DOI:
10.1039/C1FO10026B
(Review Article)
Food Funct., 2011,
2, 224-234
Inhibition of advanced glycation endproduct formation by foodstuffs
Received
16th February 2011
, Accepted 19th March 2011
First published on 8th April 2011
Abstract
The Maillard reaction, which is generally termed nonenzymatic browning or glycation, has been implicated in accelerated aging and diabetic complications in vivo. Although the molecular basis of glycation-induced pathogenesis is not well understood, the following have been noted: (1) protein glycation leads to the formation and accumulation of toxic advanced glycation endproducts (AGEs); (2) AGEs can permanently alter the structure and function of body proteins; and (3) the interaction between AGE-modified proteins and AGE-specific receptors (RAGEs) on the cell surface induces the overproduction of reactive oxygen species (ROSs) and inflammatory mediators, which leads to cellular disorders in biological systems. To date, studies that have examined the contribution of protein glycation to disease-states have primarily focused on the deleterious effects and related mechanisms of these glycotoxins. However, it remains unknown whether phytochemicals exert protective effects against glycotoxin-induced damage. Thus, the development and investigation of AGE inhibitors, especially the natural anti-AGE agents without adverse effects, may provide a therapeutic approach for delaying and preventing premature aging and diabetic complications. In this review, we provide an outline of anti-glycation properties of foodstuffs and/or their active components, and discuss their mechanisms of action.
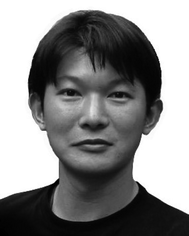 Chi-Hao Wu | Chi-Hao Wu is a post-doctoral fellow in the Department of Food Science and Biotechnology at the National Chung Hsing University (NCHU). He received his PhD in Food Science and Biotechnology from NCHU, under the guidance of Professor Gow-Chin Yen, in 2009. His research interests are functional Food chemistry and bioactivity, and his research interests cover topics such as the in vivo Maillard reaction, oxidative stress, inflammation, and nutrigenomics. |
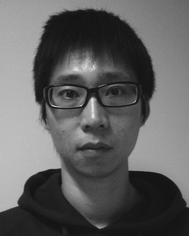 Shang-Ming Huang | Shang-Ming Huang is a PhD candidate in the Department of Food Science and Biotechnology at the National Chung Hsing University (NCHU). His research interests concern the relationship between dietary agents and nutrigenomics in human health, and his current research focuses on antidiabetic effect, modulating inflammasome and autophagy activation with dietary phytochemicals for therapeutic benefit. |
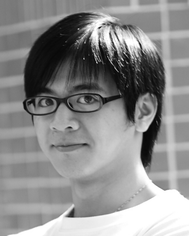 Jer-An Lin | Jer-An Lin is a PhD candidate in the Department of Food Science and Biotechnology at the National Chung Hsing University (NCHU). His current research involves the bio-function of nutraceuticals in cancer prevention. |
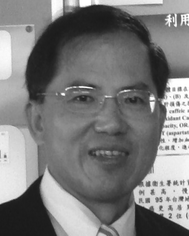 Gow-Chin Yen | Gow-Chin Yen received his PhD in Food Science and Nutrition from University of Rhode Island. Currently he is a Chair Professor in the Department of Food Science and Biotechnology at the National Chung Hsing University, Taiwan. He has won numerous awards, including being elected a Fellow of the International Academy of Food Science and Technology, and elected a Fellow of the Division of Agricultural and Food Chemistry of the American Chemical Society. His current research interests focus on molecular food chemistry and functional foods, anti-glycation effects and cancer chemoprevention with dietary phytochemicals. |
1. Introduction
Protein glycation, which is also known as the Maillard reaction or nonenzymatic browning, was originally described by Louis Maillard.1 It involves a series of complex reactions that occur between sugars and amino acids or proteins, and may result in a yellowish-brown color change. The Maillard reaction is an important reaction that occurs in foodstuffs during heating, processing and storage, and it can lead to a wide variety of food-associated phenomena, including flavors, aromas and colors. Similarly, this reaction also occurs in vivo and is seen in conjunction with elevated blood sugar levels. However, this reaction results in both positive and negative effects on food quality and safety; thus, the reaction has been studied in various fields for its chemical, physiological and toxicological properties.2–4
Diabetes is a life-long disease marked by high levels of blood sugar. The resulting nonenzymatic glycation of biological proteins that promote the irreversible formation of reactive advanced glycation endproducts (AGEs) is a significant factor associated with hyperglycemia.5 For example, the importance of the glycation reaction was investigated following the discovery of HbA1c in diabetic patients.6 Glycation occurs in vivo through the covalent binding of aldehyde or ketone groups of reducing sugars to free the amino groups of proteins, forming AGE structures that may have a yellowish-brown color and/or fluorescence. Recent evidence suggests that AGEs have a propensity to generate reactive oxygen species (ROS).7 Furthermore, glucose and other aldehydes, free or protein-bound, can undergo auto-oxidation reactions and generate radicals and other reactive intermediates (e.g., H2O2 and other peroxides) that contribute to AGE formation. This process is often termed glycoxidation.8
To study the significance of glycoxidative stress on the pathology of diabetes, the effect of antioxidant supplementations that inhibit protein modifications must be examined under diabetic conditions; the ability of these supplements to be effective in diabetic complications has been hypothesized.7,9,10 This hypothesis has been supported by clinical results, indicating that the development of diabetes and accumulation of AGEs may be reduced by the intake of natural antioxidants through the diet.11 Fruits, vegetables and beverages are important dietary sources of natural polyphenols.12 These polyphenolics are of current research interest due to their important biological and pharmacological properties, which can be attributed to their antioxidant capacities.13 However, the role of phytochemicals, specifically their ability to protect against glycotoxin-induced damage, remains unknown. This review summarizes a series of plant extracts and phytochemicals that have been identified as promising inhibitors of protein glycation and oxidative and inflammatory injury induced by AGEs in vitro and in vivo. Because these natural compounds and/or extracts behave in a similar fashion to aminoguanidine (AG), the first AGE inhibitor tested in clinical trials, they have great potential as therapeutic agents to alleviate diabetic complications.
2.
Protein glycation and AGE formation
The Maillard reaction is a series of complex reactions between the carbonyl group of a reducing sugar and the amino group of a protein. The reaction process is similar to a free-radical chain reaction, and generally consists of three stages: initiation, propagation and an advanced stage (Fig. 1). The reaction usually takes several days to several weeks to complete; the reaction rate is extraordinarily low because no enzymatic catalysis is involved.
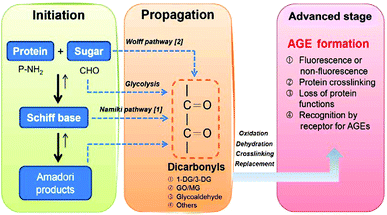 |
| Fig. 1 Chemical pathways leading to AGE formation. Schiff bases are produced during reducing glucose-protein reaction. Reactive dicarbonyl precursors of AGEs are formed through the degradation of Schiff base intermediates (Namiki pathway) or through metal-catalyzed autoxidation of the reducing sugar (Wolff pathway). | |
During the initiation reaction, a reducing sugar, such as glucose, is coupled to the free amino group of amino acids, peptides or proteins to yield an unstable Schiff base, which then leads to a relatively stable ketoamine known as an Amadori product. These reactions are all reversible up to the point of the synthesis of the Amadori product, and the equilibrium is highly dependent on substrate concentrations and incubation time. It is of note that the Schiff base is highly prone to oxidation and free-radical generation, which leads to the formation of reactive carbonyl intermediates.7Glucose itself can auto-oxidize to form hydrogen peroxide and keto aldehydes in the presence of transition-metal ions,8 and subsequently accelerate the formation of AGEs. The relationships between oxidation and glycation are thus extremely complex.
During the propagation reaction, the Amadori products react with the amino acids to form Nε-(carboxymethyl)lysine (CML) or regenerate amines through metal-ion-mediated catalysis and oxygen-induced oxidation, while the glycosyl group is dehydrated to form deoxyglucosone (DG). These steps comprise the intermediate stage of the Maillard reaction.
