DOI:
10.1039/B307435H
(Paper)
PhysChemComm, 2003,
6, 55-58
Thermoacoustical approach to the intermolecular free-length of liquid mixtures
Received
30th June 2003
, Accepted 25th July 2003
First published on 5944th August 2003
Abstract
The intermolecular free-length is evaluated by making use of thermoacoustical parameters followed by a comparative study. To achieve this objective, thermoacoustical parameters of four ternary and two quaternary liquid mixtures have been computed. These parameters have been utilized to calculate the available volume (Va), which in turn has been used to compute intermolecular free-length (Lf) for the systems under investigation. The computed values of Lf have then been compared with the values estimated from well-established thermodynamic and ultrasonic methods. To the best of our knowledge, this investigation is a pioneering attempt in evaluation of intermolecular free-length involving multicomponent liquid mixtures by making use of thermoacoustical parameters.
Introduction
New interest has been revived in the study of thermoacoustical parameters in the view of temperature and pressure coefficient of the bulk modulus. Several approaches are found in literature1 to evaluate thermoacoustical parameters for a wide variety of liquids. Pandey et al.2,3 made an effort to estimate these parameters for liquids over a wide range of temperature and pressure, and to analyse the interrelationship among various thermoacoustical properties. Tabhane et al.4 used expansivity data to compute thermoacoustical parameters in binary liquid mixtures. Very recently, Pandey et al.1 determined the available volume of few liquids and binary mixture of acetone + methyl iodide at varying temperatures by computing some of the thermoacoustical parameters.
Available volume is related to another very useful quantity, intermolecular free-length (Lf), which has been extensively used in literature5–7 to study the intermolecular interactions in mixtures. The present paper is a continuation of our previous work1 involving computation of available volume of few pure organic liquids and a binary liquid mixture, and aims to compute thermoacoustical parameters for four ternary and two quaternary liquid mixtures. Available volume, computed from thermoacoustical parameters, has been used to obtain intermolecular free-length of systems under consideration. The calculated values of Lf are compared with the values estimated from well-established thermodynamic and ultrasonic methods.8 To the best of our knowledge, this is the first time when intermolecular free-length has been evaluated using thermoacoustical parameters, and compared with the values obtained by other methods.
Theoretical
The Moelwyn-Hughes parameter (C1) and reduced molar volume (Ṽ) may be expressed as, | 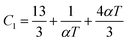 | (1) |
| 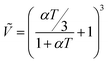 | (2) |
Here, α represents thermal expansion coefficient.
The isochoric temperature coefficient of internal pressure, X, is given by
| 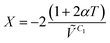 | (3) |
Expression of isothermal (K′), isochoric (K″) and isobaric (K) acoustical parameters1 are
| 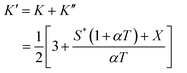 | (4) |
|  | (5) |
and
| 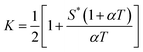 | (6) |
where
The isothermal acoustical parameter (K′) is related to the available molar volume (Va) of the substance as,1
| 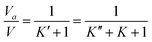 | (7) |
According to Schaaffs,8Va can be expressed as,
|  | (8) |
where u is the ultrasonic velocity at temperature T and u∞ is 1600 m s−1.
Thermodynamically,8 the value of Va can be calculated from the following equation:
| 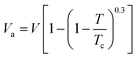 | (9) |
where Tc is the critical temperature. In the present work, the critical temperature of systems under consideration is supposed to be mole fraction additive of the values of its pure components.
The intermolecular free-length (Lf) is given by the relation,8
|  | (10) |
where Va represents the available volume per mole and Y is the surface area per mole given by
and
|  | (12) |
Here, N is the Avogadro number and V0 and V are the molar volumes at zero temperature and at temperature T, respectively.
Eqns. (1) to (7), (8) and (9) have been employed to obtain the available volume in the case of the thermoacoustical, ultrasonic and thermodynamic methods, respectively. Using the computed values of available volume, intermolecular free-lengths of liquid mixtures have been estimated, videeqns. (10) to (12).
