DOI:
10.1039/D0RA06161A
(Review Article)
RSC Adv., 2020,
10, 38937-38964
Application of bacteriocins in food preservation and infectious disease treatment for humans and livestock: a review
Received
15th July 2020
, Accepted 5th October 2020
First published on 23rd October 2020
Abstract
Infectious diseases caused by bacteria that can be transmitted via food, livestock and humans are always a concern to the public, as majority of them may cause severe illnesses and death. Antibacterial agents have been investigated for the treatment of bacterial infections. Antibiotics are the most successful antibacterial agents that have been used widely for decades to ease human pain caused by bacterial infections. Nevertheless, the emergence of antibiotic-resistant bacteria has raised awareness amongst public about the downside of using antibiotics. The threat of antibiotic resistance to global health, food security and development has been emphasized by the World Health Organization (WHO), and research studies have been focused on alternative antimicrobial agents. Bacteriocin, a natural antimicrobial peptide, has been chosen to replace antibiotics for its application in food preservation and infectious disease treatment for livestock and humans, as it is less toxic.
1. Introduction
A pathogen is an organism, which can be an infectious agent in causing diseases in human beings, animals or plants. Pathogens raise concerns from us due to their impacts on human health by infection via foods, livestock or animals. Previously, antimicrobial drugs or antibiotics have been chosen in the treatment of infectious diseases caused by pathogens. However, the increase of the usage of antibiotics in killing pathogens has led to the emergence of antibiotic-resistant bacteria, which will be the next challenges for humankind.1 Recently, studies on bacteriocin or bacteriocin-like inhibitory substances (BLIS) have been focused on finding out an alternative therapeutic option for bacterial infections. Bacteriocin has been chosen as a potential drug candidate to replace chemicals and antibiotics in future due to its lower toxicity and proteinaceous nature. Besides, the usage of antibiotics in killing pathogens may cause a disruption of the gut microbiota, as it is not only killing the targeted microbial community, but also the surrounding microbial community, as shown in Fig. 1(a).2 Consequently, the disruption of the gut microbiota leads to immunological, metabolic and neurological disorders.3 In contrast, bacteriocin re-shape the microbiota by killing the targeted pathogens without killing the other surrounding microbial community, as shown in Fig. 1(b).4 Bacteriocin is a natural antimicrobial peptide produced by bacteria to protect themselves from other bacteria or pathogens by inhibiting or killing it without harming themselves.5 Bacteriocin is usually produced by lactic acid bacteria (LAB) or other bacteria, such as Bacillus strains, Staphylococcus strains and Escherichia coli strains. LAB is a group of Gram-positive bacteria with high tolerance to acidic environment, non-spore forming, either rod or spherical in shape, which shares similar characteristics in terms of metabolism and physiology.6 They play a vital role in fermentation by using carbohydrates as their main source, producing lactic acids as the main product.7 Basically, LAB can be divided into a few genera, which comprise Lactobacillus, Lactococcus, Leuconostoc, Pediococcus, Streptococcus, Aerococcus, Alloiococcus, Carnobacterium, Dolosigranulum, Enterococcus, Oenococcus, Tetragenococcus, Vagococcus and Weissella.8 They are mainly grouped into two categories, which are homofermentative and heterofermentative LAB. During the process of fermentation, lactic acid is mainly produced by homofermentative LAB, whereas lactic acid, acetic acid or alcohol and carbon dioxide are produced by heterofermentative LAB.9 From previous studies, some LAB were found to have an ability to produce antimicrobial substances, especially bacteriocin, to protect themself from other spoilage bacteria and pathogen.
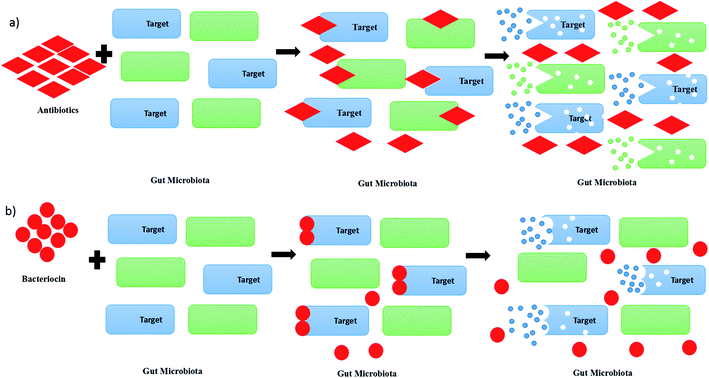 |
| Fig. 1 Killing or inhibition actions of (a) antibiotics and (b) bacteriocin on gut microbiota. | |
Bacteriocins can be categorized into mainly three classes. Class I bacteriocins are made of peptides that are small in size, which is less than 5 kDa. They contain specific post-translationally modified residues, which include lanthionine and β-methyllanthionine. The representative bacteriocin from class I are nisin Z, A and Q, enterocin W and nukacin ISK-1.10 Class II bacteriocins are bacteriocin with sizes of less than 10 kDa, resistant to heat, non-modified and hydrophobic peptides. Usually, they can be grouped into two sub-classes, which are class IIa and class IIb. Class IIa bacteriocin, such as leucocin A and pediocin PA1, are widely used in food preservation due to its pediocin-like Listeria. Class IIb bacteriocins exert or improve the antimicrobial effects via synergistic activity of two complementary peptides, such as plantaricin A and enterocin X. They contain amphiphilic and hydrophobic regions, and are mostly cationic and active in the range of nanomolar to picomolar concentrations.11 Class III bacteriocins are made of large proteins with sizes of more than 30 kDa, and are likely to be altered or degraded when subjected to heat. The representative bacteriocins from class III are lysostaphin, enterolysin A, and helveticin J.12 Nisin Z is a bacteriocin used to reduce the adhesion of Candida albicans to human oral cells.13 It is a class I bacteriocin with a size of 3.5 kDa that can be extracted from Lactococcus lactis NZ22186 or L. lactis NZ9800.14 Besides that, the combination of nisin, pediocin 34 and enterocin FH99 was found to have high antimicrobial activity against Listeria monocytogenes ATCC 53135.15 There are so many studies that have been done on the investigation of the purification techniques and application of bacteriocin. In this paper, the application of bacteriocin in food preservation, livestock health and medicine will be discussed.
2. Application of bacteriocins in food preservation
Living environments, which include soil, sea, river and air, are occupied with microorganisms.16 Some of them may cause food and beverage contamination, which leads to food and beverage spoilage. Food and beverage spoilage is always a concern in the food industry, as it may destroy the taste of the food and beverage, as well as cause some foodborne illnesses in human beings.17 The harmful microbes that cause foodborne illness can be divided into five groups, which are bacteria, viruses, parasites, protozoa, and fungi.18 They are called pathogens, in which they are usually found as the root causes of food intoxication and food infection.19 Food intoxication is caused by toxic substances produced by bacteria in food, leading to a rapid reaction of our body system after consuming the contaminated food.20 For instance, Staphylococcus aureus can cause inflammation of the small intestine in humans after consuming food contaminated with enterotoxin produced by it.21 Food infection will occur after eating the food containing living pathogens that are able to grow and multiply in the human intestinal tract, leading to an intestinal infection.22 However, the reaction will be slower. One of the examples is diarrhea illness in humans, which is caused by Salmonella infection.23
Chemical additives have been used widely to preserve food, but they can cause a lot of human health problems due to the toxicity of the chemical additives. This concern has led to the high demand of natural and chemical-free products used to preserve food in the market to avoid health problems.24 Thus, so many research studies have been recently done on investigating bio-preservatives that can be used for inhibiting pathogens that can cause food spoilage.25–28 Bio-preservation refers to the method of using non-pathogenic microorganisms or metabolites produced by microorganisms to prolong the storage period of the food.29 The most remarkable bio-preservative that has been used in food factories to prevent food spoilage is bacteriocin or BLIS, such as nisin, pediocin, enterocin and leucocin.26 Table 1 shows the bacteriocins used in food preservation, which includes nisin, enterocin, pediocin, leucocin, lactoccocin, carnocyclin, carnobacteriocin, piscicolin, sukacin, aureocin, mycocin, bacteriocin 7393A, bacteriocin 7293B and bacteriocin CAMT2.
Table 1 Application of bacteriocin from LAB in food preservation
Producing strain |
Types of bacteriocin |
Food application |
Targeted pathogens |
Pros and cons |
References |
L. lactis spp |
Nisin |
Cheddar cheese |
L. monocytogenes and S. aureus |
Pros: from sensory test, the acidic and bitter taste of cheddar cheese can be improved by adding nisin |
38 and 167 |
Cons: the use of nisin in free form is costly and low in stability |
Milk and milk products |
B. cereus, C. botulinum and C. perfringens |
Pros: the taste of milk is not affected by the additional of nisin |
39 and 168 |
Cons: the stability of nisin at neutral pH is low and the binding of cationic nisin to anionic casein will occur |
Dairy, culinary, bakery products and beverages |
L. monocytogenes, B. cereus and C. botulinum |
Pros: the taste of dairy, culinary, bakery products and beverages is not affected by the additional of nisin |
40 and 168 |
Cons: the stability of nisin at neutral pH is low and the binding of cationic nisin to anionic casein may occur |
Meat and sausages |
C. botulinum and L. monocytogenes |
Pros: the taste of meat and sausages is not affected by the additional of nisin |
31 and 41 |
Cons: the solubility of nisin to meat and sausage product is low and enzymatic destruction may occur |
L. lactis MG1614 |
Enterocin A |
Cottage cheese |
L. monocytogenes |
Pros: plasmid pEnt02 that containing entA gene can be introduced into a L. lactis strain in the production of cottage cheese. The number of pathogen can be reduced to below detectable level within two days with enterocin |
57 and 169 |
E. faecium CTC492 and L. sakei CTC494 |
Enterocins A and B, and sakacin K |
Cooked pork |
L. sakei CTC746 |
Pros: the reductions of up to 8 and 9 orders of magnitude in products stored at 7 °C for 37 days |
60 and 170 |
Cons: the hydrophobic molecules of the enterocins may bind to the hydrophobic phase of cooked pork (emulsion) which may reduces the activity of bacteriocin |
E. faecium WHE 81 |
Enterocins A and B |
Munster cheese |
L. monocytogenes |
Pros: no growth of L. monocytogenes was found even after 20 days |
169 |
E. faecium CTC492 |
Enterocins A and B |
Dry fermented sausages |
L. innocua |
Pros: enterocins are found to be inactive against LAB but active against L. innocua |
61 |
Cooked ham |
L. monocytogenes |
Pros: 2000 AU per cm2 of enterocin A and B with air packaging and vacuum packaging can reduce the growth of L. monocytogenes significantly for 8 and 15 days, respectively |
61 and 171 |
Cons: for long term storage, the cooked ham with enterocin A and B need to be submitted to a high pressure treatment of 600 MPa which may increase the cost |
Cooked ham blended with distilled water |
L. monocytogenes and L. sakei |
Pros: enterocins A and B reduce the growth of L. monocytogenes and L. sakei throughout the storage period |
61 |
Cons: for long term storage, the pressurization process is needed, which will increase the cost |
E. faecium F58 |
Enterocins L50A and B |
Goat's milk and goat milk's cheese |
L. monocytogenes |
Pros: the growth of L. monocytogenes can be inhibited to a undetectable level even after one week storage at 22 °C |
169 |
E. casseliflavus IM 416K1 |
Enterocin 416K1 |
Italian sausages and cottage cheese |
L. monocytogenes |
Pros: enterocin can either be added to the sausages with 10 AU per g or produced in situ by the addition of about 105 cfu g−1 of producer strain. The L. monocytogenes counts were found to be decreased significantly (P < 0.05) during storage for both |
62 |
Cons: L. monocytogenes (at below detection limit) can only be achieved by inoculation of enterocin producing strain to the sausage for long term storage |
E. faecalis A-48-32 |
Enterocin AS-48 |
Non-fat hard cheese |
B. cereus |
Pros: no viable of B. cereus found in the milk used in cheese production that coinoculated with AS-48-producing strain and B. cereus after 72 h |
172 |
Skimmed milk and non-fat unripened soft cheese |
S. aureus |
Pros: E. faecalis A-48-32 can produce high amounts of AS-48 in milk and give higher inhibition zones which is around 18–20 mm in the inhibition test for S. aureus |
38 and 173 |
Cons: for long term storage, heat treatment at 65 °C is needed to reduce the amount of enterocin used for cost reduction |
Infant rice-based food |
B. cereus |
Pros: the viable cell count of B. cereus decreases rapidly with adding of 20–35 μg mL−1 enterocin. Enterocin AS-48 also increase the heat sensitivity of endospores in which the inactivation of endospores can be achieved at 90 °C and 95 °C for 1 min for boiled rice and rice-based gruel, respectively |
49 and 174 |
Fruit juices |
A. acidoterrestris |
Pros: the enterocin activity is very stable for 15 days at 4 °C, and the activity is still detectable after 30 days |
49 and 175 |
Cons: the activity is inactivated faster at room or higher temperature. Activity of bacteriocin is losing gradually in juices stored at 15 and 28 °C |
Apple cider |
B. licheniformis |
Pros: in fresh-made apple cider, 3 μg mL−1 of enterocin AS-48 can inactivate B. licheniformis rapidly. Besides that, it also increases the heat sensitivity of spores |
49 and 176 |
Vegetable soups and puree |
B. cereus, B. macroides and Paenibacillus spp. |
Pros: Bacillus strains are completely inhibited with 10 μg mL−1 of enterocin AS-48 in all six vegetable products tested, which include natural vegetable cream, asparagus cream, traditional soup, homemade-style traditional soup, vegetable soup, and vichyssoise for up to 30 days at 6, 15, and 22 °C |
177 |
Cons: cocktails of B. cereus, B. macroides and Paenibacillus spp exhibit higher resistance to enterocin AS-48, in which up to 50 μg mL−1 of enterocin AS-48 required to inactivate them at 22 °C. The effectiveness of enterocin on the cocktail in natural vegetable cream can only be enhanced by combination with nisin |
Vegetable sauces |
S. aureus |
Pros: only 25 μg mL−1 of enterocin AS-48 is needed for the inactivation of all staphylococci in napoletana and pesto sauces stored at 22 °C. All detectable staphylococci in napoletana, pesto, and green sauce for fish can be eliminated at 80 μg mL−1 of enterocin AS-48 regardless of storage temperature |
49 and 178 |
Cons: limited effect of enterocin was found in carbonara sauce. The combinations of 80 μg mL−1 AS-48 and 20 mM hydrocinnamic acid or 126 mM carvacrol are needed to reduce the staphylococci below detection limits for up to 30 days at 22 °C in carbonara sauce |
Cooked ham |
L. monocytogenes |
Pros: enterocin AS-48 with concentration of 20–60 μg g−1 can work actively against L. monocytogenes at 5 and 15 °C |
49 and 179 |
Cons: enterocin alone is not sufficient to avoid the regrowth of L. monocytogenes during the 60 days storage. Combination of enterocin at 40 μg g−1 with 0.007% of nitrite/nitrate can reduce L. monocytogenes to below detection level from beginning to end of the storage |
Canned fruits and vegetable foods |
B. coagulans |
