DOI:
10.1039/B100020I
(Paper)
CrystEngComm, 2001,
3, 27-29
Crystallization from hydrochloric acid affords the solid-state structure of croconic acid (175 years after its discovery) and a novel hydrogen-bonded network
Received
2nd January 2001
, Accepted 11th January 2001
Abstract
The structure of croconic acid is determined on single crystals grown from a solution of HCl 1 M; it is shown that O–H⋯O interactions organize the H2C5O5 molecules in an ‘accordion’ type supramolecular arrangement of hydrogen-bonded molecules; the stacking of [Cl]−·H2C5O5 units in the organometallic co-crystal [(η5-C5H5)2Co][Cl]·H2C5O5 is also analysed.
Introduction
Gmelin reported in 1825 the preparation of the ion C5O52− and of the corresponding acid H2C5O5. This acid he called croconic acid because of its yellow colour.1 The dianion C5O52− belongs to the family of oxocarbon anions that include the deltate, squarate, and rhodizonate anions.2 The interest in these anions was originated by the view that cyclic oxocarbon anions of the general formula CnOn2− might show aromaticity stabilised by electron delocalisation of the π electrons around the ring.3 It is worth recalling that the isolation by Gmelin of croconic acid and of the croconate dianion is contemporary to the isolation by Faraday of benzene, the prototype of aromatic molecules. While the solid state structures of deltic4 and squaric acid5 were established a long time ago, those of the heavier members remain unknown. What could be the reason for this ‘absence’ from the vast repertoire of organic molecules characterised by diffraction methods6 is hard to say. The crystallisation of croconic acid by us is the result of our systematic quest for new building blocks to be utilised in the crystal engineering7 of hybrid organic–organometallic materials.8
Results and discussion
Yellow, transparent, single crystals of croconic acid 1 were obtained from crystallisation of croconic acid from an aqueous solution of HCl 1 M.9 The co-crystal [(η5-C5H5)2Co][Cl]·H2C5O52 was obtained via reaction of rhodizonic acid H2C6O6 with the hydroxide [(η5-C5H5)2Co][OH], in order to attain acid ring contraction and formation of the croconate dianion,10 followed by acidification of the solution with HCl.9 The structures of 1 and 2 were determined by single crystal X-ray diffraction (Table 1). Both structures possess some remarkable features which will be summarised in the following.
Properties |
1
b
|
2
c
|
Formula |
C2H2O5 |
C15H12CoClO5 |
Click b100020i.txt for full crystallographic data (CCDC nos. 147324–147325).
The hydrogen atoms in 1, directly located from the Fourier maps, are responsible for the reduction of symmetry from space group Pbca to Pca21. Data were collected on a Bruker AXS SMART diffractometer.
Data collected on a Nonius CAD4 diffractometer equipped with an Oxford Cryostream liquid N2 device. Both diffractometers equipped with a graphite monochromator (Mo-Kα radiation, λ⊕=⊕0.71073 Å). SHELXS-9711 and SHELXL9711 were used for structure solution and refinement based on F2. SCHAKAL9712was used for the graphical representation of the results.
|
T/K |
273(2) |
223(2) |
M
|
142.07 |
366.63 |
Crystal system |
Orthorhombic |
Triclinic |
Space group |
Pca21 |
P![[1 with combining macron]](https://www.rsc.org/images/entities/char_0031_0304.gif) |
a/Å |
8.7108(8) |
6.807(3) |
b/Å |
5.1683(5) |
10.787(3) |
c/Å |
10.9562(9) |
11.629(7) |
α/° |
90 |
67.52(4) |
β/° |
90 |
73.26(5) |
γ/° |
90 |
87.84(5) |
V/Å3 |
493.25(8) |
753.0(6) |
Z
|
4 |
2 |
F(000) |
288 |
372 |
μ/mm−1 |
0.179 |
1.336 |
θ/° |
4–34 |
3–30 |
Number of reflections (independent) |
6525 (1838) |
4581 (4381) |
Refinement on F2
(number of parameters) |
91 |
181 |
wR (F2, all refls.) |
0.1240 |
0.2035 |
R
1 (I
⊕>⊕2σ(I)) |
0.0549 |
0.0586 |
Fig. 1a shows the hydrogen-bonded network in crystalline 1. Since hydrogen atom positions could be observed from the Fourier maps it is possible to examine the hydrogen bond patterns in some detail. Each molecule of croconic acid is linked to four molecules by two pairs of hydrogen bonds forming sheets of large tetrameric rings. What makes this structure rather unusual is, however, the way the hydrogen-bonded sheets are arranged in three dimensions. Fig. 1c shows how the sheets are pleated in an ‘accordion’ pattern and shifted to avoid overlap between molecules in different layers. The angle between croconic acid planes is 69.90 deg with the hinges constituted of O–H⋯O units as shown in Fig. 1b. It is interesting to note that squaric acid also forms large tetramolecular rings in the solid state, but the sheets are flat with each molecule of squaric acid placed above and below the centres of the tetramolecular rings. The hydrogen bonds in 1
[O(H)⋯O 2.628(5), 2.617(5) Å] are slightly longer than in squaric acid [O(H)⋯O 2.532(4), 2.544(4) Å].
