DOI:
10.1039/D1SC01529J
(Edge Article)
Chem. Sci., 2021,
12, 7720-7726
Solvent-dependent fac/mer-isomerization and self-assembly of triply helical complexes bearing a pivot part†
Received
17th March 2021
, Accepted 16th April 2021
First published on 17th April 2021
Abstract
Tris–chelate metal complexes of unsymmetrical bidentate ligands can form two geometric stereoisomers, facial (fac) and meridional (mer) isomers. Due to the small difference in their properties, the highly-selective synthesis of one of the isomers is challenging. We now designed a series of tripodal ligands with a tris(3-(2-(methyleneoxy)ethoxy)phenyl)methane pivot. Surprisingly, the ratio of the fac/mer isomers of the triply helical FeII complexes significantly changed depending on the solvents. To the best of our knowledge, this is the first example of fac/mer isomerism of a labile tris(2,2′-bipyridine) FeII complex governed by the solvent. Furthermore, well-defined self-assemblies were quantitatively produced by imine bond formation with a suitable diamine. The supramolecular assemblies contained only the fac isomer even though a mixture of the two isomers existed in solution before the condensation reaction. Namely, the self-assembly formation effectively adjusted the geometries of the building unit that results in the suitable supramolecular structure.
Introduction
Tris–chelate metal complexes of unsymmetrical bidentate ligands can form two geometric stereoisomers, facial (fac) and meridional (mer) isomers. When an unsymmetrical bidentate ligand reacts with a metal ion to give the octahedral tris(ligand) complex, the statistical ratio of the fac and mer isomers is 1
:
3. The structures of the two isomers are different, but their physical and chemical properties are usually similar. Due to the small difference in their properties, the highly-selective synthesis of one of the isomers is challenging. Useful but limited strategies have been reported. The electronic and structural properties of ligands, such as the trans influence,1 steric hindrance,2 π–π interaction,3 tripodal structures,4 and their combination,5 have been successfully applied to control the isomerism. It is worth noting here that the ligands usually dominate the isomeric equilibrium. The conversion between the isomers using the same ligand is more difficult, but it leads to dynamic structural switching in response to environmental change or external stimuli.6–8 In supramolecular chemistry, the fac/mer isomerism has recently attracted considerable attention to create elaborate self-assemblies consisting of metal ions and ligands. Diimine ligands such as 2,2′-bipyiridine and 2-pyridylimine derivatives are widely used to construct and govern the designed structures and functions,9 and other bidentate ligands such as catechol derivatives are also effectively employed.10 For instance, completely different assembled structures were obtained by utilizing the fac/mer interconversion based on the solvent or counter ions.9,10
We have studied allosteric molecular recognition by the tripodal ligands bearing three oligoether–bipyridine arms.11 Recently, we reported the first example of the perfectly selective synthesis of a meridional triply helical complex.12 This meridional complex has a unique structure in which one bipyridine unit penetrates into the cyclic cavity provided by the other two oligoether arms and the benzene pivot unit. As a result, the ion recognition ability of the tripodal molecule has been successfully suppressed. Most of the triply helical complexes reported so far are facial isomers,4 but our example demonstrated that a tripodal ligand that is appropriately designed can be utilized as a meridional building block. We have also reported a self-assembly system using Schiff-base formation of a triply helical facial complex.13 The triply helical building unit was exclusively obtained by the complexation of Fe2+ or Ru2+ with a tripodand in which three bipyridyl ligands are directly attached to a tris(2-methyleneoxyphenyl)methane pivot.
In this context, we envisaged that a pivot structure with the appropriate space and flexibility would result in a different ligation behavior towards a metal ion to give octahedral complexes with a different fac/mer ratio in response to their environments. We now designed a series of tripodal ligands L (1a, 1b, 2a, and 2b) with a tris(3-(2-(methyleneoxy)ethoxy)phenyl)methane pivot (Fig. 1). Surprisingly, the ratio of the fac/mer isomers of the triply helical FeII complexes significantly changed depending on the solvents. We found that the Hansen solubility parameters14 fit well with the fac/mer equilibrium ratio in different solvents. To the best of our knowledge, this is the first example of fac/mer isomerism of a labile tris(2,2′-bipyridine) FeII complex depending on the solvent change. Furthermore, well-defined self-assemblies were quantitatively produced by imine bond formation of [2bFe]2+ with a suitable diamine. The supramolecular assemblies contained only the facial isomer even though a mixture of the two isomers existed in solution before the condensation reaction. Namely, the self-assembly formation effectively adjusted the geometries of the building unit that results in the suitable supramolecular structure.
