DOI:
10.1039/C1FO10128E
(Review Article)
Food Funct., 2011,
2, 515-520
Natural salicylates: foods, functions and disease prevention
Received
24th June 2011
, Accepted 9th August 2011
First published on 30th August 2011
Abstract
Salicylic acid and related compounds are produced by plants as part of their defence systems against pathogen attack and environmental stress. First identified in myrtle and willow, the medical use of salicylate-rich preparations as anti-inflammatory and antipyretic treatments may date back to the third millennium BC. It is now known that salicylates are widely distributed throughout the plant kingdom, and they are therefore present in plant products of dietary relevance. In the UK, major food sources are tomato-based sauces, fruit and fruit juice, tea, wine, and herbs and spices. In mammalian cells, salicylic acid demonstrates several bioactivities that are potentially disease-preventative, including the inhibition of production of potentially neoplastic prostaglandins, which arise from the COX-2 mediated catalysis of arachidonic acid. Moreover, it appears to be readily absorbed from the food matrix. This has led some to suggestions that the recognised effects of consuming fruit and vegetables on lowering the risk of several diseases may be due, in part, to salicylates in plant-based foods. However, published estimates of daily salicylic acid intake vary markedly, ranging from 0.4 to 200 mg day−1, so it is unclear whether the Western diet can provide sufficient salicylates to exert a disease-preventative activity. Some ethnic cuisines that are associated with lowered disease risk may contain considerably more salicylic acid than is obtainable from a Western diet. However known protective effects of acetylsalicylic acid (Aspirin™) may have lead to an over-emphasis on the importance of dietary salicylates compared with other bioactive plant phenolics in the diet.
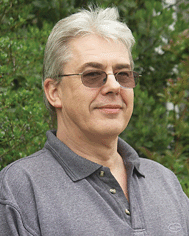
Garry G. Duthie
| Garry Duthie is Professor of Nutritional Science at the Rowett Institute of Nutrition and Health, University of Aberdeen. He is also Head of the Molecular Nutrition Research Group which investigates the biochemical, nutritional and molecular aspects of the mechanisms by which phytochemicals and micronutrients moderate cellular and genomic processes involved in the pathogenesis of diseases in man. Sources of research funding include the Scottish Government and the Department of Health, UK. |
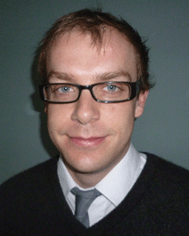
Adrian D. Wood
| Adrian Wood is a former Food Standards Agency Scholar who obtained his PhD from the University of Aberdeen in 2008. His research interests pertain to the role of phytochemicals and micronutrients in disease prevention. Currently he is a UK Department of Health funded Research Fellow in the Musculoskeletal Research Group at the University of Aberdeen, UK where he is conducting research on the influence of lifestyle factors (diet and physical activity) on cardiovascular function and risk with particular emphasis on inflammatory processes. |
Introduction
Salicylic acid (C7H6O3, 2-hydroxybenzoic acid), its phenyl glycoside and its carboxylated esters are constituents of many plants. Salicylic acid is a plant secondary metabolite with a simple phenolic structure (Fig. 1) that is mainly generated from the phenylpropanoid pathway via the β-oxidation of cinnamic acid and the subsequent hydroxylation of the resulting benzoic acid. It is primarily involved with the initiation of locally and systemically acquired resistance to pathogens and environmental stress.1 Other physiological processes in plants involving salicylic acid include the regulation of stomatal closure, the uptake of ions through the roots and the stimulation of flowering.2 In plants such as rice, barley and soybean, the level of salicylic acid is approximately 1 mg/100 g fresh mass.3 Certain bacteria also synthesise salicylic acid from isochorismate as a siderophore,4 but there is little evidence that it is produced endogenously by higher animals. Consequently, the detection of salicylic acid in the serum and urine of individuals not taking salicylate-based drugs suggests exogenous sources.5–7 As salicylic acid is widely distributed throughout the plant kingdom, its systemic presence in humans may arise from the consumption of plant based-foods. Salicylic acid and its acetylated form, Aspirin™, have a long history of therapeutic and disease-preventative use, particularly where chronic inflammation and oxidative stress are major components. This has led to the intriguing suggestion that the recognised effects of consuming fruit and vegetables on lowering disease risk may be due, in part, to salicylates in plant-based foods.8 Indeed, some believe that “salicylic acid deficiency” has important public health implications and that it should be classed as an essential vitamin, namely “Vitamin S”.9