Results and discussion
Four ternary systems, viz. benzene + chloroform + cyclohexane (I), toluene + chloroform + cyclohexane (II), chlorobenzene + chloroform + cyclohexane (III) and dioxane + chloroform + cyclohexane (IV) at 303.15 K, and two quaternary systems, viz. n-pentane + n-hexane + cyclohexane + benzene (V) and n-pentane + n-hexane + benzene + toluene (VI) at 298.15 K, have been considered for the present investigation. The experimental data of systems under consideration have been taken from the literature.9,10 The data of contributory pure components required for computation are given in Table 1. The coefficients of thermal expansivity, α, of the systems under investigation have been calculated by a method given elsewhere.11 The intermolecular free-lengths (Lf), evaluated through three different methods, are recorded in Tables 2 and 3 for ternary and quaternary mixtures, respectively. A comparative study of the three methods has also been presented graphically in Figs. 1 and 2 for ternary and quaternary systems, respectively. The average percentage values (APD) of thermoacoustical and ultrasonic methods with respect to the thermodynamic method for the four ternary and two quaternary systems are found to be −2.9, −47.2 (System (I)); −5.6, −48.6 (System (II)); −9.7, −53.9 (System (III)); −5.2, −45.9 (System (IV)); −3.0, −13.0 (System (V)) and −5.8, −14.4 (System (VI)).
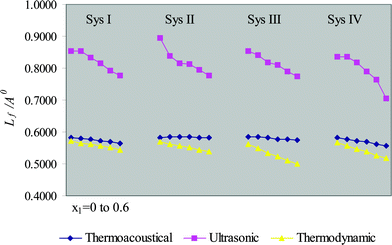 |
| Fig. 1 Intermolecular free-length (Lf) vs. mole fraction of first component (x1) for ternary systems. | |
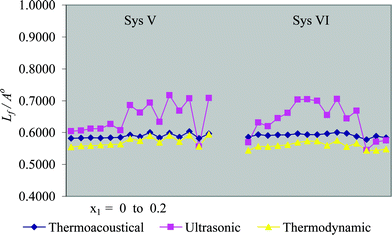 |
| Fig. 2 Intermolecular free-length (Lf) vs. mole fraction of first component (x1) for quaternary systems. | |
Table 1 Parameters of pure components
Components |
T/K |
T
c/K |
ρ/g cm−3 |
u/m s−1 |
α 103/K−1 |
Cyclohexane |
303.15 |
553.50 |
0.7662 |
1230.3 |
1.2390 |
Chloroform |
303.15 |
536.40 |
1.4665 |
966.6 |
1.3000 |
Benzene |
303.15 |
562.05 |
0.8677 |
1278.3 |
1.3200 |
Toluene |
303.15 |
591.75 |
0.8539 |
1285.3 |
1.0650 |
Chlorobenzene |
303.15 |
632.40 |
1.0957 |
1250.0 |
0.9900 |
Dioxane |
303.15 |
587.00 |
1.0185 |
1324.7 |
1.1150 |
n-Pentane |
298.15 |
469.60 |
0.6215 |
991.2 |
1.6226 |
n-Hexane |
298.15 |
507.40 |
0.6552 |
1076.2 |
1.3897 |
Cyclohexane |
298.15 |
553.40 |
0.7736 |
1253.0 |
1.2150 |
Benzene |
298.15 |
562.09 |
0.8783 |
1295.0 |
1.2265 |
Toluene |
298.15 |
591.70 |
0.8626 |
1304.3 |
1.0740 |
Table 2 Intermolecular free-length of ternary liquid mixtures at 303.15 K
x
1
|
x
2
|
ρ/g cm−3 |
u/m s−1 |
L
f/Å |
Thermoacoustical |
Ultrasonic |
Thermodynamic |
Benzene(1)
+ chloroform (2)