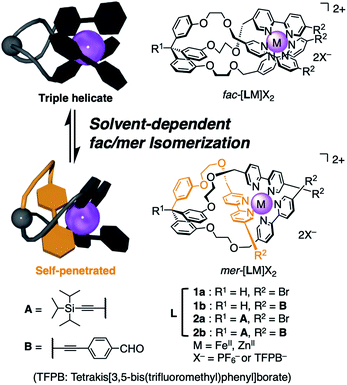 |
| Fig. 1 Schematic representations of the interconversion between the facial (fac) and meridional (mer) isomers and chemical structures of both isomers of [LM]X2 (L: tripodal ligands (1a, 1b, 2a, and 2b); M: octahedral metal; X: anion). | |
Results and discussion
Synthesis and characterization of tripodal ligands and their FeII complexes
Four tripodal ligands 1a, 1b, 2a, and 2b were synthesized. The common part is a tris(3-(2-(methyleneoxy)ethoxy)phenyl)methane pivot and three 2,2′-bipyridine units. The meta-substituted pivot instead of ortho-substituted one in the previous study13 provides more molecular freedom. Moreover, the flexible 2-(methyleneoxy)ethoxy linker also allows many conformations without steric constraints. 1a is the simplest ligand with a methine proton at the pivot unit and a 5-bromo group at the bipyridyl units. 2a and 2b have a (triisopropylsilyl)ethynyl group (A in Fig. 1) at the pivot carbon to investigate the structural effect and use for possible structural extension in future studies. In 1b and 2b, three 4-formylphenylethynyl groups (B in Fig. 1) were introduced, which are employed for the self-assembly of the triply helical complexes via imine bond formation.
The tripodal ligand 2b possessing both units A and B is explained in detail below. 2b was synthesized by the reaction of a tris(3-hydroxyphenyl)methane derivative (“a pivot part”) and a mesylated 2-(5′-bromo-2,2′-bipyridyl)ethanol derivative (“a coordination part”), followed by the Sonogashira cross-coupling reaction with 4-ethynylbenzaldehyde to introduce unit B. [2bFe](PF6)2 was then obtained by the complexation of this ligand with Fe(BF4)2 and the exchange of the counter anion to PF6−. The synthetic procedures for 2b and [2bFe](PF6)2, as well as those for 1a, 1b, 2a and their FeII complexes, are described in the ESI (1H NMR spectra and ESI-MS are shown in Fig. S1–S18, S28–S35 and S41–S45†).
The 1H NMR spectrum of [2bFe](PF6)2 in CD3CN indicated that this complex is a mixture of the facial and meridional isomers (Fig. 2a and S41†). Focusing on the signals of the proton f (2 position of the benzene ring of the pivot, see Fig. S41† for the assignment of the other 1H NMR signals), there are one large signal at 6.08 ppm (denoted with a red circle in Fig. 2) and three small signals with an equal intensity at 6.18, 6.30, and 6.35 ppm (denoted with blue triangles in Fig. 2). The signal at 6.08 ppm was assigned to the facial isomer and the three signals at 6.18, 6.30, and 6.35 ppm were assigned to the meridional isomer based on symmetry: The facial isomer has C3 symmetry but the meridional isomer does not. It is considered that the appropriate length and flexibility of the ethylene glycol chains enabled the formation of these two isomers. The fac/mer ratio calculated from the integral values of the proton signals was 71
:
29. Considering the statistical ratio of fac/mer = 25
:
75, the facial isomer of [2bFe](PF6)2 was favored in CD3CN.