During the advanced stage of the reaction, the highly reactive DG reacts with lysine residues in the protein to produce pyrraline, or a pentose reacts with the lysine and arginine residues in the protein to generate pentosidine and other products. Consequently, intermolecular or intramolecular heterocyclic crosslinking and fragmentation occur in the protein molecule, leading to protein denaturation and irreversible damage. In addition to pyrraline and pentosidine, other types of irreversible AGEs can be generated from the Amadori products, including CML, Nε-(carboxyethyl)lysine (CEL), 2-(2-furoyl)-4(5)-(2-furanyl)-1H-imidazole (FFI), glyoxal-lysine dimer (GOLD) and methylglyoxal-lysine dimer (MOLD), as well as other compounds that have not yet been identified. The final products of the Maillard reaction, AGEs, have high heat stability, and thus these steps constitute the final stage of the Maillard reaction.
On the basis of chemical structure, two types of AGEs may be distinguished by their fluorescence properties and crosslinking structures: (A) fluorescent and crosslinking AGEs, such as crossline, FFI, GOLD, MOLD, fluorolink, pentosidine and vesperlysine A, B and C (Fig. 2A), and (B) non-fluorescent and non-crosslinking AGEs, such as argpyrimidine, CML, Nε-(carboxyethyl)lysine (CEL) and pyrraline (Fig. 2B).
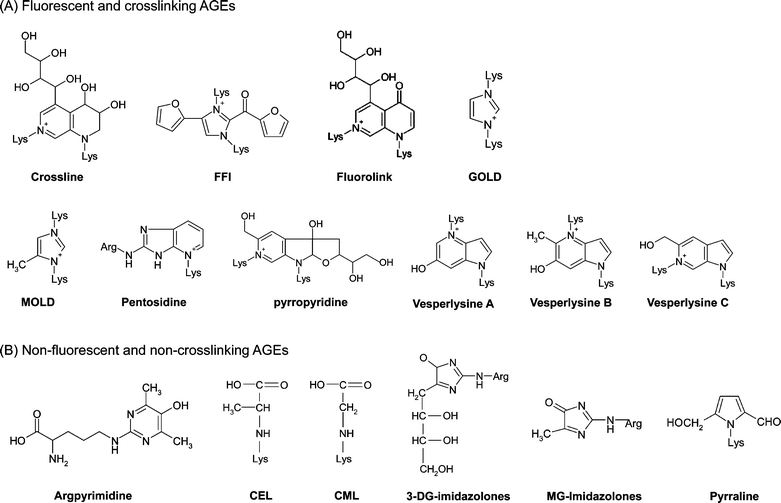 |
| Fig. 2 Chemical structures of two types of AGEs: (A) fluorescence and crosslinking AGEs; (B) non-fluorescence and non-crosslinking AGEs. | |
In addition, the glycation intermediates and the two reactive carbonyl groups, namely the reactive carbonyl species (RCSs), are of great importance for the cytotoxicity of AGEs. These toxic AGE precursors, such as glyoxal (GO), methylglyoxal (MG) and 3-deoxyglucosone (3-DG) (Fig. 3), are characteristic of individuals susceptible to diabetic complications. Although the chemical reactions leading to AGE formation are nonenzymatic, the RCS levels are ultimately controlled by enzymatic mechanisms involving glyoxalase, MG and 3-DG reductase. The RCS intermediates act as potent crosslinkers. Protein crosslinking results in aggregates that form intracellular protease-resistant and ubiquitin-proteasome-resistant deposits, and consequently affect specific protein functions. Therefore, formation of RCSs is considered the key event in the intermediate stage of protein glycation.14
3. Physiological and pathophysiological significance of protein glycation
A more succinct descriptive term for AGEs is glycotoxins, because they are toxic to the body when they are present for a prolonged period.15AGE accumulation in vivo has been implicated as a major factor in the pathogenesis of diabetic complications and other health disorders. Clinical evidence and experimental data have shown that AGE levels are particularly high in diabetic patients, and elevated blood sugar levels accelerate protein glycation, facilitating AGE formation.5 Hyperglycemia and hyperglycemia-associated glycotoxins also play a crucial role in chronic inflammatory processes that can cause cells to emit signals that trigger the overproduction of ROSs and inflammatory mediators through both the AGE receptor (RAGE)-dependent and RAGE-independent pathways.16
As mentioned above, nonenzymatic glycation has both physiological and pathophysiological significance. AGEs are now known to contribute to accelerated microvasculopathies and macrovasculopathies observed in diabetes. Enhanced AGE formation occurs in the blood and tissues of diabetics and in various other pathophysiological conditions, such as atherosclerosis, Alzheimer's disease, end-stage renal disease, rheumatoid arthritis and liver cirrhosis. The reaction products may act as oxidative stressors or agents that can contribute to a high risk of diabetes-associated complications.4,5,7 Nagai et al.17 have reported that AGE deposition can be observed in macrophages and located in atherosclerotic lesions. In addition, AGE concentration in tissues is positively correlated with the severity of atherosclerotic lesions and the quantity of plasma proteins, lipoproteins and lipids accumulated in the vascular wall.18 Thus, AGEs play an important role in the formation of atherosclerosis. Reducing or inhibiting AGE formation may be effective in delaying and preventing the development and progression of diabetes and its complications.
4. Proposed mechanisms of anti-glycation
In general, the biochemical mechanisms of anti-glycation reactions include any mechanisms that can delay or prevent the glycation process in vivo, such that they inhibit AGE formation. These can be any of the following:19
(1) Free-radicals are generated during the early stage of glycation. Schiff bases are prone to oxidation, generating free-radicals and reactive carbonyl groups, and glycated protein can also catalyze the generation of free-radicals. Therefore, the generation of reactive carbonyl or dicarbonyl groups is related to oxidative metabolism. At an early stage, anti-glycation strategies involve scavenging hydroxyl radicals and superoxide radicals to alleviate oxidative stress and reducing the generation of reactive carbonyl or dicarbonyl groups. Thus, these strategies inhibit glycation.
(2) During the glycation process, blocking the carbonyl or dicarbonyl groups in reducing sugars, Schiff bases or Amadori products can inhibit glycation.
(3) AGE formation is related to the presence of transition metal ions. Therefore, metal ion chelation might also inhibit AGE formation.
(4) Inhibiting the formation of late-stage Amadori products.
(5) Breaking the crosslinking structures in the formed AGEs can inhibit glycation.
(6) Blocking RAGEs can repress the occurrence of oxidative stress and inflammation in biological systems, which is a strategy of inhibiting glycation at a later stage.
Among the anti-glycation mechanisms described above, the first four mechanisms are found in the anti-glycation properties of natural foodstuffs, whereas the other two are usually part of suppression strategies employed by pharmacological drugs. Many antioxidants can protect against free-radicals generated during glycation; metal chelators can prevent reducing sugars and Amadori products from self-oxidation, leading to the inhibition of AGE formation. These antioxidant-containing foodstuffs are among the best candidates for anti-glycation foods. Several approaches to the design of AGE inhibitors are summarized in Fig. 4.
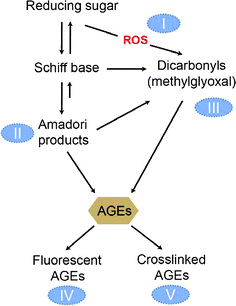 |
| Fig. 4 Antiglycation mechanisms of foodstuffs. Several approaches to the design of AGE inhibitors have been summarized as indicated by the circled numbers I–V. Type I inhibitors act to inhibit ROS formation during the glycation process, in order to prevent glycoxidative reactions. Type II inhibitors act to inhibit Amadori adduct formation, in order to prevent the initial stages of glycation. Type III inhibitors act as the RCS scavengers. Type IV and V inhibitors are likely to impact the development of AGE-specific fluorescence and the subsequent protein crosslinking, which are the advanced stages of protein glycation. | |
5. Foodstuffs as AGE inhibitors
The development and investigation of AGE inhibitors, especially natural anti-AGE agents with few side-effects, may yield a potential therapeutic approach for delaying and preventing premature aging and diabetic complications. Anti-AGE agents may act through the mechanisms of trapping carbonyl groups on reducing sugars, Amadori products and RCSs to inhibit AGE formation.20–22 Several pharmacological compounds that can cleave AGE crosslinks have been developed, which presents the possibility of reversing diabetic complications.10,19,23 Similarly, RAGE blockers have garnered considerable interest because AGEs can induce cellular disorders through interactions with RAGEs.24Antioxidants may protect against glycation-derived free-radicals and act as the chelators by removing transition metal ions, and may prevent the self-oxidation of reducing sugars, Amadori products and RCSs.
