted (0.1 mL) into 0.5 mL of 0.1 M NaOH and swirled until clear. ReadyProteinR (5 mL) solution was added to the mixture for scintillation counting. The levels of radioactivity in the samples were determined by liquid scintillation counting in a Packard 1500 Tri—Carb Scintillation Counter with computerized automatic external standardization for quench correction. The radioactivity in disintegrations per minute (dpm) was calculated as dpm per gram of sample. The total radioactivity in each sample obtained from GI tissues, liver, blood or faeces was calculated and expressed as a percentage of the total radioactivity administered.
The results were also expressed as follows:
% Total wall (Tissues) = % Small Intestine (SI) + % Caecum + % Colon = 100% |
This shows relative distribution of radioactivity in the GI tract tissue.
% Total lumen = 100% (whole of rat digestive system, including faeces) |
This indicates the relative distribution of the radioactivity, and not absolute amounts, to better show the transit of the bioactives.
The amount recovered as a percentage of the total dose administered was calculated as follows:
% Total dose = [dpm in sample/Total Dose given (in dpm) × 100%] |
This was calculated for lumenal washings (lumenal contents including colon pellets and faeces), GI tissues, liver and blood.
Statistical analyses
Statistical analyses were performed on data at each of the time points (4, 9 and 14 h) comparing: a) groups of rats that received free oil or microencapsulated oil that were fed after dosing, b) groups of rats that received free oil or microencapsulated oil that were not fed after dosing, c) the free oils groups fed after dosing or not fed after dosing and d) the microencapsulated groups fed or not fed after dosing. There were two outliers in the data obtained and these were not included in the statistical analysis. These outliers were the data for one rat from the group administered free oil analysed 4 h after dosing and for one rat from the group administered microencapsulated oil analysed 9 h after dosing. Comparisons were made by one-way ANOVA with Bonferroni post tests using GraphPad Prism version 4.00 for Windows (GraphPad Software, San Diego California USA, www.graphpad.com). The mean and standard error of the mean (SEM) calculations were performed using Microsoft Excel 2003 (Microsoft Corporation, Redmond, WA, USA, www.microsoft.com).
Results
The levels of the [14C-linolenin] radiolabeled tracer present in the GI tract, blood and liver at different times up to 14 h after gavage of either unencapsulated (free) or microencapsulated fish oil/tracer mix into rats that were subsequently either fed or fasted were measured and the impact of the various treatments on oil transit and bioavailability determined.
Relative distribution of radioactivity in the lumen
To highlight the change in relative distribution of fish oil in the lumenal contents within the different GI compartments of the rats over time, radioactivity in those compartments at the various time points (4, 9, and 14 h) was expressed as a percentage of the total lumen radioactivity at each time point. The profiles of lumenal radioactivity for rats in Fig. 1 depict the passage of the free oil and microencapsulated oil and/or their metabolites through the GI tract.
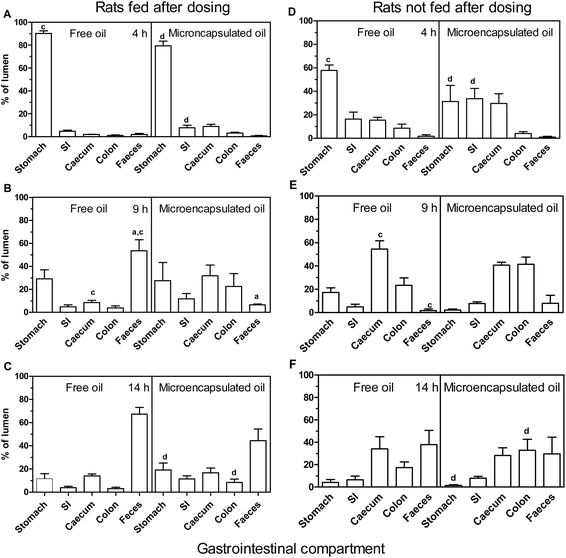 |
| Fig. 1 Time course of percentage of lumen radioactivity of gastrointestinal (GI) section washings to show the relative distribution of radioactivity and not absolute amounts. Rats were gavaged with unencapsulated (free) oil (left panel) or microencapsulated oil (right panel) and were either fed after dosing for 4 h (A), 9 h (B) or 14 h (C) or not fed after dosing at 4 h (D), 9 h (E) or 14 h (F). For simplicity, the small intestine data is the combination of upper and lower small intestinal (SI) washings while the colon is a combination of colon washings and colon contents/pellets. Data are expressed as mean ± SEM for n = 5 rats per group except for free oil group fed after dosing (4 h) microencapsulated oil group fed after dosing (9 h) where n = 4. Analyses between groups of free oil and microencapsulated oil and between fed and not fed treatments were by ANOVA with Bonferroni multiple comparison post tests. At equivalent time points and tissue sections, significant difference (P < 0.05) (1) between free oil and microencapsulated oil groups fed after dosing is indicated by a (2) between free oil and microencapsulated oil groups not fed after dosing by b (3) between free oil groups fed and not fed after dosing by c and (4) between microencapsulated groups fed and not fed after dosing by d. The absence of the alphabet superscript “b” in the diagram indicates that there were no significant differences for the specific comparison made. | |
Comparison between free oil and microencapsulated oil groups.
In rats that were fed after dosing, the patterns of distribution of radioactivity between GI compartments seen 4 h after dosing were, in the main, quite similar regardless of whether free or microencapsulated oil was used at gavage. Gavaging of free oil resulted in 90% of the radioactivity (expressed as % of lumen contents plus faeces) still remaining in the stomach and 2% in the faeces at 4 h (Fig. 1A). For the microencapsulated oil, the majority of the radioactivity was also in the stomach (79%) 4 h after treatment (Fig. 1A). At 9 h after dosing significantly more radioactivity appeared in the faecal compartment of rats gavaged with free oil (54%) relative to those receiving microencapsulated oil (6%) (P < 0.05) (Fig. 1B). Consistent with this, lower amounts of radioactivity were also retained in each of the other lumenal compartments [i.e. stomach (29%), small intestine (4.5%), caecum (9%) and colon (4%)] in the free oil group compared to those for rats dosed with microencapsulated oil [i.e. stomach (27%), small intestine (12%), caecum (32%) and colon (23%)]. However, these differences were not statistically significant (Fig. 1B). At 14 h after dosing there were also lower amounts of radioactivity in the lumenal contents of rats fed free oil (33%) [i.e. stomach (12%), small intestine (4%), caecum (14%) colon (3%)] compared to corresponding lumenal contents of rats fed microencapsulated oil (56%) [i.e stomach (19%), small intestine (11%), caecum (17%) and colon (8%)]. Once again, these differences in the lumenal contents between the free oil and microencapsulated groups were not statistically different (Fig. 1C). At 14 h, there was a higher amount of the radiolabel in the faeces of rats fed free oil (67%) compared to that in the faeces of rats fed microencapsulated oil (44%) but these differences were not statistically different (Fig. 1C). These results are consistent with a trend towards slower transit kinetics for oil or its metabolites delivered from microcapsules.