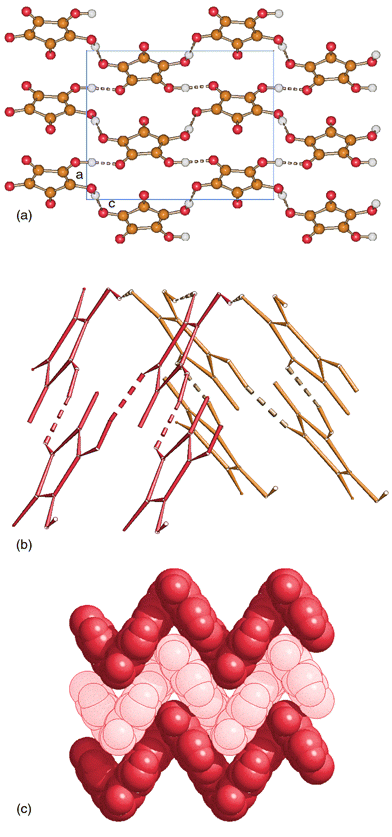 |
| Fig. 1
a) A pleated sheet, extending in the ac-plane, formed by hydrogen-bonded molecules of croconic acid in crystalline 1. Click image or fig1.htm to access a 3D representation. b) The hinge of the ‘accordion’ is defined by O–H⋯O bonds. c) Space-filling representation down the c-axis of the ‘accordion’ type arrangement adopted by the croconic acid sheets.
| |
Crystalline 2 was obtained in an attempt to intercalate croconic acid moieties between the cyclopentadienyl rings of the cobalticinium cation. The idea stemmed from our recently reported success in intercalating hydrogen squarate anions between [(η5-C5H5)2Co]+ and [(η6-C6H6)2Cr]+ cations.13 Since direct utilisation of croconic acid failed to give the desired products, we attempted in situ preparation of the hydrogen croconate anion by exploiting the well established rhodizonate ring contraction upon treatment with a base, presently the [(η5-C5H5)2Co][OH] hydroxide. The addition of HCl to the C5O52− anion thus formed, however, results in a strong interaction of the chloride anion with croconic acid, leading to crystallisation of [(η5-C5H5)2Co][Cl]·H2C5O5. Fig. 2a is a space-filling representation of how the acid H2C5O5 chelates the chloride anion by means of a twin O–H⋯Cl hydrogen bond [O(H)–Cl− distances 2.986(5) and 2.939(5) Å]. In addition to this, the whole [Cl]−·H2C5O5 system is encapsulated within a system of six cobalticinium cations, which interact via C–H⋯O interactions [(C)H⋯O distances shorter than 2.4 Å: 2.195(6), 2.182(6) Å, 2.347(6) and 2.376(6) Å] with the available O-atoms’ lone-pairs on the acid molecules (see Fig. 2a). The anions alternate along the pile resulting in a sort of interdigitation of croconic acid molecules between chloride anions as shown in Fig. 2b. The distance between [Cl]−·H2C5O5 planes is ca. 3.3 Å. Finally, it is worth noting that the croconic acid molecule interacting with the chloride anion in 2 shows syn arrangement of the protons while the conformation is anti in crystalline 1 and differs slightly, but appreciably, in terms of molecular geometry in the two crystalline forms, as shown in Table 2. These differences should be ascribed to the different intermolecular interactions established by the croconic acid in crystalline 1 and 2. The difference reflects the different intermolecular interactions involving the OH groups in the two systems.
Table 2
Relevant structural parameters for the croconic acid molecules in 1 and 2
(Å, deg)
|
1
|
2
|

|
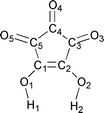
|
Distances based on observed hydrogen atom positions.
|
C1–O1 |
1.295(4) |
1.329(8) |
C2–O2 |
1.306(4) |
1.325(8) |
C3–O3 |
1.233(5) |
1.206(8) |
C4–O4 |
1.213(4) |
1.175(10) |
C5–O5 |
1.205(2) |
1.208(8) |
C1–C2 |
1.382(5) |
1.364(9) |
C2–C3 |
1.442(3) |
1.435(10) |
C3–C4 |
1.508(6) |
1.520(11) |
C4–C5 |
1.518(6) |
1.515(11) |
C5–C1 |
1.466(6) |
1.453(10) |
O1–H1a |
0.933 |
0.889 |
O2–H2a |
1.002 |
0.995 |
O1–C1–C2 |
122.1(4) |
130.6(7) |
O2–C2–C1 |
128.9(4) |
129.4(7) |
![a) Space-filling representation of crystalline 2 along the a axis, showing the chelation of the Cl− anion (green balls) by the croconic acid. b) A side-view of the [Cl]−·H2C5O5 pile surrounded by the cobalticinium cations. H(cp) omitted for clarity. Click image or here to access a 3D representation.](/image/article/2001/CE/b100020i/b100020i-f2.gif) |
| Fig. 2
a) Space-filling representation of crystalline 2 along the a axis, showing the chelation of the Cl− anion (green balls) by the croconic acid. b) A side-view of the [Cl]−·H2C5O5 pile surrounded by the cobalticinium cations. H(cp) omitted for clarity. Click image or fig2.htm to access a 3D representation.
| |
Summary
The quest for new synthons14 to be utilised in crystal engineering has afforded some new insights into a piece of structural chemistry that goes back to the early days of organic chemistry. Crystallisation of croconic acid alone or in its co-crystal 2 has been possible only from acidic solutions.