5.1 Plant extracts
5.1.1
Cinnamon
.
Cinnamon is an evergreen tree that belongs to the Lauraceae family. It is a traditional spice, and the leaves and bark are often used as additives to enhance the aroma and flavor during cooking. It possesses antioxidant, antibacterial and antipyretic properties. Whole cinnamon or aqueous extracts of cinnamon have been shown to ameliorate the symptoms of metabolic syndromes, such as insulin resistance, elevated levels of glucose and lipids, inflammation, decreased antioxidant activity, increased weight gain and increased protein glycation.25 Using the BSA-glucose assay, Peng et al.26 analyzed the anti-glycation activity of cinnamon bark extracts prepared with different solvents. They found that the ethyl acetate extracts exhibited the highest anti-glycation activity. Catechin, epicatechin and procyanidin B2, isolated from fractions of the ethyl acetate extracts, were shown to inhibit the generation of oxidative AGEs, CML and pentosidine. Procyanidin B2 can also function as an RCS scavenger to form adducts with MG, thus providing an anti-glycation mechanism similar to that of AG.27
5.1.2 Garlic (Allium sativum).
Garlic is known to play a role in delaying aging-related diseases and preventing cardiovascular diseases. It can reduce the levels of cholesterol and triglyceride, prevent hypertension and inhibit platelet aggregation and low-density lipoprotein (LDL) oxidation. S-Allylcysteine in garlic extracts is a strong antioxidant and free-radical scavenger that can increase the in vivo activity of antioxidative enzymes, such as catalase, superoxide dismutase and glutathione peroxidase. In vitro studies have shown that aged garlic extracts can inhibit the formation of AGEs (such as CML) and that S-allylcysteine is the major active component.28 Furthermore, in animal studies, S-ethylcysteine and S-propylcysteine were shown to reduce the physiological anti-glycation activities of plasma HbA1c and renal CML.29 In an in vitro model, six types of organic sulfur compounds, isolated from garlic extracts, were shown to antagonize LDL glycation. Among these, the cysteine-containing compounds, including S-allylcysteine, S-ethylcysteine, S-methylcysteine and S-propylcysteine, exhibited higher anti-glycation activity than diallyl sulfide and diallyl disulfide.30 Although garlic extracts have been shown to exert in vivoantioxidant and hypoglycemic activities in STZ-induced diabetic rats,31 further in vivo verification studies are required to assess whether aged garlic extracts can protect against the harmful effects of glycation.
5.1.3
Ilex paraguariensis
.
I. paraguariensis, also known as yerba maté or just maté, originates from the central region of South America. The most remarkable property of I. paraguariensis is its unparalleled levels of nutritional ingredients. Many studies have revealed that it is not only rich in vitamins and minerals, but maté tea leaves also contain over three times more polyphenols, including catechin and flavonoids, than green tea; therefore, it contains high levels of antioxidants.32 Currently, millions of people in South America drink maté tea on a daily basis, and this traditional beverage has spread to major cities around the world; in the last few years, it has gained popularity in the United States. Lunceford and Gugliucci33 showed that I. paraguariensis extracts exhibit a stronger anti-glycation activity than green tea extracts in an in vitro system of MG-induced BSA glycation. Therefore, the effect is mainly due to an inhibition of the second phase of the glycation reaction, namely the free-radical-mediated conversion of Amadori products to AGEs. Caffeic acid and chlorogenic acid are likely the major active components of the anti-glycation activity in I. paraguariensis extracts.34
5.1.4 Rosemary (Rosmarinus officinalis).
R. officinalis is a small aromatic shrub from the Mediterranean region. Its slender leaves have a sweet fragrance and are enriched with essential oil components. The leaves are frequently used to enhance food flavors. The main constituents of rosemary extracts are carnosic acid and carnosol. Kim and Kim35 first discovered the anti-glycation activity of rosemary extracts, and this may be closely related to their antioxidant activity. Hsieh et al.36 further investigated the possible protective role of the anti-glycation activity of R. officinalis in cardiovascular diseases and neurodegenerative disorders. In the model system of collagen glycation by methylglyoxal, rosemary extracts exerted an anti-glycation effect equivalent to that of AG. They also showed an inhibitory effect on the LDL oxidation and glycation induced by glucose and RCSs. Dearlove et al.37 further demonstrated a comparable level of anti-glycation activity in rosemary among multiple culinary herbs and spices. These findings suggest that rosemary is an excellent multifunctional therapeutic herb because of its potential potent anti-glycation activity.
5.1.5 Tea (Camellia sinensis).
Green tea has a long history of being a popular beverage worldwide. Green tea contains large quantities of tannins (flavonoids), and, therefore exhibits excellent antioxidant activity. A study by Nakagawa et al.38 showed that the anti-glycation activity of the aqueous extracts of green tea may be due to tea polyphenols, such as (−)-epigallocatechin 3-O-gallate (EGCG) and (−)-epicatechin 3-O-gallate (ECG). Animal studies have demonstrated that the administration of green tea extracts for fourteen consecutive weeks inhibited the formation of collagen crosslinking and fluorescent products induced by aging in C57BL/6 mice.39 The administration of green tea extracts (300 mg per kg body weight) for four weeks improved the solubility of tail-tendon collagen and reduced AGE formation in STZ-induced diabetic rats.40,41 Another report indicated that the supplementation of 1.25% green tea or black tea extracts in drinking water can delay cataract formation in diabetic rats.42 Matcha, a powdered green tea, has been found to inhibit the accumulation of CML and CEL, as well as the activation of RAGE, in the kidney of type 2 diabetic OLETF rats.43 Taken together, these findings suggest that tea may be a functional beverage that can improve diabetes complications.
5.1.6 Tomato paste.
Tomato paste is a common, healthy, processed food that contains lycopene, an important carotenoid, and water-soluble polyphenol. Kiho et al.44 found that a water-soluble and low-molecular-weight fraction of tomato paste showed a strong capability to inhibit glycation by immunofluorescence, ELISA and western blot analyses using anti-AGE antibodies. In comparison, the rutin and tomato paste fractions of the water-soluble tomato paste extracts exhibited 75% and 50% inhibition of glycation, respectively, whereas AG (1 mM) only showed 55%.
5.1.7 Herb extracts.
Some Chinese herbal medicines have shown therapeutic potential in the treatment of diabetes and its complications. In recent years, saponins have been reported to exert inhibitory effects on lipid peroxidation, protecting vascular endothelial cells from diabetes complications and reducing the levels of blood glucose and triglycerides. The Chinese herbal medicines used for diabetes treatment are usually rich in saponins. Xi et al.45 evaluated the antioxidant and anti-glycation properties of 11 Chinese herbal medicine extracts. Among these herbal medicines, Acanthopanax senticosus (sometimes known, misleadingly, as Siberian ginseng), Aralia taibaiensis and Panax ginseng (Asian ginseng) contained the highest levels of saponins. Using the percentage of glycated hemoglobin as an indicator for the inhibition of early-stage glycation, Acanthopanax senticosus, Aralia taibaiensis and Poria cocos showed the best results; their anti-glycation activities were even higher than AG. A. senticosus and A. taibaiensis displayed an inhibitory effect similar to AG in the BSA-glucose assay that examined the inhibition of AGE formation. A. senticosus and A. taibaiensis also showed the strongest inhibitory effect on protein crosslinking in the G.K. peptide-ribose assay. Interestingly, Chinese herbal medicines with high antioxidant activity do not necessarily exhibit high anti-glycation activity.
In East Asia, Plantago asiatica, commonly known as the Chinese plantain, has been used as a medicinal herb. Its main chemical components include platagin, plantamajoside and aucubin. Recent studies indicated that it has antipyretic, antitussive and wound-healing effects.46P. asiatica extracts also showed a glycation inhibition rate of 50% at 1 μg mL−1in vitro. Its major active component, plantamajoside, displayed a better anti-glycation effect than AG at the same dose and showed no toxicity after 90 days of oral administration in a safety study in rats.47
Corn silk is used as a diuretic in traditional Chinese herbal medicine to prevent hypertension. It contains polyphenolic compounds, such as luteolin and maysin. Maysin acts as a natural insecticide within corn silk. Therefore, the newly cultivated pest-resistant maize varieties have relatively high maysin contents in their silk. Farsi et al.48 studied the anti-glycation properties of pest-resistant maize varieties and found that the maize genotype CO441-1 showed an IC50 for anti-glycation higher than AG (9.5 μg mL−1vs. 44.4 μg mL−1). They also discovered that the anti-glycation activity is positively correlated with the total polyphenol content. Suzuki et al.49 isolated 16 components from corn silk, and of these, alternanthin, chrysoeriol 6-C-β-fucopyranoside, genistein and guanosine showed inhibitory activity against glycation.