+ cyclohexane (3) |
0.1015 |
0.3019 |
0.9453 |
1124.6 |
0.5831 |
0.8534 |
0.5707 |
0.2019 |
0.2990 |
0.9574 |
1121.9 |
0.5793 |
0.8539 |
0.5654 |
0.2998 |
0.2993 |
0.9656 |
1128.6 |
0.5766 |
0.8344 |
0.5612 |
0.4001 |
0.3002 |
0.9813 |
1134.0 |
0.5725 |
0.8162 |
0.5557 |
0.5045 |
0.2983 |
0.9963 |
1141.8 |
0.5683 |
0.7928 |
0.5500 |
0.6031 |
0.2976 |
1.0119 |
1146.3 |
0.5643 |
0.7771 |
0.5446 |
Toluene (1)
+ chloroform (2)
+ cyclohexane (3) |
0.0911 |
0.3685 |
0.9815 |
1106.4 |
0.5828 |
0.8960 |
0.5684 |
0.2198 |
0.2939 |
0.9478 |
1138.8 |
0.5858 |
0.8377 |
0.5618 |
0.3004 |
0.3036 |
0.9627 |
1142.9 |
0.5843 |
0.8155 |
0.5562 |
0.4013 |
0.3026 |
0.9691 |
1144.8 |
0.5841 |
0.8116 |
0.5504 |
0.5016 |
0.2999 |
0.9813 |
1151.9 |
0.5825 |
0.7942 |
0.5435 |
0.5976 |
0.3035 |
0.9948 |
1158.7 |
0.5811 |
0.7779 |
0.5373 |
Chlorobenzene (1)
+ chloroform (2)+ cyclohexane (3) |
0.1001 |
0.3034 |
0.9659 |
1126.4 |
0.5853 |
0.8535 |
0.5621 |
0.1988 |
0.3034 |
0.9998 |
1131.5 |
0.5835 |
0.8401 |
0.5487 |
0.3150 |
0.2875 |
1.0326 |
1141.0 |
0.5816 |
0.8170 |
0.5337 |
0.4005 |
0.2994 |
1.0768 |
1141.7 |
0.5782 |
0.8114 |
0.5219 |
0.5023 |
0.2982 |
1.1116 |
1151.2 |
0.5763 |
0.7888 |
0.5101 |
0.5997 |
0.3007 |
1.1488 |
1156.4 |
0.5741 |
0.7755 |
0.4990 |
Dioxane (1)
+ chloroform (2)+ cyclohexane (3) |
0.1023 |
0.2998 |
0.9589 |
1132.0 |
0.5819 |
0.8353 |
0.5656 |
0.2024 |
0.3000 |
0.9818 |
1129.9 |
0.5779 |
0.8346 |
0.5566 |
0.2981 |
0.3011 |
1.0132 |
1134.0 |
0.5724 |
0.8179 |
0.5466 |
0.3991 |
0.2912 |
1.0328 |
1144.5 |
0.5686 |
0.7897 |
0.5378 |
0.5001 |
0.3007 |
1.0784 |
1152.4 |
0.5616 |
0.7633 |
0.5267 |
0.6046 |
0.2966 |
1.1108 |
1177.3 |
0.5562 |
0.7043 |
0.5169 |
Table 3 Intermolecular free-length of quaternary liquid mixtures at 298.15 K
x
1
|
x
2
|
x
3
|
ρ/g cm−3 |
u/m s−1 |
L
f/Å |
Thermoacoustical |
Ultrasonic |
Thermodynamic |
n-Pentane (1)
+ n-hexane (2)
+ cyclohexane (3)
+ benzene (4) |
0.0488 |
0.1238 |
0.1831 |
0.8051 |
1240.1 |
0.5822 |
0.6046 |
0.5550 |
0.0658 |
0.1078 |
0.2036 |
0.8004 |
1239.9 |
0.5832 |
0.6059 |
0.5573 |
0.0813 |
0.0934 |
0.2238 |
0.7988 |
1237.2 |
0.5834 |
0.6115 |
0.5588 |
0.1006 |
0.0778 |
0.2430 |
0.7995 |
1236.6 |
0.5830 |
0.6122 |
0.5601 |
0.1180 |
0.0629 |
0.2615 |
0.7941 |
1230.2 |
0.5842 |
0.6263 |
0.5627 |
0.1243 |
0.0466 |
0.2842 |
0.7908 |
1240.1 |
0.5849 |
0.6070 |
0.5632 |
0.1410 |
0.1304 |
0.3129 |
0.7709 |
1205.4 |
0.5921 |
0.6862 |
0.5805 |
0.1560 |
0.1262 |
0.1513 |
0.7828 |
1213.5 |
0.5866 |
0.6627 |
0.5747 |
0.1285 |
0.1192 |
0.5888 |
0.7549 |
1206.2 |
0.6001 |
0.6940 |
0.5897 |
0.1537 |
0.0925 |
0.1685 |
0.7906 |
1226.4 |
