Transgene delivery and gelonin cytotoxicity enhanced by photochemical internalization in fibroblast-like synoviocytes (FLS) from rheumatoid arthritis patients
Received
1st November 2004
, Accepted 22nd February 2005
First published on 11th March 2005
Abstract
The objective of this study was to determine if photochemical internalization (PCI) of gelonin can improve the treatment outcome as compared to photodynamic therapy (PDT) and gene transduction of fibroblast-like synoviocytes (FLS)
in vitro. For this purpose synovial tissue was obtained under synovectomy of rheumatoid arthritis (RA) patients. Primary single cell suspensions were treated with the photosensitizer meso-tetraphenylporphine (TPPS2a) and light exposure (PDT) followed by evaluation of the cell survival by flow cytometry. PCI of gelonin was performed on FLS in passages 4 and 5 after removal from patients followed by measurements of protein synthesis 24 h after treatment. Additionally FLS were transduced with an adenovirus encoding the E.coli. lacZ gene and treated with PCI to evaluate the effect on the transduction rate. As a result all the cells in the primary cell suspension were susceptible to PDT but CD 106- (FLS) and CD14-positive (monocytes) cells were more sensitive to inactivation by PDT than CD2- (T-cells) and CD19-positive (B-cells) cells. With respect to protein synthesis FLS became up to 4-fold more sensitive to light when combining the photochemical treatment with the gelonin incubation. The fraction of virally transduced FLS was approximately doubled by means of PCI. In conclusion our experiments showed that PCI increased the cytotoxic effect of gelonin and adenoviral transduction of FLS derived from RA patients.
Introduction
Chronic synovitis as it is found in rheumatoid arthritis, is difficult to treat when it is not responding to the anti-inflammatory treatment. The indication for surgical synovectomy is seen if medical treatment does not have reasonable effect on pain and disability or if persistent tenosynovitis can harm tendons until they spontaneously rupture. Even by skilful arthroscopy it is technically difficult to achieve complete removal of synovitis tissue, especially in the chondro-synovial junction. This may lead to early recurrent synovitis. The value of surgical synovectomy especially in the smaller joints is therefore still discussed and most orthopaedic surgeons see the need for additional treatment. Methods such as chemical synoviorthesis, with osmic acid or thiotepa metabolites, and radioisotope synovectomy are not selective for the pathologic synovial tissue and ultra structural changes in the cartilage, menisci and subchondral bone may appear after the treatment.1–4 Although the recently introduced immunomodulatory and biologically treatment of RA patients has given remarkable results, about 30% of the patients suffer from severe oligo- or polyarthritis due to a lack of response to the medication or side effects which leads to discontinuation of the treatment. In conclusion there is still the need for minimal invasive procedures for synovectomy, which are suitable for all joints.
Photodynamic therapy (PDT) as a mode of treatment is characterised by cytotoxic reactions following activation of photosensitising substances by means of light. The photosensitizers are excited by the absorption of light at appropriate wavelengths and the mechanism of cytotoxicity involves the direct interaction of the excited dye with a cellular target (type I-reaction) or the generation of the reactive oxygen species singlet oxygen by the excited dye (type II-reaction).5 It is generally assumed that type II-reactions are predominant in PDT. In PDT red light is used in order to reach targets in deeper tissue layers, but shorter wavelengths may also be used when necessary due to the absorption of light by the photosensitizers in most of the visible wavelength region. PDT is approved for several cancer indications as well as age-related macula degeneration.6,7 Since the mid 1990s several authors have investigated the possibility to use PDT in the treatment of rheumatoid arthritis,8–12 mainly by inducing cell necrosis in the treated tissue. The aim has been to develop a photodynamic minimally invasive synovectomy technique and some encouraging results have been published,13,14 but this is still in the early stage of development.
Several macromolecules, such as genes, proteins, oligonucleotides and peptides, are under development for therapeutic purposes. One of the major limitations in their therapeutic effect is their limited ability to penetrate the plasma membrane, which is needed in order to reach their intracellular targets. Instead the macromolecules are to a large extent taken up into the cells by endocytosis where they are degraded in late endosomes and lysosomes. Some photosensitizers are also taken up into cells by endocytosis. When the photosensitizer is located in endocytic vesicles of the target cells the photochemical reaction causes the rupture of these vesicles even at sublethal doses. Thus, the endosomal escape of genes and other macromolecules by such photochemical treatment may increase their biological activity up to 100-fold15 instead of being degraded inside the lysosomes. The technique is named photochemical internalisation (PCI)16 and has the advantages of high efficacy and minimal side effects since the therapeutic effect is localised to the irradiated area.
PCI could potentially be utilized for the treatment of RA as a minimally invasive synovectomy with high specificity or as an immunomodulatory mode of treatment. PCI has been shown to improve the cytotoxic effect of several therapeutically relevant compounds, such as gelonin and gelonin-based immunotoxins, on cancer cells. Gelonin is a type I ribosome-inactivating protein (RIP) toxin, inhibiting protein synthesis by its rRNA glycosidase activity. In contrast to the type II RIPs, such as ricin and abrin, type I RIPs are relatively non-toxic to intact cells. This is due to the inability of these proteins to reach cytosol where they can exert their rRNA glycosidase activity. The enhanced efficacy of gelonin cytotoxicity by means of PCI has been shown in cancer cells to occur even at sublethal PDT doses. Thus, PCI may improve both the efficacy and, by means of cell specific targeting of the protein toxin, the specificity of this potential mode of treatment. Furthermore, gene therapy-based treatment of RA is currently under development. However, low efficacy and specificity as well as immunological reactions have limited the outcome of the gene therapy-based clinical trials. Gene therapy may be utilized to deliver genes expressing cytotoxic and immunomodulatory proteins, both of which are of interest in the treatment of RA. PCI has been shown to enhance the transgene expression of both reporter and therapeutic genes in cancer cells delivered by both non-viral and viral vectors.