In rats not fed after dosing, there were no statistical differences between the radioactivity from free oil and microencapsulated oil and/or their metabolites in any of the compartments of the rat GI tract or the faeces at any of the time points tested (Fig. 1D, 1E and 1F). At 4 h, there was higher retention of radioactivity in the stomach contents (58%) of the group administered free oil compared to 31% for the microencapsulated oil. However, the converse was true for the contents of the small intestine and caecum. There were lower levels of radioactivity in the intestine (16%) and caecum (15%) for the free oil group compared to that in the intestine (34%) and caecum (30%) for the microencapsulated oil group (Fig. 1D). At 9 h, there was higher radioactivity in the caecum (54%) and colon (23%) than in the stomach (17%) for the free oil. Similarly there were also higher levels of the radiolabel in the caecum (41%) and colon (41%) than in the stomach (2%) for the microencapsulated oil (Fig. 1E). There were higher levels of radioactivity in the faeces (8%) of the group fed microencapsulated oil compared to free oil group where the level were non-detectable. At 14 h, there was more excretion of the radiolabel in the faeces where 38% of the label was found in the free oil group and 30% in the microencapsulated oil group (Fig. 1F). However, none of these apparent differences achieved statistical significance.
Comparison between rats fed and not fed after dosing.
The radioactivity profiles for rats that were fed or not fed after dosing displayed similar trends with some significant differences (P < 0.05) for corresponding oil groups (Fig. 1).
The rats not fed after dosing had lower levels of radioactivity in the stomach, expressed as a percentage of total lumen radioactivity plus faeces, and higher levels of radioactivity in the caecum at all time points compared to rats that were fed after dosing regardless of whether they had receive free or microencapsulated oil (compare Fig. 1A and 1D, 1B and 1E, 1C and 1F for corresponding oil groups). For the free oil group, there were significant differences (P < 0.05) between the rats fed and not fed after dosing in the radiolabel in the stomach at 4 h and in the caecum and faeces at 9 h. By 14 h, however, there were no significant differences in the radiolabel in the lumenal contents of corresponding GI compartments. The radioactivity was largely observed in faeces 9 and 14 h post-gavage in the free oil group that were fed after dosing (Fig. 1B and 1C) and only at 14 h in the rats that were not fed after dosing (Fig. 1F).
In the case of the rats fed and not fed after dosing there was significantly less radioactivity retained in the stomach and significantly more retained in the small intestine of rats that had received microencapsulated oil 4 h previously (P < 0.05). While no significant differences were seen in lumenal contents between fed and non-fed animals at the 9 h time point, by 14 h there was significantly less radioactivity in the stomach and significantly more in the colon of animals that had not been fed after dosing with microencapsulated oil (P < 0.05) (compare Fig. 1A and 1D, 1B and 1E, 1C and 1F for corresponding microencapsulated oil groups). In the free oil group the radioactivity was largely observed in the faeces at 9 and 14 h (Fig. 1B and 1C) in rats fed after dosing, and only at 14 h in the rats that were not fed after dosing (Fig. 1F). In the case of the microencapsulated oil group, the radiolabel in the faeces was largely found only after 14 h for both fed (Fig. 1C) and not fed rats after dosing (Fig. 1F).
Total recovery of radiolabel
The percentage of the administered dose of radioactivity recovered in all samples examined (i.e. lumen contents, faeces, GI tissues, liver and blood combined) after dosing with free or microencapsulated oil at various time points in both fed and not fed rats is shown in Fig. 2.
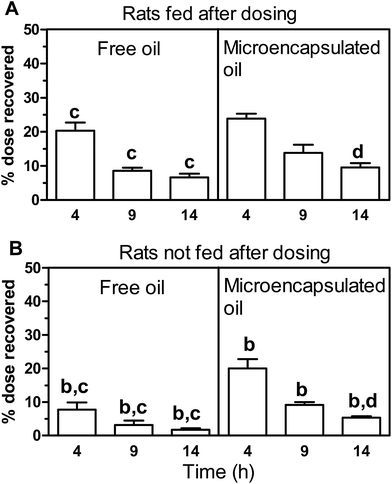 |
| Fig. 2 Percentage of administered dose of radioactivity recovered in all samples combined after dosing (gastrointestinal (GI) lumen contents, colon pellets and faeces, GI tissues, liver and blood). The data shows the time course of recovery of radioactivity from rats gavaged with free oil or microencapsulated oil, which were either fed after dosing at 4 h, 9 h or 14 h (A) or not fed after dosing for 4 h, 9 h or 14 h (B). Data are expressed as mean ± SEM for n = 5 rats per group except for free oil group fed after dosing (4 h) microencapsulated oil group fed after dosing (9 h) where n = 4. Analyses between groups of free oil and microencapsulated oil and between fed and not fed treatments were by ANOVA with Bonferroni multiple comparison post tests. At equivalent time points and tissue sections, significant difference (P < 0.05) (1) between free oil and microencapsulated oil groups fed after dosing is indicated by a (2) between free oil and microencapsulated oil groups not fed after dosing by b (3) between free oil groups fed and not fed after dosing by c and (4) between microencapsulated groups fed and not fed after dosing by d. The absence of the alphabet superscript “a” in the diagram indicates that there were no significant differences for the specific comparison made. | |
Comparison between free oil and microencapsulated oil groups.
In the rats fed after dosing, the recovery of radiolabel in the free oil group over 4–14 h was 20–7% while that in rats administered microencapsulated oil was higher (24–10%) (Fig. 2A). A similar trend was observed for rats that were not fed after dosing, where there was higher radioactivity recovered in the rats administered microencapsulated oil (20–5% over 4–14 h) compared to 8–2% over the same time period that for the free oil group (Fig. 2B).
At 9 h in the case of rats that were fed after dosing, the recovery of radiolabel was 9% of the administered dose for the free oil compared to 14% at this time point in rats administered microencapsulated oil (Fig. 2A). A similar trend was found for rats not fed after dosing, where the recovery of radiolabel was 9% of the administered dose for the microencapsulated oil compared to 3% recovered in rats administered free oil at 9 h after dosing (Fig. 2B).
These results are consistent with a trend for slight protection of the microencapsulated fish oil against early uptake and metabolism in the stomach and upper GI tract, and for slightly longer retention (at least for up to 9 h) in the rat when compared to the free oil (Fig. 2). Statistical analysis performed on total radiolabel recovered between free and microencapsulated oil results at all time points showed that there were only significant differences (P < 0.05) observed with free oil and microencapsulated oil in the groups that were not fed after dosing at all time points (Fig. 2B).