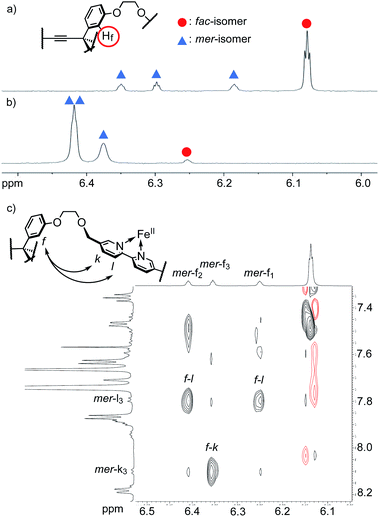 |
| Fig. 2 (a and b) 1H NMR spectra of [2bFe](PF6)2 (600 MHz, 5.9–6.5 ppm). (a) CD3CN. (b) CDCl3. (c) 1H–1H ROESY spectrum of [2bFe](TFPB)2. See Fig. S47† for the detailed assignment of the signals. The characteristic ROE correlations supporting the structure of mer-[2bFe](TFPB)2 are highlighted (signals of the mer-isomer are numbered for each tripodal arm). | |
Solvent dependence on fac/mer isomerism of [2bFe]2+
When the solvent of the sample of [2bFe](PF6)2 was changed to CDCl3 from CD3CN, only a small signal was found at 6.25 ppm, and two large signals were observed at 6.38 and 6.42 ppm with the ratio of 2
:
1 (Fig. 2b and S77†). This result showed that the equilibrium significantly shifted toward the meridional isomer in CDCl3. The sample solution was heated at 50 °C for 12 hours and cooled to r.t. to reach the fac/mer equilibrium of 4
:
96. To further investigate this interesting phenomenon, the fac/mer ratios of [2bFe]2+ (as well as the Gibbs free energy ΔG) were determined by 1H NMR measurements in various deuterated solvents. Due to the poor solubility of [2bFe](PF6)2 in low-polar solvents, [2bFe](TFPB)2 (TFPB: tetrakis[3,5-bis(trifluoromethyl)phenyl]borate) was synthesized (Fig. S46–S51†), which exhibited a good solubility in a wide range of solvents (CD3CN, CDCl3, CD3OD, acetone-d6, CD3NO2, CD3CO2D, CD2Cl2, THF-d8, DMF-d7, dioxane-d8, o-dichlorobenzene-d4: o-DCB-d4, tetrachloroethane-d2: TCE-d2). The 1H–1H ROESY spectrum of [2bFe](TFPB)2 showed cross peaks between the proton f (pivot unit) and the protons k,l (3,4 positions of bipyridyl) of the meridional isomer (Fig. 2c and S47†). This suggested that the meridional isomer had a self-penetrated structure in which one bipyridine unit was surrounded by the other two bipyridines and the triphenylmethane of the pivot unit.
The fac/mer ratios of [2bFe](TFPB)2 changed from 10
:
90 to 71
:
29 depending on the solvents (Table 1 and Fig. S66†). The meridional isomers are favored in halogenated solvents (TCE-d2 (10
:
90), CDCl3 (10
:
90)), but other than this point, it seems difficult to find a good factor to fit this trend at first glance. DMF-d7 (32
:
68), which is generally regarded as a highly polar solvent (ε (dielectric constant): 36.7), and CD2Cl2 (40
:
60), which is generally regarded as a less polar solvent (ε: 8.9), gave similar isomer ratios. Thus, this solvent dependence was assumed to be a complex phenomenon involving factors other than polarity.
Table 1 The ratios of the fac/mer isomers of [2bFe](TFPB)2 and thermodynamic parameters for the isomerization from mer to fac in various solvents (298 K)
Solvent |
fac/mer |
ΔG (kJ mol−1) |
ΔHa (kJ mol−1) |
TΔSa (kJ mol−1) |
Determined by van't Hoff analysis of VT NMR measurements.
|
TCE-d2 |
10 : 90 |
5.5 |
— |
— |
CDCl3 |
10 : 90 |
5.5 |
−9.2 |
−14 |
o-DCB-d4 |
13 : 87 |
4.8 |
— |
— |
Dioxane-d8 |
20 : 80 |
3.4 |
— |
— |
DMF-d7 |
32 : 68 |
1.8 |
−4.2 |
−6.0 |
THF-d8 |
34 : 66 |
1.7 |
−11 |
−13 |
CD2Cl2 |
40 : 60 |
1.1 |
— |
— |
CD3CO2D |
53 : 47 |
−0.31 |
— |
— |
CD3NO2 |
57 : 43 |
−0.70 |
— |
— |
CD3OD |
64 : 36 |
−1.5 |
— |
— |
Acetone-d6 |
69 : 31 |
−2.0 |
−22 |
−20 |
CD3CN |
71 : 29 |
−2.2 |
−12 |
−10 |
For some solvents, the changes in enthalpy ΔH and entropy ΔS upon the conversion from the mer isomer to the fac isomer were determined by the van't Hoff analysis of variable-temperature NMR measurements of [2bFe](TFPB)2 (Table 1 and Fig. S67–S71†). As a result, both ΔH and ΔS were negative in all the investigated solvents. Thus, the facial isomer is enthalpically favorable and the meridional isomer is entropically favorable. It appears that the changes in the fac/mer ratio (i.e., ΔG) were not correlated very well with ΔH or ΔS, which suggested that this isomerization cannot be explained by a simple factor. One of the causes would be the degree of formation of ion pairs, but the details could not be explained (Table S2, Fig. S72 and S73†).
