Discovery of potent and selective activity-based probes (ABPs) for the deubiquitinating enzyme USP30†
Received
29th January 2024
, Accepted 7th March 2024
First published on 13th March 2024
Abstract
Ubiquitin-specific protease 30 (USP30) is a deubiquitinating enzyme (DUB) localized at the mitochondrial outer membrane and involved in PINK1/Parkin-mediated mitophagy, pexophagy, BAX/BAK-dependent apoptosis, and IKKβ-USP30-ACLY-regulated lipogenesis/tumorigenesis. A USP30 inhibitor, MTX652, has recently entered clinical trials as a potential treatment for mitochondrial dysfunction. Small molecule activity-based probes (ABPs) for DUBs have recently emerged as powerful tools for in-cell inhibitor screening and DUB activity analysis, and here, we report the first small molecule ABPs (IMP-2587 and IMP-2586) which can profile USP30 activity in cells. Target engagement studies demonstrate that IMP-2587 and IMP-2586 engage active USP30 at nanomolar concentration after only 10 min incubation time in intact cells, dependent on the presence of the USP30 catalytic cysteine. Interestingly, proteomics analyses revealed that DESI1 and DESI2, small ubiquitin-related modifier (SUMO) proteases, can also be engaged by these probes, further suggesting a novel approach to develop DESI ABPs.
Introduction
Decoration of proteins with the small protein modifier ubiquitin (Ub) is a reversible post translation modification (PTM) that regulates almost all cellular functions including proteolysis, transcriptional regulation, cellular trafficking, localization, inflammation, and autophagy.1 Hundreds of Ub ligases and deubiquitinases (DUBs) are involved in the addition or removal of ubiquitin, respectively, and modulation of this system has emerged as an important therapeutic strategy across many diseases.2 Ubiquitin specific peptidase 30 (USP30) is a member of the USP DUB family, which typically harbours a Cys-His-Asp catalytic triad.3 USP30 is the only DUB known to be present in the outer mitochondrial membrane due to its unique transmembrane domain.4 USP30 deubiquitylates specific mitochondrial proteins preferentially by cleaving Lys6-linked ubiquitin chains and opposes mitophagy driven by the E3 ligase Parkin.5–8 It has recently been shown that USP30 can also antagonise basal mitophagy mediated by the Parkinson's disease-associated kinase PINK1, even in cells which do not express Parkin.9 Overexpression of USP30 and dysregulation in mitochondrial turnover has been associated with neurodegenerative diseases including Parkinson's disease, Alzheimer's disease and motor neuron disease.10–12 USP30 may also play a role in drug resistant lymphoma, leukaemia, multiple myeloma, and BAX/BAK-dependent apoptosis.3 USP30 depletion sensitizes cancer cells to BH3-mimetics (e.g., ABT-737), making it a potential target for cancer therapy.8 The USP30 inhibitor MTX652 entered clinical trials recently for Acute Kidney Injury, after exhibiting protective outcomes in various preclinical models.13 Despite being important therapeutic target and ongoing clinical trials of USP30 inhibitors, the endogenous substrates of USP30 have yet to be firmly established in vivo and regulation of its activity remains only partly understood.
Ub-derived activity-based probes (Ub-ABPs) bearing varied electrophilic warheads have been explored to better understand the function and mechanism of DUBs as well as for DUB inhibitor screening.11 While these Ub-ABPs are widely used and have greatly expanded our knowledge of DUB biology, they can be applied only in cell lysate due to their lack of cell permeability.14 In contrast, ABPs based on small molecules offer complementary properties since they are readily cell permeable, and whilst selective probes are challenging to develop they can be powerful tools to profile DUB activity in live cells, which is helpful to uncover their function in health and disease.15,16 Moreover, they have the potential to identify off-targets of structurally-related DUB inhibitors, which is not possible with highly DUB-specific Ub-ABPs.17
Several USP30 inhibitors based on N-cyanopyrrolidine (CNPy), oxospiramilactone, benzenesulfonamides, and naphthylsulfonamide have been reported recently,18–20 and in the recent patent literature.21–25 However, no ABP specific for USP30 has been developed to date, limiting opportunities to profile USP30 activity in intact cells. Here we report the first small molecule ABPs which target USP30 in an activity-dependent manner and demonstrate their application to identify active DUBs in cancer cells by activity-based protein profiling (ABPP).