Comparison between rats fed and not fed after dosing.
In rats that were not fed after gavage with free oil, the recovery of the radiolabel over 4–14 h was 8–2% (Fig. 2B), which was low compared to rats that were fed (20–7%) (Fig. 2A). In rats administered free oil, there was significantly more (P < 0.05) radiolabel in the fed groups compared to the not fed groups at all time points (compare Fig. 2A and 2B). The recovery of the radiolabel in rats not fed after dosing with microencapsulated oil was 20–5% over 4–14 h (Fig. 2B), which was only slightly lower than when the rats were fed (24–10%) (Fig. 2A). For the microencapsulated groups, there was significantly more of the administered dose of radiolabel present at 14 h in the fed group than in the not fed group (Fig. 2A compared to 2B, P < 0.05).
Tissue radiolabel distribution
Fig. 3 shows the percentage of the administered dose of radioactivity recovered in different tissues after dosing with [14C] trilinolenin as free or microencapsulated oil. This includes GI tract tissues, liver and blood.
![Time course showing percentage of administered dose of radioactivity in tissues including gastrointestinal tract, liver and blood, after dosing with [14C] trilinolenin either as unencapsulated (free) oil (A) or microencapsulated oil (B) fed after dosing, or as free oil (C) or microencapsulated oil (D) not fed after gavaging indicated for 4 h, 9 h or 14 h. For simplicity, the small intestine (SI) is the combination of upper and lower small intestinal washings. Data are expressed as mean ± SEM for n = 5 rats per group except for free oil group fed after dosing (4 h) and microencapsulated oil group fed after dosing (9 h) where n = 4. Analyses between groups of free oil and microencapsulated oil and between fed and not fed treatments were by ANOVA with Bonferroni multiple comparison post tests. At equivalent time points and tissue sections, significant difference (P < 0.05) (1) between free oil and microencapsulated oil groups fed after dosing is indicated by a (2) between free oil and microencapsulated oil groups not fed after dosing by b (3) between free oil groups fed and not fed after dosing by c and (4) between microencapsulated groups fed and not fed after dosing by d.](/image/article/2011/FO/c1fo10149h/c1fo10149h-f3.gif) |
| Fig. 3 Time course showing percentage of administered dose of radioactivity in tissues including gastrointestinal tract, liver and blood, after dosing with [14C] trilinolenin either as unencapsulated (free) oil (A) or microencapsulated oil (B) fed after dosing, or as free oil (C) or microencapsulated oil (D) not fed after gavaging indicated for 4 h, 9 h or 14 h. For simplicity, the small intestine (SI) is the combination of upper and lower small intestinal washings. Data are expressed as mean ± SEM for n = 5 rats per group except for free oil group fed after dosing (4 h) and microencapsulated oil group fed after dosing (9 h) where n = 4. Analyses between groups of free oil and microencapsulated oil and between fed and not fed treatments were by ANOVA with Bonferroni multiple comparison post tests. At equivalent time points and tissue sections, significant difference (P < 0.05) (1) between free oil and microencapsulated oil groups fed after dosing is indicated by a (2) between free oil and microencapsulated oil groups not fed after dosing by b (3) between free oil groups fed and not fed after dosing by c and (4) between microencapsulated groups fed and not fed after dosing by d. | |
Comparison between free oil and microencapsulated oil groups.
For animals gavaged with microencapsulated oil, the percentage of radioactive dose recovered in the blood at all time points, the liver and caecum at 4 h and the small intestine at 9 h were all significantly higher (P < 0.05) than for rats that received the free oil where animals were fed after dosing (compare Fig. 3A and 3B). In the groups not fed after dosing, significantly higher (P < 0.05) radioactivity was found in the small intestine tissue and the blood at all time points, in the colon at 4 h and 9 h and in the liver at 4 h after administration of microencapsulated oil when compared to those receiving free oil (compare Fig. 3C and 3D). The accumulation of the radiolabel for both the free and microencapsulated oils and/or their metabolites was highest in the liver. Only minor amounts were found in the GI tract tissues and blood regardless of whether oil was administered in free or microencapsulated form, but in all cases by 14 h, the levels were minimal (Fig. 3). This is in line with the literature indicating almost complete conversion of fats to CO2 is achieved by14 h.35
Comparison between rats fed and not fed after dosing.
In both groups of rats administered free oil, both fed and not fed after dosing, the percentages of the dose of radioactivity recovered in the GI tract tissues and blood were similar with only small differences between the groups at any of the time points, with the difference only reaching significance (P < 0.05) in the blood (compare Fig. 3A and 3C). It was noted that in rats administered free oil, regardless of feeding status post-gavage, the percentage of dose of radioactivity recovered from the small intestine tissue was higher than from the other sections of the GI tract (Fig. 3). The highest recovery in both groups of rats (fed and non-fed) administered free oil was from the liver with up to 5% of the dose recovered in this tissue. More label was recovered from the livers of rats that were fed after dosing with significant differences achieved at the 9 and 14 h time points (compare Fig. 3A and 3C, P < 0.05).
Similarly in the two groups administered microencapsulated oil (fed and not fed post gavage) the percentages of the administered radioactivity dose recovered from the GI tract walls were similar, with only small differences between the groups at any given time point. As in the free oil group, this percentage was slightly higher for the small intestine tissue in rats administered microencapsulated oil, irrespective of post-gavage feeding status and only a significantly higher (P < 0.05) level in the colon of microencapsulated oil group that was not fed after dosing (Fig. 3B and 3D). There were significant differences (P < 0.05) in the radiolabel in the liver and blood at 9 and14 h. Again, independent of feeding regimen post-gavage with microencapsulated oil, the tissue containing the highest level of radiolabel was the liver where the level was 3–9% (Fig. 3B and 3D). The elevated liver accumulation observed with both free and microencapsulated fish oil is consistent with the known pathway for fatty acid metabolism.36
Absolute amounts of radioactivity in the lumen (as % of dose given)
The amounts of radioactivity immediately after gavage and during transit were examined.
Immediately after gavage.
Immediately after gavage some rats were sacrificed to confirm delivery of free or microencapsulated fish oil to the stomach. The percentage of the administered dose of radioactivity recovered in the stomach immediately after gavaging at t = 0 h was about 20% and 9% for free fish oil in groups of five rats that were either fed or not fed after dosing, respectively. The percent recovery at t = 0 h was about 77% for microencapsulated fish oil in both groups of five rats, i.e. rats fed and rats not fed after dosing. This suggests that where free oil was administered, significant quantities of oil could have remained attached to the stomach wall in a fashion that resisted dislodgement by the washing protocol used. Whilst the introduction of food after dosing did not affect the recovery in the case of the microencapsulated oil, the denial of food after fasting resulted in higher amounts of radioactivity being recovered when free oil was gavaged. The amount of the dose remaining associated with the stomach tissue at t = 0 h was not measured in this study.