Solvent dependence of the other complexes
To investigate the effect of substituents (units A and B in Fig. 1) of the tripodand on the solvent dependence, [1aFe](TFPB)2, [1bFe](TFPB)2, and [2aFe](TFPB)2 were synthesized (Fig. S19–S27 and S36–S40†), and their fac/mer ratios were determined in CD3CN and CDCl3 (Table 2). As a result, the fac/mer ratios of all the complexes depended on the solvents in a similar manner with [2bFe](TFPB)2, that is, facial isomers were favored in CD3CN, while meridional isomers increased in CDCl3 (Fig. S74–S77†). Furthermore, it was confirmed that the fac/mer ratio of a ZnII complex, [2bZn](TFPB)2 (Fig. S52–S56†), showed the same tendency (90
:
10 in CD3CN, 67
:
33 in CD3CN/CDCl3 = 1
:
4, Fig. S78†). For comparison, the monobipyridine ligand 3b and its tris(ligand) complex [(3b)3Fe](TFPB)2 were synthesized (Fig. 3, spectral data of characterization are shown in Fig. S57–S65†). As a result, the fac/mer ratio of [(3b)3Fe](TFPB)2 showed no solvent dependence (22
:
78 in CD3CN, 21
:
79 in CDCl3, Fig. S79†). Thus, the tris(3-(2-methoxyethoxy)phenyl)methane unit of [2bFe](TFPB)2 plays a key role in the solvent-dependent fac/mer isomerism.
Table 2 Solvent effects on the fac/mer-isomerization equilibrium of various complexesa
Complex |
Difference from [2bFe](TFPB)2 |
fac/mer in CD3CN |
fac/mer in CD3CN/CDCl3 = 1/4 |
fac/mer in CDCl3 |
The fac/mer ratios were calculated from the signals of the proton f (2 position of the benzene ring of the pivot) (see Fig. 2).
Calculated from the signals of the formyl proton q (see Fig. S61).
|
[2bFe](TFPB)2 |
— |
71 : 29 |
39 : 61 |
10 : 90 |
[1aFe](TFPB)2 |
No group A, B |
83 : 17 |
— |
64 : 36 |
[1bFe](TFPB)2 |
No group A |
76 : 24 |
— |
22 : 78 |
[2aFe](TFPB)2 |
No group B |
75 : 25 |
— |
13 : 87 |
[2bFe](PF6)2 |
PF6− |
71 : 29 |
— |
4 : 96 |
[2bZn](TFPB)2 |
ZnII |
90 : 10 |
67 : 33 |
— |
[(3b)3Fe](TFPB)2 |
Without pivot |
22 : 78b |
— |
21 : 79b |
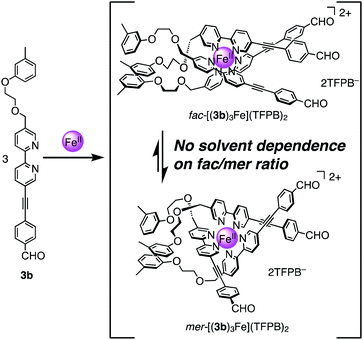 |
| Fig. 3 Chemical structures of both isomers of [(3b)3Fe](TFPB)2. | |
Correlation with Hansen solubility parameters
In order to clarify the factor involved in the solvent dependency of the fac/mer isomerism of [2bFe](TFPB)2, the correlations between the ΔG of the fac/mer isomerization and the major solvent parameters were evaluated in terms of the correlation coefficient R by a linear regression analysis (Fig. 4, Tables S1 and S3†). The correlations of ΔG with the dielectric constant ε and dipole moment μ, both of which are typical solvent parameters, were less than 0.7, indicating no strong correlation (Fig. 4a and b). The donor number DN and the acceptor number AN15 had very low correlation coefficients (|R| < 0.3), which suggested that coordination or basicity of the solvents did not affect this fac/mer isomerism (Fig. 4c and d). Meanwhile, the molar volume Vm showed a good correlation (R = 0.83) (Fig. 4e).