Pros: no viable cells are detected in any samples after 15 days with enterocin AS-48 |
180 |
Cons: enterocin AS-48 shows no significant effect on B. coagulans spores. The combination of enterocin AS-48 and heat treatment at 80–95 °C for 5 min is needed to eliminate the spores |
E. faecium CCM 4231, E. faecium, RZS C13 and L. sakei CTC494 |
Enterocin CCM 4231, enterocin 13 and sakacin K |
Spanish style dry fermented sausages |
L. monocytogenes and L. innocua |
Pros: the taste of sausages is not affected and the bacteriocins are active against L. monocytogenes and L. innocua but not LAB |
63 |
E. faecalis BFE 1071 |
Enterocin 1071 A and B |
Fish spread |
L. innocua, S. epidermis and P. vulgaris |
Pros: the number of microbial cell can be reduced from 1 × 108 to 8 × 106 in fish spread with enterocin after 21 days at 4 °C |
64 and 181 |
Cons: enterocin is not proved to inactivate the pathogens in fish spread to below detectable level |
E. faecium CRL35 |
Enterocin CRL 35 |
Goat cheese |
L. monocytogenes |
Pros: enterocin CRL 35 is stable at extreme pH, heat treatment and storage in different conditions |
52 and 182 |
E. faecalis EJ97 |
Enterocin EJ97 |
Vegetable (Zucchini) puree |
B. macroides and B. maroccanus |
Cons: inhibition of B. macroides and B. maroccanus in vegetable puree needs 10-fold of enterocin EJ97 higher than in cell culture |
183 |
E. faecalis N1-33 |
Enterocin MR-10A |
Custard cream |
B. cereus |
Pros: B. cereus in custard cream was found to be inhibited for 3 days at neutral pH. It is also stable to heat and cooking process |
66 |
E. faecium L50 |
Enterocins L50A and B |
Alcoholic and non-alcoholic beer |
L. brevis and P. damnosus |
Pros: enterocins L50A and B can withstand heat treatment, which is beneficial to the alcoholic and non-alcoholic beer. It still retain the antimicrobial activity after long term storage at 8 and 25 °C |
66 |
E. faecium CCM 4231 |
Enterocin CCM 4231 |
Skimmed milk and yoghurt |
S. aureus and L. monocytogenes |
Pros: the viable bacterial cells were decreased by 3.77 log cfu mL−1 in skimmed milk and yoghurt |
63 and 184 |
Cons: this application at present is legislation and lack of experimental data |
Bryndza |
L. innocua, E. coli and S. aureus |
Pros: pathogen in sample is inhibited with 6400 AU per mL |
63 |
Cons: the inhibitory effect of enterocin on L. innocua, E. coli and S. aureus in Bryndza only last for 4 days, 103 cfu mL−1 g−1 is found on samples on 7th day |
Saint-Paulin cheese |
L. monocytogenes |
Pros: the taste of cheese is not affected by the addition of enterocin |
37 and 185 |
Cons: the bacteriocin activity cannot be tested via agar test even it is clearly shown in 1 week cheese |
Dry fermented Hornad salami |
L. monocytogenes |
Pros: pH and the water content of the salami were not affected |
186 |
P. acidilactici MCH14 |
Pediocin PA-1 |
Dried sausages and fermented meat products |
L. monocytogenes and C. perfringens |
Pros: 5000 BU per mL of pediocin PA-1 was proved able to reduce the L. monocytogenes by 2 or 0.6 log cycles after storage at 4 °C for 60 days and at 15 °C for 30 days, respectively, as compared to the control. Besides that, 5000 BU per mL of pediocin can reduce C. perfringens by 2 and 0.8 log cycles after storage at 10 °C for 60 days and at 15 °C for 30 days, respectively, as compared to the control |
69 |
Salad dressings |
Lactobacillus biofermentans |
Pros: the taste of salad dressings is not affected by the bacteriocin |
70 |
Fresh beef, vacuum-packed beef, cottage cheese, ice cream mix |
Ln. mesenteroides |
Pros: the activity of pediocin PA-1 is stable in the environment of food, and the taste of foods was not affected by the bacteriocin |
71 |
Fish fillets, chicken meat |
L. monocytogenes |
Pros: the taste of foods was not affected by the bacteriocin |
72 |
Sous vide products |
B. subtilis, B. licheniformis |
Pros: with the presence of pediocin in sous vide products, no vegetative cells is detected after 90 days |
46 and 187 |
Cons: pediocin alone is not enough in the preservation of sous vide products. Combination of pediocin and nisin is needed as nisin is more effective in reducing the thermal resistance of B. subtilis spores and pediocin is more effective against B. licheniformis |
E. faecium NCIM 5423 and L. plantarum Acr2 |
Pediocin PA-1 |
Fermented soymilk products |
L. monocytogenes |
Pros: the curd formed from the soymilk fermentation has good firmness, anti-oxidant property and acceptable sensory score in the presence of the bacteriocin. The shelf life of soymilk product is extended |
188 |
P. pentosaceous 34 |
Pediocin 34 |
Milk products and meat |
L. monocytogenes |
Pros: the pH and taste of milk products and meat are not affected by pediocin 34 |
15 |
Ln. gelidum UAL187 |
Leucocin A |
Milk product, fresh meat and sausage |
L. monocytogenes |
Pros: the activity of leucocin A on L. monocytogenes is stable in foods |
76 |
Meat |
C. divergens UAL9 |
Pros: the storage of meat is extended and the taste is not affected |
77 |
Ln. mensenteroides K7 |
Leucocin K7 |
Milk |
L. monocytogenes |
Pros: 80 AU per mL of leucocin K7 able to inhibit the growth of L. monocytogenes by 2.3–3.9 log units in milk |
189 |
Cons: leucocin alone is unable to eliminate L. monocytogenes in milk. For completely eliminate L. monocytogenes over 7 days, leucocin need to be mixed with 5 mg mL−1 of glycine |
Ln. pseudomesenteroides KM432Bz |
Leucocin B-KM432Bz |
Spanish style dry fermented sausages |
L. innocua CIP 80.11 |
Pros: the inhibitory effect of leucocin B-KM432Bz on L. innocua CIP 80.11 is 4 to 32 fold better efficiency than pediocin PA-1 |
78 and 190 |
Meat and meat products |
L. monocytogenes CIP 82.110 |
Pros: the MIC of leucocin B-KM432Bz needed to inhibit L. monocytogenes CIP 82.110 is lower than pediocin PA-1. The MIC of leucocin B-KM432Bz and pediocin PA-1 needed to inhibit L. monocytogenes CIP 82.110 are 16 and 512 nM, respectively |
79 and 190 |
Leuconostoc carnosum Ta11a |
Leucocin B |
Meat and meat products |
L. monocytogenes |
Pros: the pH and taste of meat and meat products are not affected |
79 |
L. lactis spp. (lactis BZ) |
Lactococcin BZ |
Both skim and full-fat milk |
L. monocytogenes |
Pros: lactococcin BZ has high antilisterial activity. The viable cell numbers of L. monocytogenes can be reduced to undetectable level with 400–2500 AU per mL of lactococcin BZ at 4 °C or 20 °C |
191 |
Cons: the antilisterial activities of lactococcin BZ will be decreased by increasing the fat content of milk |
C. maltaromaticum UAL307 |
Carnocyclin A, carnobacteriocin BM1 and piscicolin 126 |
Milk products and meat |
E. coli DH5α, P. aeruginosa ATCC 14207 and S. typhimurium ATCC 23564 |
Pros: carnocyclin A, carnobacteriocin BM1 and piscicolin 126 have high antimicrobial activities against pathogens in milk products and meat as compared to leucocin A. Besides that, the taste of food is not affected |
81 |
L. sakei |
Sukacin |
Meat product |
L. monocytogenes |
Pros: the pH and taste of meat product are not affected by sukacin |
80 |
S. aureus |
Aureocin A70 |
Skimmed milk |
L. monocytogenes |
Pros: aureocin A70 is not toxic to the Vero and the L-929 cell lines. Besides that, it does not exhibit a hemolytic activity against sheep red blood cells. Aureocin A70 was proved to be completely stable for one month at 25 °C, 16 weeks at 4 °C and 20 weeks at −20 °C |
192 |
S. natalensis or S. gilvosporeus |
Natamycin |
Cheese, fresh dairy products, processed meat and beverages |
Yeasts and molds |
Pros: the inhibitory activities of natamycin were stable, and it does not affect the taste of foods |
193 |
D. hansenii DSMZ70238 |
Mycocin |
Meat and meat products |
L. monocytogenes |
Pros: the antibacterial effect of mycocin on meat and meat products is stable during storage and the taste of meat is not affected |
85 |
W. hellenica BCC 7239 |
Bacteriocins 7293A and 7293B |
Meat and meat products |
P. aeruginosa, A. hydrophila, S. typhimurium and E. coli |
Pros: the bacteriocins are stable in the environment of foods and it does not affect pH and taste of foods |
31 |
B. amyloliquefaciens ZJHD3-06 |
Bacteriocin CAMT2 |
Meat, milk products and meat products |
L. monocytogenes, S. aureus, E. coli and Vibrio parahaemolyticus |
Pros: the bacteriocin is stable to heat up to 100 °C and pH with range pH 2–10 |
86 and 194 |
2.1 Nisin
Nisin is one of the bacteriocins that has been approved by the US Food and Drug Administration (FDA) and World Health Organization (WHO) to be applied in food factories.30 The first identification of Nisin was done in 1928 from fermented milk cultures, and sold in England in 1953 as an bio-preservative due to its ability to inhibit the growth of pathogens, which is beneficial to food preservation.31 Nisin is a bacteriocin with a molecular size of 3354 kDa, and is made up of 34 amino acids. It is produced by Gram-positive bacteria, including Lactococcus and Streptococcus species. Nisin belongs to class I (lantibiotic) bacteriocin, which contains five lanthionine rings. Its solubility in water and stability can be increased by decreasing the pH value, with the highest solubility of 57 mg mL−1 achieved at pH 2–3.32 Nisin is safe to be used for humans with a concentration of less than 83.25 mg kg−1 and less than 66.7 mg kg−1 for mouse. However, it may cause a contraceptive effect in humans at a concentration of 300–400 μg mL−1.33 Nisin is found to exert inhibition activities against many groups of Gram-positive foodborne bacteria, such as Bacillus cereus, Clostridium botulinum, L. monocytogenes and S. aureus.34 Besides that, many studies proved that the combination of nisin with antibiotics can kill or inhibit the growth of Gram-negative pathogens. Up to now, there are a lot of natural and bio-engineered variants of nisin that have been reported. Natural nisin refers to the nisin that has been extracted from Lactococcus or Streptococcus strains without gene modification, while the bio-engineered nisin variant is derived from the genetically modified Lactococcus or Streptococcus strains to enhance their inhibitory effects against Gram-negative pathogens.35 For example, nisin Z can be produced by L. lactis NZ22186 naturally, while nisin Z N20K can be extracted from the genetically modified L. lactis NZ9800.36
Nisin plays a vital role in preventing food spoilage, as it can kill or inhibit many foodborne pathogens in a wide range of foods, either in liquid form or solid form.37 Nisin is widely used to inhibit the growth or kill L. monocytogenes and S. aureus in cheese to prolong its storage duration.38 Besides that, a previous study demonstrated that the prevention of milk and milk product spoilage can be done by adding nisin to the food matrix to inhibit the growth of B. cereus, C. botulinum and Clostridium perfringens.39 Furthermore, L. monocytogenes, B. cereus and C. botulinum are always found in dairy, culinary, bakery products and beverages, which may cause food spoilage and bacterial infection. Nisin, as a antimicrobial agent, can inhibit the growth of these pathogens and prolong the expiration date.40 Nisin is also used in meat and meat products to inhibits the growth of C. botulinum and L. monocytogenes.41 From previous studies, nisin Z was proved to exhibit inhibition activity against L. monocytogenes 13 and 699 with MIC of 1 and 0.2 μg mL−1, respectively.42 Besides that, nisin Z was found to exert inhibitory activity against methicillin-resistant Staphylococcus aureus (MRSA), a pathogen that might cause skin and lung infection in humans with MIC of 4.17 μg mL−1.43 B. cereus is a Gram-positive, motile and facultative anaerobe with a rod-like shape that may cause abdominal pain, watery diarrhea, rectal tenesmus and moderate nausea.44 B. cereus IFR-NL94–25 was proved to be inhibited by nisin with MIC around 5–10 μg mL−1.45 Furthermore, 25 μg mL−1 of MIC of nisin was needed for the inhibition of C. botulinum.46 Moreover, C. perfringens is a Gram-positive anaerobe with a rod-like shape that may cause food poisoning, leading to diarrhea and abdominal cramps.47 It was proved to be inhibited by nisin with MIC at 0.75 μg mL−1.48
2.2 Enterocin
Enterocin is a circular bacteriocin produced by Enterococcus spp., which comprises 70 amino acid chains. Enterococcus is a Gram-positive bacteria that belongs to the large genus of LAB.49 Two species of Enterococcus that can be found in the human intestines are Enterococcus faecalis and Enterococcus faecium.50 In 1899, Thiercelin was the first scientist to describe the Enterococci group as ‘enterocoque’ due to their enteral habit. However, the genus Enterococcus was described by Thiercelin and Jouhaud in 1903, and classification of Streptococci was done by Lancefield in 1933.51 Basically, enterocin can be divided into four classes. However, classes II and III enterocins, such as enterocin AS-48, have been of interest due to their ability to inhibit a wide range of pathogens that cause food spoilage. Basically, enterocin can be used as a food preservative in two ways, either produce it with in situ method, in which enterocin-producing strains are added to food to produce enterocin, or adding purified or semi-purified enterocin to food for preventing food spoilage.52 The Enterococcus species are used wisely as a artisanal starter culture in dairy food production, such as cheeses.53 Meanwhile, some of the enterococcal strains are able to produce enterocin, which can inhibit the growth of other foodborne pathogens in food, prolonging the storage period. The in situ bacteriocin production is definitely giving double advantages to food industry, in which the enterococcal strain can be used as a starter culture for food and as a food preservative.52 However, many researchers have proved that the use of purified or semi-purified enterocin for the sole purpose of food preservation is more beneficial, as compared to in situ enterocin production, due to some negative effects of the enterocin-producing strains to the environment of hostile foods.38