Historical context
It is debatable whether a deficiency in salicylic acid is detrimental to health. However, there is considerable evidence that this plant phenolic acid has a marked bioactivity in mammalian cells. Extracts of certain plants, such as willow, myrtle, poplar and meadowsweet, which are rich in salicylates, have been used for millennia to treat human maladies. The Ebers papyrus (a collection of medicinal recipes dating back to the third millennium BC in Egypt) describes an infusion of dried myrtle leaves for easing the pain of rheumatism and for back problems. Ancient texts also indicate the medical use of willow bark and leaves by Babylonians, Assyrians and Chinese.10 An extract of willow bark was prescribed by Hippocrates of Cos for its analgesic effect, and its use to treat fever and to alleviate pain during childbirth is mentioned in the corpus Hippocratian, which was completed about 300 BC.10,11 In 1763, the Reverend Edward Stone informed the Royal Society that willow bark contained substances that effectively relieved the symptoms of “ague” (probably malarial fever).12 The active ingredient, salicylic acid, was isolated and purified from willow and meadowsweet in the first half of the 19th Century,13 and in 1860 was chemically synthesised by the carboxylation of sodium phenoxide.14 In perhaps the first documented controlled clinical trial, Thomas John MacLagan, a physician from Dundee, Scotland, established in 1876 that salicylic acid had antipyretic, analgesic and anti-inflammatory properties.15 Subsequently, large doses of bitter-tasting salicylic acid were routinely used to treat fever, pain and inflammation. In a (now known to be relatively unsuccessful) attempt to ameliorate the serious side effects of vomiting and ulceration of the stomach, salicylic acid was acetylated.16 Since its clinical introduction in 1899, under the trademark Aspirin™, acetylsalicylic acid remains the most commonly used drug in the world. Aspirin™ can be regarded as a prodrug as it is very rapidly de-acetylated after absorption from the stomach and small intestine. Consequently, much of its bioactivity (apart from the unique antiplatelet effect) in mammalian systems can be ascribed to salicylic acid.
Salicylates in foods and dietary intakes
The first published estimate of the salicylic acid content of a food appeared as a letter in the Lancet in 1903.17 The anonymous contributor states that it is present in strawberries and other fruits at a concentration of “1/64th of a grain/two pounds of fruit”. Subsequently, there have been several publications giving very disparate values for the salicylate content of similar food items.18–25 This can be ascribed, in part, to differences in the analytical methodology employed between studies. For example, redox colorimetric assays26 rely on ferric ions binding with the phenol and carboxyl groups of salicylic acid. Reactions with structurally related phenolic acids in foods may artefactually increase absorbance, leading to an overestimation of their salicylate content.27 In contrast, poor extraction efficiency through the use of inappropriate solvents and the co-elution of compounds that quench detection intensity may lead to an underestimation.22 Possibly the most definitive estimate to date of the salicylate content of foods is a systematic review of the literature,28 where data were accepted only if studies used food items randomly selected and purchased from various commercial outlets during different seasons of the year. Moreover, optimized sample extraction and hydrolysis conditions, and validation and quality assurance measures had to be clearly described. Salicylates are present in appreciable amounts in fruits and vegetables, wines, tea, fruit juices, herbs and spices (Table 1). However, despite a consistent methodology, there remains a marked variation of salicylate levels in different varieties of the same food item.28 For example, the salicylate content of five brands of orange juice ranged from 0.47 to 3.02 mg l−1. As with other phytochemicals, the salicylate content of primary food products is likely to be influenced by numerous other factors, including plant varieties, seasonality, growing conditions, storage and cooking.29
Table 1 Examples of total salicylate content of food itemsa
Food item |
Salicylates/mg kg−1 |
Food item |
Salicylates/mg kg−1 |
Adapted from ref. 28.