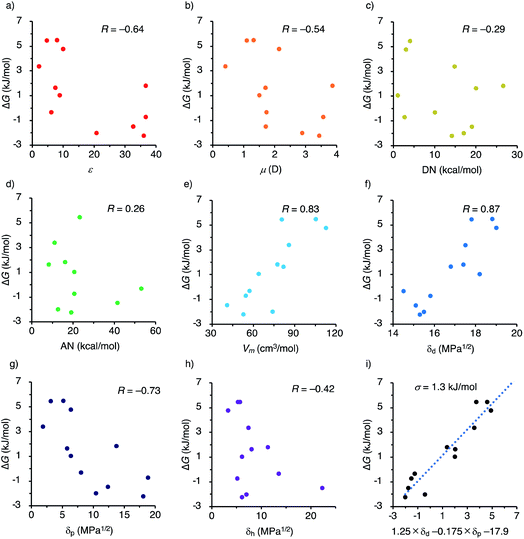 |
| Fig. 4 ΔG (mer to fac) plotted versus various solvent parameters and their correlation coefficient. (a) Dielectric constant ε,15a (b) dipole moment μ (D),15a (c) donor number DN (kcal mol−1),15a,b (d) acceptor number AN (kcal mol−1),15a (e) molar volume Vm (cm3 mol−1),14 (f–h) the Hansen solubility parameters,14 (f) dispersion term δd (MPa1/2),14 (g) dipole interaction term δp (MPa1/2),14 (h) hydrogen bonding term δh (MPa1/2),14 and (i) a prediction formula using δd and δp as explanatory variables. | |
Among the tested parameters, we found that the Hansen solubility parameters (HSPs)14 showed the strongest correlations. HSPs are the parameters used for the prediction of solubility, and represented by three independent values; i.e., the dispersion term (δd), the dipole interaction term (δp), and the hydrogen bonding term (δh). HSPs have long been used in the fields of polymers and coatings in which understanding of the interaction between solvents and solutes is essential, and been also recently applied to many fields such as drugs16 and supramolecular gels.17 We found that the ΔG of fac/mer isomerization is strongly correlated (R = 0.87) with δd, the dispersion term of HSPs (Fig. 4f). The correlations with the other two terms (δp and δh) were weak to moderate (Fig. 4g and h), but the multiple correlation coefficient with the combination of δd and δp was as high as 0.91 (Fig. 4i and Table S3†). Thus, this result suggested that a difference in the solvation by dispersion forces between the fac/mer isomers plays a key role in the solvent-dependent isomerization equilibrium.
Structural comparison of fac/mer isomers
In efforts to obtain single crystals of the complexes, we have succeeded in the structural analysis of fac-[1aFe](PF6)2 by X-ray diffraction measurements (Fig. 5a–c and S80†). As suggested by the 1H NMR measurements, the facial isomer has a pseudo C3 symmetry in the crystal. The methine proton of the triarylmethane pivot unit was directed outward. For comparison, the structure of mer-[1aFe](PF6)2 obtained using DFT calculations is shown in Fig. 5d–f. Considering the result of linear regression analyses and the difference in molecular structures, one possible explanation for the solvent-dependent isomerization is the degree of exposure to the solvent. The [Fe(bpy)3]2+ of the facial isomer is exposed to the solvent. Meanwhile, the self-penetrated bipyridyl unit of mer-[Fe(bpy)3]2+ is surrounded by the triarylmethane pivot and the ethylene glycol chains. As shown in Fig. 4, the correlation efficient between the fac/mer ratio and δd (R = 0.87) indicates that the solvation by the dispersion force is more favored for the mer isomer than the fac isomer. Meanwhile, the correlation efficient with δp (R = −0.73) indicates that the solvation by the dipole interaction is more favored for the fac isomer than the mer isomer. The structural difference of the fac/mer isomers, i.e., exposure of [Fe(bpy)3]2+ unit to the solvent, seems to be a factor to explain this dependency of the fac/mer ratio on the HSPs. Other possible factors to be included in the causes of the solvent-dependence are the inclusion of a solvent molecule in the fac-[Fe(bpy)3]2+ (an acetone molecule was found in the cavity, Fig. S80† (X-ray crystallography)) and the formation of an ion pair (Table S2, Fig. S72 and S73 (1H DOSY)†).
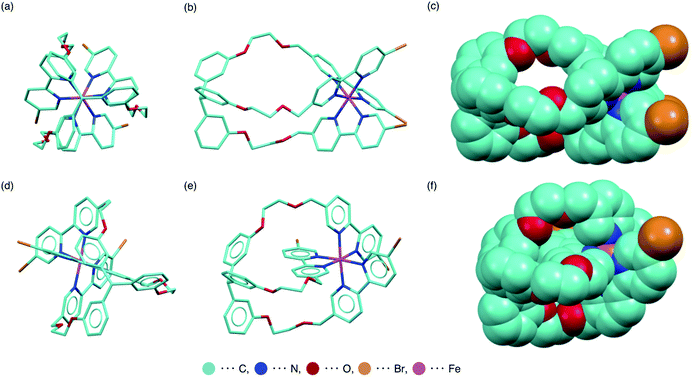 |
| Fig. 5 Comparison of the two isomers of [1aFe]2+ (hydrogen atoms are omitted. (a, b, d and e); stick model, (c and f); space-fill model). (a–c) A molecular structure of fac-[1aFe]2+ determined by X-ray diffraction analysis. (a) Top view. (b and c) Side view. (d–f) A structure of mer-[1aFe]2+ obtained by DFT calculation (B3LYP/6-31G*). (d) Top view. (e and f) Side view. | |
Reversibility in fac/mer conversion and self-assembly with diamines
The solvent-dependent fac/mer isomerization of [2bFe](TFPB)2 was found to be reversible (Fig. S82†). The sample was dried in vacuo, alternatively redissolved in CD3CN and CDCl3, and 1H NMR was performed. The interchange between the fac/mer ratios in the solvents can be repeated at least five times (Fig. S81†).