Luobuma tea (Apocynum venetum) and its seven polyphenol components, including (±)-gallocatechin (GC), (−)-epigallocatechin (EGC), (±)-catechin, (−)-epicatechin (EC), epicatechin-(4β–8)-gallocatechin, epigallocatechin-(4β–8)-epicatechin and procyanidin B2, have been shown to exhibit anti-glycation activity.50 Dearlove et al.37 analyzed 24 commonly used culinary herbs and spices, and demonstrated that spice extracts generally exhibited higher levels of anti-glycation activity than herb extracts. Furthermore, this activity was significantly correlated with the total phenolic content (R2 = 0.89). Among the tested spice extracts, the most potent glycation inhibitors were the extracts of cloves, ground Jamaican allspice and cinnamon; among the tested herb extracts, the potent glycation inhibitors were sage, marjoram, tarragon and rosemary.
5.1.8 Other extracts.
The dried pericarp of Garcinia indica (commonly known as kokum) has been traditionally used in curry dishes and folk medicine. It contains 2–3% garcinol, which has antioxidant activity. Garcinol has two-thirds the chelating ability of EDTA, and the ability is similar to citrates. Its DPPH radical-scavenging capability is slightly lower than catechin and is approximately 85% of that of vitamin C. It is a scavenger of lipid-soluble superoxide anions. Garcinol showed a better anti-glycation effect than quercetin and AG at both 0.01 mM and 0.1 mM.51
Extracts from leaves or fruits of Ginkgo biloba trees have been used medicinally for thousands of years. EGb761, a defined extract prepared from the leaves of G. biloba containing 24% flavonoids and 6% terpenoids, is effective for treating disorders related to oxidative stress and cognitive impairment.52 Recent studies indicate that G. biloba extracts can inhibit RAGE activation in microvascular endothelial cells induced by hypoxic and hypoglycemic conditions.53 In addition, G. biloba extracts have been shown to have protective effects against AGE-induced renal fibrosis and oxidative stress in NRK-49F cells54 and against apoptosis in neuronal cells.55
2,3,5,4′-Tetrahydroxystilbene 2-O-β-D-glucoside, the major bioactive compound isolated from Polygonum multiflorum, can also efficiently inhibit the formation of AGEs by trapping reactive MG under physiological conditions.56 The two unsubstituted carbons at positions 4 and 6 on the A-ring appeared to be the major active sites for stilbene-type compounds to trap reactive dicarbonyl species.
5.2
Food products and food bioactive compounds
5.2.1
Carnosine
.
Carnosine is a dipeptide compound (β-alanyl-L-histidine) that is ubiquitous in large amounts in animal tissues, especially in the brain and muscles where its concentration can reach millimolar levels.57 However, its content has been shown to decrease with age.58 A large number of studies have demonstrated that diabetes results in reduced levels of carnosine. For example, diabetic patients have a significantly lower carnosine level in their red blood cells, compared to healthy individuals.59 Similarly, diabetic rats also exhibit a lower carnosine level than normal rats.60 Experimental evidence has demonstrated that carnosine can inhibit LDL glycation, prevent the formation of foam cells and impede the progression of atherosclerotic plaque.61 Multiple in vitro studies have shown that carnosine can inhibit protein carbonyl formation and protein crosslinking caused by reducing sugars and other reactive aldehydes (such as malondialdehyde and MG).57,62Carnosine may also serve as an anti-glycation agent by acting as a dicarbonyl scavenger.63 Hipkiss et al.57 indicated that carnosine itself is not mutagenic even after it is glycated. It can inhibit glycation in the model system consisting of acetyl-Lys-His-amide and dihydroxyacetone and can protect SOD, catalase and α-crystallin from being crosslinked by reducing sugar molecules. The immobilization of carnosine in human leg muscle tissues may be related to adduct formation through the interaction between carnosine and carbonyl groups.64 Plasma AGE levels in vegetarians have been shown to be higher than those in omnivores,65 which may be related to a large amount of fructose in the vegetarian diet66 or insufficient carnosine intake.67 Lee et al.68 showed that carnosine supplementation can delay the progression of diabetes in mice and protect human LDL from oxidation and glycation. Carnosine and its derivative acetyl-carnosine have been shown to inhibit the pathogenesis of cataracts that result from aging and glycation in humans.69
5.2.2
Curcumin
.
Curcuma
longa has a long history in traditional medicine and has been widely used as a Chinese medicinal herb. Its stems are frequently used as an anti-inflammatory medicine. Its roots are often ground into a golden powder to be used in food, and this powder is commonly known as turmeric. A thousand years ago, Indians used the dry powder of turmeric roots and stems as a food ingredient (curry) for flavoring, food preservation and yellow food coloring. To date, no significant toxic side-effects of C. longa have been discovered in its use in Chinese medicine.70 Animal studies have demonstrated that turmeric has anti-oxidative, anti-inflammatory, anti-cancer and hepatoprotective functions.71 There is a health care trend concerning the use of foods that can cure disease, and turmeric is an example of a healthy food with therapeutic effects that have been supported by modern medical studies.
Curcumin (diferuloylmethane) is an orange-yellow component of C. longa. Previous studies have shown that curcumin can reduce the blood glucose level in type 1 and type 2 diabetic mice and can improve the metabolic balance in the body. Furthermore, the activity of sorbitol dehydrogenase, which catalyzes the conversion of sorbitol to fructose, has been shown to be significantly reduced by curcumin treatment.72 Sajithlal et al.73 showed that the accelerated accumulation of AGE-collagen in diabetic animals, detected by ELISA, was prevented by curcumin. Extensive cross-linking of collagen in the tail tendon and skin of diabetic animals was also prevented to a greater extent by curcumin treatment. Furthermore, curcumin prevents streptozotocin-induced islet damage by scavenging free-radicals.74Tetrahydrocurcumin, one of the major metabolites of curcumin, significantly reduced the accumulation and crosslinking of collagen in streptozotocin- and nicotinamide-induced diabetic rats.75
Recently, Jain et al.76 demonstrated that curcumin supplementation reduces the blood levels of inflammatory cytokines, glucose and glycosylated hemoglobin in diabetic rats. In a background study, a series of classical polyphenol antioxidants, including 10 flavonoids,14,77,78 12 phenolic acids,79,80curcumin and silymarin,81 were screened in vitro to evaluate their effects on protein glycation, crosslinking and AGE formation. Of particular note, two of these polyphenolic compounds showed consistent inhibitory effects on AGE formation in experimental animals: one of these is curcumin, which has been proposed as an efficient AGE inhibitor as mentioned above.81 This finding suggests a potential role of curcumin in anti-glycotoxin therapy.
5.2.3
Flavonoids
.
Flavonoids are present in various types of vegetables, tea and red wine. The primary structure of flavonoids is three benzene rings with one or more hydroxyl groups. This structure is also the key factor that determines their anti-oxidant activity. The anti-glycation effects of naturally occurring flavonoids can be partly attributed to their antioxidant properties. Wu et al.14 conducted a study using several established in vitro screening models that could uniquely distinguish specific inhibitors of the early-stage (Amadori products), intermediate-stage (RCS) and last-stage of glycation (AGE formation and cross-linking). Ten flavonoids were investigated, including flavanols (catechin, EC, ECG, EGC and EGCG), a flavone (luteolin), flavonols (kaempferol, quercetin and rutin) and a flavanone (naringenin). At the early stage of protein glycation, luteolin, quercetin and rutin exhibited significant inhibitory effects on HbA1C formation, and they were more effective than AG (10 mM). At the intermediate stage, luteolin and rutin showed more significant inhibitory effects on MG-medicated protein modification; the IC50 values were 66.1 and 71.8 μM, respectively. At the last stage of glycation, luteolin was found to be a potent inhibitor of both AGE formation and subsequent protein crosslinking.