|
Fruits
|
Vegetables
|
Blackberries |
0.81 |
Asparagus |
1.29 |
Blueberries |
0.57 |
Carrots |
0.16 |
Gala melon |
0.62 |
Celery |
0.04 |
Grapefruit |
0.44 |
Green bean |
0.07 |
Green apple |
0.55 |
Mange tout |
0.20 |
Kiwi fruit |
0.31 |
Mushroom (button) |
0.13 |
Nectarine |
3.29 |
Onion (white) |
0.80 |
Strawberry |
0.61 |
Tomato |
0.13 |
Drinks
|
Spices and herbs
|
Apple |
0.83 |
Black cumin |
25.05 |
Cranberry |
0.99 |
Cumin
|
29.76 |
Grapefruit |
0.10 |
Chat masala |
5.74 |
Orange |
0.68 |
Garam masala |
12.85 |
Pineapple |
4.06 |
Paprika |
28.25 |
Tomato |
1.32 |
Turmeric |
20.88 |
White wine |
0.44 |
Thyme |
28.60 |
Red wine |
0.50 |
Mint |
54.20 |
Tea |
1.06 |
Fennel |
14.00 |
A lack of information on how varietal and growing conditions, and the effects of processing and storage affect the salicylate content of foods imposes a degree of uncertainty in the estimation of dietary intake. An early estimate of salicylate intakes in a Western population of up to 200 mg day−1 is generally regarded as being an overestimation.19 More recently, the application of semi-quantitative food frequency questionnaires to a food composition database28 estimated median daily salicylate intakes in the UK population of 4.4 and 3.2 mg day−1 for males and females, respectively. This is similar to estimated intakes in a Bavarian population calculated using 7-day weighed records,30 and is comparable with estimated intakes in a Scottish population of other phenolic compounds, including types of flavonols, flavones, flavonones and proanthocyanins.31 Major dietary sources of salicylates (Fig. 2) in the UK are alcoholic beverages (22%), herbs and spices (17%), fruit (16%) and non-alcoholic beverages, including fruit juice (13%), tomato-based sauces (12%) and vegetables (9%). Herbs and spices appear to be particularly rich sources of dietary salicylates (Table 1), meaning that UK populations, such as the Indian community (Fig. 2), who incorporate substantial amounts of such condiments in foods, may have markedly higher daily intakes of salicylates. For example, a typical south Indian vegetarian diet may provide 12–13 mg salicylic acid per day (Table 2). Additional oral sources of salicylates include toothpaste, mouthwash and food preservatives.32 However, it is unclear if these contribute significantly to the total daily intake.
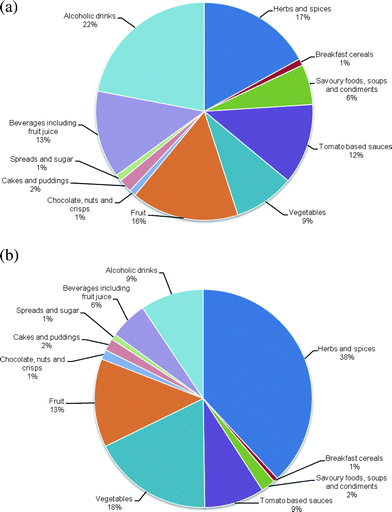 |
| Fig. 2 The relative contribution of different food groups to total salicylate intake in (a) Caucasian and (b) Indian males in NE Scotland. Adapted from ref. 2. | |
Table 2 Estimated salicylate content of a typical south Indian daily menua
Food
|
Weight/g |
Total salicylates/mg |
Salicylate content of foods derived from ref. 28. Daily menu provided by Parimala Swamy (personal communication).
|
Idly |
240 |
0.00 |
Tomato chutney |
100 |
0.29 |
Sambar |
50 |
0.58 |
Vada |
150 |
1.20 |
Rice |
200 |
0.00 |
Sambar |
100 |
1.15 |
Garlic rasam |
50 |
0.46 |
Pumpkin poriyal |
100 |
0.60 |
Ginger pickle |
5 |
0.29 |
Banana |
100 |
0.03 |
Roasted peanuts |
50 |
0.56 |
Dosa |
100 |
0.06 |
Mint chutney |
100 |
7.10 |
Banana |
100 |
0.03 |
TOTAL
|
1455
|
12.32
|
Mechanisms of action
From a nutritional perspective, the important question is whether dietary intakes of salicylic acid are sufficient to exert disease-preventative effects. Increased concentrations of salicylic acid and metabolites in serum and urine have been observed following both the acute and sustained consumption of salicylate-rich foods, including fruit, vegetables, cranberry juice and curries.22,33–37 Moreover, serum concentrations are linearly related to the number of servings consumed per day.33 This indicates that salicylic acid is readily absorbed from the food matrix, either passively or viaH+ and Na+ transport systems, and/or monocarboxylic acid transporters.38,39 Serum concentrations in such studies rarely exceed 2.5 μmol l−1 and are low compared with those achieved with the doses of Aspirin™ associated with the prevention of diseases such as colon cancer and vascular disease (Table 3). Empirically, any beneficial effects are likely to arise due to a low chronic exposure to salicylate-rich foods.