Results
To identify a suitable ABP scaffold for USP30, we considered several reported covalent USP30 inhibitors with low nanomolar potency, prioritising those bearing a CNPy warhead, expected to react with the DUB active cite cysteine residue to form an isothiourea adduct.16,26 We synthesised CNPy 1 (Fig. 1 and Scheme S1, ESI†) which has a reported IC50 of 1–10 nM against USP3025 (following 30 minutes incubation), and designed ABP (2, which we named IMP-2587) (Fig. 1 and Scheme 1) bearing a terminal alkyne functionality, based on reported structure–activity relationship (SAR) data suggesting that meta substitution on the aryl ring would retain activity, and compatibility with bioorthogonal click chemistry. IMP-2587 was synthesised through a short synthetic route (Scheme 1a). In parallel, we explored a CNPy with a different scaffold, synthesising the highly potent CNPy-bearing covalent inhibitor 3 (Fig. 1 and Scheme S1, ESI†) with reported IC50 value = 1.5 nM against USP3018 (following 30 minutes incubation), and high biochemical selectivity for USP30 among a diverse set of DUB family members, although with some appreciable off-target specificity against USP6 at higher concentration.18 We designed ABP IMP-2586 based on 3. For installation of the affinity handle (azide), the reported SAR supported para substitution at the terminal aryl ring, so we synthesised 4 by a short synthetic route (Scheme 1b). An azide bioorthogonal tag was preferred in this case due to the problematic instability of an alkyne in this relatively electron rich position during pyrrolidine Boc deprotection.
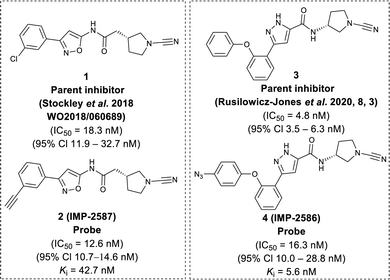 |
| Fig. 1 Chemical structure of parent compounds and activity-based probes with their determined IC50 and Ki accordingly. Fluorescent Polarisation assay were performed for IC50 (30 minutes incubation against USP30), kobs/I, and kinact/Ki determination of IMP-2587, and IMP-2586 against USP30 using Ub-Lys(TAMRA)-Gly (Fig. S1, ESI†). | |
 |
| Scheme 1 Synthetic route to the compounds described in this work. (a) synthetic route to compound 2 (IMP-2587); (b) synthetic route to compound 4 (IMP-2586). | |
We first confirmed biochemical inhibition of USP30 with our probes in a fluorescence polarisation (FP) assay using a TAMRA labelled Lys-Gly dipeptide linked to ubiquitin via an isopeptide bond (Ub-Lys(TAMRA)-Gly). USP30 (5 nM) was incubated across a concentration series of test compound for 30 min, followed by FP measurement after addition of Ub-Lys(TAMRA)-Gly (100 nM). IMP-2587 showed IC50 value of 12.6 nM (95% CI 10.7–14.7 nM) against USP30, whereas parent inhibitor 1 showed IC50 value of 18.3 nM (95% CI 11.9–32.7 nM). IMP-2586 displayed IC50 value of 16.3 nM (95% CI 10.0–28.8 nM), while the original inhibitor 3 showed IC50 value of 4.8 nM (95% CI 3.5–6.3 nM) (Fig. 1 and Fig. S1, ESI†). Cross-screening of IMP-2587 and IMP-2586 against an unrelated DUB (UCHL1) displayed no activity (Table S1, ESI†).
We further characterised inhibition kinetics for ABPs in a FP assay using Ub-Lys(TAMRA)-Gly. kobs/I, Ki and kinact determination assay was performed using 30 nM USP30 and 100 nM Ub-Lys(TAMRA)-Gly as described in ESI,† Section S3.1.c. FP was monitored kinetically over an hour after addition of the substrate, and response curves fitted to y = (vi/kobs) (1 − exp(−kobsx)) to calculate kobs/I, 5680 (95% Cl = 4723–6638) M−1 s−1 for IMP-2587, and 3021 (95% Cl = 2350–3693) M−1 s−1 for IMP-2586 (Fig. S1, ESI†). Ki and kinact were estimated by nonlinear regression to kobs = kinact/(1 + (Ki/x)) for probes IMP-2587 (Ki 42.8 nM, kinact 0.0014 s−1) and IMP-2586 (Ki 5.6 nM and kinact 0.00067 s−1), confirming robust covalent inhibition activity and affinity for these ABPs (Fig. S1, ESI†).