The inhibitory effects of the tested flavonoids on AGE formation approximately followed the order of flavone > flavonols > flavanols > flavanone, with a few exceptions (ECG and EGCG). Structure–activity relationship analysis suggested that the hydroxyl group at the C-3 position contributed to the inhibitory effects on AGE formation of these compounds. Similar to flavanols, the presence of the hydroxyl group at the C-5 and C-3 positions in the B ring affects their inhibitory effects against glycoxidation. These results are in agreement with another study, suggesting that the single hydroxyl group at C-5 position of the B ring is involved in glycation inhibition.38,82 Electron spin resonance has shown that protein glycation is accompanied by oxidative reactions, and the inhibitory effects of flavonoids on protein glycation are significantly related to the scavenging of free-radicals derived from the glycoxidation process.
Daflon 500, a clinical drug consisting of flavonoids, reduced HbA1C and protein glycation in a group of 28 Type 1 diabetic patients.83 The administration of rutin (1 g kg−1 day−1) decreased collagen-linked fluorescence in the skin of diabetic rats.84 The rutin metabolites 3,4-dihydroxyphenylacetic acid and 3,4-dihydroxytoluene were recently demonstrated to be potential novel inhibitors of non-oxidative AGEs.85 Phytoestrogenic isoflavonoids, such as daidzein and genistein, have been shown to interfere with AGE-mediated oxidative DNA damage in hypertensive rats, which appeared to be attributed to the direct scavenging of AGE-derived radicals.86 Our recent studies have shown that introducing the flavonoids, EGCG or rutin, into LDL particles can protect the lipoproteins against glycotoxin-mediated adverse effects.77,79 Also, Ho and coworkers20,21,87 have demonstrated that reactive dicarbonyl species, such as methylglyoxal and glyoxal, can be trapped by EGCG and genistein. Recently, Suzuki et al.88 isolated two flavoneC-glycosides from the style of Zea mays that exhibited an in vitro inhibitory effect on glycation (53–64%), which was similar to AG at 1 mM.
In fact, the anti-glycation activities of the food ingredients discussed above are mostly due to their richness in antioxidant components: luteolin is mainly derived from the plants Ajuga decumbus, Dracocephalum integrifolium and Lonicera japonica; rutin is present in common buckwheat, the leaves and stems of the plants in the genus Rheum and the fruits of the fava d'anta tree (originating from Brazil); and daidzein and genistein are mainly from soybeans.
5.2.4 Phenolic acids.
Phenolic acids, especially hydroxybenzoic and hydroxycinnamic acids, are secondary plant products that are commonly found in plant-derived foodstuffs at higher concentrations than polyphenolic flavonoids.89 Supplementation of 5% caffeic acid or ellagic acid in the diet for 12 consecutive weeks reduced the levels of plasma HbA1c, urinary glycated albumin and renal CML and the accumulation of pentosidine, sorbitol and fructose in diabetic mice.90 Recent results suggest that vanillic acid can inhibit neuron cell apoptosis in response to an RCS challenge, suggesting that phenolics might possess cytoprotective properties in the prevention of diabetic neuropathy complications.91Vanillic acid is also a moderate AGE inhibitor because it blocks MG-mediated intracellular glycation.92
We previously screened the effects of dietary phenolic acids on individual stages of protein glycation.79 Phenolic acids, especially methoxyphenolic acids, were potent inhibitors at multiple stages of glycation, including the early stage, evidenced by the decrease of HbA1c levels shown in the δ-Glu assay. Chlorogenic acid, ferulic acid, vanillic acid, sinapic acid and syringic acid significantly inhibited post-Amadori glycation and protein crosslinking. However, no direct structure–activity relationship has been established to explain the anti-glycation activity that was shown in the assays above. A possible mechanism by which phenolic acids inhibit protein glycation could be related to their antioxidant properties.
5.2.5
Silymarin
.
The flavonoid blend silymarin is an extract from milk thistle (Silybum marianum), and it is mainly composed of silybin A and B, isosilibybin A and B, silychristin and silydianin. These flavonolignans are well known for displaying a remarkable spectrum of biological activities, including antioxidant, cytoprotective and anticarcinogenic activities.93 In addition to its free-radical scavenging properties, silymarin has been clinically used for its beneficial effects on various hepatic diseases, where the pathogenesis involves an inflammatory response. Wu et al.81 evaluated the effects of silymarin on AGE formation in vitro and in vivo. The in vivo anti-glycation activity was examined by a 12-week administration of silymarin in STZ-diabetic rats. In vitro glycation assays revealed that silymarin can exert marked inhibitory effects on the late-stage glycation and subsequent crosslinking. Dual action mechanisms, namely antioxidant and reactive carbonyl trapping activities, might contribute to this anti-glycation effect. Silymarin induced a significant decrease in the level of monocytic IL-1β and COX-2 and prevented oxidant formation caused by S100b, which appeared to be mediated by inhibiting the membrane translocation of p47phox. In vivo, silymarin reduced tissue accumulation of AGEs, crosslinking of tail collagen and the concentration of plasma glycated albumin. Levels of oxidative and inflammatory biomarkers also significantly decreased in silymarin-treated groups, compared to those in the diabetic groups. These new findings indicate that silymarin supplementation may reduce AGE accumulation in diabetes and can possibly inhibit resulting complications.
5.2.6
Terpenes
.
The crude extract ‘Astragali Radix’ inhibits the formation of CML and pentosidine during the incubation of BSA with ribose. Research has shown that a cycloartane-type triterpenoid compound, astragaloside V, may be its major active component for anti-glycation.94 A compound that was recently isolated from Panax ginseng (Asian ginseng), 20(S)-ginsenoside Rg(3), exhibited activities that decreased the levels of blood glucose, glycosylated protein and lipid peroxide in animal studies.95 Similarly, heat-processed American ginseng also showed inhibitory effects on renal CML accumulation and RAGE activation in diabetic rats.96
Oleanolic acid and ursolic acid are naturally occurring triterpenoids that have been used in traditional medicine for centuries as antibacterial, antifungal and anti-inflammatory agents.97 Recently, much interest has been focused on the anticancer properties of these triterpenoids.98,99 Previously, Wang et al.100 showed for the first time that oleanolic acid and ursolic acid intake at 0.1 or 0.2% reduced the levels of renal CML, urinary glycated albumin and urinary albumin in diabetic mice. Simultaneously, another study indicated that ursolic acid may play a significant role in diabetes mellitus by alleviating hyperglycemia, hepatic glucose production, hyperlipidemia and the influx of glucose through the polyol pathway.101 These findings suggest that supplementation of oleanolic acid and ursolic acid may be helpful for preventing or alleviating glycation-associated renal diseases.
5.2.7
Vitamins
.
Typical antioxidants, such as vitamin E102 and lipoic acid,103 have proven to be effective in delaying the peroxidation of LDL and membrane lipids. Both in vivo and in vitro experiments showed that vitamin E supplementation reduced the level of serum albumin glycation in diabetic patients and suppressed in vivoAGE formation in rats.104,105Lipoic acid can alleviate complications in diabetic patients, such as cataracts, vascular injuries and polyneuritis; it can also improve vasodilation dysfunction and provide unique health benefits by maintaining the balance between the antioxidant defense system and oxidative stresses.103 Animal studies have shown that oral administration of vitamin C (1000 mg day−1) for four consecutive weeks reduced the level of in vivoprotein glycation by 46.8%.106 Tanaka et al.107 demonstrated that glucose-induced cytotoxicity in HIT-T15 β cells was related to the level of ROS production, and the addition of N-acetylcysteine significantly reduced the formation of serum 8-OH-dG and MDA in Zucker diabetic rats. Vinson and Howard106 investigated the roles of various nutrients in glycation and AGE formation; the results showed that vitamin C, niacinamide and other nutrients displayed an anti-AGE effect at physiological concentrations. Similar studies, such as those on thiamine pyrophosphate and pyridoxamine, found that the nutrients studied were more effective in inhibiting AGE formation, compared to AG, in preliminary in vitro experiments.10,108
5.2.8
Food ingredients with angiotensin-converting enzyme inhibitor (ACEI) activity.
