DOI:
10.1039/C6RA03259A
(Communication)
RSC Adv., 2016,
6, 26954-26958
Mg(ClO4)2-promoted [4 + 3] cycloaddition of oxindole derivatives with conjugated dienes: concise synthesis of spirocycloheptane oxindole derivatives†
Received
4th February 2016
, Accepted 4th March 2016
First published on 4th March 2016
Abstract
We herein reported a novel Mg(ClO4)2-promoted intermolecular [4 + 3] cycloaddition reaction of oxindole derivatives and cyclopentadiene. This new strategy provides a convenient approach to the concise synthesis of a series of spirocycloheptane oxindole derivatives, with moderate to good yields and high diastereoselectivities.
The spirooxindole skeletons have drawn tremendous attention among synthetic and medicinal chemists during the past few years due to their functional diversity and their significance in organic synthesis as crucial intermediates.1 Among them, the spirocycloheptane oxindole frameworks represent ubiquitous structural motifs in a broad range of alkaloids and bioactive compounds (Fig. 1). Most of these alkaloids have been found to exhibit remarkable biological and pharmacological activities. For example, the gelsemine, which was first isolated from Carolina jasmine (Gelsemium sempervirens)2 in 1876,3 have recently been found to exhibit potent and specific antinociception in chronic pain by acting at the three spinal glycine receptors.4 In this context, the development of flexible synthetic methods for the synthesis of spirocycloheptane oxindole skeletons would thus be beneficial for the synthesis of those bioactive alkaloids.5 Although the chemistry of spirooxindole has been studied quite extensively, the investigations are mostly focused on the construction of spirocyclopentane6 or spirocyclohexane oxindole7 moieties. To date, there has been limited work on the direct construction of spirocycloheptane oxindole frameworks from oxindole derivatives.8,9
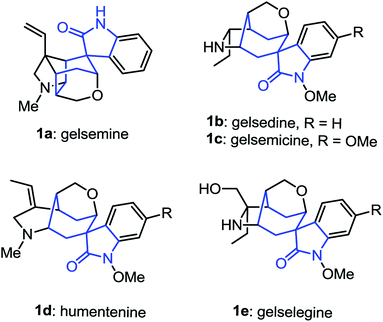 |
| Fig. 1 Structures of natural products possessing the spirocycloheptane oxindole skeleton. | |
The [4 + 3]-cycloaddition between allylic cations and conjugated dienes has been proved to be a powerful method for the direct synthesis of seven-membered rings.10–13 The acid-catalysed [4 + 3] cycloaddition reactions of furfurylalcohols and 1,3-diene were first reported by Pattenden in 2009 to construct the 5,5,7-tricyclic skeletons.13f The substituted furfuryl alcohols could undergo acid-catalysed hydrolysis transformations, and accompanied by displacement of the hydroxyl group, then leading to the furanoxonium ion intermediates, which could react with 1,3-diene via a [4 + 3] cycloaddition.10e Our group have recently disclosed the intermolecular [4 + 3] cycloaddition of heterocyclic compounds (such as benzofuran, benzothiophene and indole)14b,c with conjugated dienes to construct various 6,5,7-polycyclic skeletons and their application in the total synthesis of liphagal and frondosin B.14a In continuation of our previous work, we envisaged that a [4 + 3] cycloaddition reaction between oxindole derived allylic cations and conjugated dienes would result in the straightforward formation of spirocycloheptane oxindoles. Herein, we report a highly diastereoselective [4 + 3] cycloaddition route to the synthesis of spirocycloheptane oxindoles (Scheme 1).