We next determined cellular engagement of endogenous USP30 with IMP-2587 or IMP-2586. HEK293T cells were treated with ABPs for 1 hour followed by western blot analysis of in-lysate competitive activity-based profiling against hemagglutinin-tagged Ub-vinyl methyl ester probe (HA-Ub-VME) (Fig. 2a). In-cell concentration-dependent competition by IMP-2587 and IMP-2586 for USP30 were confirmed below 100 nM probe (Fig. 2b and c), whilst engagement of an exemplar unrelated DUB, UCHL1, occurred only at a much higher concentration (30
000 nM). We hypothesise that incomplete modification of USP30 by HA-Ub-VME is likely due to limited retention of USP30 activity in cell lysates, which have likely had the USP30 transmembrane domain compromised, highlighting the potential utility of a small molecule USP30 ABP applicable directly in intact cells. Direct USP30 target engagement by IMP-2587 and IMP-2586 was confirmed in HEK293T cells following 1 h probe treatment followed by cell lysis and CuAAC bio-orthogonal ligation to capture reagent azido-TAMRA-biotin (AzTB) for IMP-2587 or alkyne-TAMRA-biotin for IMP-2586 (Fig. 3a).27 In-gel fluorescence revealed one major band at ca. 60 kDa for IMP-2587 starting as low as 3 nM probe, with some labelling of additional bands above 30 nM (Fig. 3b). Similarly, labelling with IMP-2586 revealed a major band at ca. 60 kDa, but in this case in-gel fluorescence showed a degree of unspecific labelling across all concentrations, including the vehicle (DMSO)-treated control (Fig. 3d). This unspecific background labelling is presumably due to the previously reported higher off-target CuAAC reactivity of an alkyne-bearing capture reagent, applied here due to incorporation of an azide tag in IMP-2586.28 Moreover, the labelling of USP30 was further confirmed by biotin pulldown and immunoblotting. USP30 was enriched at 10 nM probe and maximal at 100 nM, resulting in almost complete engagement and enrichment with both IMP-2587 and IMP-2586 (Fig. 3c and e), which was in stark contrast to the ∼50% USP30 engagement observed with the Ub-based probe, HA-Ub-VME (Fig. 2b and c).