0.5842 |
0.6355 |
0.5691 |
0.1649 |
0.1013 |
0.5177 |
0.7547 |
1194.3 |
0.5985 |
0.7175 |
0.5910 |
0.1368 |
0.1258 |
0.1507 |
0.7851 |
1210.5 |
0.5861 |
0.6686 |
0.5712 |
0.0910 |
0.1721 |
0.6137 |
0.7509 |
1201.6 |
0.6030 |
0.7075 |
0.5920 |
0.0649 |
0.1378 |
0.1103 |
0.8048 |
1263.7 |
0.5815 |
0.5570 |
0.5567 |
0.1810 |
0.1656 |
0.2971 |
0.7542 |
1197.4 |
0.5968 |
0.7083 |
0.5944 |
n-Pentane (1)
+ n-hexane (2)
+ benzene (3)
+ toluene (4) |
0.0943 |
0.0918 |
0.4587 |
0.8216 |
1260.1 |
0.5843 |
0.5689 |
0.5438 |
0.1300 |
0.1373 |
0.2974 |
0.8011 |
1232.2 |
0.5936 |
0.6312 |
0.5565 |
0.1278 |
0.1288 |
0.3589 |
0.8054 |
1237.3 |
0.5908 |
0.6198 |
0.5551 |
0.1450 |
0.1291 |
0.3376 |
0.7991 |
1225.7 |
0.5924 |
0.6452 |
0.5588 |
0.1492 |
0.1384 |
0.3421 |
0.7974 |
1217.9 |
0.5925 |
0.6614 |
0.5611 |
0.1843 |
0.1484 |
0.2711 |
0.7855 |
1200.1 |
0.5963 |
0.7032 |
0.5693 |
0.1823 |
0.1640 |
0.3613 |
0.7838 |
1197.9 |
0.5940 |
0.7047 |
0.5729 |
0.1819 |
0.1606 |
0.3842 |
0.7824 |
1200.1 |
0.5937 |
0.6996 |
0.5732 |
0.1250 |
0.1665 |
0.2455 |
0.7975 |
1223.1 |
0.5960 |
0.6548 |
0.5592 |
0.1691 |
0.2041 |
0.2218 |
0.7769 |
1201.3 |
0.6001 |
0.7051 |
0.5752 |
0.1866 |
0.0826 |
0.1250 |
0.7995 |
1229.3 |
0.5974 |
0.6438 |
0.5559 |
0.1372 |
0.1580 |
0.5548 |
0.7926 |
1211.4 |
0.5875 |
0.6686 |
0.5662 |
0.0660 |
0.1053 |
0.7033 |
0.8273 |
1268.1 |
0.5776 |
0.5453 |
0.5436 |
0.0524 |
0.1434 |
0.4201 |
0.8176 |
1260.5 |
0.5885 |
0.5714 |
0.5441 |
0.1568 |
0.0468 |
0.4582 |
0.8184 |
1256.3 |
0.5843 |
0.5749 |
0.5482 |
A close observation of Tables 2 and 3 and Figs. 1 and 2 clearly illustrates a fairly good agreement in the values of intermolecular free-length, computed from thermoacoustical and thermodynamic methods. The credibility of the results is further enhanced by the APD (average percentage deviation) values of the six systems under consideration. The values obtained from thermoacoustical method lie in between the values of thermodynamic and ultrasonic methods. The values computed from ultrasonic method seem to be on the higher side, as compared to those determined from thermoacoustical and thermodynamic methods. The higher values obtained with ultrasonic method over thermodynamic method have also been observed for liquid methane and liquid tetrafluoromethane.8 The superiority of thermodynamic method over ultrasonic method has been established in literature.8 The thermodynamic approach appears to be good for the estimation of Lf, provided T is not very close to TC. The ultrasonic method fails completely when the ultrasonic velocity in liquids or liquid mixtures exceeds 1600 m