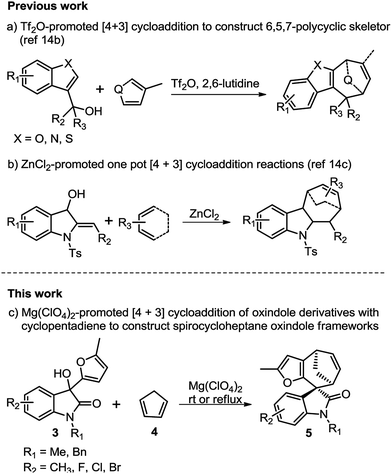 |
| Scheme 1 [4 + 3] cycloaddition reactions of heterocyclic compounds with conjugated dienes. | |
We initiated our studies using oxindole derivative 3a15 as dienophiles and cyclopentadiene 4 as conjugated dienes to explore the cycloaddition conditions. Compounds 3a could be readily prepared from N-substituted isatins and 2-methyl furan (for details see ESI†). Selected representative screening results for this model reaction are presented in Table 1. Initially, a variety of frequently used Brønsted acids as well as Lewis acids were examined for the cycloaddition reaction at room temperature in DCM. However, Brønsted acids and most of Lewis acids resulted in no reaction at all (for details see ESI†). Lewis acids such as SnCl4 and ZnCl2 could promote the reaction, but resulted in low yields, probably due to the polymerization of dienes or decomposition of the starting materials in these conditions (Table 1, entries 4 and 5). Finally, Mg(ClO4)2 was proved to be the optimum Lewis acid. Upon treatment with 1.5 equiv. of anhydrous Mg(ClO4)2 in DCM at ambient temperature, 3a underwent cycloaddition with 4 to afford the spirooxindole 5a in 58% yield within 2 h (Table 1, entry 7). Further optimization of the reaction conditions by changing the temperature and solvents did not improve the yields (Table 1, entries 6–10).
Table 1 Optimization of the reaction conditiona
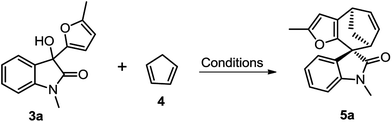
|
Entry |
Conditions |
Solvent |
T (°C) |
Yieldb (%) |
Unless otherwise noted, all reaction was performed with 3 (0.1 mmol, 1.0 eq.), 4 (1.0 mmol, 10.0 eq.) and Lewis acids or Brønsted acids (0.15 mmol, 1.5 eq.) in solvent (15 mL). Yield of isolated product. Determined by 1H NMR (400 MHz) analysis (starting material was decomposed). NR = no reaction (starting material was recovered); ND = not determined (starting material was decomposed). |
1 |
TFA |
DCM |
−78 to 0 |
Tracec |
2 |
CSA |
DCM |
−78 to rt |
NRd |
3 |
HClO4 |
DCM |
−78 |
NDd |
4 |
SnCl4 |
DCM |
−78 to rt |
18 |
5 |
ZnCl2 |
DCM |
rt |
30 |
6 |
Mg(ClO4)2 |
DCM |
0 |
<5 |
7 |
Mg(ClO4)2 |
DCM |
rt |
58 |
8 |
Mg(ClO4)2 |
DCM |
Reflux |
NDd |
9 |
Mg(ClO4)2 |
THF |
rt |
20 |
10 |
Mg(ClO4)2 |
Et2O |
rt |
25 |
Having established the optimal reaction conditions, we then investigated the reactivity of a series of oxindole compounds 3a–l towards cyclopentadiene 4 (Fig. 2). As is shown in Fig. 2, this novel [4 + 3] cycloaddition reaction was found to be applicable for a series of oxindole substrates, and a number of spirocycloheptane oxindole derivatives were afforded in moderate to good yields. The electronic properties of R1 group on the N-atom of oxindole derivatives clearly influenced the reactivity of substrates. The substrates with electron-donating groups (R1 = Me/Bn) afforded products 5 in good yields (Fig. 2, 5a–5b), whereas those with electron-withdrawing groups (R1 = Ac) could not afford the desired products. Similarly, electronic effects of R2 also substantially affected the yields. In general, the presence of halogen atoms on the phenyl ring obviously reduced the reactivity of substrates (Fig. 2, 5d–5j), therefore the higher temperatures were needed for these reactions. While the electron-donating substituents had positive effects (Fig. 2, 5c and 5k). However, the reaction could not proceed when the substituents on the phenyl ring were two fluorine atoms (Fig. 2, 5l). Notably, this [4 + 3] cycloaddition reaction showed excellent diastereoselectivities for all substrates, and the relative configuration could be determined by the single X-ray crystallographic analysis data of 5a (Fig. 2).