The aim of this study was to investigate the possibility of using photochemical internalisation to improve the light induced treatment effect on fibroblast-like synoviocytes derived from rheumatoid arthritis patients by introducing a protein toxin and to show the possibility of using PCI to achieve transduction of genes in the cells of the same origin. The results indicate that both approaches would be promising as new, minimal invasive synovectomy techniques.
Materials and methods
Cell culture
Synovial tissue was obtained under synovectomy from four RA patients according to the revised criteria from the American College of Rheumatology (ACR, formerly the American Rheumatism Association).17 All patients gave their informed consent and the local ethical committee approved the study protocol. The tissue was cut into small pieces under sterile conditions and digested with 2 mg ml−1 collagenase (Sigma, St. Louis, MO, USA) in phosphate buffered saline (PBS) for 90 min at 37 °C in a shaking incubator. The cell suspension was sheared by repeatedly passing through a sterile syringe and washed twice with PBS. The cell pellet obtained after the last wash was resuspended in RPMI 1640 supplemented with 10% fetal calf serum, 1%
L-glutamine, 100 u ml−1 penicillin and 100 μg ml−1 streptomycin at 37 °C in an incubator flushed with 5% CO2 in air (standard conditions). After 24 h culturing the non-adherent cells were removed by washing the cells with RPMI 1640. Further culturing was done as a monolayer culture in RPMI 1640 supplemented with 10% fetal calf serum, 1%
L-glutamine and 1% streptomycin–penicillin solution at 37 °C with 5% CO2. Cells, containing mainly fibroblast-like synoviocytes (FLS), were cultured after seeding until passages 3 to 5, before use in the experiments.
Chemicals
TPPS2a
(meso-tetraphenylporphine with two sulfonate groups on adjacent phenyl rings) was used as photosensitizing substance and was provided by PCI Biotech AS, Oslo, Norway. The purity of the substance has previously been tested and showed one peak on HPLC.18,19 The stock solution of 2 mg ml−1 in dimethyl sulfoxide was kept at −20 °C in the dark until use. Gelonin was purified from Gelonium multiflorum by Dr Gowsala Sivam, Bastyr University, Seattle, USA. A stock solution of 2 mg ml−1 was kept at −20 °C until use. Phycoerythrin (PE) conjugated mouse anti-human CD 106 (VCAM-1) was purchased from BD Biosiences (Franklin Lake, USA) and PE conjugated mouse anti-human CD 2, CD 14 and CD 19 were purchased from Dako Cytomation Denmark A/S. The non-replicative adenovirus serotype 5 (AdHCMV-lacZ), encoding for the β-galactosidase gene lacZ controlled by the human cytomegalovirus promoter was a kind gift from Dr Frank L. Graham, McMaster University, Hamilton, ON, Canada. The amount of infectious particles is given in multiplicity of infection (MOI).
PDT effect on primary tissue cells
Synovial tissue was obtained from three RA patients according to guidelines from the ACR.17 All patients gave their informed consent and the local ethical committee approved the study protocol. Patients were referred to surgical synovectomy for therapy of painful synovitis resistant to medical therapy. The synovial tissue was processed and digested as described above. The cell pellet obtained after the last wash was resuspended in RPMI 1640 supplemented with 0.4 μg ml−1 TPPS2a, 10% fetal calf serum, 1%
L-glutamine, 100 u ml−1 penicillin and 100 μg ml−1 streptomycin and 150 × 103 cells were seeded in 25 cm3 flasks (Nunc, Denmark) and incubated at 37 °C in an incubator flushed with 5% CO2 in air (standard conditions) under subdued light. After 18 h the non-adherent cells were separated by removing the supernatant from the flasks and the photosensitizer was removed from the medium by washing three times with RPMI 1640 followed by light exposure 4 h later. 18 h after PDT the cells were removed from the flasks with EDTA and washed twice in PBS. The pellets were stained with 50 μl phycoerythrin conjugated antibodies at 4 °C for 30 min for each cell type in a separate sample. After resuspention in 400 μl RPMI the cells were filtered through a 50 μm mesh nylon filter. The cells were then analyzed in a FACS Calibur flow cytometer (Becton Dickinson, CA, USA). 1 μl propidium iodine (PI)
(0.5 mg ml−1, Calbiochem Corporation, CA, USA) was added to each sample to discriminate dead cells from viable cells, and pulse processing was performed to discriminate cell doublets from single cells. Viable single cells were gated and analyzed for PE fluorescence. For each sample 5000 events were collected and the data were analyzed with CELLQuest Software (Becton Dickinson, CA, USA).
Protein synthesis assay
Sixty thousand cells were seeded out and incubated in 24-well plates (Nunc, Denmark) in 0.5 ml RPMI medium containing 0.2 μg ml−1 TPPS2a and 5 μg ml−1 gelonin for 18 h. The photosensitizer and gelonin were then removed by washing the cells three times with RPMI medium and the cells were exposed to light 4 h later. 18 h after light exposure the cells were incubated for 30 min in leucine-free medium followed by 30 min incubation in MEM containing 2 μl ml−13[H]leucine. Cells were then fixed with 200 μl 5% TCA for 15 min, followed by treatment with 200 μl 0.1 M KOH before they were analyzed for 3[H]leucine incorporation in the proteins.