During 4–14 h transit.
Lumen contents at various time points (4, 9 and 14 h) were expressed as a percentage of the dose of radioactivity given to indicate relative abundance between the treatment groups. Apart from the finding that in all rats the percentage of the dose recovered in the stomach at the 4 h time point for both free oil and microencapsulated oil groups was about 2–10% and 3% for that in the stomach at the 9 h time point for rats not fed after dosing in the microencapsulated group, other levels of radioactivity detected in the lumen of the GI tract represented 1% or less of the administered dose after gavage (Fig. 4). These results suggest that in all groups, even by 4 h, there may be significant uptake and metabolism to CO2 and this is confirmed by the low value of the percentage dose recovered as already noted in Fig. 2. However, there were some significant differences in the passage of the label for the small amount that remained in the lumen.
![Time course showing percentage of administered dose of radioactivity recovered in the lumen of gastrointestinal tract sections after dosing with [14C] trilinolenin either as unencapsulated (free) oil (A) or microencapsulated oil (B) fed after dosing or as free oil (C) or microencapsulated oil (D) not fed after gavaging for 4 h, 9 h or 14 h as indicated. For simplicity, the small intestine (SI) is the combination of upper and lower small intestinal washings while the colon is a combination of colon washings and colon contents/pellets and faeces. Data are expressed as mean ± SEM for n = 5 rats per group except for free oil group fed after dosing (4 h) and microencapsulated oil group fed after dosing (9 h) where n = 4. Analyses between groups of free oil and microencapsulated oil and between fed and not fed treatments were by ANOVA with Bonferroni multiple comparison post tests. At equivalent time points and tissue sections, significant difference (P < 0.05) (1) between free oil and microencapsulated oil groups fed after dosing is indicated by a (2) between free oil and microencapsulated oil groups not fed after dosing by b (3) between free oil groups fed and not fed after dosing by c and (4) between microencapsulated groups fed and not fed after dosing by d. The absence of the alphabet superscripts “a” and “d” in the diagram that there were no significant differences for the specific comparisons made.](/image/article/2011/FO/c1fo10149h/c1fo10149h-f4.gif) |
| Fig. 4 Time course showing percentage of administered dose of radioactivity recovered in the lumen of gastrointestinal tract sections after dosing with [14C] trilinolenin either as unencapsulated (free) oil (A) or microencapsulated oil (B) fed after dosing or as free oil (C) or microencapsulated oil (D) not fed after gavaging for 4 h, 9 h or 14 h as indicated. For simplicity, the small intestine (SI) is the combination of upper and lower small intestinal washings while the colon is a combination of colon washings and colon contents/pellets and faeces. Data are expressed as mean ± SEM for n = 5 rats per group except for free oil group fed after dosing (4 h) and microencapsulated oil group fed after dosing (9 h) where n = 4. Analyses between groups of free oil and microencapsulated oil and between fed and not fed treatments were by ANOVA with Bonferroni multiple comparison post tests. At equivalent time points and tissue sections, significant difference (P < 0.05) (1) between free oil and microencapsulated oil groups fed after dosing is indicated by a (2) between free oil and microencapsulated oil groups not fed after dosing by b (3) between free oil groups fed and not fed after dosing by c and (4) between microencapsulated groups fed and not fed after dosing by d. The absence of the alphabet superscripts “a” and “d” in the diagram that there were no significant differences for the specific comparisons made. | |
Comparison between free oil and microencapsulated oil groups.
There were no significant differences between free oil and microencapsulated oil groups (P > 0.05) in rats fed after dosing (compare Fig. 4A and 4B). In the rats that were not fed after dosing, significantly higher levels (P < 0.05) were found both in the caecum and colon for the microencapsulated oil group at 9 h (compare Fig. 4C and 4D). Although there was a small amount of radioactive label recovered in the lumen in absolute terms, the data nevertheless suggest that microencapsulation did offer some protection against early metabolism and uptake of the oil in rats that were not fed after dosing.
Comparison between rats fed and not fed after dosing.
For rats fed free oil, significantly higher levels (P < 0.05) of the administered dose were found only in the stomach at 4 h and in the colon at 9 h in rats that were fed after dosing. In contrast, in the microencapsulated oil group, there were no significant differences (P > 0.05) in rats that were not fed compared to rats fed after dosing.
Administered dose of radioactivity recovered as dpm per g of tissue
The level of radioactivity (as dpm per g tissue) after dosing with microencapsulated oil or free oil for 4, 9 and 14 h detected in tissues, liver or blood is shown in Fig. 5.
![Time course showing recovery of administered dose of radioactivity in the gastrointestinal tract including liver and blood expressed as dpm per g tissue after dosing with [14C] trilinolenin either as unencapsulated (free) oil (A) or microencapsulated oil (B) fed after gavage or free oil (C) or microencapsulated oil (B) not fed after gavaging. Times as indicated are 4 h, 9 h or 14 h. For simplicity, the small intestine is the combination of upper and lower small intestinal (SI) tissue. Data are expressed as mean ± SEM for n = 5 rats per group except for free oil group fed after dosing (4 h) microencapsulated oil group fed after dosing (9 h) where n = 4. Analyses between groups of free oil and microencapsulated oil and between fed and not fed treatments were by ANOVA with Bonferroni multiple comparison post tests. At equivalent time points and tissue sections, significant difference (P < 0.05) (1) between free oil and microencapsulated oil groups fed after dosing is indicated by a (2) between free oil and microencapsulated oil groups not fed after dosing by b (3) between free oil groups fed and not fed after dosing by c and (4) between microencapsulated groups fed and not fed after dosing by d. The absence of the alphabet superscript “a” in the diagram that there were no significant differences for the specific comparison made.](/image/article/2011/FO/c1fo10149h/c1fo10149h-f5.gif) |
| Fig. 5 Time course showing recovery of administered dose of radioactivity in the gastrointestinal tract including liver and blood expressed as dpm per g tissue after dosing with [14C] trilinolenin either as unencapsulated (free) oil (A) or microencapsulated oil (B) fed after gavage or free oil (C) or microencapsulated oil (B) not fed after gavaging. Times as indicated are 4 h, 9 h or 14 h. For simplicity, the small intestine is the combination of upper and lower small intestinal (SI) tissue. Data are expressed as mean ± SEM for n = 5 rats per group except for free oil group fed after dosing (4 h) microencapsulated oil group fed after dosing (9 h) where n = 4. Analyses between groups of free oil and microencapsulated oil and between fed and not fed treatments were by ANOVA with Bonferroni multiple comparison post tests. At equivalent time points and tissue sections, significant difference (P < 0.05) (1) between free oil and microencapsulated oil groups fed after dosing is indicated by a (2) between free oil and microencapsulated oil groups not fed after dosing by b (3) between free oil groups fed and not fed after dosing by c and (4) between microencapsulated groups fed and not fed after dosing by d. The absence of the alphabet superscript “a” in the diagram that there were no significant differences for the specific comparison made. | |
Comparison between free oil and microencapsulated oil groups.