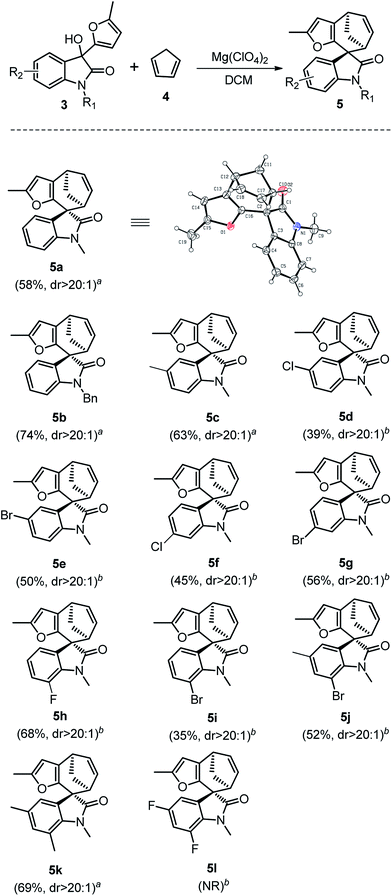 |
| Fig. 2 Scope of the [4 + 3] cycloaddition reaction. a Unless otherwise noted, the reaction was performed with 3 (0.1 mmol, 1.0 eq.), 4 (1.0 mmol, 10.0 eq.), and Mg(ClO4)2 (0.15 mmol, 1.5 eq.) in DCM (15 mL) at rt. b The reaction was performed at refluxing temperature. c Diastereomeric ratios were determined by 1H NMR (400 MHz) analysis. In the experimental results, the diastereoisomer could not be detected in 1H NMR spectra, therefore we identified the dr as >20 : 1. | |
The details of the [4 + 3] cycloaddition mechanism have not yet been fully understood. The theoretical results proposed by Winne et al. pointed to a two-step cationic cyclization process,16a and this can also be supported by Wu's report via the DFT calculations.16b On the basis of our previous research14,16e and literature reports,16a–d a possible mechanism was proposed, as is shown in Scheme 2. Firstly, an allylic cation intermediate 6a could be achieved from the oxindole compounds under Lewis acid conditions. Then, an intermolecular [4 + 3] cycloaddition between 6a and dienes 4 via endo-cycloaddition transition state 8a resulted in the spirocycloheptane oxindole skeleton 5a. Most intermolecular oxyallyl cation-type reactions usually prefer an exo- (or extended) addition mode; while in our experiment, we proposed the transformation was taking place under kinetic control (performed at room temperature or ca. 40 °C), which probably prefer the endo-transition state for maximizing the π–π stacking interaction between the oxindole cation and conjugated cyclopentadiene.17
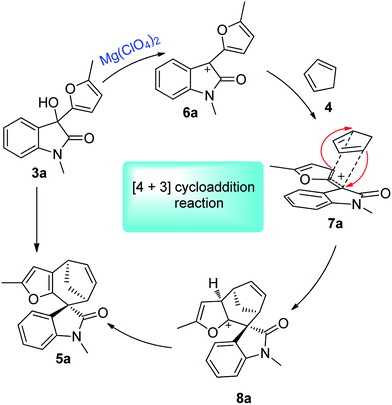 |
| Scheme 2 A plausible mechanism for the [4 + 3] cycloaddition reaction. | |
Conclusions
In summary, we have developed a novel and convenient approach for the construction of spirocycloheptane oxindole skeletons through a Mg(ClO4)2-promoted [4 + 3] cycloaddition of oxindole derivatives with conjugated dienes. This method could achieve the concise synthesis of a series of spirocycloheptane oxindoles in moderate yields with high diastereoselectivities. We believe that the strategy demonstrated here may be utilized in the further synthesis of natural products and potential bioactive compounds containing spirocycloheptane oxindole skeletons. Further investigations towards the refinement of the reaction mechanism, as well as applications in total synthesis, are now under way in our lab and will be reported in due course.
Acknowledgements
The authors acknowledge the financial support provided by the National Natural Science Foundation of China (No. 21472078, 21272099, and 21572092). We thank professor Zhi-Xiang Xie (Lanzhou University) for helpful discussion.