Light source
The cells were exposed to blue light from a bank of 4 light tubes emitting light in the region 375–450 nm with a maximum around 435 nm (irradiance 13 mW cm−2, LumiSource® PCI Biotech, Oslo, Norway).
Cell proliferation assay
One hundred thousand cells were plated in 25 cm2 flasks (Nunc, Denmark) and inoculated under standard conditions. For each measurement, cells from 2 flasks were trypsinised and analyzed for cell content by a Coulter Counter. At least 10 000 cells were measured from each culture.
Fluorescence microscopy
Fluorescence microscopy of the cells was carried out in 10 cm2 dishes (Falcon 3002) in PBS and a cover glass gently put on top. A Zeiss Axioplan epi-fluorescence and phase contrast microscope equipped with a 63× magnification oil immersion objective (Zeiss, Obercochem, Germany) was used. A HBO/100 W mercury lamp was used for excitation in the fluorescence analysis. The microscope was equipped with a cooled charge-coupled device (CCD) camera (Photometrics Inc., Tucson, AZ, USA), which was used to record the phase contrast and the fluorescence micrographs. A 390–440 nm band pass excitation filter, a 470 nm dichroic beam splitter and a 610 nm long pass filter were used for recording TPPS2a fluorescence.
Adenoviral transduction of fibroblast-like synoviocytes
Seventy five thousand FLS (passages 4 and 5) were seeded out in 12-well plates (Nunc, Denmark). Under subdued light, 1 ml medium containing 0.4 μg ml−1 TPPS2a was added. The cells were incubated at 37 °C for 18 h, washed three times with RPMI medium to remove the photosensitizer from the medium and incubated for another 3 h before infection. AdhCMV-lacZ was diluted in PBS containing 0.68 mM CaCl2 and 0.5 mM MgCl2 to obtain the correct viral concentrations. The cells were incubated with virus (MOI 50 and MOI 100) for 30 min at 37 °C before removal of the virus by washing with RPMI. After another 30 min, the cells were exposed to light and kept in culture for 3 d before analysis. The cells were trypsinized, centrifuged, resuspended in 25 μl medium and incubated for 5 min at 37 °C. Twenty five μl of 2 mM fluorescein di-β-D-galactopyranoside (Molecular Probes, Eugene, OR, USA) were added, and the cells were incubated for 1 min at 37 °C before being diluted by adding 450 μl ice-cold growth medium. The samples were maintained on ice for 30–60 min, filtered through a 50 μm mesh nylon filter and analysed in a FACS-Calibur flow cytometer (Becton Dickinson, CA, USA). For each sample 10 000 events were collected. Fluorescein-fluorescence was measured through a 510–530 nm filter after excitation with an argon laser (15 mW, 488 nm). Dead cells were discriminated from single viable cells by gating on forward scattering versus side scattering. The data were analysed with the CELLQuest Software (Becton Dickinson).
Results
Photodynamic treatment of single cell suspension
The sensitivity of different cell types from synovial tissue to photodynamic therapy was measured by flow cytometric cell counting the day after PDT (Fig. 1). The cell populations analyzed in our experiments were T-cells (CD 2 positive), B-cells (CD19 positive), monocytes/tissue-macrophages (CD14 positive) and fibroblast-like synoviocytes (CD106/VCAM-1 positive).20–22 All the investigated cells were susceptible to PDT in a light dose dependent manner. CD 106- and CD 14-positive cells were more sensitive than CD 2- and CD 19-positive cells and were effectively killed by the maximum light dose. We could not find differences in susceptibility to PDT between primary FLS (CD 106+) and FLS from passage 3 (data not shown). Tissue macrophages, B-cells and T-cells were not analyzed for cell passage dependency because these cells are not proliferating in culture.
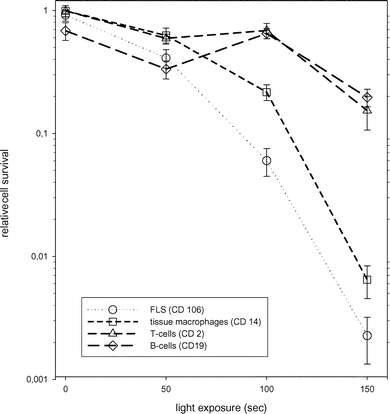 |
| Fig. 1 Sensitivity of various cell types from synovial tissue of RA patients to PDT. All values are the mean of 6 experiments (±standard error) with tissue from 3 RA patients. The cytotoxic effect of PDT on the 4 major cell types (see inset in the figure) of synovial tissue was measured by flow cytometry as described in ‘Materials and methods’. | |
Intracellular localization of TPPS2a in fibroblast-like synoviocytes (FLS)
Fluorescence microscopy analyses of FLS after incubation with 0.4 μg ml−1 TPPS2a showed fluorescence inside the cells. Compared to the phase contrast picture of the same cells it can be stated that the fluorescence is located to the intracellular granules resembling endocytic vesicles (Fig. 2). After light treatment the fluorescence showed a more diffuse pattern which is a sign of the relocation of TPPS2a into the cytosol (data not shown).