Acknowledgements
We thank M. U. R. S. T. (projects Supramolecular Devices 1999–2000 and Solid Supermolecules 2000–2001) and the Universities of Bologna (project Innovative Materials) and Sassari for financial support.
Notes and references
- ‘Von τó
χρóχoν, der Safran, oder das Eigelb, wegen der gelben und rothgelben Farbe der Krokonsäure und vieler ihrer Verbindungen. Sollte es sich bestätigen, daß sie eine Wasserstoffsäure ist, so wäre sie Hydrokrokonsäure zu nennen und ihr Radical: Krokon.’
(a) L. Gmelin, Ann. Phys. Chem., 1825, 4, 31 Search PubMed
(from the Greek τó
χρóχoν, saffron or yalk, because of the yellow and orange colour of the croconic acid and of many of its compounds. Were it confirmed that it is a hydroxy acid, then it should be given the name of hydrocroconic acid and its radical should be called crocon)..
-
(a)
Oxocarbons, ed. R. West, Academic Press, New York, 1980. Search PubMed;
(b) F. Serratosa, Acc. Chem. Res., 1983, 16, 170 CrossRef CAS;
(c) P. R. Schleyer, K. Najafian, B. Kiran and H. Jiao, J. Org. Chem., 2000, 65, 426 CrossRef.
-
(a) R. West and D. L. Powell, J. Am. Chem. Soc., 1963, 82, 6204;
(b) J. Aihara, J. Am. Chem. Soc., 1981, 103, 1633 CrossRef CAS.
- D. Semmingsen and P. Groth, J. Am. Chem. Soc., 1987, 109, 7238 CrossRef CAS.
- Y. Wang, G. D. Stucky and J. M. Williams, J. Chem. Soc., Perkin Trans. 2, 1974, 35, 2 Search PubMed.
- The number of organic compounds in the April 2000 version of the Cambridge Structural Database is ca. 97
000..
-
(a)
Crystal Engineering: from Molecules and Crystals to Materials, ed. D. Braga, F. Grepioni and A. G. Orpen, Kluwer Academic Publishers, Dordrecht, 1999. Search PubMed;
(b) D. Braga, F. Grepioni and G. R. Desiraju, Chem Rev., 1998, 98, 1375 CrossRef CAS;
(c) See also the perspective article in the Dalton Discussion Inorganic Crystal Engineering, S. Mann, J. Chem. Soc., Dalton Trans., 2000, 3753 Search PubMed and references therein.
-
(a) D. Braga, C. Bazzi, L. Maini and F. Grepioni, CrystEngComm, 1999, 5 RSC;
(b) D. Braga and F. Grepioni, J. Chem. Soc., Dalton Trans., 1999, 1 RSC;
(c) D. Braga, L. Maini, F. Grepioni, A. DeCian, O. Felix, J. Fisher and M. W. Hosseini, New. J. Chem., 2000, 24, 547 RSC;
(d) D. Braga and F. Grepioni, Acc. Chem. Res., 2000, 33, 601 CrossRef CAS.
-
1: Croconic acid purchased from Aldrich was dissolved in HCl 1 M. Yellow crystals suitable for X-ray diffraction were obtained from slow evaporation of the solution. 2: 100 mg (0.53 mmol) of cobaltocene were oxidised in 15 ml of water in air, then 107 mg (0.53 mmol) of rhodizonic acid were added under stirring; after 3 h HCl 1 M was added dropwise until pH⊕=⊕1 was reached. Crystals suitable for X-ray diffraction were obtained by slow evaporation of the solution..
- R. Nietzki, Ber. Dtsch. Chem. Ges., 1887, 20, 1617 Search PubMed.
-
G. M. Sheldrick, SHELXL97, Program for Crystal Structure Determination; University of Göttingen: Göttingen, Germany, 1997. Search PubMed.
-
E. Keller, SCHAKAL97 Graphical Representation of Molecular Models; University of Freiburg, Germany, 1997. Search PubMed.
- D. Braga, L. Maini, L. Prodi, A. Caneschi, R. Sessoli and F. Grepioni, Chem. Eur. J., 2000, 6, 1310 CrossRef CAS.
- G. R. Desiraju, Angew. Chem., Int. Ed. Engl., 1995, 34, 2311 CrossRef CAS.
|
This journal is © The Royal Society of Chemistry 2001 |