Notes and references
- For reviews of spirooxindole skeletons, see:
(a) D.-J. Cheng, Y. Ishihara, B. Tan and C. F. Barbas III, ACS Catal., 2014, 4, 743 CrossRef CAS
;
(b) A. K. Franz, N. V. Hanhan and N. R. Ball-Jones, ACS Catal., 2013, 3, 540 CrossRef CAS
;
(c) C. V. Galliford and K. A. Scheidt, Angew. Chem., Int. Ed., 2007, 46, 8748 CrossRef CAS PubMed
. - For reviews of Gelsemium alkaloid, see:
(a) B.-F. Zhang, G.-X. Chou and Z.-T. Wang, Helv. Chim. Acta, 2009, 92, 1889 CrossRef CAS
;
(b) J.-M. Yu, T. Wang, X.-X. Liu, J. Deschamps, J. Flippen-Anderson, X.-B. Liao and J. M. Cook, J. Org. Chem., 2003, 68, 7565 CrossRef CAS PubMed
;
(c) H. Takayama and S. Sakai, J. Synth. Org. Chem., Jpn., 1990, 48, 876 CrossRef CAS
. - F. L. Sonnenschein, Ber. Dtsch. Chem. Ges., 1876, 9, 1182 CrossRef
. - J.-Y. Zhang, N. Gong, J.-L. Huang, L.-C. Guo and Y.-X. Wang, Pain, 2013, 154, 2452 CrossRef CAS PubMed
. - For recent selected examples of synthesis of Gelsemine alkaloids:
(a) H. Lin and S. J. Danishefsky, Angew. Chem., Int. Ed., 2003, 42, 36 CrossRef CAS
;
(b) A. Madin, C. J. O'Donnell, T. B. Oh, D. W. Old, L. E. Overman and M. J. Sharp, Angew. Chem., Int. Ed., 1999, 38, 2934 CrossRef CAS
;
(c) S. Yokoshima, H. Tokuyama and T. Fukuyama, Angew. Chem., Int. Ed., 2000, 39, 4073 CrossRef CAS
;
(d) X. Zhou, T. Xiao, Y. Iwama and Y. Qin, Angew. Chem., Int. Ed., 2012, 51, 4909 CrossRef CAS PubMed
;
(e) S. Atarashi, J. K. Choi, D. C. Ha, D. J. Hart, D. Kuzmich, C. S. Lee, S. Ramesh and S. C. Wu, J. Am. Chem. Soc., 1997, 119, 6226 CrossRef CAS
;
(f) W. G. Earley, J. E. Jacobsen, A. Madin, G. P. Meier, C. J. O'Donnell, T. Oh, D. W. Old, L. E. Overman and M. J. Sharp, J. Am. Chem. Soc., 2005, 127, 18046 CrossRef CAS PubMed
;
(g) T. Fukuyama and G. Liu, J. Am. Chem. Soc., 1996, 118, 7426 CrossRef CAS
;
(h) A. Madin, C. J. O'Donnell, T. Oh, D. W. Old, L. E. Overman and M. J. Sharp, J. Am. Chem. Soc., 2005, 127, 18054 CrossRef CAS PubMed
;
(i) F. W. Ng, H. Lin and S. J. Danishefsky, J. Am. Chem. Soc., 2002, 124, 9812 CrossRef CAS PubMed
;
(j) A. J. Pearson and X.-L. Wang, J. Am. Chem. Soc., 2003, 125, 13326 CrossRef CAS PubMed
;
(k) X. Chen, S. Duan, C. Tao, H. Zhai and F.-G. Qiu, Nat. Commun., 2015, 6, 7204 CrossRef PubMed
. - For recent selected examples of spirocyclopentane oxindole skeletons, see:
(a) J. Dugal-Tessier, E. A. O'Bryan, T. B. H. Schroeder, D. T. Cohen and K. A. Scheidt, Angew. Chem., Int. Ed., 2012, 51, 4963 CrossRef CAS PubMed
;
(b) X. Zhao, X. Liu, Q. Xiong, H. Mei, B. Ma, L. Lin and X. Feng, Chem. Commun., 2015, 51, 16076 RSC
;
(c) R. Zhou, K. Zhang, Y. Chen, Q. Meng, Y. Liu, R. Li and Z. He, Chem. Commun., 2015, 51, 14663 RSC
;
(d) Y.-M. Cao, F.-F. Shen, F.-T. Zhang and R. Wang, Chem.–Eur. J., 2013, 19, 1184 CrossRef CAS PubMed
;
(e) W. Guo, X. Wang, B. Zhang, S. Shen, X. Zhou, P. Wang, Y. Liu and C. Li, Chem.–Eur. J., 2014, 20, 8545 CrossRef CAS PubMed
;
(f) J. Xu, L.-D. Shao, D. Li, X. Deng, Y.-C. Liu, Q.-S. Zhao and C. Xia, J. Am. Chem. Soc., 2014, 136, 17962 CrossRef CAS PubMed
;
(g) Y. Tian, L. Tian, X. He, C. Li, X. Jia and J. Li, Org. Lett., 2015, 17, 4874 CrossRef CAS PubMed