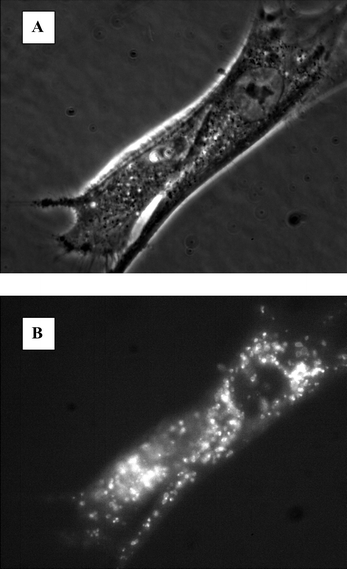 |
| Fig. 2 Micrographs of fibroblast-like synoviocytes after treatment with TPPS2a. The cells were treated with 0.4 mg ml−1 TPPS2a as described in ‘Materials and methods’. A: phase contrast micrograph of FLS; B: fluorescence of the photosensitizer in the same cells. | |
PCI of gelonin
The high sensitivity of FLS for TPPS2a-based PDT and the intracellular localization and relocalization upon light activation indicated that FLS could be susceptible to PCI treatment.23,24 The treatment of FLS with 0.2 μg ml−1 TPPS2a at selected light doses had no significant effect on the protein synthesis 24 h after treatment (Fig. 3), which has previously been found to reflect cell survival.16 In contrast, the treatment in combination with gelonin showed a light dose dependent attenuation of protein synthesis. In combination with emerging gelonin concentrations FLS became more sensitive to the photochemical treatment. Gelonin alone had no effect on the rate of protein synthesis at any of the concentrations used in this study.
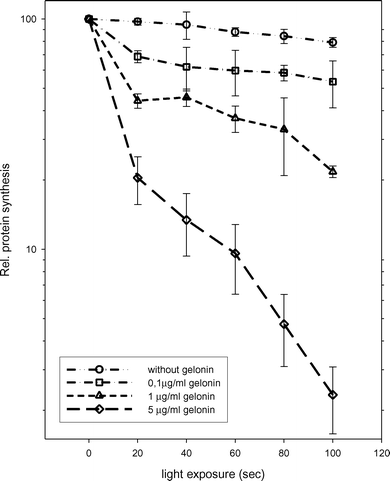 |
| Fig. 3 Cytotoxicity of gelonin on FLS when combined with TPPS2a and light. The FLS cells (passage 5) were treated with 0.2 μg ml−1 TPPS2a and gelonin as described in ‘Materials and methods’ and on the figure. The cytotoxic effect was measured by a protein synthesis assay as described in ‘Materials and methods’. All values are expressed as the mean of 6 experiments (±standard error) with tissue from 3 RA patients. | |
Adenoviral transduction of the cells
Adenoviral transduction of synoviocytes and other relevant cells are under consideration as a method for the delivery of therapeutic genes in the treatment of RA.25,26 A high adenoviral transduction rate in vivo requires a treatment with a high number of particles, which may cause serious immunological reactions.27 Thus, PCI was evaluated as a means to enhance adenoviral transduction.
The incubation of FLS with AdhCMV-lacZ at MOI 50 and MOI 100 alone induced transduction in 1.5% (±0.03) and 4.3% (±0.5) of the cells respectively. When the adenovirus treatment was combined with PCI the transduction efficiency increased 4-fold to 6.3% (±1.1) for MOI 50 and 2-fold to 8.3% (±0.1) for MOI 100, respectively (Fig. 4). PDT alone did not influence the numbers of positive cells.
Cell proliferation measurements comparing FLS with a malignant cell line (CME-1) could confirm a significantly lower mitotic rate for FLS with a doubling time that was found to be 96 h (data not shown). Thus we have not evaluated the possibility of transfecting FLS by non-viral vectors because it has been shown that slowly dividing cells are not efficiently transfected by means of PCI.28
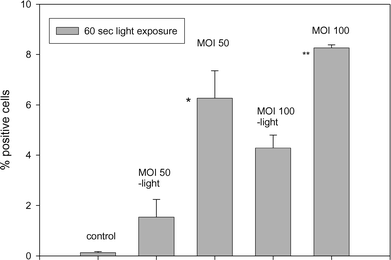 |
| Fig. 4 Transduction of FLS by means of PCI. The cells were treated with TPPS2a and the adenovirus AdhCMV-lacZ as described in ‘Materials and methods’ and on the figure and analyzed for expression of β-galactosidase activity 3 d after 60 s of light exposure. The results were analysed for statistical differences (Student’s t-test) between cells treated with AdhCMV-lacZ only and in combination with PCI; (*, p < 0.08; **, p < 0.002). All values are expressed as the mean of 6 experiments (±standard error) with tissue from 3 RA patients. | |
Discussion
Photodynamic therapy of chronic synovitis is thought to be a minimally invasive mode of treatment for patients suffering of persistent arthritis in spite of the efficient medical treatment of the underlying disease. Several authors have concluded that PDT is feasible to treat synovitis with different approaches.10,11,13,14 However since the first results were presented nine years ago and until today to our knowledge no clinical trial has been undertaken. One of the reasons may be the poor selectivity of the first generation of photosensitizing substances like Photofrin and Photosan used in some of the studies. Another issue that could impede the use of PDT for the treatment of rheumatoid arthritis is the complex structure especially of the larger joints which may lead to an inhomogeneous light distribution. Thus the principle of using PDT for synovitis is documented, but the specificity and efficacy need to be improved before a good clinical outcome can be expected. The PCI technique combines the advantages of the PDT treatment with the possibility of photoinduction of toxins or genes into the treatment area. Hereby it may increase the level of specificity and efficiency of the mode of treatment.