s−1, since at T > TC the right hand side of eqn. (8) disappears, and when u > u∞, the right hand side of eqn. (9) vanishes. Therefore, the thermoacoustical method is found to be more effective than the other two methods. It is also evident from the tables that Lf decreases with increasing mole fraction of the first component for all systems, while, ultrasonic velocity exhibits the reverse trend. The variation of ultrasonic velocity is related to free-length. The increase in ultrasonic velocity and corresponding decrease in free-length for all the systems under study, resembles the view proposed by Eyring and Kincaid,12 according to which the ultrasonic velocity increases with decrease in free-length and vice versa. The increases or decreases in the values of ultrasonic velocity and intermolecular free-length with composition, for all the systems studied, indicate interactions between contributing molecules.7
In conclusion, the present investigation throws light on the evaluation of thermoacoustical parameters in multicomponent systems, and the determination of intermolecular free-length through three different methods. The present approach is found to be accurate and simple for computation of intermolecular free-length, and thus, provides a novel pathway for meaningful study of intermolecular interactions in liquid mixtures.
Acknowledgements
One of the authors (J. C.) wishes to acknowledge the financial assistance provided by CSIR-New Delhi, India.
References
- J. D. Pandey, R. Dey and B. Datt Bhatt, PhysChemComm, 2002, 5(6), 37 RSC.
- J. D. Pandey, G. P. Dubey, R. Dey and S. N. Dubey, Acta Acust., 1997, 83, 90 Search PubMed.
- J. D. Pandey, V. Vyas, R. Dey and D. K. Dwivedi, Indian J. Chem., 2000, 39(A), 993 Search PubMed.
- V. A. Tabhane, S. Ghosh and S. Agrawal, J. Pure Appl. Ultrason., 1999, 21, 122 Search PubMed.
- P. S. Nikam, V. M. Kapade and M. Hasan, J. Pure Appl. Ultrason., 2000, 22, 16 Search PubMed.
- A. Pal, G. Dass and H. Kumar, J. Pure Appl. Ultrason., 2001, 23, 10 Search PubMed.
- A. Ali, K. Tiwari, A. K. Nain and V. Chakravortty, Indian J. Phys., 2000, 74B(5), 351 Search PubMed.
- J. D. Pandey and S. N. Srivastava, Acustica, 1982, 51, 66 Search PubMed.
- V. Rajendran, Indian J. Pure Appl. Phys., 1994, 32, 19 Search PubMed.
- J. D. Pandey, R. D. Rai, R. K. Shukla and A. K. Shukla, Indian J. Pure Appl. Phys., 1993, 31, 84 Search PubMed.
- J. D. Pandey, S. B. Tripathi and V. Sanguri, J. Mol. Liq., 2002, 100(2), 153 CrossRef CAS.
- H. Eyring and J. F. Kincaid, J. Chem. Phys., 1938, 6, 620 CrossRef.
|
This journal is © The Royal Society of Chemistry 2003 |