;
(h) C. Zheng, W. Yao, Y. Zhang and C. Ma, Org. Lett., 2014, 16, 5028 CrossRef CAS PubMed
. - For recent selected examples of spirocyclohexane oxindole skeletons, see:
(a) T.-P. Gao, J.-B. Lin, X.-Q. Hu and P.-F. Xu, Chem. Commun., 2014, 50, 8934 RSC
;
(b) W. Yang and D.-M. Du, Chem. Commun., 2013, 49, 8842 RSC
;
(c) W. Cao, X. Liu, J. Guo, L. Lin and X. Feng, Chem.–Eur. J., 2015, 21, 1632 CrossRef CAS PubMed
;
(d) H.-L. Cui and F. Tanaka, Chem.–Eur. J., 2013, 19, 6213 CrossRef CAS PubMed
;
(e) Y. Shi, A. Lin, H. Mao, Z. Mao, W. Li, H. Hu, C. Zhu and Y. Cheng, Chem.–Eur. J., 2013, 19, 1914 CrossRef CAS PubMed
;
(f) Y.-Y. Han, W.-Y. Han, X. Hou, X.-M. Zhang and W.-C. Yuan, Org. Lett., 2012, 14, 4054 CrossRef CAS PubMed
;
(g) S. Zhao, J.-B. Lin, Y.-Y. Zhao, Y.-M. Liang and P.-F. Xu, Org. Lett., 2014, 16, 1802 CrossRef CAS PubMed
. -
(a) P. Drouhin, T. E. Hurst, A. C. Whitwood and R. J. Taylor, Org. Lett., 2014, 16, 4900 CrossRef CAS PubMed
;
(b) L. Wang, Y. Su, X. Xu and W. Zhang, Eur. J. Org. Chem., 2012, 33, 6606 Search PubMed
;
(c) L. Gorokhovik, Neuville and J.-P. Zhu, Org. Lett., 2011, 13, 5536 CrossRef PubMed
;
(d) J. R. Fuchs and R. L. Funk, Org. Lett., 2005, 7, 677 CrossRef CAS PubMed
;
(e) A. C. Peterson and J. M. Cook, Tetrahedron Lett., 1994, 35, 2651 CrossRef CAS
;
(f) V. Nair, P. M. Treesa, N. P. Rath, A. C. Kunwar, K. S. KiranKumar, A. RaviSankar, M. Vairamani and S. Prabhakar, Tetrahedron, 2002, 58, 7221 CrossRef CAS
. - G. Zhan, M. L. Shi, Q. He, W. Du and Y. C. Chen, Org. Lett., 2015, 17, 4750 CrossRef CAS PubMed
. - For reviews of [4 + 3] cycloaddition reactions, see:
(a) M. Harmata, Acc. Chem. Res., 2001, 34, 595 CrossRef CAS PubMed
;
(b) J. K. Cha and J. Oh, Curr. Org. Chem., 1998, 2, 217 CAS
;
(c) M. Harmata, Chem. Commun., 2010, 46, 8886 RSC
;
(d) M. Harmata, Chem. Commun., 2010, 46, 8904 RSC
;
(e) M. J. Palframan and G. Pattenden, Chem. Commun., 2014, 50, 7223 RSC
. - For recent selected examples of [4 + 3] cycloaddition reactions, see:
(a) L. Chen, G.-M. Yang, J. Wang, Q.-F. Jia, J. Wei and Z.-Y. Du, RSC. Adv., 2015, 5, 76696 RSC
;
(b) C. S. Jeffrey, D. Anumandla and C. R. Carson, Org. Lett., 2012, 14, 5764 CrossRef CAS PubMed
;
(c) C. S. Jeffrey, K. L. Barnes, J. A. Eickhoff and C. R. Carson, J. Am. Chem. Soc., 2011, 133, 7688 CrossRef CAS PubMed
;
(d) E. H. Krenske, K. N. Houk and M. Harmata, J. Org. Chem., 2015, 80, 744 CrossRef CAS PubMed
;
(e) B. Lo and P. Chiu, Org. Lett., 2011, 13, 864 CrossRef CAS PubMed
;
(f) A. G. Lohse and R. P. Hsung, Chem.–Eur. J., 2011, 17, 3812 CrossRef CAS PubMed
;
(g) A. G. Lohse, R. P. Hsung, M. D. Leider and S. K. Ghosh, J. Org. Chem., 2011, 76, 3246 CrossRef CAS PubMed
;
(h) J. M. Winne, S. Catak, M. Waroquier and V. Van Speybroeck, Angew. Chem., Int. Ed., 2011, 50, 11990 CrossRef CAS PubMed
;
(i) O. A. Ivanova, E. M. Budynina, A. O. Chagarovskiy, A. E. Kaplun, I. V. Trushkov and M. Y. Melnikov, Adv. Synth. Catal., 2011, 353, 1125 CrossRef CAS
. - For recent selected examples of [4 + 3] cycloaddition reactions, see:
(a) B. W. Gung, D. T. Craft, L. N. Bailey and K. Kirschbaum, Chem.–Eur. J., 2010, 16, 639 CrossRef CAS PubMed