Nowadays, there is a trend to take ready-to-eat vegetables without or with minimal food processing to minimize the lost nutrients. Nevertheless, the consumption of ready-to-eat vegetables may cause food contamination by pathogens, leading to infectious diseases in humans.54 To solve this problem, the use of enterocin AS-48 produced by E. faecalis A-48-32 was found to be an effective antimicrobial agent in inhibiting the growth of B. cereus, Bacillus macroides, Paenibacillus spp. and S. aureus in fresh vegetable sources. The growth of B. cereus, B. macroides, Paenibacillus polymyxa, Paenibacillus amylolyticus and S. aureus in food was proved to be inhibited by 10 μg mL−1 of enterocin AS-48.49 Besides that, enterocin AS-48 is also used to preserve soybean sprouts, canned fruits and vegetable foods by inhibiting the growth of L. monocytogenes at MIC of 1 μg mL−1 and Bacillus coagulans at MIC of 6 μg mL−1.49 Milk is a dairy product consumed by humans for the source of protein, and it is widely used in cheese production. However, it can be easily contaminated with pathogens due to its high nutrient content. Enterocin plays an important role in preserving milk and milk products. There have been so many previous research studies done on investigating the inhibitory effects of enterocin on the pathogens found in milk and milk products.31,55,56 Enterocin A from E. faecium CTC492 and Enterocin 416K1 from E. casseliflavus IM 416K1 were found to be used in cottage cheese to inhibit the growth of L. monocytogenes with 4.57 μg mL−1 of MIC.57 The combination of enterocins A and B was also applied in the bio-preservation of munster cheese against the pathogen L. monocytogenes.58 For the preservation of goat's milk and goat milk's cheese, enterocins L50A and B from E. faecium F58 and enterocin CRL 35 from E. faecium CRL35 were utilized in the inhibition of the growth of L. monocytogenes.52 In the production of non-fat hard cheese, enterocin AS-48 produced by E. faecalis A-48-32 was added to inhibit the growth of B. cereus.49 Furthermore, it was also used in the preservation of skimmed milk to inhibit the growth of S. aureus.38 Besides that, enterocin CCM 4231 from E. faecium CCM 4231 was claimed to have antimicrobial effects against S. aureus and L. monocytogenes in the preservation of skimmed milk, yoghurt and Saint-Paulin cheese.37 In addition, enterocin is also widely used in meat, fish, meat products and some beverages.59 Enterocins A and B, and sakacin K from E. faecium CTC492 and L. sakei CTC494, respectively, were used to inhibit the growth of Lactobacillus sakei CTC746 for maintaining the quality of cooked pork.60 Enterocins A and B produced from E. faecium CTC492 were claimed to have antimicrobial effects against pathogens, such as Listeria innocua, L. monocytogenes and L. sakei, that can be found in dry fermented sausages and cooked ham.61 Enterocin 416K1 extracted from Enterococcus casseliflavus IM 416K1 was found to be a bio-preservative in Cacciatore (Italian sausages) to inhibit the growth of L. monocytogenes.62 Furthermore, enterocin AS-48 produced by E. faecalis A-48-32 is used widely in the preservation of infant rice-based food, fruit juices and apple cider against the growth of B. cereus, Alicyclobacillus acidoterrestris and Bacillus licheniformis.49 The combination of enterocin CCM 4231, enterocin 13 and sakacin K from E. faecium CCM 4231, E. faecium RZS C13 and L. sakei CTC494, respectively, were demonstrated to have antimicrobial effects against L. monocytogenes and L. innocua in Spanish style dry fermented sausages.63 In the production of fish spread, enterocin 1071 A and B extracted from E. faecalis BFE 1071 was found to have an inhibitory effect against L. innocua, Staphylococcus epidermis and Proteus vulgaris.64 It was claimed that enterocin EJ97 from E. faecalis EJ97 can be used in the production of vegetable (Zucchini) puree to inhibit B. macroides and Bacillus maroccanus.65 Besides that, B. cereus (that causes spoilage of custard cream) can be inhibited by enterocin MR-10A produced by E. faecalis N1-33. In the production of alcoholic and non-alcoholic beer, enterocins L50A and B from E. faecium L50 play the important roles in inhibiting the growth of Lactobacillus brevis and Pediococcus damnosus for preventing spoilage.66
2.3 Pediocin
Pediocin is a class II bacteriocin with a molecular weight of 2.7–17 kDa, which comprises a hydrophilic N-terminal and a hydrophobic C-terminal variable.67 It was first described in 1990. It is made of 44 non-posttranslational modified peptides, which comprises aliphatic and aromatic amino acids.68 Pediocin, which is produced by Pediococcus strains, has high stability towards heat, a wide range of pH values, and some protease enzymes. Pediocin was found to have ability in the inhibition of pathogens that may cause food spoilage, such as C. perfringens and L. monocytogenes, by absorbing the amino acids at the phospholipid layer of the cytoplasmic membrane of the targeted cells.69 Pediocin can be applied in food via two ways, either through the in situ method by inoculating the food matrix with Pediococcus, Enterococcus or Lactobacillus strains with the optimal control to produce pediocin for inhibiting the growth of pathogens in food, or the direct use of pediocin to the food matrix with optimal concentration. However, adding pediocin directly to food has some disadvantages, such as changes in its solubility and amphiphilic nature.38
Pediocin plays a vital role in preventing the problems of food and beverage spoilage. For instant, pediocin PA-1 produced by Pediococcus acidilactici MCH14 was demonstrated to exert antimicrobial effects against L. monocytogenes and C. perfringens, which extend the shelf life of dried sausages and fermented meat products. The count of L. monocytogenes was proved to be reduced by 2 log cycle after 1 month storage at 4 °C and 0.6 log cycle after a half-month storage at 15 °C by adding 5000 BU per mL. Besides that, the count of L. monocytogenes was proved to be reduced by 2 log cycle after 1 month storage at 10 °C and 0.8 log cycle after half month storage at 15 °C by adding 5000 BU per mL.69 Pediocin PA-1 was also used in salad dressings to inhibit the growth of Lactobacillus bifermentans.70 Besides that, it was found to exert an antimicrobial effect against Leuconostoc mesenteroides, which is beneficial to the preservation of fresh beef, vacuum-packed beef and cottage cheese with MIC of 4 mg mL−1.71 Furthermore, pediocin PA-1 has been proved that it can help in preserving the fish fillets by inhibiting the growth of L. monocytogenes.72 For sous vide products, Bacillus subtilis and B. licheniformis can be inhibited by pediocin PA-1 to extend its shelf life.46 In the production of fermented soymilk, pediocin PA-1 from E. faecium NCIM 5423 or Lactobacillus plantarum Acr was claimed to exert the antimicrobial effect against L. monocytogenes to prolong the storage period.73 Besides that, pediocin 34 extracted from Pediococcus pentosaceous 34 was proved to have an inhibitory effect on L. monocytogenes in milk products and meat.15
2.4 Leucocin
Leucocin is a class IIa bacteriocin that is produced by Leuconostoc spp. Leucocin A produced by Leuconostoc gelidum UAL187 was demonstrated to exhibit an antimicrobial effect on the growth of L. monocytogenes in the preservation of milk products, fresh meat and sausage.74 Leucocin A is a plasmid-mediated bacteriocin with a molecular size of 3.93 kDa that is made up of 37 amino acid residues.75 It was found to exhibit inhibition activity against L. monocytogenes FSL C1-056, FSL J1-177, FSL N3-013, FSL R2-499 and FSL N1-227 with MIC of >2200.0 μM.76 Besides that, it was proved to exert an inhibitory effect against C. divergens UAL9, a pathogen that may cause the spoilage of meats. C. divergens UAL9 was proved to be inhibited by leucocin A with MIC of 1.7 μM.77 In the preservation of milk, leucocin K7 from Leuconostoc mensenteroides K7 was claimed to has inhibition activity against L. monocytogenes with MIC of 28 μg mL−1.42 Furthermore, L. innocua CIP 80.11, which had been found in Spanish style dry fermented sausages, was proved to be inhibited by leucocin B-KM432Bz produced by Leuconostoc pseudomesenteroides KM432Bz with MIC of 64 nM.78 Moreover, it was claimed to exhibit an inhibitory effect on the foodborne pathogen L. monocytogenes CIP 82.110 in meat and meat products. The MIC of leucocin B-KM432Bz needed in the inhibition of L. monocytogenes CIP 82.110 was 16 nM.79
2.5 Other bacteriocins
There are still many other bacteriocins that have been demonstrated by researchers to be used as antimicrobial agents in food preservation, which includes leucocin A,75 leucocin B,79 sukacin,80 carnocyclin A,81 carnobacteriocin BM,81 piscicolin 126,81 lactococcin BZ,82 aureocin A70,83 natamycin,84 mycocin,85 bacteriocin CAMT2,86 bacteriocin 7293A31 and bacteriocin 7293B.31 The combined use of carnocyclin A, carnobacteriocin BM1 and piscicolin 126 produced by Carnobacterium maltaromaticum UAL307 was proved to exert antimicrobial effects against food pathogens, such as E. coli DH5α, Pseudomonas aeruginosa ATCC 14207 and Salmonella typhimurium ATCC 23564 in milk products and meat.81 Besides that, for the preservation of meat products, sukacin produced by L. sakei is also used to inhibit the growth of L. monocytogenes.80 In the production of skimmed and full-fat milk, lactococcin BZ was demonstrated to exert an antimicrobial effect against L. monocytogenes to prevent the spoilage of milk.82 Besides that, aureocin A70 produced by S. aureus was proved to have an inhibitory ability against L. monocytogenes in skimmed milk.83 The contamination of yeasts and molds in cheese, fresh dairy products, processed meat and beverages are always of concerns in food industries as it causes food spoilage, which influences the revenue of the company. To solve this problem, natamycin extracted from Streptomyces natalensis or Streptomyces gilvosporeus is claimed to have antimicrobial effects against yeasts and molds, which can prolong the storage period of cheese, fresh dairy products, processed meat and beverages.84 In addition, mycocin is a bacteriocin produced by Debaryomyces hansenii DSMZ70238 that can be used in the preservation of meat and meat products by inhibiting the growth of L. monocytogenes.85 Furthermore, bacteriocins 7293A and 7293B, which are produced by lactic acid bacteria, Weissella hellenica BCC 7239 was proved to be an effective antimicrobial agent for the inhibition of P. aeruginosa, Aeromonas hydrophila, S. typhimurium and E. coli in meat and meat products.31 In the production of meat, milk products and meat products, bacteriocin CAMT2 from Bacillus amyloliquefaciens ZJHD3-06 can be used to inhibit the growth of L. monocytogenes, S. aureus, E. coli and Vibrio parahaemolyticus.86
3. Application of bacteriocin in medicine
The antimicrobial effects of penicillin against a wide range of pathogens were considered a big contribution in the medical field to cure many infections caused by bacteria or viruses. During the 1950s and 1960s, there were a lot of new antibiotics that had been discovered and used in the treatment of infectious diseases.87 However, the findings of new antibiotics began decreasing after 1985. Meanwhile, the discoveries of bacteria that were resistant to antibiotics increased significantly, and this threatened human beings. Pathogens had been proved to develop mechanisms for resisting drugs, in which the drug binding sites were altered and the access of the antimicrobial agents to its intracellular site had been reduced or inhibited.88 One of the examples of drug-resistant bacteria is MRSA, which is the general pathogen that may cause skin infection. MRSA was first discovered in hospitals during the 1960s after methicillin was used wisely in the treatment of skin infections. This problem alerted humans to find out alternative antimicrobial agents that can be used in killing or inhibiting pathogens. Bacteriocin, with its proteinaceous nature, was recommended by many researchers to replace antibiotics for the treatment of infectious disease due to its low toxicity.89–91 Many research studies have been done to investigate bacteriocin, which can be used to solve human health's problems, such as urinary tract infection, skin infection, diarrhoea, dental carries, lung infection, bloodstream infection, mastitis, respiratory tract infection and cancer.92 Table 2 shows the bacteriocins applied in the infectious disease treatment for humans, comprising nisin, lacticin, salivaricin, subtilosin, mersacidin, enterocin, gallidermin, epidermin and fermencin.
Table 2 Application of bacteriocin from LAB in infectious disease treatment for humans
Producing strain |
Types of bacteriocin |
Application in medicine |
Targeted pathogens or cells |
Pros and cons |
References |
L. lactis spp. |
Nisin |
Skin infections |
MRSA |
Pros: the wound dressing with nisin can stimulate wound closure and accelerate wound healing of excisional wounds with no adverse effects |
93 |
Lung infections |
P. aeruginosa |
Pros: nisin can be used to enhance the efficacy of polymyxins in the lung infection treatment by reducing the concentration of polymyxins needed. This may reduce polymyxin toxicity through the administration of significantly lower levels of polymyxin antibiotics |
101 and 195 |
Stomach ulcers |
H. pylori |
Pros: nisin is a potential antibacterial agent for the treatment of stomach ulcers due to its high resistance to a wide range of pH environments |
101 |
Nisin Z |
Mucosal and bloodstream infections |
C. albicans |
Pros: nisin Z inhibits the transformation of C. albicans from the blastospore to hyphal form, leading to ultrastructural disturbances |
13 |
Nisin A |
Cancer |
Reduce HNSCC tumorigenesis by inducing preferential apoptosis |
Pros: it can be alternative therapeutic for HNSCC instead of undergoing surgery and chemo- and radiation therapy, which are detrimental to normal cells and tissues and cause further morbidity. It inhibits tumorigenesis in vivo and prolongs survival in vivo |
196 |
Cons: no preliminary study with an animal model and humans is carried out |
Nisin A (combined with doxorubicin) |
Cancer |
Reduce tumour severity in skin carcinogenesis |
Pros: the combination of nisin A with doxorubicin shows significant increase in treatment effect of cancer as compared to docorubicin alone. This may decrease the doses of doxorubicin and its side effects |
197 |
Cons: no preliminary study with an animal model and humans is carried out |
Nisin F |
Respiratory tract infection |
S. aureus |
Pros: nisin with concentration at 8192 AU was proved to be safe as no abnormal of trachea, lungs, bronchi and haematology of rats detected |
198 |
Cons: no preliminary study with an animal model and humans is carried out |
Nisin A and Z |
Diarrhoea and inflammation of colon |
C. difficile |
Pros: both nisin A and Z can inhibit the growth of C. difficile and C. difficile spores were also susceptible to nisin A |
199 |
Cons: there is no in vivo test for the activity of nisin A and Z on C. difficile |
L. lactis ssp. lactis DPC3147 |
Lacticin 3147 |
Skin and surgical site and prosthetic joint infections |
MRSA and C. acnes |
Pros: lacticin 3147 improve the antimicrobial efficacy of penicillin G or vancomycin against and reduce the dose of antibiotics needed |
14 and 104 |
Dental carries |
S. mutans |
Pros: a food grade lacticin 3147 spray dried powder can reduce S. mutans in human saliva up to a 4-log reduction in counts after 20 min |
106 |
L. lactis subsp. lactis A164 and L. lactis subsp. lactis BH5 |
Lacticin A164 and BH5 |
Stomach ulcers |
H. pylori |
Pros: lacticin A164 and BH5 are potential antibacterial agents for the treatment of stomach ulcers due to its high resistance to a wide range of pH environment |
107 |
S. salivarius K12 |
Salivaricin A2 |
Pneumonia, sinus infection, ear infection, bacteremia and meningtis |
S. pneumoniae |
Pros: salivaricin A2 has high resistance to heat and a wide range pH environment |
110 |
Cons: no preliminary study with an animal model and humans is carried out |
Salivaricin B |
Scarlet fever, rheumatic fever, pharyngitis, tonsillitis, cellulitis, erysipelas and necrotizing fasciitis |
S. pyogenes |
Pros: salivaricin B has high resistance to heat and a wide range pH environment |
112 |
Cons: no preliminary study with an animal model and humans is carried out |
Pharyngitis, endocarditis, gastrointestinal tract infection and skin infection |
Corynebacterium spp |
Pros: salivaricin B has high resistance to heat and a wide range pH environment |
112 |
Cons: no preliminary study with an animal model and humans is carried out |
S. salivarius 5M6c |
Salivaricin D |
Empyema and pneumonia |
C. bifermentans |
Pros: salivaricin D is heat stable and the MIC needed to inhibit C. bifermentans is very low, which is 0.01 nM |
109 |
Cons: no preliminary study with an animal model and humans is carried out |