Recent studies have shown that an angiotensin-converting enzyme inhibitor (ACEI), a hypertension drug, can delay the progression of cardiovascular diseases and the development of nephropathy in diabetic patients, promote thrombolysis and reduce AGE formation.109 Clinical studies have also shown that an ACEI can increase the soluble RAGE (sRAGE) level in the plasma of type I diabetic patients and reduce AGE accumulation.110 In STZ-induced diabetic rats, a 24-week administration of ramipril not only reduced the skin collagen crosslinking products, CML and pentosidine, but also decreased the AGE levels in the blood and kidneys. In recent years, many peptides with ACEI activity have been isolated from a variety of foodstuffs, including bovine casein, skipjack tuna, sake, soy sauce and livestock and poultry muscle.111 Although no experimental evidence has confirmed the anti-glycation activity in these food-derived peptides with ACEI activity, these ingredients with ACEI activity may play important roles in anti-glycation.
6. Other putative anti-glycation agents
The first anti-glycation drug was aspirin, which competes with sugar in acetylating the amino terminus of a protein, reducing the amounts of AGEs.112 However, aspirin must be administered for a long period and at high doses, leading to increased side-effects that make it unsuitable for glycation prevention.
Later, Brownlee and others found that AG can prevent glucose-induced intermolecular collagen crosslinking and the production of fluorescent substances in diabetic animal models.113 Nucleophilic hydrazine compounds, namely AG, are effective inhibitors for preventing AGE-induced crosslinking and fluorescent substance production. The underlying mechanism for this effect is that AG can capture the RCSs generated in the Maillard reaction, such as 1-alkylamino-1 and 4-dideoxyosone, which terminates the reaction.114 Animal experiments have shown that AG can prevent diabetic vasculopathy. However, clinical trials of AG that aimed to prevent the progression of type I115 and type II116 diabetic nephropathy were terminated due to safety concerns.
Currently, sulfite is the most promising compound for inhibiting the Maillard reaction because it can inhibit the production of furan derivatives by turning reducing sugars into non-reducing sugars that do not react with proteins. Thus, it prevents the progression of the Maillard reaction.117
The oxidation and crosslinking of sugars can be inhibited by diethylenetriaminepentaacetic acid (DTPA) as a result of chelating transition metal ions. Transition metal ions, such as copper and iron, are catalysts for oxygen free-radical reactions. The inhibitory effects of DTPA are mostly due to its effects on autoxidative glycosylation of proteins; in other words, these effects are due to the self-oxidative decomposition of glucose and the subsequent reactions between proteins and the pyrolysis products of glucose, rather than the glycation-mediated inhibition of glucose itself through Amadori product rearrangement. The inhibitory activity of Tiron (1,2-dihydroxy-3,5-benzenedisulfonic acid) may be related to both the chelation and the antioxidant activities.118
OPB-9195 (2-isopropylidenehydrazono-4-oxo-thiazolidine-5-ylacetanilide) is a novel glycosylation inhibitor that is a thiazolidine derivative. In vitro, the inhibitory effects of OPB-9195 on AGE formation and AGE-derived crosslinking can be detected by ELISA and SDS-PAGE, respectively.119 OPB-9195 displays a better inhibitory effect on AGE formation than AG even at very low doses, effectively preventing the progression of nephropathy in diabetic patients.120
Currently, anti-AGE compounds are being developed with completely different chemical structures from AG, such as pyridoxamine,1212,3-diaminophenazine,122ALT-711 (Alagebrium);123 LR-90124 and others.
7. Concluding remarks
AGE accumulation in vivo has been implicated as a major factor in the pathogenesis of diabetic complications and other health disorders. Clinical evidence and experimental data have shown that AGE levels are particularly high in diabetic patients, and elevated levels of blood sugar accelerate protein glycation and facilitate AGE formation. Furthermore, hyperglycemia or hyperglycemia-associated glycotoxins have been shown to play a crucial role in chronic inflammatory processes that can cause cells to emit signals that trigger an overproduction of ROS and inflammatory mediators through both RAGE-dependent and RAGE-independent pathways. However, the clinical trials of several anti-AGE drugs to prevent the progression of type I and type II diabetic nephropathy were terminated due to safety concerns. It is hoped that the development and investigation of AGE inhibitors, especially natural anti-AGE agents with fewer side-effects, may provide a therapeutic approach for delaying and preventing premature aging and diabetic complications.
8. Abbreviations
Acknowledgements
This research work was supported by the National Science Council (NSC94-2321-B005-001, NSC96-2628-B005-004-MY3 and NSC 99-2628-B-005-002-MY3), Taiwan, Republic of China.
References
- L. C. Maillard, C. R. Hebd. Seances Acad. Sci., 1912, 154, 66 Search PubMed.
- J. O'Brien and P. A. Morrissey, Crit. Rev. Food Sci. Nutr., 1989, 29, 273.
- V. Somoza, Mol. Nutr. Food Res., 2005, 49, 663 CrossRef CAS.
- J. M. Ames, Mol. Nutr. Food Res., 2007, 51, 1085 CrossRef CAS.
- A. Negre-Salvayre, R. Salvayre, N. Augé, R. Pamplona and M. Portero-Otin, Antioxid. Redox Signal., 2009, 11.
- R. J. Koenig, C. M. Peterson, R. L. Jones, C. Saudek, M. Lehrman and A. Cerami, N. Engl. J. Med., 1976, 295, 417 CrossRef CAS.
- D. Bonnefont-Rousselot, Curr. Opin. Clin. Nutr. Metab. Care, 2002, 5, 561 CrossRef CAS.
- S. P. Wolff and R. T. Dean, Biochem. J., 1987, 245, 243 CAS.
- D. Bonnefont-Rousselot, J. Soc. Biol., 2001, 195, 391 Search PubMed.
- R. G. Khalifah, J. W. Baynes and B. G. Hudson, Biochem. Biophys. Res. Commun., 1999, 257, 251 CrossRef CAS.
- J. Montonen, P. Knekt, R. Jarvinen and A. Reunanen, Diabetes Care, 2004, 27, 362 CrossRef CAS.
- R. Amarowicz, R. Carle, G. Dongowski, A. Durazzo, R. Galensa, D. Kammerer, G. Maiani and M. K. Piskula, Mol. Nutr. Food Res., 2009, 53, S151 CrossRef.
- S. Martinez-Florez, J. Gonzalez-Gallego, J. M. Culebras and M. J. Tunon, Nutr. Hosp., 2002, 17, 271 Search PubMed.
- C. H. Wu and G. C., J. Agric. Food Chem., 2005, 53, 3167 CrossRef CAS.
- N. V. Chuyen, Mol. Nutr. Food Res., 2006, 50, 1140 CrossRef.
- R. Ramasamy, S. F. Yan and A. M. Schmidt, Mol. Nutr. Food Res., 2007, 51, 1111 CrossRef CAS.
- R. Nagai, Y. Fujiwara, K. Mera and Otagiri, Mol. Nutr. Food Res., 2007, 51, 462 CrossRef CAS.
- G. Basta, A. M. Schmidt and R. De Caterina, Cardiovasc. Res., 2004, 63, 582 CrossRef CAS.
- S. J. Zieman and D. A. Kass, Drugs, 2004, 64, 459 CrossRef CAS.
- C. Y. Lo, S. Li, D. Tan, M. H. Pan, S. Sang and C. T. Ho, Mol. Nutr. Food Res., 2006, 50, 1118 CrossRef CAS.
- S. Sang, X. Shao, N. Bai, C. Y. Lo, C. S. Yang and C. T. Ho, Chem. Res. Toxicol., 2007, 20, 1862 CrossRef CAS.
- D. Tan, Y. Wang, C. Y. Lo and C. T. Ho, Asia Pac. J. Clin. Nutr., 2008, 17, 261 CAS.
- J. Peyroux and M. Sternberg, Pathol. Biol., 2006, 54, 405 CrossRef CAS.
- C. W. Heizmann, Mol. Nutr. Food Res., 2007, 51, 1116 CrossRef CAS.
- B. Qin, K. S. Panickar and R. A. Anderson, J. Diabetes Sci. Technol., 2010, 4, 685 Search PubMed.
- X. Peng, K. W. Cheng, J. Ma, B. Chen, C. T. Ho, C. Lo, F. Chen and M. Wang, J. Agric. Food Chem., 2008, 56, 1907 CrossRef CAS.
- X. Peng, J. Ma, J. Chao, Z. Sun, R. C. C. Chang, I. Tse, E. T. S. Lit, F. Chen and M. Wang, J. Agric. Food Chem., 2010, 58, 6692 CrossRef CAS.