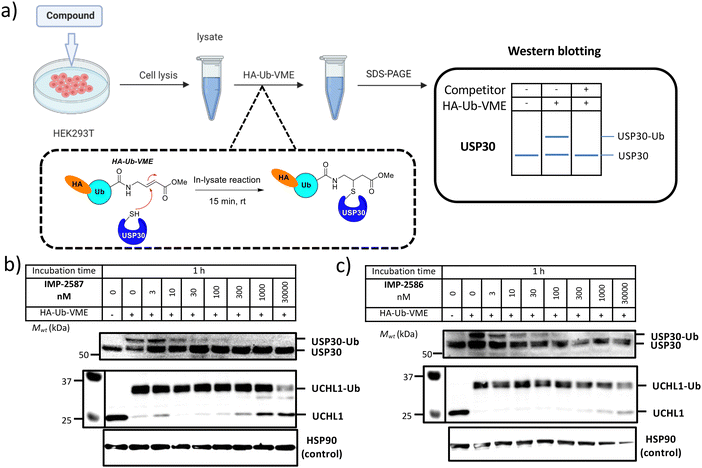 |
| Fig. 2 (a) Workflow of immunoblot analysis of HA-Ub-VME USP30 labelling following HEK293T treatment with IMP-2587 (b) and IMP-2586 (c) for 1 h. Dose-dependent competition for USP30 labelling occurs for both the ABPs but not with UCHL1 at similar concentration range. | |
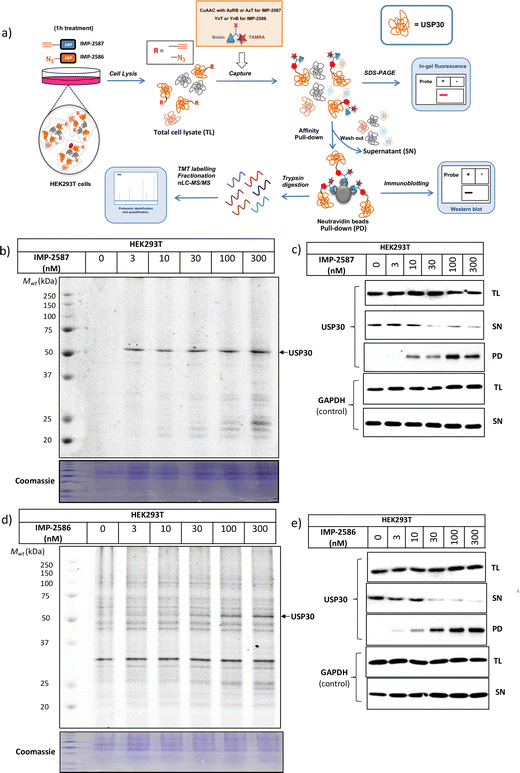 |
| Fig. 3 USP30 activity profiling using IMP-2587 and IMP-2586 in HEK293 cells. (a) Affinity pulldown and chemical proteomics workflow. Cells were incubated with IMP-2587 and IMP-2586 for 1 hour, separately and labelled proteins ligated via CuAAC to AzRB or AzT for IMP-2587 and YnB or YnT for IMP-2586 for in-gel fluorescence and/or affinity enrichment for immunoblotting and proteomics. Total cell lysates are labelled as TL samples; supernatants following affinity enrichment were collected as SN samples; protein bound to the beads was collected as PD samples. (b) In-gel fluorescence shows dose-dependent labelling by IMP-2587. (c) Probe-labelled (IMP-2587) protein identification by enrichment and immunoblotting for USP30. (TL: Total Lysate, SN: Supernatant, PD: Pull-Down) (d) In-gel fluorescence shows dose-dependent labelling by IMP-2586. (e) Probe-labelled (IMP-2586) protein identification by enrichment and immunoblotting for USP30. | |
To validate these probes as ABPs, we sought to demonstrate dependence on USP30 catalytic activity for cellular target engagement, and specificity for the active site Cys residue among the >20 Cys residues present in USP30. We expressed HA-tagged wild-type (WT) USP30 and active site cysteine to serine mutant (CS) in HEK293T cells (Fig. 4a). Cells were treated by IMP-2587 or IMP-2586 for 10 min or pre-treated with corresponding parent inhibitors for 1 hour followed by probe treatment. Western blot analysis of affinity enriched USP30 demonstrates that USP30 labelling for both probes requires the presence of the catalytic cysteine, and this enrichment can be readily outcompeted by the parent inhibitors (Fig. 4b and c), confirming the activity-dependence of these ABPs.