Infections in immunocompromised humans |
Ln. lactis |
Pros: salivaricin D is heat stable and the MIC needed to inhibit Ln. lactis is low, which is 0.1 nM |
109 |
Cons: no preliminary study with an animal model and humans is carried out |
Pneumonia, sinus infection, ear infection, bacteremia and meningtis |
S. pneumoniae |
Pros: salivaricin D is heat stable and the MIC needed to inhibit S. pneumoniae is very low, which is around 0.03–0.06 nM |
109 |
Cons: no preliminary study with an animal model and humans is carried out |
Scarlet fever, rheumatic fever, pharyngitis, tonsillitis, cellulitis, erysipelas and necrotizing fasciitis |
S. pyogenes |
Pros: salivaricin D is heat stable |
109 |
Cons: no preliminary study with an animal model and humans is carried out |
B. subtilis 168 |
Subtilosin A |
Urinary tract infection |
E. faecalis |
Pros: subtilosin A exert inhibitory effect against E. faecalis with MIC of 3.125 mg L−1 |
109 |
Cons: no preliminary study with an animal model and humans is carried out. Besides that, no information about characterization of the bacteriocin is available |
Periodontitis and tooth loss |
P. gingivalis |
Pros: subtilosin A exert inhibitory effect against P. gingivalis with MIC of 3.125–6.25 mg L−1 |
109 |
Cons: no preliminary study with an animal model and humans is carried out. Besides that, no information about characterization of the bacteriocin is available |
Pneumonia, urinary tract infection, skin infections and meningitis |
K. pneumoniae |
Pros: subtilosin A exert inhibitory effect against K. pneumoniae with MIC of 1.25–25 mg L−1 |
120 |
Cons: no preliminary study with an animal model and humans is carried out. Besides that, no information about characterization of the bacteriocin is available |
Methylmalonic aciduria in immunocompromised patients |
K. rhizophila |
Pros: subtilosin A exert inhibitory effect against K. rhizophila with MIC of 1.25 mg L−1 |
121 |
Cons: no preliminary study with an animal model and humans is carried out. Besides that, no information about characterization of the bacteriocin is available |
Gastrointestinal infection, meningitis, urinary tract infection, skin infection and respiratory infection |
E. aerogenes or K. aerogenes |
Pros: subtilosin A exert inhibitory effect against K. aerogenes with MIC of 1.25 mg L−1 |
109 |
Cons: no preliminary study with an animal model and humans is carried out. Besides that, no information about characterization of the bacteriocin is available |
Scarlet fever, rheumatic fever, pharyngitis, tonsillitis, cellulitis, erysipelas and necrotizing fasciitis |
S. pyogenes |
Pros: subtilosin A exert inhibitory effect against S. pyogenes with MIC of 1.25 mg L−1 |
109 |
Cons: no preliminary study with an animal model and humans is carried out. Besides that, no information about characterization of the bacteriocin is available |
Shigellosis |
S. sonnei |
Pros: subtilosin A exert inhibitory effect against S. sonnei with MIC of 1.25 mg L−1 |
123 |
Cons: no preliminary study with an animal model and humans is carried out. Besides that, no information about characterization of the bacteriocin is available |
Pneumonia, urinary tract infections and bacteremia |
P. aeruginosa |
Pros: subtilosin A exerts inhibitory effect against P. aeruginosa with MIC of 50 mg L−1 |
124 |
Cons: no preliminary study with an animal model and humans is carried out. Besides that, no information about characterization of the bacteriocin is available |
Dental plaque formation |
S. gordonii |
Pros: subtilosin A exerts inhibitory effect against S. gordonii with MIC of 83.25 mg L−1 |
109 |
Cons: no preliminary study with an animal model and humans is carried out. Besides that, no information about characterization of the bacteriocin is available |
Bacillus sp. strain HIL Y-85, 54728 |
Mersacidin |
Abscesses, furuncle, bloodstream infection and pneumonia |
MRSA |
Pros: mersacidin is heat stable. Elevated interleukin-1β and tumour necrosis factor-α titres were not found in mice treated with mersacidin, but found in untreated mice. Besides that, differences in the cytokine profiles were not induced by mersacidin |
200 |
Cons: no study on site of action (epithelium versus blood) of mersacidin and its application on humans is carried out |
Pneumonia, sinus infection, ear infection, bacteremia and meningtis |
S. pneumoniae |
Pros: mersacidin is heat stable. It can be used to enhance the inhibition effect of antibiotics such as penicillin and ampicillin on S. pneumoniae and reduce the dosage of antibiotics |
128 |
Cons: no study on site of action (epithelium versus blood) of mersacidin and its application on humans is carried out |
Pneumonia and meningitis |
M. luteus |
Pros: mersacidin is heat stable and produces antibacterial activity earlier than nisin and subtilin. It can be used to enhance the inhibition effect of antibiotics such as penicillin and ampicillin on M. luteus and reduce the dosage of antibiotics |
125 and 129 |
Cons: no study on site of action (epithelium versus blood) of mersacidin and its application on humans is carried out |
Infections of abdomen, skin, bloodstream and urinary tract |
E. faecium and E. faecalis |
Pros: mersacidin is heat stable. It can be used to enhance the inhibition effect of antibiotics such as ampicillin on E. faecium and E. faecalis and reduce the dosage of antibiotics |
130 |
Cons: no study on site of action (epithelium versus blood) of mersacidin and its application on humans is carried out |
L. lactis MG1614 |
Enterocin A |
Listeriosis |
L. monocytogenes |
Pros: enterocin A is thermostable, proteinaceous, resistant to catalase and does not produce hemolysin |
132 and 133 |
Cons: the inhibition effect of enterocin A on L. monocytogenes is not obvious. It can be enhanced by the combination use of thyme essential oils and enterocin A |
Infection of bladder |
E. coli |
Pros: enterocin A is thermostable, proteinaceous, resistant to catalase and does not produce hemolysin |
132 and 133 |
Cons: the inhibition effect of enterocin A on E. coli is not obvious. It can be enhanced by the combination use of thyme essential oils and enterocin A |
E. hirae LD3 |
Enterocin LD3 |
Pneumonia and meningitis |
M. luteus |
Pros: enterocin LD3 is thermostable up to 121 °C (at 15 psi pressure) and high resistance to acidic environment with range pH 2–6 |
56 and 134 |
Cons: no preliminary study with an animal model and humans is carried out. Besides that, no information about characterization of the bacteriocin is available |
E. faecalis |
Enterocin AS-48 |
Diarrhoea and infections of respiration tract and wounds |
B. cereus |
Pros: the viable cell count of B. cereus decreases rapidly with adding of 20–35 μg mL−1 enterocin. Enterocin AS-48 also increase the heat sensitivity of endospores in which the inactivation of endospores can be achieved at 90 °C and 95 °C for 1 min |
49 |
E. hirae 20C |
Enterocin E20C |
Gastroenteritis, bacteremia and enteric fever |
S. enterica |
Pros: enterocin E20C is not only can be used alone in the treatment of gastroenteritis, bacteremia and enteric fever, but also has synergistic interaction with antibiotics such as ampicillin, penicillin, ceftriaxone, and ciprofloxacin against a ciprofloxacin- and penicillin-resistant strain of S. enterica. It was proved to reduce the MIC needed by ampicillin, penicillin, ceftriaxone, and ciprofloxacin to inhibit S. enterica, which were decreased 6.9, 13.1, 6.4 and 12.8 folds, respectively |
55 |
S. epidermidis |
Epidermin |
Infections of respiratory tract, skin and surgical site |
S. aureus |
Pros: epidermin has high resistance to heat and a wide range pH environment |
137 |
Cons: no preliminary study of the inhibition effect of epidermin on S. aureus with an animal model and humans is carried out |
Pneumonia, sinus infection, ear infection, bacteremia and meningtis |
S. pneumoniae |
Pros: epidermin has high resistance to heat and a wide range pH environment |
137 |
Cons: no preliminary study of the inhibition effect of epidermin on S. pneumoniae with an animal model and humans is carried out |
Scarlet fever, rheumatic fever, pharyngitis, tonsillitis, cellulitis, erysipelas and necrotizing fasciitis |
S. pyogenes |
Pros: epidermin has high resistance to heat and a wide range pH environment. Besides that, it can reduce the dose of penicillin |
138 |
Cons: no preliminary study of the inhibition effect of epidermin on S. pyogenes with an animal model and humans is carried out |
Infections of urinary tract, wound and soft tissue |
S. faecalis |
Pros: epidermin has high resistance to heat and a wide range pH environment |
139 |
Cons: no preliminary study of the inhibition effect of epidermin on S. faecalis with an animal model and humans is carried out |
Endocarditis, cerebrospinal fluid shunt infection in an infant, mediastinitis and spontaneous bacterial peritonitis |
C. xerosis |
Pros: epidermin has high resistance to heat and a wide range pH environment |
137 |
Cons: no preliminary study of the inhibition effect of epidermin on C. xerosis with an animal model and humans is carried out |
Pneumonia and meningitis |
M. luteus |
Pros: epidermin has high resistance to heat and a wide range pH environment |
140 |
Cons: no preliminary study of the inhibition effect of epidermin on M. luteus with an animal model and humans is carried out |
Infection of brain, liver, breast, and lung abscesses |
P. anaerobicus |
Pros: epidermin has high resistance to heat and a wide range of pH environment |
138 |
Cons: no preliminary study of the inhibition effect of epidermin on P. anaerobicus with an animal model and humans is carried out |
Acnes |
P. acnes |
Pros: epidermin has high resistance to heat and a wide range of pH environment |
138 |
Cons: no preliminary study of the inhibition effect of epidermin on P. acnes with an animal model and humans is carried out |
S. gallinarum |
Gallidermin |
Infections of respiratory tract, skin and surgical site |
S. aureus |
Pros: gallidermin inhibits not only the growth of S. aureus in a dose-dependent manner but also efficiently prevents biofilm formation. Besides that, it displayed no cytotoxic effects on fibroblasts and hemolyzed less than 1% of human RBCs, and did not induce reactive oxygen species production or cell aggregation in whole blood |
89 and 137 |
Prosthetic valve endocarditis (PVE) infections and intracardiac abscesses |
S. epidermidis |
Pros: gallidermin inhibits not only the growth of S. epidermidis in a dose-dependent manner but also efficiently prevents biofilm formation. Besides that, it displayed no cytotoxic effects on fibroblasts and hemolyzed less than 1% of human RBCs, and did not induce reactive oxygen species production or cell aggregation in whole blood |
89 and 137 |
Osteoarticular infections |
S. simulans |
Pros: gallidermin can be used to enhance the inhibition effect of antibiotics on S. simulans and reduce the dosage of antibiotics. Besides that, it displayed no cytotoxic effects on fibroblasts and hemolyzed less than 1% of human RBCs, and did not induce reactive oxygen species production or cell aggregation in whole blood |
89 and 141 |
Pneumonia, sinus infection, ear infection, bacteremia and meningtis |
S. pneumoniae |
Pros: gallidermin can be used to enhance the inhibition effect of antibiotics on S. pneumoniae and reduce the dosage of antibiotics. Besides that, it displayed no cytotoxic effects on fibroblasts and hemolyzed less than 1% of human RBCs, and did not induce reactive oxygen species production or cell aggregation in whole blood |
89 and 137 |
Scarlet fever, rheumatic fever, pharyngitis, tonsillitis, cellulitis, erysipelas and necrotizing fasciitis |
S. pyogenes |
Pros: gallidermin can be used to enhance the inhibition effect of antibiotics on S. pyogenes and reduce the dosage of antibiotics. Besides that, it displayed no cytotoxic effects on fibroblasts and hemolyzed less than 1% of human RBCs, and did not induce reactive oxygen species production or cell aggregation in whole blood |
89 and 137 |
Infections of urinary tract, wound and soft tissue |
S. faecalis |
Pros: gallidermin can be used to enhance the inhibition effect of antibiotics on S. faecalis and reduce the dosage of antibiotics. Besides that, it displayed no cytotoxic effects on fibroblasts and hemolyzed less than 1% of human RBCs, and did not induce reactive oxygen species production or cell aggregation in whole blood |
89 and 137 |
Endocarditis, cerebrospinal fluid shunt infection in an infant, mediastinitis and spontaneous bacterial peritonitis |
C. xerosis |
Pros: gallidermin can be used to enhance the inhibition effect of antibiotics on C. xerosis and reduce the dosage of antibiotics. Besides that, it displayed no cytotoxic effects on fibroblasts and hemolyzed less than 1% of human RBCs, and did not induce reactive oxygen species production or cell aggregation in whole blood |
89 and 137 |
Pneumonia and meningitis |
M. luteus |
Pros: gallidermin can be used to enhance the inhibition effect of antibiotics on M. luteus and reduce the dosage of antibiotics. Besides that, it displayed no cytotoxic effects on fibroblasts and hemolyzed less than 1% of human RBCs, and did not induce reactive oxygen species production or cell aggregation in whole blood |
89 and 137 |
Infection of brain, liver, breast, and lung abscesses |
P. anaerobicus |
Pros: gallidermin can be used to enhance the inhibition effect of antibiotics on P. anaerobicus and reduce the dosage of antibiotics. Besides that, it displayed no cytotoxic effects on fibroblasts and hemolyzed less than 1% of human RBCs, and did not induce reactive oxygen species production or cell aggregation in whole blood |
89 and 137 |
Acnes |
P. acnes |
Pros: gallidermin can be used to enhance the inhibition effect of antibiotics on P. acnes and reduce the dosage of antibiotics. Besides that, it displayed no cytotoxic effects on fibroblasts and hemolyzed less than 1% of human RBCs, and did not induce reactive oxygen species production or cell aggregation in whole blood |
89 and 137 |
L. fermentum GA715 |
Fermencin SA715 |
Pneumonia, urinary tract infections and bacteremia |
P. aeruginosa |
Pros: fermencin SA715 possesses high thermal and pH stability |
28 |
Cons: no preliminary study of the inhibition effect of fermencin SA715 on P. aeruginosa with an animal model and humans is carried out |
Pneumonia and meningitis |
M. luteus |
Pros: fermencin SA715 possesses high thermal and pH stability |
28 |
Cons: no preliminary study of the inhibition effect of fermencin SA715 on M. luteus with an animal model and humans is carried out |
Infection of bladder |
E. coli |
Pros: fermencin SA715 possesses high thermal and pH stability |
28 |
Cons: no preliminary study of the inhibition effect of fermencin SA715 on E. coli with an animal model and humans is carried out |
Infections of respiratory tract, skin and surgical site |
S. aureus |
Pros: fermencin SA715 possesses high thermal and pH stability |
28 |
Cons: no preliminary study of the inhibition effect of fermencin SA715 on S. aureus with an animal model and humans is carried out |
Pharyngitis, endocarditis, gastrointestinal tract infection and skin infection |
Corynebacterium spp |
Pros: fermencin SA715 possesses high thermal and pH stability |
28 |
Cons: no preliminary study of the inhibition effect of fermencin SA715 on Corynebacterium spp with an animal model and humans is carried out |
L. fermentum SD11 |
Fermencin SD11 |
Dental carries and gingivitis |