Our experiments on primary cell suspensions from RA patients showed light dose and cell type dependent cytotoxicity towards PDT. Macrophages and FLSs were found to be more sensitive to TPPS2a-based PDT than the T- and B-cells. The high sensitivity of macrophages to TPPS2a-mediated PDT correlates well with the high endocytic–phagocytic activity of such cells. The high sensitivity of FLS to PDT may also indicate high endocytotic activity of these cells. Macrophages and FLSs are regarded as promoters of destruction in synovitis and are therefore of great interest from a therapeutic point of view.29,30 The PDT effect we have shown on these cells may effectively halt pannus development. Thus, PDT using photosensitizers targeting mainly FLS and macrophages should be considered for the treatment of RA. The low susceptibility of B-cells and T-cells to PDT may be due to a lower rate of endocytosis causing a lower intracellular sensitizer-concentration compared to tissue macrophages and FLS. This is in accordance with the low effect of PCI of gelonin in B-cells (unpublished results).
The specificity of TPPS2a-mediated PDT for FLS led us to investigate PCI of gelonin on FLSs. Gelonin was used in this study to investigate the effect of PCI on the release of therapeutic substances from endosomes and lysosomes. A clearly synergistic effect was induced when subtoxic PDT doses were combined with non-toxic doses of gelonin. The increase in cell death induced by PCI of gelonin indicates that gelonin is released into the cytosol in an active form. Previous studies of the PCI concept have been performed with cancer cells and synoviocytes from RA patients have never previously been evaluated. The PCI method is dependent on the photochemical release of the therapeutic macromolecule prior to its degradation in the hydrolytic environment of the endocytic vesicles. The levels of different cathepsins, which are the hydrolytic enzymes of major importance for cytotoxicity, may vary between cancer cells, synoviocytes and normal cells31 and a therapeutic window for PCI with respect to the release of functionally active gelonin could have not been predicted. Additionally, the release of cathepsins into the cytosol is usually assumed to be a highly cytotoxic event.32,33 This does not seem to be the case after PCI due to rupture of only a fraction of the endocytic vesicles, a high sensitivity of the cathepsins to photoinactivation and inhibition of functionally active cathepsins in the cytosol by cathepsin inhibitors of the stefin superfamily.34,35 A fraction of the cathepsins has been found to be released into the cytosol after TPPS2a-mediated PDT, but cytosolic cathepsin inhibitors have to a high extent inhibited this activity.34 In cells with low levels of cathepsin inhibitors the cathepsins will be able to exert their hydrolytic activity when released into the cytosol and thereby cause cell death. In such a scenario cells will be killed by the release of cathepsins and the benefit of the endocytic escape of the therapeutics will be minor. An imbalance between cathepsins and their inhibitors has been observed in rheumatoid arthritis synovium.36 Photochemical rupture of the endocytic vesicles in the cells from the RA synovium could therefore have introduced higher cathepsin-based cytotoxicity and thereby less PCI-induced activation of potential therapeutics such as gelonin and DNA. However, the present study based on primary synoviocytes from three different patients indicates that the hydrolytic activity in the endocytic vesicles and the balance between cathepsins and the cytosolic inhibitors do not impair the possible use of PCI of gelonin and other macromolecular therapeutics for the treatment of RA. The potentiation of the cytotoxicity of gelonin by means of PCI seems similar to or better than what is observed in cancer cells.37
The specificity of PCI of gelonin in RA treatment relies on the preferential uptake of the photosensitizer by the synoviocytes and the macrophages and the requirement for light activation. Gelonin itself is not expected to have any particular cell type preference although cellular uptake should be expected to correlate with the endocytotic activity as well as mannose receptor-mediated endocytosis of the cells.38 Intraarticular delivery of gelonin should therefore be considered as a treatment option. Systemic delivery of gelonin is not expected to be efficient due to the small size and the short half-life in the circulation.39 However, the use of PCI of immunotoxins has already been proved efficient in cancer cells40 and should also be considered in RA treatment.41,42
Gene therapy has been investigated for the treatment of synovitis in RA43–48 and the first clinical studies with synoviocytes expressing IL-1Ra from a retroviral vector demonstrated that such an approach can be safe and feasible.44 The different approaches can be divided into pannus-addressing and immune response-addressing. The former attempts to induce apoptosis or necrosis in the synovial lining cells similar to a chemical synovectomy49,50 and the latter tries to induce a long lasting modification or blocking of the immunoregulatory systems. Various types of vectors are proposed in the literature with each vector having advantages and disadvantages. There is still no perfect vector for the delivery of genes and targeting of gene therapy to the arthritic joint is an unsolved problem.48 Immunological reactions against vectors or vector particles, is regarded as a serious threat to the patient. PCI has been shown to efficiently enhance adenoviral transduction in cancer cells.51 It was of great interest to evaluate this treatment in primary fibroblast-like synoviocytes from RA patients mainly because the local delivery of genes into the inflamed joint by means of PCI may limit the possibility of systemic side effects and may increase the specificity and efficiency of gene transfer.
In our experiments the fraction of transduced FLS improved after PCI. Even if the number of transduced FLS cells remains low we could increase the amount from 1.5% to over 6%. It should be noted that the MOI used was relatively low compared with other publications. This may reduce the development of an immunological response in a clinical setting. It is known from the literature that when using therapeutic genes with bystander effects (such as HSV-thymidine kinase) less than 10% of the cells needs to be transduced in order to obtain complete responses.52 Such genes are of great interest and clinical studies on the use of thymidine kinase have already been proposed.48 The relatively low rate of spontaneous transduction and the fact that the PCI treatment will limit the enhancement of transduction to the area of light treatment, will together address one of the main hazards of in vivo gene therapy: the uncontrolled general activity of therapeutic gene in humans. The relatively low adenoviral transduction efficacy is due to the lack of Coxsackie adenovirus receptors (CAR) on the cell surface of synoviocytes.26 An efficient adenoviral transduction of synoviocytes may therefore be obtained by PCI of retargeted adenoviral particles.