As a further application of [2bFe]2+ bearing three terminal formyl groups, the self-assembly via the Schiff-base formation was investigated (Fig. 6). [2bFe](PF6)2 was treated with 1.5 equiv. of 1,3-propanediamine in CD3CN/CDCl3 = 1/1. 1H NMR spectrum after the reaction revealed the disappearance of the signals of the formyl group at 10.00 ppm, and the appearance of a singlet of an imine bond at 8.31 ppm (Fig. S83†), which confirmed quantitative imine bond formation. The obtained product has C3 symmetry on the NMR time scale. The ESI-TOF mass measurements gave signals assigned to chemical species formed from two [2bFe]2+ and three diamines (Fig. S86†). The NMR and MS data indicated that the obtained product was the bicyclic dimer D-PPD4+ composed of the facial isomer (Fig. 6, spectral data of characterization are shown in Fig. S83–S86†). Thus, fac/mer equilibrium of [2bFe]2+ was completely shifted to the fac-isomer during the process of Schiff-base formation. When the carbohydrazide was used as a linker molecule, the 1H NMR and ESI-MS indicated that a similar bicyclic dimer, D-CHZ4+, was constructed (Fig. S88 and S89†). On the other hand, when trans-1,4-cyclohexanediamine reacted under the same condition, the ESI-TOF MS indicated that the species formed from four [2bFe]2+ and six diamines (Fig. S93†). The 1H NMR spectrum supported the quantitative formation of an imine bond and the high symmetry of the obtained product (Fig. S90†). These results suggested the formation of the tetrahedral tetramer T8+ composed of the facial isomer (Fig. 6, spectral data of characterization are shown in Fig. S90–S93†). The hydrodynamic radii evaluated from the DOSY spectra increased in the order of the mononuclear complex [2bFe](PF6)2, bicyclic dimer D-PPD(PF6)4, and tetrahedral tetramer T(PF6)8 (12, 17, and 25 Å, respectively, Table S4, Fig. S87 and S94†), which supported the formation of the assemblies. The discrete self-assemblies depending on the components can be applied to the dynamic combinatorial library or adaptive chemistry.18
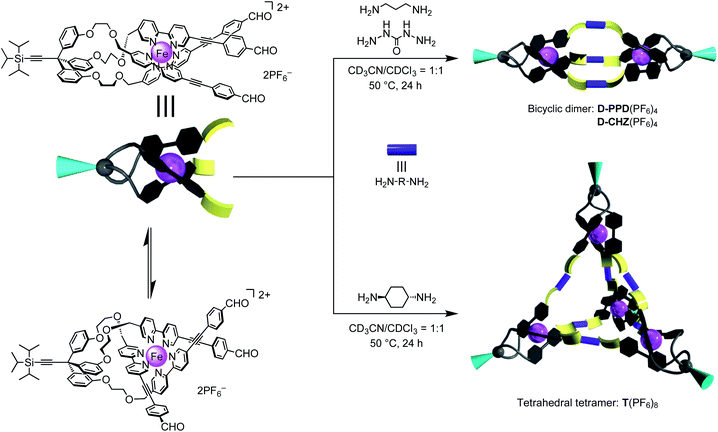 |
| Fig. 6 Bicyclic dimer D-PPD4+ and D-CHZ4+, and tetrahedral tetramer T8+, which are constructed by the imine bond formation of [2bFe]2+ existing as a fac/mer isomeric mixture. | |
Conclusions
We have synthesized novel tripodal ligands L (1a, 1b, 2a, and 2b), and revealed that the fac/mer ratios of their octahedral complexes [LM]X2 (M = FeII or ZnII) (X = PF6 or TFPB) largely depend on the solvents. This solvent-dependent isomerization occurs regardless of the counter anion, the metal center, and the substituents on the bipyridine and the pivot unit. It was found that the dispersion term δd of the Hansen solubility parameters (HSPs) showed a good correlation with ΔG of the fac/mer isomerization. Considering the good fitting result of this study, it would be interesting to apply HSPs to other supramolecular systems in which solvation plays an important role. Moreover, when using [2bFe]2+ as a component for the self-assembly via imine bond formation, discrete dimeric and tetrameric structures were obtained accompanied by the conversion from the fac/mer isomeric mixture to the pure facial isomer. This structure with an appropriate pivot part with flexible chains is demonstrated to be a good building block for supramolecular systems that change their structures in response to the environmental change.