S. mutans, S. sobrinus, A. actinomycetemcomitans, F. nucleatum, P. gingivalis and C. albicans |
Pros: fermencin SD11 is stable at acidic environment and may be used an alternative approach for promoting oral health or prevention of oral diseases |
144 and 146 |
Cons: no preliminary study of the inhibition effect of fermencin SD11 with an animal model and humans is carried out |
3.1 Nisin
Nisin, a bacteriocin produced by Lactococcus lactis spp., is found to be used not only in the food preservation, but also can be used in the treatment of infectious disease caused by bacteria.34 Skin infection is usually caused by S. aureus, and MRSA has emerged from the usage of methicillin in the treatment of the infection.43 Recently, there are many research studies that have been done on the investigation of bacteriocin to replace methicillin in the treatment of skin infection, as the failure to treat the infection caused by MRSA may lead to death. Electrospun nanofibre wound dressing that comprises nisin has been proved that can be used in wound healing to prevent skin infection by MRSA. In the treatment of skin infection, wound dressing (that contains nisin) was found to be effective in the reduction of the colonization of S. aureus, and it accelerated the wound healing process.93 Besides that, nisin was demonstrated to have an antimicrobial effect against Clostridium difficile. C. difficile is a bacterium that may cause diarrhea and inflammation of the colon.94 Previous studies proved that older people in hospital, or for those who are taking antibiotics for long term treatment, may be infected by C. difficile as compared to others. Around a half million of citizens from United States was reported to be infected by C. difficile every year and the infection cases was getting severe and more difficult to treated.95 Nisin A and nisin Z were claimed to be effective bacteriocin that can be utilized in the inhibition of the growth of C. difficile. The minimum inhibitory concentration (MIC) of nisin A and nisin Z were 0.8 μg mL−1 and 6.2 μg mL−1, respectively.96 Furthermore, dental caries are a common health problem that affects all ages of humans. Nisin was also proved to have inhibitory abilities against pathogens that cause dental caries, which were Streptococcus mutans, Streptococcus sanguinis and Streptococcus sobrinus. The MIC of nisin needed to inhibit the growth of S. mutans, S. sanguinis and S. sobrinus are 625–1250 IU per mL, 156.25–312.5 IU per mL and 1250–2500 IU per mL, respectively.97
In addition, nisin Z has been demonstrated to be used for the treatment of mucosal and bloodstream infections caused by C. albicans.13 Bloodstream infection, which is also called candidemia, can lead to infection of other parts of the body, such as the eyes, kidney, liver, and brain by the spreading of C. albicans from your bloodstream. Candidemia may cause illness, such as fever, skin rash, low blood pressure and abdominal pain, as well as death if without treatment.98 Previous studies proved that nisin Z was able to inhibit the growth of C. albicans at 500 μg mL−1 by disturbing the cell membrane structure of C. albicans and increasing the granulation of the cytoplasm.99 Besides that, nisin F was proved to have inhibition activity against S. aureus, which may cause infection of the respiratory tract. 8192 AU per mL of nisin F was proved to has significant antimicrobial effect against S. aureus.100 Furthermore, nisin was demonstrated to be used in the treatment of lung infections and stomach ulcers to inhibit the growth of P. aeruginosa and Helicobacter pylori, respectively.101 In addition, nisin A was proved to be an alternative agent used in the treatment of cancer. Nisin A can be used to reduce tumorigenesis by inducing preferential apoptosis, and the combination of nisin with doxorubicin was claimed to be an effective agent used in the reduction of tumour severity in skin carcinogenesis.102
3.2 Lacticin
Lacticin is a broad-spectrum bacteriocin produced by L. lactis subsp. that belongs to the lantibiotics groups.103 Lacticin 3147 produced by L. lactis ssp. lactis DPC3147 has been demonstrated to exert an antimicrobial effect against a range of pathogenic bacteria. Lacticin 3147 was proved to be an effective antimicrobial agent that can be used in the treatment of skin and surgical site or prosthetic joint infection.104 The most common bacterium that causes skin infections is S. aureus. Lacticin 3147 was proved to have inhibitory ability on the growth of MRSA ST291. Lacticin 3147 showed lower MIC against MRSA ST291 as compared to nisin Z and penicillin G, which is 3.85 μg mL−1.14 Besides that, lacticin 3147 was claimed to have inhibition activity against the growth of Cutibacterium acnes.104 C. acnes is grouped as a lipophilic anaerobic, Gram-positive bacterium that can be commonly found on normal skin, oral environment, nose, urogenital tract and large intestine. C. acnes may cause infections during orthopedic surgery, especially those that are related to implants and prosthetic joint infections.105 The MIC needed for the inhibition of the growth of C. acnes LMG 16711 was 2.50 μg mL−1.14
Furthermore, lacticin 3147 can be used in the prevention of dental caries. It was shown to exert inhibitory effect against S. mutans, which is the common pathogen that causes oral plaque formation in humans. A previous study reported that the concentration of lacticin 3147 at 1280–5120 AU per mL was needed to inhibit 50% growth of S. mutans. The study claimed that the S. mutans strain in human saliva could be eliminated if 40
000 AU per mL was used.106 In addition, lacticins A164 and BH5 produced by L. lactis subsp. lactis A164 and L. lactis subsp. lactis BH5, respectively, were demonstrated as the most strong bacteriocins among lacticins that could be used in the treatment of stomach ulcers caused by H. pylori.107 H. pylori is a pathogen that can be found in the gastric mucosa. It is the main root cause of gastritis and peptic ulcer. The antimicrobial activities of lacticin A164 and lacticin BH5 against H. pylori ATCC 43504 were 1
310
000 AU per mL and 655
000 AU per mL, respectively. MIC of lacticin A164 and lacticin BH5 needed to inhibit the growth of H. pylori ATCC 43504 was 12.5 mg l−1.108
3.3 Salivaricin
Salivaricin is a bacteriocin that is produced by Streptococcus salivarius and belongs to class II lantibiotics.109 Many research studies proved that salivaricin has contributed to the health sustainability in humans. Salivaricin was shown that it not only can be used to maintain oral health, but also can be used in the treatment or prevention of skin or lung infections. Streptococcus pneumoniae is a pathogen that causes pneumonia, sinus infection, ear infection, bacteremia and meningtis. Salivaricin A2 produced by S. salivarius K12 was demonstrated to exert antimicrobial effects against S. pneumoniae ATCC 27336, AI8, AI11, AI14, AI6 and AI7. The MIC of salivaricin A2 to be required in the inhibition of the growth of S. pneumoniae ATCC 27336, AI8, AI11, AI14, AI6 and AI7 were 32, 32, 16, 32, 128 and 128 μg mL−1, respectively.110 Besides that, Streptococcus pyogenes is a pathogens that causes many infectious diseases in humans, such as scarlet fever, rheumatic fever, pharyngitis, tonsillitis, cellulitis, erysipelas and necrotizing fasciitis.111 Salivaricin B from S. salivarius K12 was found to exert inhibition activities against S. pyogenes with MIC of 2.16 μM. The growth of S. pyogenes was proved to be inhibited by 50% by using 1.0 μM of salivaricin B. The inhibition activity can be increased to 90% by using 2.0 μM of salivaricin B, and the cell will be totally inhibited if treated with 2.5 μM.112
Furthermore, salivaricin B was also shown to have inhibition activity against Corynebacterium spp GH17.112 Corynebacterium spp was found in triggering diseases, such as pharyngitis, endocarditis, gastrointestinal tract infection and skin infection.113 The MIC for the inhibition of growth of Corynebacterium spp GH17 by salivaricin B was 2690 nM.112 Besides that, salivaricin D from S. salivarius 5M6c was proved to exert inhibitory ability against Clostridium bifermentans.109 C. bifermentans is a Gram-positive, spore-forming, anaerobic bacterium that always causes infectious diseases, such as empyema and pneumonia to humans.114 The MIC of salivaricin D for the inhibition of the growth of C. bifermentans was found at around 0.01 nM.109 Leuconostoc lactis is a Gram-positive, catalase-negative and facultatively anaerobic, which may cause infections in immunocompromised hosts. Up to now, there are still limited treatments and antibiotics that are available for the infections caused by Ln. lactis.115 To solve this problem, salivaricin D was claimed to have an antimicrobial effect on Ln. lactis. The MIC of salivaricin D to inhibit the growth of Ln. lactis was proved at around 0.1 nM.109 Salivaricin D was also demonstrated as an antimicrobial agent in the inhibition of the growth of S. pneumoniae D39, TIGR4 and R6 for the prevention of pneumonia. The MIC of salivaricin D to inhibit the growth of S. pneumoniae D39, TIGR4 and R6 were proved at 0.03, 0.06 and 12, respectively. Besides that, salivaricin D also exerted inhibitory effects against S. pyogenes 08198 with MIC value of 7.2 nM.109
3.4 Subtilosin
Subtilosin is a bacteriocin that can be produced by Bacillus strains. Bacillus spp is an aerobic, Gram-positive bacteria with rod shape, which can be usually found in soil, water or natural flora in the intestines.116 Subtilosin A is a bacteriocin with a molecular weight of 3398.9, which consists of 32 usual amino acids and some non-amino acid residues. Subtilosin A, produced by B. subtilis 168, was claimed to exert antimicrobial effects against many Gram-positive and Gram-negative pathogens.117 Subtilosin A was proved to have an inhibitory effect against E. faecalis. E. faecalis is a Gram-positive bacterium that may cause infections in humans, especially infection of the urinary tract. The MIC at 3.125 mg L−1 of subtilosin A was demonstrated to have significant inhibition activity against E. faecalis OGX-1. Besides that, subtilosin A was proved that it could be used to inhibit the growth of Porphyromonas gingivalis.118 P. gingivalis is a Gram-negative, anaerobic, non-motile bacterium with rod shape, which binds to the human oral cavity and always causes periodontitis and tooth loss.119 The MIC values of subtilosin A needed for the inhibition of the growth of P. gingivalis ATCC 33277, P. gingivalis ATCC 33277 KDP129, P. gingivalis ATCC 33277 KDP133 and P. gingivalis W83 were 3.125, 3.125, 6.25 and >100 mg L−1, respectively.118
Furthermore, subtilosin A was proved to exert an inhibitory effect against Klebsiella pneumoniae. K. pneumoniae is a Gram-negative, non-motile and rod-shaped facultative anaerobe that may cause pneumonia, urinary tract infection, skin infections and meningitis. The MIC of subtilosin A for the inhibition of the growth of K. pneumoniae ATCC 4352, K. pneumoniae UMN1, K. pneumoniae UMN5, K. pneumoniae UMN3 and K. pneumoniae UMN4 were >200, >200, 1.25, 5.0 and 25.0 mg L−1, respectively.120 Subtilosin A was also found to have an inhibitory effect against K. rhizophila. K. rhizophila is a Gram-positive bacterium that can be normally found on the normal skin and mucous membrane of humans, but it may cause infections, such as methylmalonic aciduria in immunocompromised patients. 1.25 mg L−1 of subtilosin A was proved to be the MIC needed to inhibit K. rhizophila ATCC 9341.121 In addition, the growth of Enterobacter aerogenes was demonstrated to be inhibited by subtilosin A. E. aerogenes, known as Klebsiella aerogenes now, is a Gram-negative bacterium with rod shape, which may cause gastrointestinal infection, meningitis, urinary tract infection, skin infection and respiratory infection. The growth of E. aerogenes ATCC 13408 was proved to be inhibited by the MIC of subtilosin A at 1.25 mg L−1.118 Subtilosin A was also claimed to have inhibition activity against S. pyogenes, a pathogen that may cause infections in human. The MIC of subtilosin A to inhibit S. pyogenes ATCC 19615 was proved at 1.25 mg L−1.122 Besides that, subtilosin A was proved to be used as an antimicrobial agent in the treatment of shigellosis. Shigellosis is the infection that may be caused by Shigella sonnei, leading to diarrhea, fever and stomach cramps. 1.25 mg L−1 of subtilosin A was proved to be the MIC to inhibit the growth of S. sonnei ATCC 25931.123 Moreover, the inhibition of the growth of P. aeruginosa was achieved using subtilosin A. P. aeruginosa is a Gram-negative, encapsulated, rod-shaped bacterium that may cause infectious diseases, such as pneumonia, urinary tract infections and bacteremia in humans. The MIC of subtilosin A needed to inhibit the growth of P. aeruginosa ATCC 9027 was 50 mg L−1.124 In addition, subtilosin A was proven to exert inhibitory effect against S. gordonii Challis, which was the pathogen found in the oral cavity to cause dental plaques. A MIC of 83.25 of subtilosin A was claimed to exert significant inhibitory effect on S. gordonii Challis ATCC 49818.118
3.5 Mersacidin
Mersacidin is a bacteriocin that belongs to the lantibiotic group, which consists of 20 amino acids and a lanthionine group in its structure. It is produced by Bacillus spp and proved to have inhibition activities against Gram-positive pathogens.125 S. aureus is a Gram-positive bacterium with rod shape, which may cause infectious diseases in humans, such as abscesses, furuncle, bloodstream infection and pneumonia.126 Mersacidin produced by the Bacillus sp. strain HIL Y-85, 54728 was claimed to exert antimicrobial effects on a few S. aureus strains, which including S. aureus SH1000, R33 MRSA, SG511, SA137/93A and SA137/93G. The MIC of mersacidin needed to inhibit the growth of S. aureus SH1000, R33 MRSA, SG511, SA137/93A and SA137/93G was proved at 32, 32, 1, 35 and 30 μg mL−1, respectively.127 Besides that, mersacidin was shown to have inhibition activities against S. pneumoniae BAA-255, a pathogen that causes pneumonia in humans. The MIC of mersacidin needed to inhibit the growth of S. pneumoniae BAA-255 was 2 μg mL−1.128 Furthermore, mersacidin was demonstrated to have inhibition activity against Micrococcus luteus. M. luteus is a Gram-positive and non-motile bacterium reported as a opportunistic pathogen that may cause pneumonia and meningitis. The MIC of mersacidin needed to inhibit M. luteus ATCC4698 was 1.2 μg mL−1.129 In addition, mersacidin was proved to have an inhibitory effect on the E. faecium and E. faecalis strains, which might cause infections in the abdomen, skin, bloodstream and urinary tract. The MIC of mersacidin needed for the inhibition of the growth of E. faecium ATCC19579, E. faecalis ATCC29212 and E. faecium 7131121 VRE were proved at 32, 64, 64 μg mL−1, respectively.130
3.6 Enterocin