Table 3 Concentration of salicylic acid in the serum of different dietary groups compared with regular Aspirin™ takersa
Group
|
No. in group |
Concentration/μmol l−1 |
Median |
Range |
Data compiled from refs. 22 and 33.
|
Non-vegetarians |
39 |
0.07 |
0.02–0.20 |
Vegetarians |
37 |
0.11 |
0.04–2.47 |
Mediterranean |
36 |
0.12 |
0.03–0.29 |
South Indian |
21 |
0.26 |
0.05–0.64 |
Aspirin™ takers (75mg day−1) |
14 |
10.03 |
0.23–25.44 |
Rodent models using “nutritional” rather than “pharmaceutical” dietary intakes indicate that salicylic acid modulates a range of proteins involved in protein folding, transport, energy metabolism and cytoskeletal regulation.40,41 The biological significance of these changes is unclear. However, several of the identified proteins are known to be involved in two major redox pathways (thioredoxin and glutathione). This strongly suggests that salicylic acid modulates interactive components of the cellular redox system, such as glutathione S-transferase yb-2, p53 and AP-1.42 Oxidative stress is implicated in the pathogenesis of many diseases, including heart disease, diabetes and several cancers.43 Consequently, cellular exposure to low concentrations of salicylic acid may benefit health by regulating the activity or expression of transcription factors involved in modulating oxidative stress, such as the antioxidant response element.44 This potential effect is not, however, unique to salicylic acid as other dietary-derived phenolic compounds, such as flavonoids, also activate this enhancer sequence, thus mediating the transcriptional activation of genes in cells exposed to oxidative stress.45
A more recognised effect of salicylic acid is as an anti-inflammatory agent. Chronic inflammation appears to be linked to a wide range of progressive conditions, including several cancers, vascular disease and metabolic syndrome.46 Inflammation involves the enhanced expression of inducible genes, a process that is initiated by the binding of transcription factors to promoter regions.47 At concentrations detected in plasma (<10 μmol), salicylic acid appears to modify the binding of key transcription factors to the promoter region of genes that activate pro-inflammatory processes. For example, in cytokine-stimulated human endothelial vascular cells, concentrations of salicylic acid as low as 0.75 μmol suppress the synthesis of pro-inflammatory and neoplastic prostaglandins.48 This appears to occur by salicylic acid interacting with transcription factors such as the CCAT/enhancer binding protein-β (C/EBP-β) in the nucleus, with the consequent suppression of prostaglandin H2-synthase gene expression (also referred to as cyclo-oxygenase-2).49 Micromolar concentrations of salicylate also suppress the transcriptional activation of other genes involved in inflammatory reactions, such as iNOS. This would result not only in decreased prostaglandin synthesis but also a reduced exposure of the cell to potentially detrimental peroxynitrite.50 However, the higher salicylate concentrations (> 1 mmol) required to inhibit the activation of NFκB,51 a transcription factor that activates genes involved in inflammatory responses and apoptosis, are unlikely to be achieved by diet alone.
Numerous in vitro studies suggest that salicylic acid regulates many other diverse cellular targets, including the apoptopic genes Par-452 and Bcl-XL,53p38 MAP kinase,54 mitochondrial cytochrome c release,55phenol sulfotransferase activity,56DNA mismatch repair57 and mitochondrial calcium ion uptake.58 In many cases, such effects require exposure to concentrations of salicylates in excess of 10 mmol. While this is obviously interesting from a pharmacological perspective, it is extremely unlikely that they have important biological significance at the lower concentrations achieved through the consumption of salicylate-rich foods.