;
(b) X. Han, H. Li, R. P. Hughes and J. Wu, Angew. Chem., Int. Ed., 2012, 51, 10390 CrossRef CAS PubMed
;
(c) D. Shu, W. Song, X. Li and W. Tang, Angew. Chem., Int. Ed., 2013, 52, 3237 CrossRef CAS PubMed
;
(d) B. Song, L.-H. Li, X.-R. Song, Y.-F. Qiu, M.-J. Zhong, P.-X. Zhou and Y.-M. Liang, Chem.–Eur. J., 2014, 20, 5910 CrossRef CAS PubMed
. - For recent selected examples of [4 + 3] cycloaddition reactions, see:
(a) A. H. E. Hassan, J. K. Lee, A. N. Pae, S. J. Min and Y. S. Cho, Org. Lett., 2015, 17, 2672 CrossRef CAS PubMed
;
(b) D. R. Laplace, B. Verbraeken, K. Van Hecke and J. M. Winne, Chem.–Eur. J., 2014, 20, 253 CrossRef CAS PubMed
;
(c) Y. Lian, L. C. Miller, S. Born, R. Sarpong and H. M. L. Davies, J. Am. Chem. Soc., 2010, 132, 12422 CrossRef CAS PubMed
;
(d) J. Wang, S.-G. Chen, B.-F. Sun, G. Q. Lin and Y.-J. Shang, Chem.–Eur. J., 2013, 19, 2539 CrossRef CAS PubMed
;
(e) J. Xu, E. J. E. Caro-Diaz and E. A. Theodorakis, Org. Lett., 2010, 12, 3708 CrossRef CAS PubMed
;
(f) G. Pattenden and J. M. Winne, Tetrahedron Lett., 2009, 50, 7310 CrossRef CAS
. -
(a) J. Zhang, L. Li, Y. Wang, W. Wang, J. Xue and Y. Li, Org. Lett., 2012, 14, 4528 CrossRef CAS PubMed
;
(b) W. Gong, Y. Liu, J. Zhang, Y. Jiao, J. Xue and Y. Li, Chem.–Asian J., 2013, 8, 546 CrossRef CAS PubMed
;
(c) J. Zhang, J. Shao, J. Xue, Y. Wang and Y. Li, RSC Adv., 2014, 4, 63850 RSC
. - We have tried many types of allylic alcohols: such as ethenyl-, alkynyl-, phenyl-, thienyl-, furyl-, benzothiophenyl- group, etc. The unsubstituted furyl-oxindole derivatives could not exist stably for the C2 position of furan is the most reactive site towards electrophiles. And the others could not react with cyclopentadiene under the optimal reaction
conditions to achieve the expected spirocycloheptane oxindole skeletons (for details see ESI†).
-
(a) J. M. Winne, S. Catak, M. Waroquier and V. van Speybroeck, Angew. Chem., Int. Ed., 2011, 50, 11990 CrossRef CAS PubMed
;
(b) X. Han, H. Li, R. P. Hughes and J. Wu, Angew. Chem., Int. Ed., 2012, 51, 10390 CrossRef CAS PubMed
;
(c) M. J. Palframan and G. Pattenden, Tetrahedron Lett., 2013, 52, 324 CrossRef
;
(d) G. Pattenden and M. Palframan, Synlett, 2013, 24, 2720 CrossRef
;
(e) In the experimental results, when we used thiophenes as dienes, only a Friedel–Crafts intermolecular alkylation reaction occurred, giving an ordinary electrophilic adducts 9a (determined by the single X-ray crystallographic analysis, for details see ESI†). We expected that these results might support for a two-step pathway mechanism of the intermolecular [4 + 3] cycloaddition reactions (for details see ESI†). -
(a) M. Harmata, S. Elomari and C. L. Barnes, J. Am. Chem. Soc., 1996, 118, 2860 CrossRef CAS
;
(b) J. I. Garcia, J. A. Mayoral and L. Salvatella, Acc. Chem. Res., 2000, 33, 658 CrossRef CAS PubMed
.
Footnote |
† Electronic supplementary information (ESI) available. CCDC 1434481. For ESI and crystallographic data in CIF or other electronic format see DOI: 10.1039/c6ra03259a |
|
This journal is © The Royal Society of Chemistry 2016 |