Enterocin is a bacteriocin produced by Enterococcus spp that is active against Gram-positive pathogens.90 Enterocin A was claimed to exert inhibitory effect against L. monocytogenes. L. monocytogenes is a facultative anaerobic that may cause listeriosis.131 The groups of people that can be easily infected by L. monocytogenes are newborns, older adults, pregnant women, and people with immunodeficiency disorders.132 The MIC of enterocin A needed to inhibit the growth of L. monocytogenes EGDe was around 4.57 μg mL−1. It was found that the inhibition activity of enterocin A against L. monocytogenes EGDe can be improved by the combination of both enterocin A and thyme essential oil, in which only 0.9 and 1.2 μg mL−1 of MIC of enterocin and thyme essential oil, respectively, were needed for the inhibition of the L. monocytogenes EGDe.133 Besides that, enterocin LD3 from Enterococcus hirae LD3 was proved to have antimicrobial effects against M. luteus MTCC 106 with MIC at 80 μg mL−1 and MBC at 128 μg mL−1.134 Enterocin A was also found to have inhibition activity against E. coli NCDC 135 with MIC at 112 μg mL−1 and MBC at 180 μg mL−1.134 In addition, enterocin AS-48 was proved to have inhibition against B. cereus ATCC 14579. B. cereus is a Gram-positive, motile and facultative anaerobe with rod shape, which can be usually found in the soil environment, and causes diarrhea and infections in the respiration tract and wounds. The MIC of enterocin AS-48 needed for the inhibition of the growth of B. cereus ATCC 14579 was 2.5 μg mL−1.49 Enterocin E20C produced by E. hirae 20C was also demonstrated to exert an antimicrobial effect against Salmonella enterica. S. enterica is a Gram-negative and facultative aerobe with rod shape that may cause gastroenteritis, bacteremia and enteric fever. The MIC of enterocin E20C needed for the inhibition of the growth of S. enterica was 0.5 μg mL−1.55
3.7 Epidermin
Epidermin is a tetracyclic bacteriocin that belongs to type A lantibiotic. It comprises 21 amino acids with meso-lanthionine, 3-methyllanthionine, and S-(2-aminovinyl)-D-cysteine in the structure. Epidermin produced by Staphylococcus epidermidis exhibits antimicrobial activities against a wide range of Gram-positive bacteria.135 Epidermin was claimed to exhibit inhibition activities against pathogens S. aureus SG 511 and E 88, which might cause respiratory tract, skin and surgical site infections. The MIC of epidermin needed to inhibit the growth of S. aureus SG 511 and E 88 were the same at 8 μg mL−1.136 Besides that, epidermin was also applied in the treatment of infection caused by S. epidermidis. S. epidermidis is a Gram-positive and facultative anaerobe that can be usually found in normal human flora. A MIC of 6.25 μg mL−1 epidermin was needed for the inhibition of S. epidermidis 12228.137 Furthermore, epidermin was also claimed to exhibit inhibitory effect against S. pneumoniae ATCC 6302, a pathogen that may cause pneumonia. A MIC of 4 μg mL−1 epidermin was required to inhibit the growth of S. pneumoniae ATCC 6302.137
Moreover, S. pyogenes ATCC 8668, a pathogen that might cause respiratory tract infections in humans, was found to be inhibited by epidermin with MIC of 1 μg mL−1.138 Epidermin was also proved to inhibit S. faecalis ATCC 29212, a pathogen that may cause infections in the urinary tract, wound and soft tissue. The MIC of epidermin needed to inhibit the growth of S. faecalis ATCC 29212 was proved at 64 μg mL−1.139 In addition, epidermin was proved to have inhibition activity against Corynebacteriurn xerosis. C. xerosis is a Gram-positive aerobe that can be found in the normal flora of the nasopharynx and skin, and may cause endocarditis, cerebrospinal fluid shunt infection in an infant, mediastinitis and spontaneous bacterial peritonitis. The MIC of epidermin needed to exert inhibition activity against C. xerosis was 1 μg mL−1.137 Besides that, M. luteus ATCC 9341 and 15957, pathogens that cause pneumonia and meningitis, were proved to be inhibited by epidermin with MIC of 0.25 and 1 μg mL−1, respectively.140 Peptostreptococcus anaerobicus is a Gram-positive and non-spore forming anaerobe that may lead to infection of the brain, liver, breast, and lung abscesses in humans. It was claimed to be inhibited by epidermin with MIC of 0.25 μg mL−1.138 Furthermore, epidermin exhibited inhibition activity against Propionibacterium acnes IF 31002. P. acnes is a Gram-positive anaerobe that may cause acne in humans. It was proved to be inhibited by epidermin with MIC of 0.25 μg mL−1.138
3.8 Gallidermin
Gallidermin is a bacteriocin that is produced by Staphylococcus gallinarum, which is made up of 22 amino acids in its structure. It is grouped as lantibiotic due to its lanthionine-containing peptide structure.138 It was demonstrated to have inhibition activity against a wide range of Gram-positive pathogens, such as S. aureus and S. epidermidis. S. aureus is the common pathogen that causes respiratory tract, skin and surgical site infections in humans. Gallidermin was claimed to exhibit inhibition activities against S. aureus SG 511, E 88, MSSA and MRSA with MIC at 4, 8, 5.77 and 0.72 μg mL−1, respectively.137 Besides that, gallidermin was proved to exhibit antimicrobial activity against S. epidermidis (12228, 117, RP62A and N15) with MIC of 6.25 μg mL−1.137 Gallidermin was also proved to exert an inhibitory effect on the growth of Staphylococcus simulans. S. simulans is a Gram-positive pathogen that may cause osteoarticular infections in humans. 0.15 μg mL−1 of gallidermin was needed to inhibit the growth of S. simulans 22.141
Furthermore, gallidermin was claimed to exhibit an inhibitory effect against S. pneumoniae ATCC 6302, a pathogen that may cause pneumonia. The MIC of gallidermin required to inhibit the growth of S. pneumoniae ATCC 6302 was 4 μg mL−1.137 Moreover, S. pyogenes ATCC 8668, a pathogen that might cause respiratory tract infections in humans, was found to be inhibited by gallidermin with MIC of 1 μg mL−1.137 Besides that, gallidermin was proved to inhibit S. faecalis ATCC 29212, a pathogen that may cause infections in the urinary tract, wound and soft tissue with MIC of 64 μg mL−1.89 In addition, gallidermin was proved to have inhibition activity against C. xerosis with MIC of 1 μg mL−1.142 Gallidermin was also proved to exhibit an inhibitory effect against M. luteus ATCC 9341 and 15957 with MIC of 0.25 and 0.5 μg mL−1, respectively.137 Furthermore, P. anaerobicus was claimed to be inhibited by gallidermin with MIC of 0.5 μg mL−1. P. acnes, a pathogen that causes acne in humans, was demonstrated by gallidermin with MIC of 0.125 μg mL−1.143
3.9 Fermencin
Fermencin is a novel bacteriocin with a broad-spectrum, non-pore forming and cell wall that can be produced and extracted from Lactobacillus fermentum. L. fermentum is a Gram-positive and facultative anaerobe with rod shape that belongs to the LAB group.28 Up to now, there is still less fermencin to be reported. From previous studies, there were two types of fermencins, which included fermencin SA715 and SD11, and they had been characterized and proved to have inhibition activities on pathogens. Fermencin SA715 produced from L. fermentum GA715 was proved to exhibit an inhibitory effect on the growth of P. aeruginosa PA7, a pathogen that might cause infectious diseases such as pneumonia, urinary tract infections and bacteremia in humans. The MIC of fermencin SA715 needed for the inhibition of the growth of P. aeruginosa PA7 was proved at around 51.79 μM.28 Besides that, fermencin SA715 was proved to exert an inhibitory effect on M. luteus ATCC 10240, a pathogen that might cause pneumonia and meningitis with a MIC value of 8.93 μM.28
Furthermore, it was also claimed to have inhibition activity against E. coli UT181, a pathogen that might cause diarrhea, urinary tract infection and pneumonia. The MIC of fermencin SA715 needed for the inhibition of E. coli UT181 was 103.57 μM. Moreover, S. aureus RF122 was proved to be inhibited by 103.57 μM of fermincin SA715.28 Fermencin SA715 was also proved to exhibit inhibition activity against Corynebacterium spp. GH17, pathogen that might cause pharyngitis, endocarditis, gastrointestinal tract infection and skin infection with MIC of 12.95 μM.28 Fermencin SD11 is produced by L. fermentum SD11 with a molecular size of 33
593 Da, and was found to exhibit a wide range of inhibition activity against Gram-positive bacteria and fungus.144 Fermencin SD11 was proved to be a useful antimicrobial agent to combat the oral pathogens, such as S. mutans ATCC 25175, S. sobrinus ATCC 33478, Aggregatibacter actinomycetemcomitans ATCC 33384, Fusobacterium nucleatum ATCC 25586, P. gingivalis ATCC 33277 and C. albicans ATCC 90028.145 Fermencin SD11 was proved to exhibit more than 125
000 AU per mL of antimicrobial activity against S. mutans ATCC 25175 and S. sobrinus ATCC 33478, and exhibit more than 9090.9 AU per mL of antimicrobial activity against A. actinomycetemcomitans ATCC 33384, F. nucleatum ATCC 25586, P. gingivalis ATCC 33277 and C. albicans ATCC 90028. In addition, it was also claimed to exert an inhibitory effect against LAB strains, which included Lactobacillus casei ATCC 393, L. fermentum ATCC 14931, Lactobacillus paracasei CCUG 32212. L. plantarum ATCC 14917, Lactobacillus rhamnosus ATCC 7469 and Lactobacillus salivarius ATCC 11741 with more than 125
000 AU per mL of antimicrobial activities.146
4. Application of bacteriocin in livestock health
Livestock are domesticated animals that are raised agriculturally to provide labor and commodities, such as milk, meat, eggs, furs and leathers. Livestock, as food sources, provide nutrients needed by humans everyday, such as proteins, fats and vitamins. It is important to have proper feeding and hygiene to sustain the livestock health and enhance the economic via maximized production. However, animals on the farm are still easily infected by viruses and bacteria. The examples of infectious diseases caused by bacteria in livestock are mastitis, post-weaning diarrhea, meningitis, arthritis, endocarditis, pneumonia and septicemia.147 Mastitis is a common disease that can be found in dairy cattle. It refers to the inflammation of the mammary gland and under tissue as the results of bacteria infection, chemical or thermal injury. Mastitis is normally caused by the contamination of the milking machine or hands with pathogens, such as S. aureus, Streptococcus uberis, and Streptococcus dysgalactiae, leading to damage in the milk-secreting tissues and ducts, and can be fatal for severe cases without proper treatments.148 To solve this problem, there are limitations in using antibiotic treatments, such as gentamicin, which may lead to the emergence of pathogens that are resistant to antibiotics.88 Besides that, another infectious disease that can be commonly transmitted in swine is post-weaning diarrhea (PWD). PWD is caused by an E. coli infection in the intestine of pigs, leading to diarrhea, dehydration, growth retardation in surviving piglets, as well as death without treatments.149 As a alternative to antibiotics, many research studies have investigated the use of antimicrobial agents or bacteriocin from LAB or other bacteria that exert inhibitory ability to inhibit or kill the pathogens in livestock.150–152 However, the bacteriocins that have been proven for health sustainability in livestock are less, which include nisin, lacticin and garvicin, and macedocin, as shown in Table 3.
Table 3 Application of bacteriocin from LAB in infectious disease treatment for livestock
Producing strain |
Types of bacteriocin |
Application in Livestock |
Targeted pathogens |
Pros and cons |
References |
L. lactis |
Nisin |
Meningitis, arthritis, endocarditis, pneumonia, and septicemia in swine and cow |
S. suis, S. aureus, A. suis, A. pleuropneumoniae and H. parasuis |
Pros: nisin possesses rapid bactericidal activity on S. suis, S. aureus, A. suis, A. pleuropneumoniae and H. parasuis. Besides that. It has synergistic effects in combination with several antibiotics, including penicillin, amoxicillin, tetracycline, streptomycin and cefazolin |
155 |
L. lactis subsp. lactis |
Nisin A |
Mastitis in lactating dairy cows |
S. aureus, S. intermedius, S. agalactiae, S. dysgalactiae, E. faecalis and E. coli |
Pros: nisin A can be used to replace cefazolin for mastitis treatment |
151 |
Cons: no preliminary study of the inhibition effect of nisin A with an animal model is carried out |
Nisin A and cefazolin |
Mastitis in lactating dairy cows |
S. aureus, S. intermedius, S. agalactiae, S. dysgalactiae, E. faecalis and E. coli |
Pros: nisin A can enhance the activity of cefazolin and reduce the antibiotic dose for mastitis treatment |
151 |
Cons: no preliminary study of the inhibition effect of combination of nisin A and cefazolin with an animal model is carried out |
L. lactis subsp. lactis DPC3147 |
Lacticin 3147 |
Mastitis in lactating dairy cows |
S. dysgalactiae M, S. aureus 10 and S. uberis |
Pros: the teat seal treated with lacticin 3147 show a decrease in the number of teats shedding viable cells significantly |
201 |
Lactococcus garvieae KS1546 |
Garvicin KS |
Mastitis in lactating dairy cows |
A. baumannii and S. aureus |
Pros: garvicin KS can be used alone in the inhibition of A. baumannii. It also acts synergistically with nisin in the inhibition of S. aureus. The combination of garvicin KS and nisin was proved to cause rapid eradication of all the S. aureus strains tested |
164 and 202 |
Cons: no preliminary study of the inhibition effect of garvicin KS on mastitis with an animal model is carried out |
S. gallolyticus subsp. macedonicus ST91KM |
Macedocin ST91KM |
Mastitis in lactating dairy cows |
S. agalactiae, S. dysgalactiae, S. uberis, S. aureus and S. epidermidis |
Pros: macedocin ST91KM has high heat stability in which it still remains active even at 100 °C. It shows rapid bacteriocidal mode of action against the mastitis pathogens |
203 |
Cons: no preliminary study of the inhibition effect of macedocin ST91KM on mastitis with an animal model is carried out. Besides that, there is a lack of studies on the synergetic effect of macedocin ST91KM with the currently applied antibiotics on mastitis |
4.1 Nisin
Nisin is a bacteriocin with a size of 3.5 kDa that has been approved by the FDA and WHO. Not only it can be applied in food preservation, but also can be used in the treatment of livestock diseases. The inhibitory activity of nisin is higher at neutral pH, and it has high resistance to heat.153 Nisin plays an important role in the treatment of respiratory tract infections in swine and cow. The most common respiratory infections caused by bacteria in swine are pneumonia and pleurisy, which lead to losses in the country economy. These diseases are mainly caused by the pathogen found in the respiratory tracts of pigs, such as S. aureus, S. suis, Actinobacillus suis, Actinobacillus pleuropneumoniae, and Haemophilus parasuis, which may be transmitted via contact between swine in a farm. There are broad-spectrum antibiotics needed to kill the pathogens, which cause respiratory infections in swine, leading to the emergence of drug-resistant pathogens that are harmful to animals and humans.154 To solve this problem, nisin extracted from L. lactis was proved to exert inhibitory effect against S. suis. Nisin was found to inhibit the growth of S. suis by disrupting the cell membrane of S. suis, which leads to cell lysis. Other than infection of the respiratory tract, nisin also contributes to the treatment of meningitis, arthritis, endocarditis, pneumonia and septicemia in swine. In the inhibition of the growth of S. suis, the minimum inhibitory concentration (MIC) and the minimum bactericidal concentration (MBC) of purified nisin needed are in the range of 1.25–5 μg mL−1 and 5–10 μg mL−1, respectively.155 Furthermore, nisin A was demonstrated as an antimicrobial agent that can inhibit the growth of pathogens, which cause mastitis in lactating dairy cows, including S. aureus, Staphylococcus intermedius, Streptococcus agalactiae, S. dysgalactiae, E. faecalis and E. coli with MIC of 1, 0.06, 0.25, 1, 4, 128 μg mL−1, respectively. However, the inhibition activity of both nisin A and cefazolin on the growth of mastitis pathogens was claimed to be improved when the combination of nisin A and cefazolin was used instead of using alone. The MIC of cefazolin alone needed for the inhibition of S. aureus, S. intermedius, S. agalactiae, S. dysgalactiae, E. faecalis and E. coli were 0.5, 0.5, 0.13, 0.13, 32 and 2 μg mL−1, respectively. For the combination use, the MIC of nisin A and cefazolin needed to inhibit S. aureus, S. intermedius, S. agalactiae, S. dysgalactiae, E. faecalis and E. coli were 0.25 and 0.06 μg mL−1, 0.03 and 0.13 μg mL−1, 0.13 and 0.06 μg mL−1, 0.5 and 0.06 μg mL−1, 0.5 and 8 μg mL−1, 32 and 1 μg mL−1, respectively.151