Disease prevention
Several recent systematic reviews reinforce the view that the habitual consumption of plant-based foods decreases the risk of developing diseases whose clinical horizon is middle age.59–61 Which dietary components, either singly or in combination, confer such protection remains unclear.62 Fibre, antioxidant vitamins, plant lipids and various phytochemicals all have their advocates. However, the results of intervention trials with such dietary components have generally failed to have a major effect on morbidity and mortality.63–66 In contrast, there are a substantial number of intervention trials suggesting that the consumption of salicylates, albeit in an acetylated form, prevent or delay the development of several cancers and cardiovascular disease. These benefits appear to transcend study designs and cohort characteristics (e.g. age, gender, nationality).67
The bioactivity of Aspirin™ is unique in that it is characterised by two compounds within the same molecule. In addition to salicylic acid, the acetyl moiety is released within minutes of Aspirin™ consumption by enzymatic hydrolytic cleavage by esterases in the intestine, blood and liver.68 The binding of the acetyl group to serine530 in platelets inactivates the active site of cycloxygenase-1, thus preventing the access of arachidonic acid and irreversibly inhibiting prostaglandin synthesis. The resultant prevention of platelet aggregation is a major reason for the widespread use of Aspirin™ in the prevention and treatment of cardiovascular disease.69 However, the other primary metabolite of Aspirin™, salicylic acid, has a much longer half-life of several hours.70 It is likely that its broad spectrum of cellular targets, already discussed, contributes to disease prevention through, for example, its anti-inflammatory, antiproliferative and antioxidant activity. This view is supported by meta-analyses,71–73 which strongly corroborate the hypothesis that the regular consumption of Aspirin™-derived salicylic acid can inhibit the incidence and progression of several diseases where platelet function is not a major consideration. However, the doses employed in such trials (30–1300 mg day−1) exceed that which can be obtained from diet alone. Current clinical data are most supportive of a 75 mg daily dose of Aspirin™74 (equivalent to 66 mg salicylic acid), which is readily available from pharmacies and retail outlets in the UK. Larger doses may be required to obtain significant anti-cancer effects, although a recent population study75 showed for the first time that doses of around 100 mg day−1 may confer some protection. This does not preclude the possibility that the sustained lower intakes achievable by the regular consumption of salicylate-rich diets may also have a disease-preventative activity. However, to date, no double-blind randomised controlled trials using doses of 5–10mg salicylic acid have been conducted in support of the hypothesis that dietary salicylates have long term health benefits. It should also be pointed out that even with therapeutic doses of acetylated salicylic acid of 75mg day−1, there is a peptic ulcer incidence of 5–10% over 3–6 months of usage, a bleeding complication rate of 0.5–2.0 per 100 patient years and a mortality rate of ulcer complications of about 5% of those who have been admitted to hospital due to ulcer bleeding.76 It is likely that the risk of GI toxicity from salicylate levels in food is low, but as a note of caution, Aspirin™ intakes as low as 10mg day−1 are reported to produce demonstrable gastric damage in humans.77
Future perspectives
Interest in the potential beneficial effects of dietary salicylates has arisen, in part, because of the extensive literature on the disease-preventative effects of Aspirin™. However, it should not be forgotten that plant products found to contain salicylic acid are generally rich sources of other phenolic acids. For example, rich sources of hydroxycinnamic acids such as ferulic, synapic and caffeic acids include legumes, cocoa, fruit, herbs, nuts and cereals. In addition to salicylic acid, other hydroxybenzoic acids such as protocatechuic, vanillic and syringic acids are present in wine, berries, herbs, fruit juices and tea.78 Daily intakes of many may markedly exceed that of salicylic acid. Estimated intakes of caffeic and ferulic acid in a Finnish population are 417 and 129 mg, respectively.79 Many of these compounds also have a marked anti-inflammatory and redox-related bioactivity in mammalian cells.80 Their potential protective effects should not be overlooked. In this context, the importance of dietary salicylic acid should not perhaps be over emphasised.
Acknowledgements
Funding is gratefully acknowledged from the Scottish Government Rural and Environment Science and Analytical Services Division (RESAS) and the Food Standards Agency.
References
- N. Yalpani, J. Leon, M. A. Lawton and I. Raskin, Plant Physiol., 1993, 57, 35.
- W. S. Pierpoint, Adv. Botanical Res., 1994, 20, 164 Search PubMed.
- I. Raskin, H. Skubatz, W. Tang and B. J. D. Meeuse, Ann. Bot., 66, 376 Search PubMed.