In the present study the cells have been exposed to blue light because of the high extinction coefficient of the photosensitizer TPPS2a in this wavelength region. However, in vivo red light is usually more efficient due to the more efficient transmission of red light through tissues than that of blue light. The photosensitizer used in present study has absorption properties similar to those of Photofrin and may be utilized in vivo. On the other hand AlPcS2a has physico-chemical properties similar to TPPS2a, can be substituted for TPPS2a in PCI experiments53 and have been used in vivo to document PCI of gelonin in a tumor model.54 Thus, in an in vivo clinical setting a photosensitizer with better spectral properties than TPPS2a, such as AlPcS2a, will be utilized.
Our results provided evidence that PCI has a potential impact on viral gene delivery and protein toxins in fibroblast-like synoviocytes derived from RA patients. To our knowledge this is the first time that the PCI principle has been applied to human cells derived from patients suffering from RA. Our studies have demonstrated that both the amount of sensitizer and light needed to induce a cytotoxic reaction in FLS could be reduced significantly by combining a photochemical treatment with a protein toxin. Furthermore, our experiments demonstrate that PCI has a potential effect on the endosomal escape of therapeutic substances after their endocytotic uptake in FLS. This prevents highly active substances from their lysosomal digestion. The PCI principle may be used with a variety of drugs, which are not feasible for the treatment of RA today. The effective and safe delivery of genes into synovitis tissue, while sparing the normal tissue, is the main problem in gene therapy. PCI combines the possibility of light control and molecular targeting, which may reduce the side effects of the treatment.
In conclusion, PCI may be a new instrument for the phototherapeutic treatment of synovitis in RA patients and other diseases.
Abbreviations
AdhCMV-lacZ, adenovirus serotype 5 encoding the E. coli lacZ gene; CAR, Coxsackie and adenovirus receptor; MOI, multiplicity of infection; PCI, photochemical internalization; PDT, photodynamic therapy; TPPS2a, meso-tetraphenylporphine with 2 sulfonate groups on adjacent phenyl rings; FLS, fibroblast-like synoviocytes; RA, rheumatoid arthritis; RIP, ribosome-inactivating protein; rRNA, ribosomal RNA.
Acknowledgements
This work has been supported in part by the laboratory staff of the PCI-group at the Department of Biophysics, The Norwegian Radium Hospital, Oslo, Norway and by Professor O Reikerås, Orthopaedic Department, Rikshospitalet University Hospital, Oslo, Norway.
References
- R. Bessant, A. Steuer, S. Rigby and M. Gumpel, Osmic acid revisited: factors that predict a favourable response, Rheumatology, 2003, 42, 1036–1043 Search PubMed.
- M. Heim, R. Tiktinsky, Y. Amit and U. Martinowitz, Yttrium synoviorthesis of the elbow joints in persons with haemophilia, Haemophilia, 2004, 10(Suppl. 2), 590–592 CrossRef CAS.
- X. Ayral, Chemical synoviorthesis with osmic acid in haemophilia, Haemophilia, 2001, 7, 20–25 CrossRef.
- A. Falcon de Vargas and F. Fernandez-Palazzi, Cytogenetic studies in patients with hemophilic hemarthrosis treated by 198Au, 186Rh, and 90Y radioactive synoviorthesis, J. Pediatr. Orthop. B, 2000, 9, 52–54 Search PubMed.
- T. J. Dougherty, C. J. Gomer, B. W. Henderson, G. Jori, D. Kessel, M. Korbelik, J. Moan and Q. Peng, Photodynamic therapy, J. Natl. Cancer Inst., 1998, 90, 889–905 CrossRef CAS.
- S. B. Brown, E. A. Brown and I. Walker, The present and future role of photodynamic therapy in cancer treatment, Lancet Oncol., 2004, 5, 497–508 Search PubMed.
- T. J. Dougherty, An update on photodynamic therapy applications, J. Clin. Laser Med. Surg., 2002, 20, 3–7 CrossRef.
- G. R. Burmester, A. Dimitriu-Bona, S. J. Waters and R. J. Winchester, Identification of three major synovial lining cell populations by monoclonal antibodies directed to Ia antigens and antigens associated with monocytes/macrophages and fibroblasts, Scand. J. Immunol, 1983, 17, 69–82 Search PubMed.
- C. Hendrich and W. E. Siebert, Photodynamic therapy for rheumatoid arthritis?, Lasers Surg. Med., 1997, 21, 359–364 CAS.
- G. Kirdaite, N. Lange, N. Busso, B. H. Van Den, P. Kucera and A. So, Protoporphyrin IX photodynamic therapy for synovitis, Arthritis Rheum., 2002, 46, 1371–1378 CrossRef CAS.
- L. G. Ratkay, R. K. Chowdhary, H. C. Neyndorff, J. Tonzetich, J. D. Waterfield and J. G. Levy, Photodynamic therapy; a comparison with other immunomodulatory treatments of adjuvant-enhanced arthritis in MRL-lpr mice, Clin. Exp. Immunol., 1994, 95, 373–377 CAS.
- K. B. Trauner and T. Hasan, Photodynamic treatment of rheumatoid and inflammatory arthritis, Photochem. Photobiol., 1996, 64, 740–750 CrossRef CAS.