Author contributions
R. M. and T. Nabeshima conceived the project. T. M., R. M. and T. Nabeshima designed the experiments. T. M. carried out the experimental work. T. M. and R. M. performed X-ray measurements and analysis. All the authors analysed the data, prepared the manuscript, and contributed to the writing of the paper.
Conflicts of interest
There are no conflicts to declare.
Acknowledgements
This research was supported by JSPS KAKENHI (Grant Numbers JP18H01959, JP19K15579, JP19K15578, JP19H04559, and JP20H05202), the Mazda Foundation, Inamori Foundation, Ogasawara Foundation, and Iketani Science and Technology Foundation.
References
-
(a) A. Grabulosa, M. Beley and P. C. Gros, Eur. J. Inorg. Chem., 2008, 1747–1751 CrossRef CAS
;
(b) L. Aboshyan-Sorgho, T. Lathion, L. Guénée, C. Besnard and C. Piguet, Inorg. Chem., 2014, 53, 13093–13104 CrossRef CAS
.
-
(a) M. A. Case, M. R. Ghadiri, M. W. Mutz and G. L. McLendon, Chirality, 1998, 10, 35–40 CrossRef CAS
;
(b) N. C. Fletcher, M. Nieuwenhuyzen and S. Rainey, J. Chem. Soc., Dalton Trans., 2001, 2641–2648 RSC
;
(c) M. Gochin, V. Khorosheva and M. A. Case, J. Am. Chem. Soc., 2002, 124, 11018–11028 CrossRef CAS PubMed
;
(d) M. Kyakuno, S. Oishi and H. Ishida, Chem. Lett., 2005, 34, 1554–1555 CrossRef CAS
.
- S. E. Howson, L. E. N. Allan, N. P. Chmel, G. J. Clarkson, R. van Gorkum and P. Scott, Chem. Commun., 2009, 1727–1729 RSC
.
-
(a) H. Weizman, J. Libman and A. Shanzer, J. Am. Chem. Soc., 1998, 120, 2188–2189 CrossRef CAS
;
(b) N. C. Fletcher, M. Nieuwenhuyzen, R. Prabaharan and A. Wilson, Chem. Commun., 2002, 1188–1189 RSC
;
(c) N. C. Fletcher, R. T. Brown and A. P. Doherty, Inorg. Chem., 2006, 45, 6132–6134 CrossRef CAS PubMed
;
(d) B. Brisig, E. C. Constable and C. E. Housecroft, New J. Chem., 2007, 31, 1437–1447 RSC
.
-
(a) S. E. Howson, L. E. N. Allan, N. P. Chmel, G. J. Clarkson, R. J. Deeth, A. D. Faulkner, D. H. Simpson and P. Scott, Dalton Trans., 2011, 40, 10416–10433 RSC
;
(b) M. Kieffer, B. S. Pilgrim, T. K. Ronson, D. A. Roberts, M. Aleksanyan and J. R. Nitschke, J. Am. Chem. Soc., 2016, 138, 6813–6821 CrossRef CAS
;
(c) T. Nakamura, S. Yonemura, S. Akatsuka and T. Nabeshima, Angew. Chem., Int. Ed., 2021, 60, 3080–3086 CrossRef CAS
.
-
(a) S. Sakai, Y. Shigemasa and T. Sasaki, Tetrahedron Lett., 1997, 38, 8145–8148 CrossRef CAS
;
(b) S. Sakai, Y. Shigemasa and T. Sasaki, Bull. Chem. Soc. Jpn., 1999, 72, 1313–1319 CrossRef CAS
.
- N. Ousaka, Y. Takeyama and E. Yashima, Chem.–Eur. J., 2013, 19, 4680–4685 CrossRef CAS PubMed
.
- V. Cámara, N. Barquero, D. Bautista, J. Gil-Rubio and J. Vicente, Chem.–Eur. J., 2015, 21, 1992–2002 CrossRef PubMed
.