- J. M. Meyer, P. Azelvandre and C. Georges, Biofactors, 1992, 4, 23 Search PubMed.
- M. D. Armstrong, K. N. F. Shaw and P. I. Wall, J. Biol. Chem., 1956, 218, 293 CAS.
- M. D. A. Finnie, R. S. Ersser, J. W. T. Seakens and W. Sneddon, Clin. Chim. Acta, 1976, 70, 171 CrossRef CAS.
- J. R. Paterson, C. Blacklock, G. Campbell, D. Wiles and J. R. Lawrence, J. Clin. Pathol., 1998, 51, 502 CrossRef CAS.
- J. R. Paterson and J. R. Lawrence, Q. Med. J., 2001, 94, 445 Search PubMed.
- G. Morgan, Eur. J. Public Health, 2003, 13, 283 CrossRef.
- G. Sharp, Pharmaceut. J., 1915, 94, 857 Search PubMed.
- N. H. Aboelsoud, J. Med. Plants Res., 2010, 4, 82 Search PubMed.
- E. Stone, Philos. Trans. R. Soc. London, 1763, 53, 195 CrossRef.
- H. Leroux, J. Clin. Med., 1830, 6, 340 Search PubMed.
- H. Kolbe, Liebigs Ann. Chim., 1860, 113, 125 CrossRef.
- T. J. MacLagan, Lancet, 1876, 107, 342 Search PubMed.
- W. Sneader, Br. Med. J., 2000, 321, 1591 CrossRef CAS.
- Anon, Lancet, 1903, 162, 1187 CrossRef.
- A. R. Swain and S. P. Dutton, J. Am. Diet. Assoc., 1985, 85, 950 CAS.
- D. P. Venema, P. C. H. Hollman, P. L. T. M. Janssen and M. B. Katan, J. Agric. Food Chem., 1996, 44, 1762 CrossRef CAS.
- P. L. T. M. Janssen, M. B. Katan and W. A. van Staveren, Cancer Lett., 1997, 114, 163 CrossRef CAS.
- G. J. Baxter, A. B. Graham, J. R. Lawrence and J. R. Paterson, Eur. J. Nutr., 2001, 40, 289 CrossRef CAS.
- J. R. Paterson, R. Srivatsava, G. J. Baxter, A. B. Graham and J. R. Lawrence, J. Agric. Food Chem., 2006, 54, 2891 CrossRef CAS.
- M. J. Scotter, D. P. T. Roberts, L. A. Wilson, F. A. C. Howard, J. Davis and N. Mansell, Food Chem., 2007, 105, 273 CrossRef CAS.
- G. L. Robertson and W. J. Kermode, J. Sci. Food Agric., 1982, 32, 833.
- K. Herman, Ernährungs-Umschau, 1990, 37, 108 Search PubMed.
-
Salicylic Acid in Foods: Qualitative and Quantitative Analysis No. 3, NMKL (Nordic Committee on Food Analysis), 3rd edn, 1999, 8 pp. Search PubMed.
- A. Wood, G. Baxter, J. Paterson, F. Thies and G. Duthie, Proc. Nut. Soc., 2005, 64, 87A Search PubMed.
- A. Wood, G. Baxter, F. Thies, J. Kyle and G. Duthie, Mol. Nutr. Food Res., 2011, 55, S7 CrossRef.
- R. Cermak, A. Durazzo, G. Maiani, V. Böhm, D. R. Kammerer, R. Carle, W. Wiczkowski, M. K. Piskula and R. Galensa, Mol. Nutr. Food Res., 2009, 53, S184–S193 CrossRef.
- J. Radtke, J. Linseisen and G. Wolfram, Z. Ernahrungwiss, 1998, 37, 190 Search PubMed.
- J. Kyle, L. F. Masson, D. A. Grubb, G. G. Duthie and G. McNeill, Proc. Nut. Soc., 2002, 61, 69A Search PubMed.
-
M. Ash, I. Ash, Handbook of Preservatives, Synapse Information Inc., New York, 2004, 518 pp Search PubMed.
- A. Spadafranca, S. Bertoli, G. Fiorillo, G. Testolin and A. Battezzati, Br. J. Nutr., 2007, 98, 802 CAS.