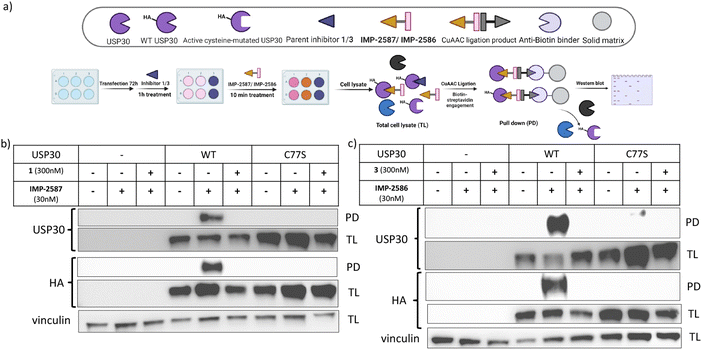 |
| Fig. 4 (a) Workflow of HA-tagged USP30 WT or C77S transfection system and IMP-2587/IMP-2586 labelling in HEK293T cells. HEK293T cells were transfected to overexpress HA-tagged USP 30 WT or catalytic CS mutants, and pulldown following treatment with IMP-2587 (b) or IMP-2586 (c) for 10 min. (PD: Pull-Down, TL: Total Lysate). | |
To explore the selectivity of IMP-2587 and IMP-2586 across the whole proteome, we performed quantitative activity-based protein profiling (ABPP) in HEK293T cells following treatment with IMP-2587 or IMP-2586 (Fig. 3a). After ABP incubation, cells were lysed, and probe-labelled proteins were ligated to biotinylated capture reagents.27 Incubation of cells with 30 nM probe for 10 min showed highly significant enrichment of USP30 (Fig. 5a and c and Tables S2 and S4, ESI†), consistent with results from in-gel fluorescence (Fig. 3) and pull-down data (Fig. 3 and 4). USP30 was outcompeted by corresponding parent inhibitors for each ABP (Fig. 5b and d and Tables S3 and S5, ESI†), confirming the high potency and rapid engagement of IMP-2587 and IMP-2586 in cells. Interestingly, we also observed less potent but still significant enrichment of two desumoylating isopeptidases (DESI1 and DESI2), which were further validated by pull-down immunoblot analyses (Fig. 5e–h). DESI1 and DESI2 are small ubiquitin-related modifier (SUMO) proteases,3,29–32 and IMP-2587 and IMP-2586 may offer a starting point for future development of DESI ABPs.
 |
| Fig. 5 Chemical proteomic analysis of IMP-2587 at 30 nM (a) or 100 nM (b) and IMP-2586 at 30 nM (c) or 100 nM (d) labelling in HEK293T cells. Volcano plots showing log2 difference (fold change) and significance (−log p-value) between protein enrichment compared to DMSO control (two sample t test, n = 3, permutation-based FDR = 0.05, S0 = 0.1). Black dots represent non-DUBs, red dots represent DUBs and blue dots represent DESIs. Probe-labelled protein identification by enrichment and immunoblotting in HEK293T cells for DESI1 with IMP-2587 (e) and IMP-2586 (f) treatment for 10 min or DESI2 with IMP-2587 (g) and IMP-2586 (h) treatment for 10 min. (TL: Total Lysate, PD: Pull-Down). | |
Discussion
Small molecule DUB ABPs circumvent the limitations of Ub-based ABPs, which can only be applied in cell lysates. Cell lysis may disrupt native DUB activity resulting in inconsistent and potentially misleading engagement by Ub-ABPs, as seen in the present study in the incomplete modification of USP30 by HA-Ub-VME. In contrast, recently developed pan-DUB small molecule ABPs enable exploration of DUB activity in intact cells, and assessment of cellular target engagement and selectivity of novel inhibitors.15,33
Dysregulation of USP30 is implicated in a range of rare genetic mitochondrial diseases, and neurodegenerative diseases including Parkinson's disease.12 Accordingly, there is significant interest in developing USP30 inhibitors for the clinic, and Mission Therapeutics initiated the first clinical trials of a USP30 inhibitor in 2022 for muscular, cardiac and kidney pathologies,34 reporting encouraging Phase I safety data. Selective and potent small molecule USP30 ABPs would be useful tools to explore the role of USP30 activity in intact cells for these diseases.
In this work, we designed, synthesised and validated two novel USP30 ABPs bearing a CNPy. Cyanoamines, particularly CNPy, have been established as a privileged warhead class for DUBs, which have proven challenging to target selectively with other warhead classes (e.g. chloroacetamides).3,16,26,33,35,36 Recent reports have shown that minimal CNPy probes lacking an extended structure lose activity toward DUBs, suggesting that the scaffold beyond the warhead plays a key role in enabling covalent DUB active site modification, and in selectivity within the DUB family.26 Interestingly, IMP-2586 and IMP-2587 showed very similar selectivity for USP30 in cells despite their divergent scaffolds. Unlike most reported DUB ABPs, IMP-2586 bears an azide tag rather than a terminal alkyne. Given the prevalence and convenience of Boc protecting group chemistry in CNPy synthetic routes, this design choice overcomes problems of acid instability of an electron rich alkyne in this position. Despite reduced CuAAC bioorthogonal ligation specificity using an alkyne capture reagent (Fig. 3), the utility of IMP-2586 as a selective USP30 ABP is preserved in cellular studies.