- M. S. Ahmad and N. Ahmed, J. Nutr., 2006, 136, 796S CAS.
- C. C. Lin and M. C. Yin, Mol. Nutr. Food Res., 2008, 52, 1358 CrossRef CAS.
- C. N. Huang, J. S. Horng and M. C. Yin, J. Agric. Food Chem., 2004, 52, 3674 CrossRef CAS.
- V. Rajani Kanth, P. Uma Maheswara Reddy and T. N. Raju, Acta Diabetol. Lat., 2008, 45, 243 Search PubMed.
- C. I. Heck and E. G. de Mejia, J. Food Sci., 2007, 72, 138.
- N. Lunceford and A. Gugliucci, Fitoterapia, 2005, 76, 419 CrossRef.
- A. Gugliucci, D. H. Bastos, J. Schulze and M. F. Souza, Fitoterapia, 2009, 80, 339 CrossRef CAS.
- H. Y. Kim and K. Kim, J. Agric. Food Chem., 2003, 51, 1586 CrossRef CAS.
- C. L. Hsieh, C. H. Peng, C. C. Chyau, Y. C. Lin, H. E. Wang and R. Y. Peng, J. Agric. Food Chem., 2007, 55, 2884 CrossRef CAS.
- R. P. Dearlove, P. Greenspan, D. K. Hartle, R. B. Swanson and J. L. Hargrove, J. Med. Food, 2008, 11, 275 CrossRef CAS.
- T. Nakagawa, T. Yokozawa, K. Terasawa, S. Shu and L. R. Juneja, J. Agric. Food Chem., 2002, 50, 2418 CrossRef CAS.
- R. Kathryn, S. David, R, F. Nalani, O. Mark, Z. Michael, S. R. Pamela and M. Vincent, Int. J. Vitam. Nutr. Res., 2003, 73, 453 CrossRef.
- P. V. Babu, K. E. Sabitha and C. S. Shyamaladevi, Chem.-Biol. Interact., 2006, 162, 157 CrossRef CAS.
- P. V. Babu, K. E. Sabitha and C. S. Shyamaladevi, Food Chem. Toxicol., 2008, 46, 280–285 CrossRef CAS.
- J. A. Vinson and J. Zhang, J. Agric. Food Chem., 2005, 53, 3710 CrossRef CAS.
- N. Yamabe, K. S. Kang, J. M. Hur and T. Yokozawa, J. Med. Food, 2009, 12, 714 CrossRef CAS.
- T. Kiho, S. Usui, K. Hirano, K. Aizawa and T. Inakuma, Biosci., Biotechnol., Biochem., 2004, 68, 200 CrossRef CAS.
- M. Xi, C. Hai, H. Tang, M. Chen, K. Feng and X. Liang, Phytother. Res., 2008, 22, 228 CrossRef CAS.
- S. Nishibe, Yakugaku Zasshi, 2002, 122, 363 CrossRef CAS.
- S. Y. Choi, S. H. Jung, H. S. Lee, K. W. Park, B. S. Yun and K. W. Lee, Phytother. Res., 2008, 22, 323 CrossRef CAS.
- D. A. Farsi, C. S. Harris, L. Reid, S. A. Bennett, P. S. Haddad, L. C. Martineau and J. T. Arnason, Phytother. Res., 2008, 22, 108 CrossRef CAS.
- R. Suzuki, M. Iijima, Y. Okada and T. Okuyama, Chem. Pharm. Bull., 2007, 55, 153 CrossRef CAS.
- T. Yokozawa and T. Nakagawa, Food Chem. Toxicol., 2004, 42, 975 CrossRef CAS.
- F. Yamaguchi, T. Ariga, Y. Yoshimura and H. Nakazawa, J. Agric. Food Chem., 2000, 48, 180 CrossRef CAS.
- S. Mahadevan and Y. Park, J. Food Sci., 2008, 73, 14.
- F. L. Yan, Y. Zheng and F. D. Zhao, Acta Neuropathol., 2008, 116, 529 CrossRef.
- H. D. Yan, X. Z. Li, J. M. Xie and M. Li, Chin. Med. J., 2007, 120, 787 Search PubMed.
- M. Nitti, C. d'Abramo, N. Traverso, D. Verzola, G. Garibotto, A. Poggi, P. Odetti, D. Cottalasso, U. M. Marinari, M. A. Pronzato and C. Domenicotti, Free Radical Biol. Med., 2005, 38, 846 CrossRef CAS.
- L. Lv, X. Shao, L. Wang, D. Huang, C. T. Ho and S. Sang, J. Agric. Food Chem., 2010, 58, 2239 CrossRef CAS.
- A. R. Hipkiss, J. Michaelis and P. Syrris, FEBS Lett., 1995, 371, 81 CrossRef CAS.
- H. J. Stuerenburg, Biochemistry, 2000, 65, 862 CAS.
- E. Gayova, I. Kron, K. Suchozova, V. Pavlisak, M. Fedurco and B. Novakova, Bratisl. Lek. Listy., 1999, 100, 500 Search PubMed.
- N. Katsuya, N. Akira, Y. Toshihiko, O. Hiroto, O. Nobuaki, T. Nobuo, N. Masaaki and K. Yoshinobu, Exp. Biol. Med., 2003, 228, 1138 Search PubMed.
- I. Rashid, D. M. van Reykb and M. J. Daviesa, FEBS Lett., 2007, 581, 1067 CrossRef CAS.
- C. Brownson and A. R. Hipkiss, Free Radical Biol. Med., 2000, 28, 1564 CrossRef CAS.
- D. L. Price, P. M. Rhett, S. R. Thorpe and J. W. Baynes, J. Biol. Chem., 2001, 276, 48967 CrossRef CAS.
- L. Schroder, C. Schmitz and P. Bachert, J. Magn. Reson., 2004, 171, 213 CrossRef.
- G. Suji and S. Sivakami, Biogerontology, 2004, 5, 365 CrossRef CAS.
- M. Krajcovicova-Kudlackova, K. Sebekova, R. Schinzel and J. Klvanova, Physiol. Res., 2002, 51, 313 Search PubMed.
- A. R. Hipkiss, Mech. Ageing Dev., 2005, 126, 1034 CrossRef CAS.
- Y. T. Lee, C. C. Hsu, M. H. Lin, K. S. Liu and M. C. Yin, Eur. J. Pharmacol., 2005, 513, 145 CrossRef CAS.
- M. A. Babizhayev and Y. E. Yegorov, Crit. Rev. Ther. Drug Carrier Syst., 2010, 27, 85 Search PubMed.
- A. L. Cheng, C. H. Hsu, J. K. Lin, M. M. Hsu, Y. F. Ho, T. S. Shen, J. Y. Ko, J. T. Lin, B. R. Lin, W. Ming-Shiang, H. S. Yu, S. H. Jee, G. S. Chen, T. M. Chen, C. A. Chen, M. K. Lai, Y. S. Pu, M. H. Pan, Y. J. Wang, C. C. Tsai and C. Y. Hsieh, Anticancer Res., 2001, 21, 2895 CAS.
- S. Bengmark, M. D. Mesa and A. Gil, Nutr. Hosp., 2009, 24, 273 Search PubMed.
- N. Arun and N. Nalini, Plant Foods Hum. Nutr., 2002, 57, 41 CrossRef CAS.
- G. B. Sajithlal, P. Chithra and G. Chandrakasan, Biochem. Pharmacol., 1998, 56, 1607 CrossRef CAS.
- K. Meghana, G. Sanjeev and B. Ramesh, Eur. J. Pharmacol., 2007, 577, 183 CrossRef CAS.
- L. Pari and P. Murugan, Fundam. Clin. Pharmacol., 2007, 21, 665 CrossRef CAS.
- S. K. Jain, J. Rains, J. Croad, B. Larson and K. Jones, Antioxid. Redox Signaling, 2009, 11, 241 Search PubMed.
- C. H. Wu, C. F. Wu, H. W. Huang, R. C. Jao and G. C. Yen, Mol. Nutr. Food Res., 2009, 53, 984 CrossRef CAS.
- C. H. Wu, J. A. Lin, W. C. Hsieh and G. C. Yen, J. Agric. Food Chem., 2009, 57, 5058 CrossRef CAS.
- C. H. Wu, C. T. Yeh, P. H. Shih and G. C. Yen, Mol. Nutr. Food Res., 2010, 54, S127 CrossRef CAS.