- G. G. Duthie, J. A. M. Kyle, A. McE. Jenkinson, S. J. Joyce, G. J. Baxter and J. R. Paterson, J. Agric. Food Chem., 2005, 53, 2897 CrossRef CAS.
- K. Zhang and Y. Zuo, J. Agric. Food Chem., 2004, 52, 227.
- C. J. Blacklock, J. R. Lawrence, D. Wiles, E. A. Malcolm, I. H. Gibson, C. J. Kelly and J. R. Paterson, J. Clin. Pathol., 2001, 54, 553 CrossRef CAS.
- J. R. Lawrence, R. Peter, G. J. Baxter, J. Robson, A. B. Graham and J. R. Paterson, J. Clin. Pathol., 2003, 56, 651 CrossRef CAS.
- Y. Konishi, Z. H. Zhao and M. Shimizu, J. Agric. Food Chem., 2006, 54, 7539 CrossRef CAS.
- Y. Konishi and M. Shimizu, Biosci., Biotechnol., Biochem., 2003, 67, 856 CrossRef CAS.
- J. E. Drew, J. R. Arthur, A. J. Farquarson, W. R. Russell, P. C. Morrice and G. G. Duthie, Biochem. Pharmacol., 2005, 70, 888 CrossRef CAS.
- J. E. Drew, S. Padidar, G. Horgan, G. G. Duthie, W. R. Russell, M. Reid, G. Duncan and G. J. Rucklidge, Biochem. Pharmacol., 2006, 72, 204 CrossRef CAS.
- C. Abate, L. Patel, F. J. Rauscher and T. Curran, Science, 1990, 249, 1157 CrossRef CAS.
- H. Sies, W. Stahl and A. Sevanian, J. Nutr., 2005, 135, 969 CAS.
- T. H. Rushmore, M. R. Morton and C. B. Pickett, J. Biol. Chem., 1991, 266, 11632 CAS.
- T. Nguyen, P. J. Sherratt and C. B. Picket, Annu. Rev. Pharmacol., 2003, 43, 233 Search PubMed.
- M.-H. Pan, C.-S. Lai and C.-T. Ho, Food Funct., 2010, 1, 3–10 RSC.
- C. M. Kaefer and J. A. Milner, J. Nutr. Biochem., 2008, 19, 347–361 CrossRef CAS.
- K. K. Wu, R. Sanduja, A. L. Tsai, B. Ferhanoglu and D. S. Loose-Mitchell, Proc. Natl. Acad. Sci. U. S. A., 1991, 88, 2384 CrossRef CAS.
- M. A. Saunders, L. Sansores-Garcia, D. W. Gilroy and K. K. Wu, J. Biol. Chem., 2001, 276, 18897 CrossRef CAS.
- R.l. Radi, G. Peluffo, M. Alvarez, M. Naviliat and A. Cayota, Free Radical Biol. Med., 2001, 30, 463 CrossRef CAS.
- L. A. Stark, F. V. N. Din, R. M. Zwacka and M. G. Dunlop, FASEB J., 2001, 15, 1273 CAS.
- Z. Zhang and R. N. DuBois, Gastroenterology, 2000, 118, 1012 CrossRef CAS.
- L. Zhang, J. Yu, B. H. Park, K. W. Kinzler and B. Vogelstein, Science, 2000, 290, 989 CrossRef CAS.
- P. Schwenger, P. Bellosta, I. Vietor, C. Basilico, Y. Skolnik and J. Vilcek, Proc. Natl. Acad. Sci. U. S. A., 1997, 94, 2869 CrossRef CAS.
- K. C. Zimmermann, N. J. Waterhouse, J. C. Goldstein, M. Schuler and D. R. Green, Neoplasia, 2000, 2, 505 CrossRef CAS.
- R. E. Harris, J. Beebe-Donk, H. Doss and D. Burr-Doss, Oncology Rep., 2005, 13, 559 Search PubMed.
- A. Goel, D. K. Chang, L. Ricciardiello, C. Gasche and C. R. Boland, Clin. Cancer Res., 2003, 9, 383 CAS.
- L. Nunez, R. A. Valero, L. Senovilla, S. Sanz-Blasco, J. Garcia-Sancho and C. Villalobos, J. Physiol., 2006, 571, 57 CAS.