-
(a) S. L. Dabb and N. C. Fletcher, Dalton Trans., 2015, 44, 4406–4422 RSC
;
(b) I. A. Riddell, M. M. J. Smulders, J. K. Clegg, Y. R. Hristova, B. Breiner, J. D. Thoburn and J. R. Nitschke, Nat. Chem., 2012, 4, 751–756 CrossRef CAS
;
(c) I. A. Riddell, Y. R. Hristova, J. K. Clegg, C. S. Wood, B. Breiner and J. R. Nitschke, J. Am. Chem. Soc., 2013, 135, 2723–2733 CrossRef CAS PubMed
;
(d) S. Zarra, J. K. Clegg and J. R. Nitschke, Angew. Chem., Int. Ed., 2013, 52, 4837–4840 CrossRef CAS
;
(e) R. A. Bilbeisi, T. K. Ronson and J. R. Nitschke, Angew. Chem., Int. Ed., 2013, 52, 9027–9030 CrossRef CAS PubMed
;
(f) I. A. Riddell, T. K. Ronson, J. K. Clegg, C. S. Wood, R. A. Bilbeisi and J. R. Nitschke, J. Am. Chem. Soc., 2014, 136, 9491–9498 CrossRef CAS PubMed
;
(g) I. A. Riddell, T. K. Ronson and J. R. Nitschke, Chem. Sci., 2015, 6, 3533–3537 RSC
.
-
(a) M. Albrecht, X. Chen and D. Van Craen, Chem.–Eur. J., 2019, 25, 4265–4273 CrossRef CAS PubMed
;
(b) X. Chen, C. Mevissen, S. Huda, C. Göb, I. M. Oppel and M. Albrecht, Angew. Chem., Int. Ed., 2019, 58, 12879–12882 CrossRef CAS PubMed
.
-
(a) T. Nabeshima, Bull. Chem. Soc. Jpn., 2010, 83, 969–991 CrossRef CAS
;
(b) T. Nabeshima, Y. Yoshihira, T. Saiki, S. Akine and E. Horn, J. Am. Chem. Soc., 2003, 125, 28–29 CrossRef CAS PubMed
;
(c) T. Nabeshima, Y. Tanaka, T. Saiki, S. Akine, C. Ikeda and S. Sato, Tetrahedron Lett., 2006, 47, 3541–3544 CrossRef CAS
;
(d) T. Nabeshima, S. Masubuchi, N. Taguchi, S. Akine, T. Saiki and S. Sato, Tetrahedron Lett., 2007, 48, 1595–1598 CrossRef CAS
.
- H. Morita, S. Akine, T. Nakamura and T. Nabeshima, Chem. Commun., 2021, 57, 2124–2127 RSC
.
- T. Nakamura, H. Kimura, T. Okuhara, M. Yamamura and T. Nabeshima, J. Am. Chem. Soc., 2016, 138, 794–797 CrossRef CAS PubMed
.
-
C. M. Hansen, Hansen Solubility Parameters: A User's Handbook, CRC Press LLC, Boca Raton, Florida, USA, 2nd edn, 2007 Search PubMed
.
-
(a) U. Mayer, V. Gutmann and W. Gerger, Monatsh. Chem., 1975, 106, 1235–1257 CrossRef CAS
;
(b) F. Cataldo, Eur. Chem. Bull., 2015, 4, 92–97 Search PubMed
.
-
(a) X. Zhang, Q. Yin, X. Li, M. Zhang, J. Huang, C. Wang, Z. Zhang, Y. Huang, M. Guo and Y. Li, J. Mol. Liq., 2017, 237, 46–53 CrossRef CAS
;
(b) A. M. Navarro, B. García, F. J. Hoyuelos, I. A. Peñacoba and J. M. Leal, Fluid Phase Equilib., 2016, 429, 127–136 CrossRef CAS
.
-
(a) J. Gao, S. Wu and M. A. Rogers, J. Mater. Chem., 2012, 22, 12651–12658 RSC
;
(b) M. Zhang, S. Selvakumar, X. Zhang, M. P. Sibi and R. G. Weiss, Chem.–Eur. J., 2015, 21, 8530–8543 CrossRef CAS PubMed
;
(c) T. Wang, X. Yu, Y. Li, J. Ren and X. Zhen, ACS Appl. Mater. Interfaces, 2017, 9, 13666–13675 CrossRef CAS PubMed
.
- J.-M. Lehn, Chem. Soc. Rev., 2007, 36, 151–160 RSC
.
Footnotes |
† Electronic supplementary information (ESI) available: Synthetic procedures, characterization data, and X-ray crystallographic analysis. CCDC 2067281. For ESI and crystallographic data in CIF or other electronic format see DOI: 10.1039/d1sc01529j |
‡ Present Address: Institute for Molecular Science, Okazaki, Aichi 444-8787, Japan. |
|
This journal is © The Royal Society of Chemistry 2021 |
Click here to see how this site uses Cookies. View our privacy policy here.