In all groups of rats fed after dosing with free or microencapsulated oil, highest tissue levels of radioactivity detected were mainly in the small intestine as well as in the liver at all time points. With the exception of the stomach at 4 h in rats that were fed after dosing with free oil, lower levels occurred in the stomach with even lower levels in the remaining GI tract tissues (i.e. caecum and colon) and blood (Fig. 5A and 5B). However, at equivalent time points, there were no significant differences between the free oil and microencapsulated oil groups that were fed after dosing.
In the rats that were not fed after dosing with either free or microencapsulated oil, higher tissue levels of radioactivity were mainly in the liver, stomach, and small intestine compared to other tissues (Fig. 5C and 5D). In all other GI tract tissues (i.e. caecum and colon) and blood, the levels of radioactivity were low (about 1000 dpm g−1 or less). There was significantly higher recovery (P < 0.05) observed at 4, 9 and 14 h in the small intestine, caecum and blood, at 4 and 14 h for the colon and at 4 h and 9 h for the liver in rats given microencapsulated oil compared to rats administered free oil in the not fed groups (compare Fig. 5C and 5D). This is confirmed by the high value of the percentage dose recovered in the liver as already noted in Fig. 3. In all of the above groups, there was less radiolabel retained in tissues at 14 h.
Comparison between rats fed and not fed after dosing.
Although there are some differences in the levels of radioactivity recovered in tissues and blood, these only reached significance at some time points. For rats fed free oil, the significant differences (P < 0.05) were obtained for levels in the liver at 9 and 14 h and in the blood at 14 h where rats fed after dosing had higher levels of radiolabel (compare Fig. 5A and 5C). A significantly higher level of radioactive label (P < 0.05) was also recovered in the liver at 14 h and a significantly lower level (P < 0.05) was found in the colon at 4 h when rats administered microencapsulated oil were fed after dosing (compare Fig. 5B and 5D).
Percentage of dose given recovered in GI tract tissues and lumen
The doses recovered, in GI tract tissue plus lumen for each section of the GI tract, are shown in Fig. 6. The data depicted in this way compensates for the efficiency of removal of radioactivity from the lumen by the washing procedure, as any unremoved radioactivity would still be retained on, or in the tissues. These data show that the trends obtained were largely similar to that for the individual tissue radiolabel distribution (see Fig. 3) and absolute amounts of radioactivity in the lumen (see Fig. 4).
![Time course showing percentage recovery of administered dose of radioactivity in gastrointestinal tract wall tissue plus percentage recovered in the lumen after dosing with [14C] trilinolenin as indicated either as unencapsulated (free) oil or microencapsulated oil (as indicated) fed after gavaging (A) or not fed after gavaging (B) for 4 h, 9h or 14 h as indicated. For simplicity, the small intestine (SI) is the combination of upper and lower small intestinal tissue. Data are expressed as mean ± SEM for n = 5 rats per group except for free oil group fed after dosing (4 h) microencapsulated oil group fed after dosing (9 h) where n = 4. Analyses between groups of free oil and microencapsulated oil and between fed and not fed treatments were by ANOVA with Bonferroni multiple comparison post tests. At equivalent time points and tissue sections, significant difference (P < 0.05) (1) between free oil and microencapsulated oil groups fed after dosing is indicated by a (2) between free oil and microencapsulated oil groups not fed after dosing by b (3) between free oil groups fed and not fed after dosing by c and (4) between microencapsulated groups fed and not fed after dosing by d.](/image/article/2011/FO/c1fo10149h/c1fo10149h-f6.gif) |
| Fig. 6 Time course showing percentage recovery of administered dose of radioactivity in gastrointestinal tract wall tissue plus percentage recovered in the lumen after dosing with [14C] trilinolenin as indicated either as unencapsulated (free) oil or microencapsulated oil (as indicated) fed after gavaging (A) or not fed after gavaging (B) for 4 h, 9h or 14 h as indicated. For simplicity, the small intestine (SI) is the combination of upper and lower small intestinal tissue. Data are expressed as mean ± SEM for n = 5 rats per group except for free oil group fed after dosing (4 h) microencapsulated oil group fed after dosing (9 h) where n = 4. Analyses between groups of free oil and microencapsulated oil and between fed and not fed treatments were by ANOVA with Bonferroni multiple comparison post tests. At equivalent time points and tissue sections, significant difference (P < 0.05) (1) between free oil and microencapsulated oil groups fed after dosing is indicated by a (2) between free oil and microencapsulated oil groups not fed after dosing by b (3) between free oil groups fed and not fed after dosing by c and (4) between microencapsulated groups fed and not fed after dosing by d. | |
Comparison between free oil and microencapsulated oil groups.
There was a trend of significantly increased recovery (P < 0.05) of the radiolabel at 9 and 14 h from the small intestine, at 4 and 9 h from the caecum and 9 h in the colon for the rats administered microencapsulated oil compared to those receiving free oil amongst rats that were fed after dosing (Fig. 6A). For the rats that were not fed post gavage, significantly higher recovery (P < 0.05) was observed in animals receiving microencapsulated oil at a broader range of time points; namely 4, 9 and 14 h in the small intestine, and 9 and 14 h in the caecum and the colon (Fig. 6B).
Comparison between rats fed and not fed after dosing.
The only significant difference was for the higher level of radiolabel (P < 0.05) in the stomach of rats that were fed free oil. There were no significant differences between the rats fed or not fed after dosing when rats were gavaged microencapsulated oil. This suggests that the presence of food has more of an influence on the metabolism of the oil when free oil is given compared to when the oil is delivered in a microencapsulated form.
Time course of relative distribution of radioactivity as percentage of total GI tract
The data in Fig. 7 denotes the relative distribution of radioactivity along the GI tract wall after dosing with free or microencapsulated oil. In all groups of rats administered free or microencapsulated oil that were either fed or not fed after dosing, the highest levels of radioactivity along the GI tract wall appears largely in the small intestine (∼64–86% of the total wall) with much lower amounts in the stomach (∼10–29% of the total wall).