- C. Hendrich, G. Huttmann, J. L. Vispo-Seara, S. Houserek and W. E. Siebert, Experimental photodynamic laser therapy for rheumatoid arthritis with a second generation photosensitizer, Knee Surg. Sports Traumatol. Arthrosc., 2000, 8, 190–194 CrossRef CAS.
- K. B. Trauner, R. Gandour-Edwards, M. Bamberg, S. Shortkroff, C. Sledge and T. Hasan, Photodynamic synovectomy using benzoporphyrin derivative in an antigen-induced arthritis model for rheumatoid arthritis, Photochem. Photobiol., 1998, 67, 133–139 CrossRef CAS.
- P. K. Selbo, A. Hogset, L. Prasmickaite and K. Berg, Photochemical internalisation: a novel drug delivery system, Tumour Biol., 2002, 23, 103–112 Search PubMed.
- K. Berg, P. Kristian Selbo, L. Prasmickaite, T. E. Tjelle, K. Sandvig, J. Moan, G. Gaudernack, O. Fodstad, S. Kjolsrud, H. Anholt, G. H. Rodal, S. K. Rodal and A. Hogset, Photochemical Internalization: A Novel Technology for Delivery of Macromolecules into Cytosol, Cancer Res., 1999, 59, 1180–1183 CAS.
- F. C. Arnett, S. M. Edworthy, D. A. Bloch, D. J. McShane, J. F. Fries, N. S. Cooper, L. A. Healey, S. R. Kaplan, M. H. Liang and H. S. Luthra, The American Rheumatism Association 1987 revised criteria for the classification of rheumatoid arthritis, Arthritis Rheum., 1988, 31, 315–324 CrossRef CAS.
- K. Berg, J. C. Bommer, J. W. Winkelman and J. Moan, Cellular uptake and relative efficiency in cell inactivation by photoactivated sulfonated meso-tetraphenylporphines, Photochem. Photobiol., 1990, 52, 775–781 CrossRef CAS.
- M. Kongshaug, J. Moan and S. B. Brown, The distribution of porphyrins with different tumour localising ability among human plasma proteins, Br. J. Cancer, 1989, 59, 184–188 CAS.
- D. Croft, P. McIntyre, A. Wibulswas and I. J. Kramer, Sustained Elevated Levels of VCAM-1 in Cultured Fibroblast-like Synoviocytes Can Be Achieved by TNF-{alpha} in Combination with Either IL-4 or IL-13 through Increased mRNA Stability, Am. J. Pathol, 1999, 154, 1149 Search PubMed.
- J. S. Smolen and G. Steiner, Therapeutic strategies for rheumatoid arthritis, Nat. Rev. Drug Discov., 2003, 2, 473–488 CrossRef CAS.
- D. Mulherin, O. Fitzgerald and B. Bresnihan, Synovial tissue macrophage populations and articular damage in rheumatoid arthritis, Arthritis Rheum., 1996, 39, 115–124 CrossRef CAS.
- L. Barbieri, M. G. Battelli and F. Stirpe, Ribosome-inactivating proteins from plants, Biochim. Biophys. Acta, 1993, 1154, 237–282 CAS.
- F. Stirpe, S. Olsnes and A. Pihl, Gelonin, a new inhibitor of protein synthesis, nontoxic to intact cells. Isolation, characterization, and preparation of cytotoxic complexes with concanavalin A, J. Biol. Chem., 1980, 255, 6947–6953 CAS.
- G. C. Tsokos and M. Tsokos, The TRAIL to arthritis, J. Clin. Invest., 2003, 112, 1315–1317 CrossRef CAS.
- P. H. Goossens, M. J. Havenga, E. Pieterman, A. A. Lemckert, F. C. Breedveld, A. Bout and T. W. Huizinga, Infection efficiency of type 5 adenoviral vectors in synovial tissue can be enhanced with a type 16 fiber, Arthritis Rheum., 2001, 44, 570–577 CAS.
- I. H. Carmen, A death in the laboratory: the politics of the Gelsinger aftermath, Mol. Ther., 2001, 3, 425–428 CrossRef CAS.
- A. Dietze, A. Bonsted, A. Hogset and K. Berg, Photochemical internalization enhances the cytotoxic effect of the protein toxin gelonin and transgene expression in sarcoma cells, Photochem. Photobiol., 2003, 78, 283–289 CrossRef CAS.
- N. J. Zvaifler and G. S. Firestein, Pannus and pannocytes. Alternative models of joint destruction in rheumatoid arthritis, Arthritis Rheum., 1994, 37, 783–789 CrossRef CAS.
- D. Lacey, A. Sampey, R. Mitchell, R. Bucala, L. Santos, M. Leech and E. Morand, Control of fibroblast-like synoviocyte proliferation by macrophage migration inhibitory factor, Arthritis Rheum., 2003, 48, 103–109 CrossRef CAS.
- S. Yan and B. F. Sloane, Molecular regulation of human cathepsin B: implication in pathologies, Biol. Chem., 2003, 384, 845–854 CrossRef CAS.
- T. Cirman, K. Oresic, G. D. Mazovec, V. Turk, J. C. Reed, R. M. Myers, G. S. Salvesen and B. Turk, Selective disruption of lysosomes in HeLa cells triggers apoptosis mediated by cleavage of Bid by multiple papain-like lysosomal cathepsins, J. Biol. Chem., 2004, 279, 3578–3587 CrossRef CAS.
- P. D. Wilson, R. A. Firestone and J. Lenard, The role of lysosomal enzymes in killing of mammalian cells by the lysosomotropic detergent N-dodecylimidazole, J. Cell Biol., 1987, 104, 1223–1229 CrossRef CAS.