Our data suggest that both parent inhibitors from which IMP-2587 and IMP-2586 are derived also target DESI1 and DESI2 in cells and these may need to be considered as off-targets where related compounds are used as inhibitors. Like DUBs, DESIs are cysteine proteases, but catalyse hydrolysis of SUMO modifications rather than Ub.29 No probe or inhibitor has been reported to date for DESIs, although these enzymes are reported to be involved in a wide range of cellular pathways, including PI3K/AKT/mTOR signalling and P53-induced apoptosis.30,31 Future optimisation of IMP-2586 and IMP-2587 to minimise USP30 activity may provide the first ABPs for studying DESIs.
Conclusions
IMP-2586 and IMP-2587 enable sensitive and rapid detection of USP30 activity in intact cells at probe concentrations as low as 3–10 nM following 1 hour incubation, or 30 nM at 10 min incubation. Parent inhibitors 1 and 3, IMP-2587 and IMP-2586, also showed high inhibitory potency against USP30 with low nM IC50 and fast USP30 modification kinetics both biochemically and in cells. Low nanomolar cellular target engagement and selectivity were examined with a range of orthogonal approaches, including HA-Ub-VME ABP assays, and analysis of direct target engagement through CuAAC bio-orthogonal ligation, in-gel fluorescence, enrichment, immunoblotting and ABPP proteomics. Both probes are strictly activity-based and selective for the active site cysteine of USP30 for labelling among the 21 Cys residues present in USP30.
In summary, IMP-2586 and IMP-2587 represent the first potent and selective ABPs to explore USP30 activity in intact cells, with the potential to facilitate studies of USP30 biology and target engagement, supporting future development of appropriate therapeutic strategies.
Data availability
The MS data from this publication have been deposited to the ProteomeXchange Consortium via the PRIDE partner repository and assigned the identifier PXD044792.
Conflicts of interest
E. W. T. is a founding director and shareholder of Myricx Pharma Ltd., an advisor of and holds share options in Sasmara Therapeutics and receives current or recent funding from Myricx Pharma Ltd, Pfizer Ltd, Kura Oncology, AstraZeneca, Merck & Co., GSK.
Acknowledgements
M. M. was supported by a grant from The European Commission (H2020 Marie Sklodowska Curie Individual Fellowship grant 840690). F. C. and E. W. T. acknowledge funding support from the Laboratory for Synthetic Chemistry and Chemical Biology under the Health@InnoHK Program of The Government of Hong Kong Special Administrative Region of the People's Republic of China. E. W. T. and H. W. A. acknowledge support from the Cancer Research UK Imperial Centre at Imperial College London (A25147). We are grateful to Robin Ketteler for informative discussions on this work.
References
- D. Komander and M. Rape, Annu. Rev. Biochem., 2012, 81, 203–229 CrossRef CAS PubMed.
- J. A. Harrigan, X. Jacq, N. M. Martin and S. P. Jackson, Nat. Rev. Drug Discovery, 2018, 17, 57–77 CrossRef CAS PubMed.
- F. Wang, Y. Gao, L. Zhou, J. Chen, Z. Xie, Z. Ye and Y. Wang, Front. Pharmacol., 2022, 13, 851654 CrossRef CAS PubMed.
- M. J. Clague, S. Urbé and D. Komander, Nat. Rev. Mol. Cell Biol., 2019, 20, 338–352 CrossRef CAS PubMed.
- A. Hoshino, W. J. Wang, S. Wada, C. McDermott-Roe, C. S. Evans, B. Gosis, M. P. Morley, K. S. Rathi, J. Li, K. Li, S. Yang, M. J. McManus, C. Bowman, P. Potluri, M. Levin, S. Damrauer, D. C. Wallace, E. L. F. Holzbaur and Z. Arany, Nature, 2019, 575, 375–379 CrossRef CAS PubMed.
- B. Bingol, J. S. Tea, L. Phu, M. Reichelt, C. E. Bakalarski, Q. Song, O. Foreman, D. S. Kirkpatrick and M. Sheng, Nature, 2014, 510, 370–375 CrossRef CAS PubMed.
- C. N. Cunningham, J. M. Baughman, L. Phu, J. S. Tea, C. Yu, M. Coons, D. S. Kirkpatrick, B. Bingol and J. E. Corn, Nat. Cell Biol., 2015, 17, 160–169 CrossRef CAS PubMed.