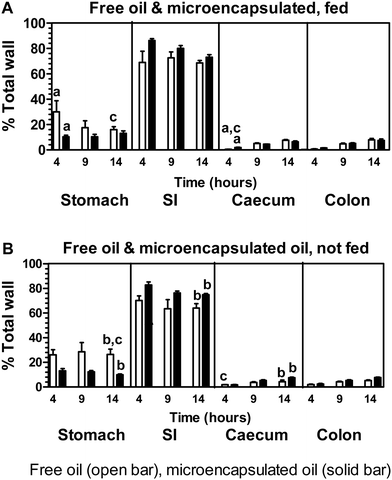 |
| Fig. 7 Time course of the relative distribution of radioactivity as percentage of total gastrointestinal (GI) tract tissue wall (100% in total). Tissues included stomach, upper and lower small intestine (combined data; SI), caecum and colon. Times are 4 h, 9 h and 14 h (as indicated) for unencapsulated (free) oil and microencapsulated oil fed after dosing (A) or not fed after dosing (B). Data are expressed as mean ± SEM for n = 5 rats per group except for free oil group fed after dosing (4 h) microencapsulated oil group fed after dosing (9 h) where n = 4. Analyses between groups of free oil and microencapsulated oil and between fed and not fed treatments were by ANOVA with Bonferroni multiple comparison post tests. At equivalent time points and tissue sections, significant difference (P < 0.05) (1) between free oil and microencapsulated oil groups fed after dosing is indicated by a (2) between free oil and microencapsulated oil groups not fed after dosing by b (3) between free oil groups fed and not fed after dosing by c and (4) between microencapsulated groups fed and not fed after dosing by d. The absence of the alphabet superscript “d” in the diagram that there were no significant differences for the specific comparison made. | |
Comparison between free oil and microencapsulated oil groups.
For rats fed after dosing, the only significant differences (P < 0.05) were the lower recovery of the radiolabel in the stomach tissue of rats fed microencapsulated oil at 4 h and the higher recovery of oil in the microencapsulated group in the caecum at 4 h. Although there was a higher recovery of the radiolabel in the small intestine for rats administered microencapsulated oil, these differences were not significant. Only low levels were found in the tissues of the caecum or colon irrespective of whether free oil or microencapsulated oil was delivered (Fig. 7A). Similar trends were found for rats that were not fed after dosing with the significant lower recovery of the radiolabel (P < 0.05) in the stomach and significantly higher (P < 0.05) recovery in the small intestine and colon all at 14 h for the rats administered microencapsulated oil compared to those gavaged with free oil (Fig. 7B). These data show that there was only slight protection against early uptake from the GI tract by microencapsulation.
Comparison between rats fed and not fed after dosing.
The trends for the accumulation in various tissues were similar, suggesting that feeding after dosing did not alter the distribution in the tissues, with the majority of the radiolabel being in the small intestine. The only significant differences for the effect of feeding after dosing were the higher accumulation in the stomach and caecum tissues for rats that were not fed after gavage with free oil (compare Fig. 7A and 7B).
Discussion
The higher recovery of the radiolabel in rats administered microencapsulated oil (77% for rats fed and non fed after dosing) compared to free oil (20% and 9%, respectively, for rats fed and fasted after dosing) immediately after gavaging suggests microencapsulation protected against an initial rapid interaction between the oil and the stomach tissue (presumably uptake or non-specific binding). The protection afforded by microencapsulation against immediate uptake in the stomach after gavaging, despite the absence of food in the non fed rats, suggests that the interface of the oil droplets, stabilised by the heated milk protein-carbohydrate matrix in the microencapsulated formulation, provided a barrier to gastric lipolysis that was absent when the free oil-in-water dispersion was exposed to stomach fluids. It has been suggested that soon after introduction of oil into a rodent's stomach (10–15 min), lipid droplets are seen within the endoplasmic reticulum of the stomach mucosal cells, which suggests that re-synthesis into triacylglycerols has occurred. Changes in the morphology and cellular physiology of the gut mucosal cells in fed and fasted states may also impact on the uptake of free oil. As an example, the rough endoplasmic reticulum (RER) of the intestinal absorptive cell extends to the area of the Golgi complex in the fasted state and after fat administration, the RER occupies a smaller area of the apical cytoplasm.1,37
The time course of transit of the radiolabel and its relative distribution along the GI tract were influenced by whether the rats were fed or fasted after dosing and the form of oil at delivery. Feeding drives lumenal free oil faster in the GI tract. As peristalsis is faster with feeding, the progress of the free oil is faster in fed animals. Microencapsulation slows down the lumenal progress of oil in the GI tract with feeding. Non feeding slows down the lumenal progress of free oil in the GI tract and this is not influenced by microencapsulation (Fig. 1). This may be due to slower peristalsis with non-feeding and consequently slower penetration into the GI tract. The rate of GI tract transit was not markedly affected by microencapsulation but a small protection against early uptake and metabolism in the stomach and upper GI tract was observed that was independent of the post-gavage feeding regimen. The main site of accumulation of the radiolabel along the GI tract wall tissues was in the small intestine, which has the largest available surface area relative to the other GI sections examined. In a previous study where fish oil was delivered in combination with tributyrin and resveratrol in a microencapsulated formulation, it was found that of the GI tract tissues, the small intestine also accumulated the most of the [14C-linolenin] radioactive label.38 The liver was the primary organ for accumulation of the radiolabel for both free and microencapsulated oils in rats whether fed or fasted after dosing.
Of the oil that remained in the lumen, there appeared to be a 3-fold greater amount of oil present in the caecum and colon of animals receiving microencapsulated oil, compared to that detected in the free oil group but these differences were not statistically significant. In a previous study, where rats were fasted overnight prior to administering microencapsulated oil and fed after dosing, there was also a 3-fold greater level of radioactivity detected in the caecum and colon.38 However, the absolute extent of protection against early uptake afforded by microencapsulation observed in the present study was much less than previously obtained where up to 60% of the radiolabel reached the large bowel where the same encapsulant formulation was used.38 The low levels of absolute recovery for the radiolabel in the lumen in this present study are more similar to those obtained for rats fed with a microencapsulated bioactive mixture of fish oil, resveratrol and tributyrin that were denied food for 9.5–14 h before dosing and again subsequently (with the exception of those on the 24 h time point) where food was reintroduced 8.5–11.5 h after dosing to avoid unacceptably prolonged fasting.34 It is possible that fasting and feeding conditions and biological variability in rats was in part responsible for the variability in GI transit of rats fed microencapsulated oil in the present study compared to the results previously obtained using the same microencapsulation system.38 Studies in humans have demonstrated that the postprandial metabolic response to lipid challenge of individuals were quite idiosyncratic.39
The total radioactivity recovered in all samples as a % of the administered dose was much lower in the non fed free oil group compared to all other groups (Fig. 2). This trend was reflected in the % dose recovered for individual tissues (Fig. 3) as well as that for the lumenal contents (Fig. 4) and confirmed by the absolute data for radioactivity uptake in the tissues (Fig. 5). The rapid absorption of oil in the non fed animals that were administered free oil is presumably followed by rapid re-packaging in the liver and distribution to peripheral tissues (where radioactivity was not measured in these experiments. In the absence of active peristalsis in the non fed animals, the time available for early absorption is longer than it would be with the more rapid transit that occurs in fed animals. Microencapsulation slows the absorption rate in non fed animals. It is suggested that the difference in the interfacial properties of protein-stabilised emulsions and that of oil droplets of the free oil-in-water dispersion accounts for the differences in the uptake of the oil. Structuring the interface of protein-stabilized oil emulsions has been suggested as a strategy for designing the delivery of lipophilic nutrients.5,10,11 In fed animals, peristalsis is rapid, the transit rate is faster and the time for absorption is lower and under these conditions, microencapsulation of oil does not affect the rate of transit.