4.2 Lacticin
Lacticin 3147 is a common bacteriocin that has been used in the treatment of mastitis in lactating dairy cows to inhibit the growth of S. dysgalactiae M and S. aureus 10.151 In 1995, the first isolation of lacticin 3147 was done by extraction from an Irish kefir grain. Lacticin 3147 is extracted from L. lactis subsp. lactis DPC3147, which consists of the two peptides, which are Ltnα and Ltnβ in structure.156 There are many in vivo tests that have been done in previous studies. In one of the tests, a group of cows was given lacticin 3147 in to their teat seal, while another group of the group was without any addition of lacticin 3147. After that, all of their teats were introduced with S. dysgalactiae. The result showed that all of the cows from the group with the help of lacticin 3147 was free from mastitis, but 6 of the cows from the group without the addition of lacticin 3147 was infected by mastitis.157 Another test was carried out by coating the teats with pathogens that can cause mastitis, dipping with the lacticin 3147-containing fermented teat dip for 10 min. The result proved that there was a obvious reduction in the level of pathogens, in which the growth of staphylococci, S. dysgalactiae and S. uberis was reduced by 80%, 97% and 90%, respectively. These studies have proven that lacticin 3147 is contributing to the prevention of cows to be infected with mastitis. The concentration of one gram of oil based teat seal that contains 2560 AU lacticin 3147 was proved to have a significant inhibition effect against the pathogens that causes mastitis in cows. However, some studies demonstrated that the addition of Tween 80 can improve the inhibition activity.158
4.3 Garvicin
Garvicin is a class II bacteriocin produced by L. garvieae strains. L. garvieae is a Gram-positive, facultative and non-spore forming LAB that produces lactic acid as the final product of fermentation. It is resistant to high pH levels, including at pH 9.6. With the medium that contains 6.5% NaCl, it was able to growth optimally at the temperature range from 4 to 45 °C.159 L. garvieae can be found in the gastrointestinal tract of humans, but it was proved to have very low virulence to humans. L. garvieae can be isolated from dairy products, and the majority of them were found to have ability in the production of bacteriocins that can be used to kill or inhibit pathogens.160 Garvicin can be produced by different strains isolated from different sources. Some examples of garvicin are garvicin Q, KS, L1-5. ML and LG 34.161 Garvicin L1-5 is the first bacteriocin produced by L. garvieae to be reported, which can be isolated from cow's milk.162 Garvicin A belongs to a class IId bacteriocin that can be produced by L. garvie 21881. Garvicin Q can be obtained from the L. garvie isolated from fermented pork sausage.159 Besides that, garvicin ML can be produced by L. garvie from the intestine of a Mallard duck source, and garvicin LG34 can be produced by L. garvie from the Chinese traditional fermented cucumber source.163 However, there are only a few types of garvicin that have been proven to have antimicrobial effects on the pathogens found in livestock to cause infection disease. Garvicin KS produced by L. garvieae KS1546 has been demonstrated to have inhibitory activity against Acinetobacter baumannii and S. aureus, which cause mastitis in cows. However, there is a study that indicates the production of garvicin KS by L. garvieae KS1546 at the optimal factors in batch culture in GM17 is relatively low, which is only 80 BU per mL. Some research studies proved that the new medium formulation can enhance the productivity of garvicin KS. From the result, a 60-fold of garvicin KS can be produced if medium that contains pasteurized milk and tryptone is used. The production of garvicin KS can be further increased to around 20
000 BU per mL, which is 4-fold further if the increment of the gene dose of their gene cluster, gak, in the native producer is done. However, the highest garvicin KS that has been reported is 164
000 BU per mL, which was achieved by increasing the gene dose, maintaining the pH value at 6 and dissolved oxygen level at 50–60% in the medium for L. garvieae KS1546.164
4.4 Macedocin
Macedocin is a bacteriocin produced by Streptococcus macedonicus spp with around 2794.76 Da of molecular mass that comprises 22 amino acid residues. It is active within the pH range of 4 and 9, and it is resistant to high temperature and pressure.165 Macedocin was found to exhibit antimicrobial activities against mastitis pathogens that might be found in cows, which include S. agalactiae, S. dysgalactiae, S. uberis, S. aureus and S. epidermidis. The MIC of macedocin ST91KM from Streptococcus gallolyticus subsp. macedonicus ST91KM needed to inhibit the growth of S. agalactiae RPSAG1 and RPSAG2, which are resistant to oxacillin and methicillin, were proved at 640 and 1280 AU per mL. Besides that, the MIC of 640 AU per mL of macedocin ST91KM was claimed to exhibit inhibition activities against S. dysgalactiae RPSD1, RPSD2, RPSD3 and RPSD4, strains that are resistant to oxacillin and methicillin. Furthermore, the MIC of macedocin ST91KM for the inhibition of the growth of S. uberis RPSU1, RPSU2, EDSU1 and EDSU2, which are resistant to oxacillin and methicillin, were 640, 160, 640 and 160 AU per mL, respectively. In addition, the MIC of 640 AU per mL of macedocin ST91KM was proved to exert an inhibitory effect on the growth of S. aureus RPSA1, RPSA2, EDSA0267 and EDSA0269, which are resistant to oxacillin, penicillin and methicillin. Moreover, S. epidermidis RPSE1 (that is resistant to oxacillin, penicillin and methicillin) was proved to be inhibited with MIC of 2560 AU per mL of macedocin ST91KM.166
5. Overall conclusion and summary
Many research studies have proved that bacteriocin exerts antimicrobial activities on pathogenic bacteria that may cause infectious diseases such as pneumonia, dental carries, urinary tract infection, mastitis and meningitis. Bacteriocin has high potential to be used as the replacement for antibiotics in the future due to its low toxicity and proteinaceous nature for solving the problem of antibiotic resistance. Besides that, bacteriocin shows synergistic interaction with antibiotics, in which the combination of bacteriocin and antibiotics can enhance the inhibition or killing activities of antibiotics on pathogens, as well as reduce the dose of antibiotics to be used in the treatment of bacterial infections. In addition, bacteriocin re-shape the microbiota by killing the targeted pathogens without killing the other surrounding microbial community, which is preferable to be used as an antibacterial agent as compared to antibiotics. From previous studies, there are pros and cons of each bacteriocin species based on their expertise. Recently, the investment in bacteriocin research has shown a clear upward trend in response to the potential applications of these antimicrobial peptides in the field of food, livestock and medicines. The patent development for bacteriocins has been increasing since the last decades. However, there are still less bacteriocins to be marketed and approved for use in food preservation and treatment for infectious diseases by the FDA and WHO, as compared to antibiotics. This may be due to the lack of clinical tests done for bacteriocins to be used in humans or animals. In the future, more research studies should be done to completely characterize potent bacteriocins and clinically test it.
Funding
Authors acknowledge the financial support received by the Ministry of Higher Education (MOHE), Malaysia under Fundamental Research Grant Scheme (203/PTEKIND/6711624).
Conflicts of interest
The authors declare that they have no conflict of interest.
Acknowledgements
The author (Ng Zhang Jin) would like to express his great appreciation and gratitude to Universiti Sains Malaysia for giving him a USM Fellowship.
References
- S. J. Baker, D. J. Payne, R. Rappuoli and E. De Gregorio, Proc. Natl. Acad. Sci. U. S. A., 2018, 115, 12887–12895 CrossRef CAS.
- A. Langdon, N. Crook and G. Dantas, Genome Med., 2016, 8, 39 CrossRef.
- Q. Ma, C. Xing, W. Long, H. Y. Wang, Q. Liu and R.-F. Wang, J. Neuroinflammation, 2019, 16, 53 CrossRef.
- A. Dobson, P. D. Cotter, R. P. Ross and C. Hill, Appl. Environ. Microbiol., 2012, 78, 1–6 CrossRef CAS.
- S.-C. Yang, C.-H. Lin, C. T. Sung and J.-Y. Fang, Front. Microbiol., 2014, 5, 241 Search PubMed.
- L. Axelsson, 2004, pp. 1–66.
- F. Mozzi, in Encyclopedia of Food and Health, ed. B. Caballero, P. M. Finglas and F. Toldrá, Academic Press, Oxford, 2016, pp. 501–508, DOI:10.1016/B978-0-12-384947-2.00414-1.
- M. P. Mokoena, Molecules, 2017, 22, 1255 CrossRef.
- A. S. Oliveira, Z. G. Weinberg, I. M. Ogunade, A. A. P. Cervantes, K. G. Arriola, Y. Jiang, D. Kim, X. Li, M. C. M. Gonçalves, D. Vyas and A. T. Adesogan, J. Dairy Sci., 2017, 100, 4587–4603 CrossRef CAS.
- Y. An, Y. Wang, X. Liang, H. Yi, Z. Zuo, X. Xu, D. Zhang, C. Yu and X. Han, Food Control, 2017, 81, 211–217 CrossRef CAS.
- N. S. Ríos Colombo, M. C. Chalón, F. G. Dupuy, C. F. Gonzalez and A. Bellomio, Biochimie, 2019, 165, 183–195 CrossRef.
- M. Vaičikauskaitė, M. Ger, M. Valius, A. Maneikis, E. Lastauskienė, L. Kalėdienė and A. Kaunietis, Int. J. Biol. Macromol., 2019, 141, 333–344 CrossRef.
- C. Lay, B. Akerey, I. Fliss, M. Subirade and M. Rouabhia, J. Appl. Microbiol., 2008, 105, 1630–1639 CrossRef.
- J.-C. Ellis, R. P. Ross and C. Hill, Future Microbiol., 2019, 14, 1573–1587 CrossRef CAS.
- G. Kaur, T. P. Singh and R. K. Malik, Braz. J. Microbiol., 2013, 44, 63–71 CrossRef.
- S. Haruta and N. Kanno, Microbes Environ., 2015, 30, 123–125 CrossRef.
- L. H. Villalobos-Delgado, G. V. Nevárez-Moorillon, I. Caro, E. J. Quinto and J. Mateo, in Food Quality and Shelf Life, ed. C. M. Galanakis, Academic Press, 2019, pp. 125–157, DOI:10.1016/B978-0-12-817190-5.00004-5.
- T. C. Bhalla, Monika, Sheetal and Savitri, in Food Safety and Human Health, ed. R. L. Singh and S. Mondal, Academic Press, 2019, pp. 319–371, DOI:10.1016/B978-0-12-816333-7.00012-6.
- C. R. Erhabor, J. O. Erhabor and L. J. McGaw, S. Afr. J. Bot., 2019, 126, 214–231 CrossRef CAS.
- M. Al-Mamun, T. Chowdhury, B. Biswas and N. Absar, in Food Safety and Preservation, ed. A. M. Grumezescu and A. M. Holban, Academic Press, 2018, pp. 307–352, DOI:10.1016/B978-0-12-814956-0.00011-1.
- A. Castro, J. Silva and P. Teixeira, in Foodborne Diseases, ed. A. M. Holban and A. M. Grumezescu, Academic Press, 2018, pp. 213–238, DOI:10.1016/B978-0-12-811444-5.00008-7.
- R. Drolia and A. K. Bhunia, Trends Microbiol., 2019, 27, 408–425 CrossRef CAS.
- D. Kuang, J. Zhang, X. Xu, W. Shi, S. Chen, X. Yang, X. Su, X. Shi and J. Meng, Int. J. Food Microbiol., 2018, 280, 1–9 CrossRef CAS.
- M. Zanetti, T. K. Carniel, F. Dalcanton, R. S. dos Anjos, H. Gracher Riella, P. H. H. de Araújo, D. de Oliveira and M. Antônio Fiori, Trends Food Sci. Technol., 2018, 81, 51–60 CrossRef CAS.
- L. B. Said, H. Gaudreau, L. Dallaire, M. Tessier and I. Fliss, Ind. Biotechnol., 2019, 15, 138–147 CrossRef.
- E. Johnson, Y.-G. Jung, Y. Jin, R. Jayabalan, S. h. Yang and J.-W. Suh, Crit. Rev. Food Sci. Nutr., 2019, 58, 2743–2767 CrossRef.
- J. R. Linares-Morales, N. Gutiérrez-Méndez, B. E. Rivera-Chavira, S. B. Pérez-Vega and G. V. Nevárez-Moorillón, Frontiers in Sustainable Food Systems, 2018, 2, 1–13 CrossRef.
- S. B. Wayah and K. Philip, Microb. Cell Fact., 2018, 17, 125 CrossRef.
- F. Iordache, I. Gheorghe, V. Lazar, C. Curutiu, L. M. Ditu, A. M. Grumezescu and A. M. Holban, in Food Preservation, ed. A. M. Grumezescu, Academic Press, 2017, pp. 305–335, DOI:10.1016/B978-0-12-804303-5.00009-2.
- J. Delves-Broughton, Food Aust., 2005, 57, 525–527 CAS.
- R. J. da Costa, F. L. S. Voloski, R. G. Mondadori, E. H. Duval and Â. M. Fiorentini, J. Food Qual., 2019, 2019, 4726510 CrossRef.
- A. Abts, A. Mavaro, J. Stindt, P. J. Bakkes, S. Metzger, A. J. M. Driessen, S. H. J. Smits and L. Schmitt, Int. J. Pept., 2011, 2011, 175145 Search PubMed.
- N. Kitagawa, T. Otani and T. Inai, Anat. Sci. Int., 2019, 94, 163–171 CrossRef CAS.
- X. Zhao, C. Shi, R. Meng, Z. Liu, Y. Huang, Z. Zhao and N. Guo, J. Food Sci. Technol., 2016, 53, 2644–2653 CrossRef CAS.
- C. Piper, C. Hill, P. D. Cotter and R. P. Ross, Microb. Biotechnol., 2011, 4, 375–382 CrossRef CAS.
- M. A. F. Saraiva, D. J. Birri, D. A. Brede, M. C. Baracat-Pereira, M. V. de Queiroz, I. F. Nes and C. A. de Moraes, Int. J. Microbiol., 2020, 2020, 9309628 Search PubMed.
- S. Choyam, A. K. Srivastava, J.-H. Shin and R. Kammara, Front. Microbiol., 2019, 10, 1736 CrossRef.
- C. C. G. Silva, S. P. M. Silva and S. C. Ribeiro, Front. Microbiol., 2018, 9, 594 CrossRef.
- A. Gharsallaoui, N. Oulahal, C. Joly and P. Degraeve, Crit. Rev. Food Sci. Nutr., 2016, 56, 1262–1274 CrossRef CAS.
- J. P. Smith, D. P. Daifas, W. El-Khoury, J. Koukoutsis and A. El-Khoury, Crit. Rev. Food Sci. Nutr., 2004, 44, 19–55 CrossRef CAS.
- V. Scott and S. Taylor, J. Food Sci., 2006, 46, 117–126 CrossRef.
- Y. Fu, D. Mu, W. Qiao, D. Zhu, X. Wang, F. Liu, H. Xu, P. Saris, O. P. Kuipers and M. Qiao, Front. Microbiol., 2018, 9, 547 CrossRef.
- J. L. Raygada and D. P. Levine, Am. Health Drug Benefits, 2009, 2, 86–95 Search PubMed.
- E. J. Bottone, Clin. Microbiol. Rev., 2010, 23, 382–398 CrossRef.
- P. M. Periago and R. Moezelaar, Int. J. Food Microbiol., 2001, 68, 141–148 CrossRef CAS.
- K. Egan, D. Field, M. C. Rea, R. P. Ross, C. Hill and P. D. Cotter, Front. Microbiol., 2016, 7, 1–21 Search PubMed.
- M. R. Popoff, in Encyclopedia of Food Microbiology, ed. C. A. Batt and M. L. Tortorello, Academic Press, Oxford, second edn, 2014, pp. 474–480, DOI:10.1016/B978-0-12-384730-0.00069-0.
- M. Bergeron, C. Lay, L. Dridi, I. Fliss and M. Ouellette, J. Med. Microbiol., 2015, 65, 169–175 Search PubMed.
- M. J. Grande Burgos, R. P. Pulido, M. Del Carmen López Aguayo, A. Gálvez and R. Lucas, Int. J. Mol. Sci., 2014, 15, 22706–22727 CrossRef.
- K. Dubin and E. G. Pamer, Microbiol. Spectrum, 2017, 5(6), 1–16 Search PubMed.
- V. Farshbaf and A. Javadi, Afr. J. Microbiol. Res., 2011, 5, 3801–3804 Search PubMed.
- H. Khan, S. Flint and P.-L. Yu, Int. J. Food Microbiol., 2010, 141, 1–10 CrossRef CAS.
- A. Terzić-Vidojević, K. Veljović, J. Begović, B. Filipić, D. Popović, M. Tolinački, M. Miljković, M. Kojić and N. Golić, Front. Microbiol., 2015, 6, 954 Search PubMed.
- J. J. Luna-Guevara, M. M. P. Arenas-Hernandez, C. Martínez de la Peña, J. L. Silva and M. L. Luna-Guevara, Int. J. Microbiol., 2019, 2019, 2894328 CAS.
- P. Sharma, M. Rashid and S. Kaur, Microb. Cell Fact., 2020, 19, 98 CrossRef CAS.