- J. Liang, A. Martinez, J. D. Lane, U. Mayor, M. J. Clague and S. Urbé, EMBO Rep., 2015, 16, 618–627 CrossRef CAS PubMed.
- E. Marcassa, A. Kallinos, J. Jardine, E. V. Rusilowicz-Jones, A. Martinez, S. Kuehl, M. Islinger, M. J. Clague and S. Urbé, EMBO Rep., 2018, 19, e45595 CrossRef PubMed.
- B. Bingol and M. Sheng, Free Radical Biol. Med., 2016, 100, 210–222 CrossRef CAS PubMed.
- E. Tsefou, A. S. Walker, E. H. Clark, A. R. Hicks, C. Luft, K. Takeda, T. Watanabe, B. Ramazio, J. M. Staddon, T. Briston and R. Ketteler, Biochem. J., 2021, 478, 4099–4118 CrossRef CAS PubMed.
- T. S. Z. Fang, Y. Sun, A. C. Pearce, S. Eleuteri, M. Kemp, C. A. Luckhurst, R. Williams, R. Mills, S. Almond, L. Burzynski, N. M. Márkus, C. J. Lelliott, N. A. Karp, D. J. Adams, S. P. Jackson, J. F. Zhao, I. G. Ganley, P. W. Thompson, G. Balmus and D. K. Simon, Nat. Commun., 2023, 14, 7295 CrossRef CAS PubMed.
- Mission Therapeutics announces US FDA approval to initiate Phase II clinical trial of its lead asset MTX652 in Acute Kidney Injury–Mission Therapeutics, accessed 19 February 2024, https://missiontherapeutics.com/mission-therapeutics-announces-us-fda-approval-to-initiate-phase-ii-clinical-trial-of-its-lead-asset-mtx652-in-acute-kidney-injury/.
- D. S. Hewings, J. A. Flygare, M. Bogyo and I. E. Wertz, FEBS J., 2017, 284, 1555–1576 CrossRef CAS PubMed.
- J. A. Ward, L. McLellan, M. Stockley, K. R. Gibson, G. A. Whitlock, C. Knights, J. A. Harrigan, X. Jacq and E. W. Tate, ACS Chem. Biol., 2016, 11, 3268–3272 CrossRef CAS PubMed.
- N. Panyain, A. Godinat, T. Lanyon-Hogg, S. Lachiondo-Ortega, E. J. Will, C. Soudy, M. Mondal, K. Mason, S. Elkhalifa, L. M. Smith, J. A. Harrigan and E. W. Tate, J. Am. Chem. Soc., 2020, 142, 12020–12026 CrossRef CAS PubMed.
- J. A. Ward, A. Pinto-Fernandez, L. Cornelissen, S. Bonham, L. Díaz-Sáez, O. Riant, K. V. M. Huber, B. M. Kessler, O. Feron and E. W. Tate, J. Med. Chem., 2020, 63, 3756–3762 CrossRef CAS PubMed.
- E. V. Rusilowicz-Jones, J. Jardine, A. Kallinos, A. Pinto-Fernandez, F. Guenther, M. Giurrandino, F. G. Barone, K. McCarron, C. J. Burke, A. Murad, A. Martinez, E. Marcassa, M. Gersch, A. J. Buckmelter, K. J. Kayser-Bricker, F. Lamoliatte, A. Gajbhiye, S. Davis, H. C. Scott, E. Murphy, K. England, H. Mortiboys, D. Komander, M. Trost, B. M. Kessler, S. Ioannidis, M. K. Ahlijanian, S. Urbé and M. J. Clague, Life Sci. Alliance, 2020, 3, e202000768 CrossRef PubMed.
- W. Yue, Z. Chen, H. Liu, C. Yan, M. Chen, D. Feng, C. Yan, H. Wu, L. Du, Y. Wang, J. Liu, X. Huang, L. Xia, L. Liu, X. Wang, H. Jin, J. Wang, Z. Song, X. Hao and Q. Chen, Cell Res., 2014, 24, 482–496 CrossRef CAS PubMed.