Differences in rates of lipolysis may also be expected in fed and fasted states. Studies in humans have shown that there are changes in the composition, bile salt concentration, buffering capacity, surface tension, and osmolality in fed and fasted states in the stomach and duodenum.18,40 These physico-chemical changes in the GI tract in concert with attendant changes in the synthesis and secretion of digestive enzymes induced by the presence of food would be expected to impact on nutrient metabolism and uptake of nutrients. Food components can also modify not only the physical properties of the digesta but also affect intestinal motility, transit of digesta and absorption of nutrients.14 Changes in fat digestibility during transit may also be altered through physiologically-mediated effects of food. It has been suggested that lipolysis in rats is activated by fasting, an effect that may be linked to the hypothyroid status of rats that have been fasted.41,42 Others have suggested that fasting-induced lipolysis in rats is due to enhanced epinephrine-induced triacylglycerol breakdown as a result of a loss of the ability of insulin to suppress epinephrine-induced lipolysis.43
Conclusion
There is increasing interest in the use of microencapsulation for the delivery of omega-3 oils. This study showed that feeding after administration of oils increases the rate of progress of oil through the lumen and that microencapsulation delays the lumenal progression of oil in fed rats. The increases in the level of radiolabel in the liver and blood are consistent with an increased bioavailability of oil delivered in the microencapsulated format.
It is essential for more animal and human studies to be carried out to ascertain the bioavailability and bioequivalence of the various forms of delivery systems in both fed and fasted states. These should include administration of neat oils, liquid emulsions of oils stabilised by various emulsifiers, dried emulsion preparations of various formulations presented either on their own or as fortifying components of processed foods. The absorption of microencapsulated oil from the diet is not presently fully understood in rat models. However, these results confirm that microencapsulation of fish oil delays the uptake and metabolism of the oil, particularly in fed states. The results add to growing consensus that the food matrix in which lipids are presented may have an impact on patterns of lipid bioavailability, metabolism, uptake and bio-distribution.
Acknowledgements
Thanks are due to Li Jiang Cheng and Jenny Rusli for assistance in the preparation of emulsions and particle sizing.
References
- H. I. Friedman and B. Nylund, Intestinal fat digestion, absorption and transport, Am. J. Clin. Nutr., 1980, 33, 1108–1139 CAS.
- M. Armand, P. Borel, P. Ythier, G. Dutot, C. Melin, M. Senft, H. Lafont and D. Lairon, Effects of droplet size, triacylglycerol composition, and calcium on the hydrolysis of complex emulsions by pancreatic lipase: an in vitro study, J. Nutr. Biochem., 1992, 3, 333–341 CrossRef CAS.
- Y. Pafumi, D. Lairon, P. L. de la Porte, C. Juhel, J. Storch, M. Hamosh and M. Armand, Mechanisms of inhibition of triacylglycerol hydrolysis by human gastric lipase, J. Biol. Chem., 2002, 277, 28070–28079 CrossRef CAS.
- G. Favé, T. C. Coste and M. Armand, Physicochemical properties of lipids: new strategies to manage fatty acid bioavailability, Cell. Mol. Biol., 2004, 50, 815–831 Search PubMed.
- D. J. McClements, E. A. Decker, Y. Park and J. Weiss, Designing food structure to control stability, digestion, release and absorption of lipophilic food components, Food Biophys., 2008, 3, 219–228 CrossRef.
- D. J. McClements, E. A. Decker and Y. Park, Controlling lipid bioavailability through physiochemical and structural approaches, Crit. Rev. Food Sci. Nutr., 2009, 49, 48–67 CrossRef.
- M. Mu and C. E. Høy, The digestion of dietary triacylglycerols, Prog. Lipid Res., 2004, 43, 105–133 CrossRef.
- M. Armand, Lipases and lipolysis in the human digestive tract: where do we stand?, Curr. Opin. Clin. Nutr. Metab. Care, 2007, 10, 156–164 CrossRef CAS.
- I. Garaiova, I. A. Guschina, S. F. Plummer, J. Tang, D. Wang and N. T. Plummer, A randomised cross-over trial in healthy adults indicating improved absorption of omega-3 fatty acids by pre-emulsification, Nutr. J., 2007, 6, 4 CrossRef.
- M. Golding and T. J. Wooster, The influence of emulsion structure and stability on lipid digestion, Curr. Opin. Colloid Interface Sci., 2010, 15, 90–101 CrossRef CAS.
- A. M. Nik, A. J. Wright and M. Corredig, Interfacial design of protein-stabilized emulsions for optimal delivery of nutrients, Food Funct., 2010, 1, 141–148 Search PubMed.
- L. R. Ferguson, B. G. Smith and B. J. James, Combining nutrition, food science and engineering in developing solutions to inflammatory bowel diseases - omega-3 polyunsaturated fatty acids as an example, Food Funct., 2010, 1, 60–72 CAS.
- G. S. Duchateau and W. Klaffke, Product composition, structure and bioavailability, Food Biophys., 2008, 3, 207–212 CrossRef.
- R. G. Lentle and P. W. Janssen, Manipulating digestion with foods designed to change the physical characteristics of digesta, Crit. Rev. Food Sci. Nutr., 2010, 50, 130–145 CrossRef CAS.
- B. Pasquier, M. Armand, F. Guilloon, C. Castelain, P. Borel, J. L. Barry, G. Pieroni and D. Lairon, Viscous soluble dietary fibres alter the emulsification and lipolysis of triacylglycerols in duodenal medium in vitro, J. Nutr. Biochem., 1996, 7, 293–302 CrossRef CAS.
- M. Armand, M. Hamosh, J. S. Dipalma, J. Gallagher, S. B. Benjamin and J. R. Philpott,
et al., Dietary-fat modulates gastric lipase activity in healthy humans, Am. J. Clin. Nutr., 1995, 62, 74–80 CAS.
- G. J. Nelson and R. G. Ackman, Absorption and transport of fat in mammals with emphasis on n-3 polyunsaturated fatty acids, Lipids, 1988, 23, 1005–1014 CrossRef CAS.