- L. Dauchet, P. Amouyel, S. Hercberg and J. Dallongeville, J. Nutr., 2006, 136, 2566.
- F. J. He, C. A. Nowson and G. A. MacGregor, Lancet, 2006, 367, 320 CrossRef.
- N. Lunet, A. Lacerda-Viera and H. Barros, Nutr. Cancer, 2005, 53, 1 Search PubMed.
- K. Brandt, L. P. Christensen, J. Hansen-Møller, S. L. Hansen, J. Haraldsdotter, L. Jespersen, S. Purup, A. Kharazmi, V. Barkholt, H. Frøkiaer and M. Kobaek-Larsen, Trends Food Sci. Technol., 2004, 15, 384 CrossRef CAS.
- D. S. Alberts, M. E. Martínez and D. J. Roe, N. Engl. J. Med., 2000, 342, 1156 CrossRef CAS.
- D. Albanes, O. P. Heinonen, J. K. Huttunen, P. R. Taylor, J. Virtamo, B. K. Edwards, J. Haapakoski, M. Rautalahti, A. M. Hartman and J. Pamgren, Am. J. Clin. Nutr., 1995, 62, 1427S CAS.
- G. Bobe, L. B. Sansbury and P. S. Albert, Cancer Epidemiol., Biomarkers Prev., 2008, 17, 1344 Search PubMed.
- G. Bjelakovic, D. Nikolova, L. L. Gluud, R. G. Simonetti and C. Gluud, JAMA, J. Am. Med. Assoc., 2007, 297, 842 CrossRef CAS.
- P. C. Elwood, A. M. Gallagher, G. G. Duthie, L. A. Muir and G. Morgan, Lancet, 2009, 373, 1301 CrossRef CAS.
- P. B. Costello, J. A. Caruana and F. A. Green, Arthritis Rheum., 1984, 27, 422 CrossRef CAS.
- J. B. Smith and A. L. Willis, Nature, 1971, 231, 235 CAS.
- M. Rowland and S. Riegelman, J. Pharmaceut. Sci., 1968, 41, 407 Search PubMed.
- J. Cuzick, F. Otto, J. Baron, P. Brown, J. Burn, P. Greenwald, J. Jankowski, C. La Vecchia, F. Meyskens, H. Jörg Senn and M. Thun, Lancet Oncol., 2009, 10, 501 CrossRef CAS.
- D. A. Corley, K. Kerlikowske, R. Verma and P. Buffler, Gastroenterology, 2003, 124, 47 CrossRef CAS.
- Antithrombotic Trialists' (ATT) Collaboration, Lancet, 1849, 2009, 373 Search PubMed.
- C. L. Campbell, S. Smyth, G. Montalescot and S. R. Steinhubl, JAMA, J. Am. Med. Assoc., 2007, 297, 2018 CrossRef CAS.
- F. V. N. Din, E. Theodoratou, S. M. Farrington, A. Tenesa, R. A. Barnetson, R. Cetnarskyj, L. Stark, M. E. Porteous, H. Campbell and M. G. Dunlop, Gut, 2010, 59, 1670 CrossRef CAS.
- N. D. Yeomans, A. I. Lanas, N. J. Talley, A. B. R. Thomson, R. Daneshjoo, B. Eriksson, S. Appelman-Eszczuk, G. Langstrom, J. Naesdal, P. Serrano, M. Singh, M. M. Skelly and C. J. Hawkey, Aliment. Pharmacol. Ther., 2005, 22, 795 CrossRef CAS.
- B. Cryer and M. Feldman, Gastroenterology, 1999, 117, 17 CrossRef CAS.
- W. Russell, A. Labat, L. Scobbie, G. J. Duncan and G. G. Duthie, Food Chem., 2009, 115, 100 CrossRef CAS.
- M.-L. Ovaskainen, R. Törrönen, J. M. Koponen, H. Sinkko, J. Hellström, H. Reinivuo and P. Mattila, J. Nutr., 2008, 138, 562 CAS.
- W. R. Russell, L. Scobbie, A. Chesson, A. J. Richardson, C. S. Stewart, S. H. Duncan, J. E. Drew and G. G. Duthie, Nutr. Cancer, 2008, 60, 636 Search PubMed.
|
This journal is © The Royal Society of Chemistry 2011 |
Click here to see how this site uses Cookies. View our privacy policy here.