- A. F. Kluge, B. R. Lagu, P. Maiti, M. Jaleel, M. Webb, J. Malhotra, A. Mallat, P. A. Srinivas and J. E. Thompson, Bioorg. Med. Chem. Lett., 2018, 28, 2655–2659 CrossRef CAS PubMed.
-
M. L. Stockley and M. I. Kemp, WO2018234775A1, 2018.
-
M. I. Kemp, M. Stockley and A. Jones, US2018/0194724A1, 2018.
-
M. I. Kemp and M. D. Woodrow, WO2017109488A1, 2017.
-
K. R. Gibson, A. Jones, M. I. Kemp, A. Madin, M. L. Stockley, G. A. Whitlock and M. D. Woodrow, WO2017141036A1, 2017.
-
M. L. Stockley, M. I. Kemp, A. Madin, M. D. Woodrow and A. Jones, WO2018/060689A1, 2018.
- C. Grethe, M. Schmidt, G.-M. Kipka, R. O’Dea, K. Gallant, P. Janning and M. Gersch, Nat. Commun., 2022, 13, 5950 CrossRef PubMed.
- E. M. Storck, J. Morales-Sanfrutos, R. A. Serwa, N. Panyain, T. Lanyon-Hogg, T. Tolmachova, L. N. Ventimiglia, J. Martin-Serrano, M. C. Seabra, B. Wojciak-Stothard and E. W. Tate, Nat. Chem., 2019, 11, 552–561 CrossRef CAS PubMed.
- G. Charron, M. M. Zhang, J. S. Yount, J. Wilson, A. S. Raghavan, E. Shamir and H. C. Hang, J. Am. Chem. Soc., 2009, 131, 4967–4975 CrossRef CAS PubMed.
- C. M. Hickey, N. R. Wilson and M. Hochstrasser, Nat. Rev. Mol. Cell Biol., 2012, 13, 755–766 CrossRef CAS PubMed.
- X. Xie, X. Wang, W. Liao, R. Fei, N. Wu, X. Cong, Q. Chen, L. Wei, Y. Wang and H. Chen, Apoptosis, 2019, 24, 135–144 CrossRef CAS PubMed.
- X. Ou, G. T. Zhang, Z. Xu, J. S. Chen, Y. Xie, J. K. Liu and X. P. Liu, Pathol. Oncol. Res., 2019, 25, 635–646 CrossRef CAS PubMed.
- C. Lin, H. Yan, J. Yang, L. Li, M. Tang, X. Zhao, C. Nie, N. Luo, Y. Wei and Z. Yuan, Oncotarget, 2017, 8, 56281–56295 CrossRef PubMed.
- D. Conole, F. Cao, C. W. A. Ende, L. Xue, S. Kantesaria, D. Kang, J. Jin, D. Owen, L. Lohr, M. Schenone, J. D. Majmudar and E. W. Tate, Angew. Chem., Int. Ed., 2023, 62, e202311190 CrossRef CAS PubMed.
- Mission Therapeutics Nominates First Development Candidates Targeting USP30, accessed 14 March 2024, https://missiontherapeutics.com/mission-therapeutics-nominates-first-development-candidates-targeting-usp30/.
- N. Panyain, A. Godinat, A. R. Thawani, S. Lachiondo-Ortega, K. Mason, S. Elkhalifa, L. M. Smith, J. A. Harrigan and E. W. Tate, RSC Med. Chem., 2021, 12, 1935–1943 RSC.
- D. Conole, M. Mondal, J. D. Majmudar and E. W. Tate, Front. Chem., 2019, 7, 876 CrossRef PubMed.
Footnotes |
† Electronic supplementary information (ESI) available. See DOI: https://doi.org/10.1039/d4cb00029c |
‡ M. M. and F. C. contributed equally to this work. |
§ Current address: Auckland Cancer Society Research Centre, Faculty of Medical and Health Sciences, University of Auckland, 85 Park Road, Grafton, Auckland, 1023, New Zealand. |
¶ Current address: Service and Central Laboratory of Hematology, University Hospital of Lausanne, Rue du Bugnon 46, 1011 Lausanne, Switzerland. |
|
This journal is © The Royal Society of Chemistry 2024 |
Click here to see how this site uses Cookies. View our privacy policy here.