- C. H. Wu, C. T. Yeh and G. C. Yen, Food Chem., 2010, 121, 639 CrossRef CAS.
- C. H. Wu, S. M. Huang and G. C. Yen, Antioxid. Redox Signaling, 2010, 14, 353 Search PubMed.
- H. Matsuda, T. Wang, H. Managi and M. Yoshikawa, Bioorg. Med. Chem., 2003, 11, 5317 CrossRef CAS.
- Y. Manuel, B. Keenoy, J. Vertommen and I. De Leeuw, Nutr. Metab., 1999, 12, 256 Search PubMed.
- P. R. Odetti, A. Borgoglio, A. De Pascale, R. Rolandi and L. Adezati, Diabetes, 1990, 39, 796 CrossRef CAS.
- S. Pashikanti, D. R. de Alba, G. A. Boissonneault and D. Cervantes-Laurean, Free Radical Biol. Med., 2010, 48, 656 CrossRef CAS.
- K. Mizutani, K. Ikeda, T. Nishikata and Y. Yamori, J. Hypertens., 2000, 18, 1833 CrossRef CAS.
- L. Lv, X. Shao, H. Chen, C. T. Ho and S. Sang, Chem. Res. Toxicol., 2011 DOI:10.1021/tx100457h.
- R. Suzuki, Y. Okada and T. Okuyama, J. Nat. Prod., 2003, 66, 564 CrossRef CAS.
- R. Choudhury, S. K. Srai, E. Debnam and C. A. Rice-Evans, Free Radical Biol. Med., 1999, 27, 278 CrossRef CAS.
- C. Y. Chao, M. C. Mong, K. C. Chan and M. C. Yin, Mol. Nutr. Food Res., 2010, 54, 388 CrossRef CAS.
- S. M. Huang, H. C. Chuang, C. H. Wu and G. C. Yen, Mol. Nutr. Food Res., 2008, 52, 940–949 CrossRef CAS.
- S. M. Huang, C. L. Hsu, H. C. Chuang, P. H. Shih, C. H. Wu and G. C. Yen, NeuroToxicology, 2008, 29, 1016 CrossRef CAS.
- R. Gazák, D. Walterová and V. Kren, Curr. Med. Chem., 2007, 14, 315 CrossRef CAS.
- K. Motomura, Y. Fujiwara, N. Kiyota, K. Tsurushima, M. Takeya, T. Nohara, R. Nagai and T. Ikeda, J. Agric. Food Chem., 2009, 57, 7666 CrossRef CAS.
- K. S. Kang, N. Yamabe, H. Y. Kim, J. H. Park and T. Yokozawa, Eur. J. Pharmacol., 2008, 591, 266 CrossRef CAS.
- H. Y. Kim, K. S. Kang, N. Yamabe, R. Nagai and T. Yokozawa, J. Agric. Food Chem., 2007, 55, 8491 CrossRef CAS.
- J. Liu, J. Ethnopharmacol., 2005, 100, 92 CrossRef CAS.
- M. H. Shyu, T. C. Kao and G. C. Yen, J. Agric. Food Chem., 2010, 58, 6110 CrossRef CAS.
- C. T. Yeh, C. H Wu and G. C. Yen, Mol. Nutr. Food Res., 2010, 54, 1285 CrossRef CAS.
- Z. H. Wang, C. C. Hsu, C. N. Huang and M. C. Yin, Eur. J. Pharmacol., 2010, 628, 255 CrossRef CAS.
- S. M. Jang, M. J. Kim, M. S. Choi, E. Y. Kwon and M. K. Lee, Metab., Clin. Exp., 2010, 59, 512 CrossRef CAS.
- S. K. Jain and M. Palmer, Free Radical Biol. Med., 1997, 22, 593 CAS.
- L. Packer, K. Kraemer and G. Rimbach, Nutrition, 2001, 17, 888 CrossRef CAS.
- A. Ceriello, D. Giugliano, A. Quatraro, C. Donzella, G. Dipalo and P. J. Lefebvre, Diabetes Care, 1991, 14, 68 CrossRef CAS.
- Y. Aoki, Y. Yanagisawa, K. Yazaki, H. Oguchi, K. Kiyosawa and S. Furuta, Diabetologia, 1992, 35, 913 CrossRef CAS.
- J. A. Vinson and T. B. Howard III, J. Nutr. Biochem., 1996, 7, 659 CrossRef CAS.
- Y. Tanaka, C. E. Gleason, P. O. Tran, J. S. Harmon and R. P. Robertson, Proc. Natl. Acad. Sci. U. S. A., 1999, 96, 10857 CrossRef CAS.
- A. A. Booth, R. G. Khalifah and B. G. Hudson, Biochem. Biophys. Res. Commun., 1996, 220, 113 CrossRef CAS.
- X. P. Liu, Y. J. Pang, W. W. Zhu, T. T. Zhao, M. Zheng, Y. B. Wang, Z. J. Sun and S. J. Sun, Clin. Exp. Pharmacol. Physiol., 2009, 36, 287 CrossRef CAS.
- J. M. Forbes, S. R. Thorpe, V. Thallas-Bonke, J. Pete, M. C. Thomas, E. R. Deemer, S. Bassal, A. El-Osta, D. M. Long, S. Panagiotopoulos, G. Jerums, T. M. Osicka and M. E. Cooper, J. Am. Soc. Nephrol., 2005, 16, 2363 CrossRef CAS.
- I. Ricci, R. Artacho and M. Olalla, Crit. Rev. Food Sci. Nutr., 2010, 50, 390 CrossRef CAS.
- M. Rendell, J. Nierenberg, C. Brannan, J. L. Valentine, P. M. Stephen, S. Dodds, P. Mercer, P. K. Smith and J. Walder, J. Lab. Clin. Med., 1986, 108, 286 CAS.
- M. Brownlee, H. Vlassara, A. Kooney, P. Ulrich and A. Cerami, Science, 1986, 232, 1629 CAS.
- D. Edelstein and M. Brownlee, Diabetes, 1992, 41, 26 CrossRef CAS.
- W. K. Bolton, D. C. Cattran, M. E. Williams, S. G. Adler, G. B. Appel, K. Cartwright, P. G. Foiles, B. I. Freedman, P. Raskin, R. E. Ratner, B. S. Spinowitz, F. C. Whittier and J. P. Wuerth, Am. J. Nephrol., 2004, 24, 32 CrossRef CAS.
- B. I. Freedman, J. P. Wuerth, K. Cartwright, R. P. Bain, S. Dippe, K. Hershon, A. D. Mooradian and B. S. Spinowitz, Controlled Clin. Trials, 1999, 20, 493 Search PubMed.
- B. L. Wedzicha, I. Bellion and S. J. Goddard, Adv. Exp. Med. Biol., 1991, 289, 217 CAS.
- M. X. Fu, K. J. Wells-Knecht, J. A. Blackledge, T. J. Lyons, S. R. Thorpe and J. W. Baynes, Diabetes, 1994, 43, 676 CrossRef CAS.
- T. Miyata, Y. Ueda, K. Asahi, Y. Izuhara, R. Inagi, A. Saito, C. Van Ypersele De Strihou and K. Kurokawa, J. Am. Soc. Nephrol., 2000, 11, 1719 CAS.
- R. Wada, Y. Nishizawa, N. Yagihashi, M. Takeuchi, Y. Ishikawa, K. Yasumura, M. Nakano and S. Yagihashi, Eur. J. Clin. Invest., 2001, 31, 513 CrossRef CAS.
- J. M. Onorato, A. J. Jenkins, S. R. Thorpe and J. W. Baynes, J. Biol. Chem., 2000, 275, 21177 CrossRef CAS.
- T. Soulis, S. Sastra, V. Thallas, S. B. Mortensen, M. Wilken, J. T. Clausen, O. J. Bjerrum, H. Petersen, J. Lau and G. Jerums, Diabetologia, 1999, 42, 472 CrossRef CAS.
- M. T. Coughlan, J. M. Forbes and M. E. Cooper, Kidney Int., 2007, 72, S54 CrossRef.
- S. Rahbar, Cell Biochem. Biophys., 2007, 48, 147 CrossRef CAS.
|
This journal is © The Royal Society of Chemistry 2011 |
Click here to see how this site uses Cookies. View our privacy policy here.