- K. Berg and J. Moan, Lysosomes as photochemical targets, Int. J. Cancer, 1994, 59, 814–822 CrossRef CAS.
- J. Kos and T. T. Lah, Cysteine proteinases and their endogenous inhibitors: target proteins for prognosis, diagnosis and therapy in cancer (review), Oncol. Rep., 1998, 5, 1349–1361 CAS.
- J. Kekow, T. Pap and S. Zielinski, Multifunctional role of proteases in rheumatic diseases, Adv. Exp. Med. Biol., 2000, 477, 467–476 CAS.
- A. Hogset, L. Prasmickaite, P. K. Selbo, M. Hellum, B. O. Engesaeter, A. Bonsted and K. Berg, Photochemical internalisation in drug and gene delivery, Adv. Drug Deliv. Rev., 2004, 56, 95–115 CrossRef CAS.
- S. Madan and P. C. Ghosh, Interaction of gelonin with macrophages: effect of lysosomotropic amines, Exp. Cell Res., 1992, 198, 52–58 CrossRef CAS.
- L. Barbieri, M. G. Battelli and F. Stirpe, Blood clearance and organ distribution and tissue concentration of native, homopolymerized and IgG-conjugated ribosome-inactivating proteins, Xenobiotica, 1990, 20, 1331–1341 CrossRef CAS.
- P. K. Selbo, G. Sivam, O. Fodstad, K. Sandvig and K. Berg, Photochemical internalisation increases the cytotoxic effect of the immunotoxin MOC31-gelonin, Int. J. Cancer, 2000, 87, 853–859 CrossRef CAS.
- J. A. van Roon, A. J. van Vuuren, S. Wijngaarden, K. M. Jacobs, J. W. Bijlsma, F. P. Lafeber, T. Thepen and J. G. van de Winkel, Selective elimination of synovial inflammatory macrophages in rheumatoid arthritis by an Fcgamma receptor I-directed immunotoxin, Arthritis Rheum., 2003, 48, 1229–1238 CrossRef CAS.
- T. M. Kuzel, DAB(389)IL-2 (denileukin diftitox, ONTAK): review of clinical trials to date, Clin. Lymphoma, 2000, 1(Suppl. 1), S33–S36 Search PubMed.
- C. H. Evans, S. C. Ghivizzani, R. Kang, T. Muzzonigro, M. C. Wasko, J. H. Herndon and P. D. Robbins, Gene therapy for rheumatic diseases, Arthritis Rheum., 1999, 42, 1–16 CrossRef CAS.
- C. H. Evans, S. C. Ghivizzani, J. H. Herndon, M. C. Wasko, J. Reinecke, P. Wehling and P. D. Robbins, Clinical trials in the gene therapy of arthritis, Clin. Orthop., 2000, S300–S307 Search PubMed.
- S. C. Ghivizzani, T. J. Oligino, J. C. Glorioso, P. D. Robbins and C. H. Evans, Direct gene delivery strategies for the treatment of rheumatoid arthritis, Drug Discov. Today, 2001, 6, 259–267 CrossRef CAS.
- J. N. Gouze, S. C. Ghivizzani, E. Gouze, G. D. Palmer, O. B. Betz, P. D. Robbins, C. H. Evans and J. H. Herndon, Gene therapy for rheumatoid arthritis, Hand Surg., 2001, 6, 211–219 CrossRef CAS.
- I. H. Tarner and C. G. Fathman, Gene therapy in autoimmune disease, Curr. Opin. Immunol., 2001, 13, 676–682 CrossRef CAS.
- P. D. Robbins, C. H. Evans and Y. Chernajovsky, Gene therapy for arthritis, Gene Ther., 2003, 10, 902–911 CrossRef CAS.
- Q. Yao, J. C. Glorioso, C. H. Evans, P. D. Robbins, I. Kovesdi, T. J. Oligino and S. C. Ghivizzani, Adenoviral mediated delivery of FAS ligand to arthritic joints causes extensive apoptosis in the synovial lining, J. Gene Med., 2000, 2, 210–219 CrossRef CAS.
- Q. Yao, S. Wang, A. Gambotto, J. C. Glorioso, C. H. Evans, P. D. Robbins, S. C. Ghivizzani and T. J. Oligino, Intra-articular adenoviral-mediated gene transfer of trail induces apoptosis of arthritic rabbit synovium, Gene Ther., 2003, 10, 1055–1060 CrossRef CAS.
- A. Hogset, E. B. Ovstebo, L. Prasmickaite, K. Berg, O. Fodstad and G. M. Maelandsmo, Light-induced adenovirus gene transfer, an efficient and specific gene delivery technology for cancer gene therapy, Cancer Gene Ther., 2002, 9, 365–371 CrossRef CAS.
- M. Caruso, Gene therapy against cancer and HIV infection using the gene encoding herpes simplex virus thymidine kinase, Mol. Med. Today, 1996, 2, 212–217 CrossRef CAS.
- L. Prasmickaite, A. Hogset and K. Berg, Evaluation of different photosensitizers for use in photochemical gene transfection, Photochem. Photobiol., 2001, 73, 388–395 CrossRef CAS.
- P. K. Selbo, G. Sivam, O. Fodstad, K. Sandvig and K. Berg,
In vivo documentation
of photochemical internalization, a novel approach to site specific cancer therapy, Int. J. Cancer, 2001, 92, 761–766 CrossRef CAS.
|
This journal is © The Royal Society of Chemistry and Owner Societies 2005 |
Click here to see how this site uses Cookies. View our privacy policy here.