- S. D. Maio and R. L. Carrier, Gastrointestinal contents in fasted state and post-lipid ingestion: In vivo measurements and in vitro models for studying oral drug delivery, J. Controlled Release, 2011, 151, 110–112 CrossRef.
- V. Karalis, P. Macheras, A. Van Peer and V. P. Shah, Bioavailability and bioequivalence: Focus on physiological factors and variability, Pharm. Res., 2008, 25, 1956–1962 CrossRef CAS.
- M. Y. Abeywardena and R. J. Head, Long chain n-3 polyunsaturated fatty acids and blood vessel function, Cardiovasc. Res., 2001, 52, 361–371 CrossRef CAS.
- G. S. Patten, M. J. Adams, J. A. Dallimore, P. F. Rogers, D. L. Topping and M. Y. Abeywardena, Restoration of depressed prostanoid-induced ileal contraction in spontaneously hypertensive rats by dietary fish oil, Lipids, 2005, 40, 69–79 CrossRef CAS.
- J. M. Wong, R. de Souza, C. W. Kendall, A. Emam and D. J. Jenkins, Colonic health: fermentation and short chain fatty acids, J. Clin. Gastroenterol., 2006, 40, 235–243 CrossRef CAS.
- C. H. S. Ruxton, S. C. Reed, M. J. A. Simpson and K. J. Milington, The health benefits of omega-3 polyunsaturated fatty acids: a review of evidence,, J. Hum. Nutr. Diet., 2007, 20, 275–285 CrossRef CAS.
- U. Gogusand and C. Smith, n-3 Omega fatty acids: a review of current knowledge, Int. J. Food Sci. Technol., 2010, 45, 417–436 CrossRef.
-
L. Sanguansri and M. A. Augustin, Microencapsulation in functional food product development, in Functional food product development, ed. J. Smith and E. Charter, Blackwell Publishing Ltd, 2010, pp. 3–23 Search PubMed.
- S. Drusch and S. Mannino, Patent-based review on industrial approaches for the microencapsulation of oils rich in polyunsaturated fatty acids, Trends Food Sci. Technol., 2007, 20, 237–244 CrossRef.
- U. Klinkesorn, P. Sophanodora, P. Chinachoti, D. J. McClements and E. A. Decker, Increasing the oxidative stability of liquid and dried tuna oil-in-water emulsions with electrostatic layer-by-layer deposition technology, J. Agric. Food Chem., 53, 4561–4566 CrossRef CAS.
- Z. Shen, M. A. Augustin, L. Sanguansri and L. J. Cheng, Oxidative stability of microencapsulated fish oil powders stabilized by blends of chitosan, modified starch, and glucose, J. Agric. Food Chem., 2010, 58, 4487–4493 CrossRef CAS.
- Y. L. Yep, D. Li, N. J. Mann, O. Bode and A. J. Sinclair, Bread enriched with microencapsulated tuna oil increases plasma docosahexaenoic acid and total omega-3 fatty acids in humans, Asia Pac. J. Clin. Nutr., 2002, 11, 285–291 CrossRef CAS.
- C. J. Barrow, C. Nolan and B. J. Holub, Bioequivalence of encapsulated and microencapsulated fish-oil supplementation, J. Funct. Foods, 2009, 1, 38–43 CrossRef CAS.
- B. Bajka, J. M. Clarke, D. L. Topping, L. Cobiac, M. Y. Abeywardena and G. S. Patten, Butyrylated starch increases large bowel butyrate levels and lowers colonic smooth muscle contractility in rats, Nutr. Res., 2010, 30, 427–434 CrossRef CAS.
- M. A. Augustin, P. Sanguansri and A. Htoon, Functional performance of a resistant starch ingredient modified using a microfluidiser, Innovative Food Sci. Emerging Technol., 2008, 9, 224–231 CrossRef CAS.
- M. I. Burgar, P. Hoobin, R. Weerakkody, L. Sanguansri and M. A. Augustin, NMR of microencapsulated fish oil samples during in vitro digestion, Food Biophys., 2009, 4, 32–41 CrossRef.
- M. A. Augustin, M. Y. Abeywardena, G. S. Patten, R. J. Head, T. J. Lockett, A. De Luca and L. Sanguansri, Effects of microencapsulation on the gastrointestinal transit and tissue distribution of a bioactive mixture of fish oil, tributyrin and resveratrol, J. Funct. Foods, 2011, 3, 25–37 CrossRef CAS.
- L. Bretillon, J. M. Chardigny, J. L. Sébédio, D. Poullain, J. P. Noël and J. M. Vatèle, Oxidative metabolism of mono-trans isomers of linoleic and α-linolenic acids in the rat, Biochim. Biophys. Acta, Lipids Lipid Metab., 1998, 1390, 207–214 CrossRef CAS.
- J. F. Mead, Lipid Metabolism, Annu. Rev. Biochem., 1963, 32, 241–248 CrossRef CAS.
- R. R. Cardell, S. Badenhausen and K. R. Porter, Intestinal triglyceride absorption in the rat, J. Cell Biol., 1967, 34, 123–155 CrossRef CAS.
- G. S. Patten, M. A. Augustin, L. Sanguansri, R. J. Head and M. Y. Abeywardena, Site specific delivery of microencapsulated fish oil to the gastrointestinal tract of the rat, Dig. Dis. Sci., 2009, 54, 511–521 CrossRef CAS.
- A. M. Zivkovic, M. M. Wiest, U. Nguyen, M. L. Nording, S. M. Watkins and J. B. German, Assessing individual metabolic responsiveness to a lipid challenge using a targeted metabolomic approach, Metabolomics, 2009, 5, 209–218 CrossRef CAS.
- L. Kalantzi, K. Goumas, V. Kalioras, B. Abrahamsson, J. B. Dressman and C. Reppas, Characterization of the human upper gastrointestinal contents under conditions simulating bioavailability/bioequivalence studies, Pharm. Res., 2006, 23, 165–176 CrossRef CAS.
- R. Ben Cheikh, A. Loireau, P. Chomard, P. Dumas and N. Autissier, Prolonged fasting on the lipolytic activity of isolated adipocytes of the rat epididymis, C.R. Seances Soc. Biol. Fil., 1989, 183, 232–239 CAS.
- R. Ben Cheikh, P. Chomard, P. Dumas and N. Autissier, Influence of prolonged fasting on thyroid hormone modulation of lipolysis in isolated epididymal adipocytes of Wistar rats, Eur. J. Endocrinol., 1994, 131, 516–521 CrossRef CAS.
- T. Szkudelski, M. Lisiecka, E. Nowicka, A. Kowalewska, L. Nogowski and K. Szkudelska, Short-term fasting and lipolytic activity in rat adipocytes, Horm. Metab. Res., 2004, 36, 667–673 CrossRef CAS.
|
This journal is © The Royal Society of Chemistry 2011 |
Click here to see how this site uses Cookies. View our privacy policy here.