- A. Gupta, S. K. Tiwari, V. Netrebov and M. L. Chikindas, Probiotics Antimicrob. Proteins, 2016, 8, 161–169 CrossRef CAS.
- A. Vimont, B. Fernandez, R. Hammami, A. Ababsa, H. Daba and I. Fliss, Front. Microbiol., 2017, 8, 865 CrossRef.
- M. Aspri, P. M. O'Connor, D. Field, P. D. Cotter, P. Ross, C. Hill and P. Papademas, Int. Dairy J., 2017, 73, 1–9 CrossRef CAS.
- V. P. Singh, Open Vet. J., 2018, 8, 104–111 CrossRef.
- M. T. Aymerich, M. Garriga, S. Costa, J. M. Monfort and M. Hugas, Int. Dairy J., 2002, 12, 239–246 CrossRef.
- P. Castellano, M. Pérez Ibarreche, M. Blanco Massani, C. Fontana and G. M. Vignolo, Microorganisms, 2017, 5, 38 CrossRef.
- C. Sabia, G. Manicardi, P. Messi, S. Niederhäusern and M. Bondi, Int. J. Food Microbiol., 2002, 75, 163–170 CrossRef CAS.
- R. Callewaert, M. Hugas and L. De Vuyst, Int. J. Food Microbiol., 2000, 57, 33–42 CrossRef CAS.
- S. Arbulu, J. J. Jiménez, L. Gútiez, C. Campanero, R. Del Campo, L. M. Cintas, C. Herranz and P. E. Hernández, BMC Microbiol., 2016, 16, 228 CrossRef.
- M. García, R. Lucas, H. Abriouel, N. El Bakali, R. Pulido, M. Grande, M. Martinez-Canamero and A. Gálvez, J. Appl. Microbiol., 2004, 97, 731–737 CrossRef.
- T. Hata, T. Sato and Y. Morimitsu, J. Food Saf., 2015, 36, 348–359 CrossRef.
- M. Papagianni and S. Anastasiadou, Microb. Cell Fact., 2009, 8, 3 CrossRef.
- C. T. Lohans and J. C. Vederas, Int. J. Microbiol., 2012, 2012, 386410 Search PubMed.
- J. Nieto-Lozano, J. Reguera-Useros, C. Peláez, G. Sacristán, A. Gutiérrez and A. Torre, Food Control, 2010, 21, 679–685 CrossRef CAS.
- G. A. Somkuti and D. Steinberg, Biotechnol. Lett., 2003, 25, 473–477 CrossRef CAS.
- H. Zhang, B. Kong, Y. Xiong and X. Sun, Meat Sci., 2008, 81, 686–692 CrossRef.
- X. Ming, G. Weber, J. Ayres and W. Sandine, J. Food Sci., 2006, 62, 413–415 CrossRef.
- H. Hassanzadazar, A. Ehsani and K. Mardani, Vet. Res. Forum, 2014, 5, 169–175 Search PubMed.
- N. Sawa, K. Okamura, T. Zendo, K. Himeno, J. Nakayama and K. Sonomoto, J. Appl. Microbiol., 2010, 109, 282–291 CAS.
- D. R. Balay, R. V. Dangeti, K. Kaur and L. M. McMullen, Int. J. Food Microbiol., 2017, 255, 25–31 CrossRef CAS.
- D. R. Balay, M. G. Gänzle and L. M. McMullen, Front. Microbiol., 2018, 9, 347 CrossRef.
- X. Liu, J. C. Vederas, R. M. Whittal, J. Zheng, M. E. Stiles, D. Carlson, C. M. A. P. Franz, L. M. McMullen and M. J. van Belkum, J. Agric. Food Chem., 2011, 59, 5602–5608 CrossRef CAS.
- E. Tirloni, V. D. Pietro, G. Rizzi, F. Pomilio, P. Cattaneo, C. Bernardi and S. Stella, Ital. J. Food Saf., 2019, 8, 8112 CAS.
- I.-C. Hwang, J. K. Oh, S. H. Kim, S. Oh and D.-K. Kang, Korean J. Food Sci. Anim. Resour., 2018, 38, 1008–1018 CrossRef.
- T. Saraoui, F. Leroi, F. Chevalier, J.-M. Cappelier, D. Passerini and M.-F. Pilet, Front. Microbiol., 2018, 9, 1564 CrossRef.
- L. Martin-Visscher, S. Yoganathan, C. Sit, C. Lohans and J. Vederas, FEMS Microbiol. Lett., 2011, 317, 152–159 CrossRef CAS.
- D. Bizani, J. Morrissy, A. Dominguez and A. Brandelli, Int. J. Food Microbiol., 2008, 121, 229–233 CrossRef CAS.
- A. F. d. S. Duarte, H. Ceotto, M. L. V. Coelho, M. A. V. d. P. Brito and M. d. C. d. F. Bastos, Food Control, 2013, 32, 313–321 CrossRef CAS.
- E. A. Elsayed, M. A. Farid and H. A. El-Enshasy, BMC Biotechnol., 2019, 19, 46 CrossRef.
- S. A. S. Al-Qaysi, H. Al-Haideri, Z. A. Thabit, W. H. A. A.-R. Al-Kubaisy and J. A. A.-R. Ibrahim, Int. J. Microbiol., 2017, 2017, 2605382 Search PubMed.
- Y. Wu, J. An, Y. Liu, Y. Wang, W. Ren, Z. Fang, L. Sun and R. Gooneratne, Arch. Microbiol., 2019, 201, 61–66 CrossRef CAS.
- W. A. Adedeji, Ann. Ib. Postgrad. Med., 2016, 14, 56–57 CAS.
- J. M. Munita and C. A. Arias, Microbiol. Spectrum, 2016, 4(2), 1–37 CAS.
- H. Mathur, D. Field, M. C. Rea, P. D. Cotter, C. Hill and R. P. Ross, npj Biofilms Microbiomes, 2018, 4, 9 CrossRef.
- H. S. Abanoz and B. Kunduhoglu, Korean J. Food Sci. Anim. Resour., 2018, 38, 1064–1079 CrossRef.
- E. Meade, M. A. Slattery and M. Garvey, Antibiotics, 2020, 9, 32 CrossRef CAS.
- H. Mathur, D. Field, M. C. Rea, P. D. Cotter, C. Hill and R. P. Ross, Front. Microbiol., 2017, 8, 1205 CrossRef.
- S. Agnes Mary and V. R. Giri Dev, J. Text. Inst., 2015, 106, 886–895 CrossRef CAS.
- C. Ghose and C. P. Kelly, Infect. Dis. Clin., 2015, 29, 145–162 CrossRef.
- C. L. Ventola, P T, 2015, 40, 277–283 Search PubMed.
- D. Field, R. O'Connor, P. D. Cotter, R. P. Ross and C. Hill, Front. Microbiol., 2016, 7, 508 Search PubMed.
- Z. Tong, L. Dong, L. Zhou and R. Tao, Peptides, 2010, 31, 2003–2008 CrossRef CAS.
- M.-C. Dignani, J. S. Solomkin and E. J. Anaissie, in Clinical Mycology, ed. E. J. Anaissie, M. R. McGinnis and M. A. Pfaller, Churchill Livingstone, Edinburgh, second edn, 2009, pp. 197–229, DOI:10.1016/B978-1-4160-5680-5.00008-6.
- N. Décanis, N. Tazi, A. Correia, M. Vilanova and M. Rouabhia, Open Microbiol. J., 2011, 5, 119–126 CrossRef.
- M. Kwaadsteniet, K. T. Doeschate and L. Dicks, Lett. Appl. Microbiol., 2008, 48, 65–70 CrossRef.
- H.-Y. Song, L. Zhou, D.-y. Liu, X.-J. Yao and Y. Li, Gastroenterology Research and Practice, 2018, 2018, 9379480 Search PubMed.
- S. Preet, S. Bharati, A. Panjeta, R. Tewari and P. Rishi, Tumor Biol., 2015, 36, 8301–8308 CrossRef CAS.
- N. Mirkovic, N. Polovic, G. Vukotic, B. Jovcic, M. Miljkovic, Z. Radulovic, D. B. Diep and M. Kojic, Appl. Environ. Microbiol., 2016, 82, 2555–2562 CrossRef CAS.
- S. M. Morgan, P. M. O'Connor, P. D. Cotter, R. P. Ross and C. Hill, Antimicrob. Agents Chemother., 2005, 49, 2606–2611 CrossRef CAS.
- G. Aubin, M. Portillo, A. Trampuz and S. Corvec, Med. Mal. Infect., 2014, 44(6), 241–250 CrossRef CAS.
- E. B. O'Connor, B. O'Riordan, S. M. Morgan, H. Whelton, D. M. O'Mullane, R. P. Ross and C. Hill, J. Appl. Microbiol., 2006, 100, 1251–1260 CrossRef.
- A. Neshani, H. Zare, M. R. Akbari Eidgahi, A. Hooshyar Chichaklu, A. Movaqar and K. Ghazvini, Helicobacter, 2019, 24, e12555 CrossRef.
- I. Titze and V. Krömker, Vet. Sci., 2020, 7, 31 CrossRef.
- D. J. Birri, D. A. Brede and I. F. Nes, Appl. Environ. Microbiol., 2012, 78, 402–410 CrossRef CAS.
- M. Geng, F. Austin, R. Shin and L. Smith, Appl. Environ. Microbiol., 2018, 84(5), 1–15 CAS.
- M. Reglinski and S. Sriskandan, in Molecular Medical Microbiology, ed. Y.-W. Tang, M. Sussman, D. Liu, I. Poxton and J. Schwartzman, Academic Press, Boston, second edn, 2015, pp. 675–716, DOI:10.1016/B978-0-12-397169-2.00038-X.
- A. Barbour, J. Tagg, O. K. Abou-Zied and K. Philip, Sci. Rep., 2016, 6, 31749 CrossRef CAS.
- D. W. Scott and W. H. Miller, in Equine Dermatology, ed. D. W. Scott and W. H. Miller, W.B. Saunders, Saint Louis, second edn, 2011, pp. 130–170, DOI:10.1016/B978-1-4377-0920-9.00004-4.
- S. Edagiz, P. Lagace-Wiens, J. Embil, J. Karlowsky and A. Walkty, Can. J. Infect Dis. Med. Microbiol., 2015, 26, 105–107 CrossRef.
- T. Patel, A. Molloy, R. Smith and I. Balakrishnan, Infect. Dis. Rep., 2012, 4, e31 CrossRef.
- S. Cooper, in Bacterial Growth and Division, ed. S. Cooper, Academic Press, San Diego, 1991, pp. 358–374, DOI:10.1016/B978-0-08-091747-4.50020-5.
- N. Khochamit, S. Siripornadulsil, P. Sukon and W. Siripornadulsil, Microbiol. Res., 2015, 170, 36–50 CrossRef CAS.
- C. E. Shelburne, F. Y. An, V. Dholpe, A. Ramamoorthy, D. E. Lopatin and M. S. Lantz, J. Antimicrob. Chemother., 2007, 59, 297–300 CrossRef CAS.
- K. Y. How, K. P. Song and K. G. Chan, Front. Microbiol., 2016, 7, 53 Search PubMed.
- P. Patilaya, D. I. Husori and L. Marhafanny, Open Access Maced. J. Med. Sci., 2019, 7, 3861–3864 CrossRef.
- K. Becker, F. Rutsch, A. Uekötter, F. Kipp, J. König, T. Marquardt, G. Peters and C. von Eiff, J. Clin. Microbiol., 2008, 46, 3537–3539 CrossRef.
- S. Macé, L. Truelstrup Hansen and H. P. V. Rupasinghe, Medicines, 2017, 4, 25 CrossRef.
- M. Â. B. Sousa, E. N. Mendes, G. B. Collares, L. A. Péret-Filho, F. J. Penna and P. P. Magalhães, Mem. Inst. Oswaldo Cruz, 2013, 108, 30–35 CrossRef.
- M. Bassetti, A. Vena, A. Croxatto, E. Righi and B. Guery, Drugs Context, 2018, 7, 212527 Search PubMed.
- S. Schmitz, A. Hoffmann, C. Szekat, B. Rudd and G. Bierbaum, Appl. Environ. Microbiol., 2006, 72, 7270–7277 CrossRef CAS.
- P. Toltzis, in Principles and Practice of Pediatric Infectious Diseases, ed. S. S. Long, C. G. Prober and M. Fischer, Elsevier, Fifth edn, 2018, pp. 706–712.e704, DOI:10.1016/B978-0-323-40181-4.00116-X.
- P. Sass, A. Jansen, C. Szekat, V. Sass, H.-G. Sahl and G. Bierbaum, BMC Microbiol., 2008, 8, 186 CrossRef.
- A. N. Appleyard, S. Choi, D. M. Read, A. Lightfoot, S. Boakes, A. Hoffmann, I. Chopra, G. Bierbaum, B. A. M. Rudd, M. J. Dawson and J. Cortes, Chem. Biol., 2009, 16, 490–498 CrossRef CAS.
- J. Barbosa, T. Caetano and S. Mendo, J. Nat. Prod., 2015, 78, 2850–2866 CrossRef CAS.
- M. Nasaj, S. M. Mousavi, S. M. Hosseini and M. R. Arabestani, Iran. J. Public Health, 2016, 45, 806–813 Search PubMed.
- Z. Yıldırım, S. Yerlikaya, N. Öncül and T. Sakin, Food Technol. Biotechnol., 2016, 54, 317–323 Search PubMed.
- T. Mateus, J. Silva, R. L. Maia and P. Teixeira, ISRN Obstet Gynecol., 2013, 2013, 851712 Search PubMed.
- T. Ghrairi and K. Hani, J. Food Sci. Technol., 2015, 52, 2148–2156 CrossRef CAS.
- P. Sheoran and S. K. Tiwari, J. Appl. Microbiol., 2019, 126, 1059–1069 CrossRef CAS.
- C. S. Sit, S. Yoganathan and J. C. Vederas, Acc. Chem. Res., 2011, 44, 261–268 CrossRef CAS.
- M. Otto, Expert Rev. Dermatol., 2010, 5, 183–195 CrossRef CAS.
- T. Bengtsson, J. Lönn, H. Khalaf and E. Palm, MicrobiologyOpen, 2018, 7, e00606 CrossRef.
- F. Götz, S. Perconti, P. Popella, R. Werner and M. Schlag, Int. J. Med. Microbiol., 2014, 304, 63–71 CrossRef.
- M. Mota-Meira, G. Lapointe, C. Lacroix and M. C. Lavoie, Antimicrob. Agents Chemother., 2000, 44, 24–29 CrossRef CAS.
- S. Siddharth and R. R. Vittal, Microorganisms, 2018, 6, 72 CrossRef CAS.
- R. R. Bonelli, T. Schneider, H.-G. Sahl and I. Wiedemann, Antimicrob. Agents Chemother., 2006, 50, 1449–1457 CrossRef CAS.
- B. L. Hardy, G. Bansal, K. H. Hewlett, A. Arora, S. D. Schaffer, E. Kamau, J. W. Bennett and D. S. Merrell, Front. Microbiol., 2020, 10, 2977 CrossRef.
- F. Götz, T. Bannerman and K.-H. Schleifer, Prokaryotes, 2006, 5–75, DOI:10.1007/0-387-30744-3_1.
- P. Wannun, S. Piwat and R. Teanpaisan, Appl. Biochem. Biotechnol., 2016, 179, 572–582 CrossRef CAS.
- S. Periasamy and P. E. Kolenbrander, J. Bacteriol., 2009, 191, 6804–6811 CrossRef CAS.
- M.-S. Kang, J.-S. Oh, H.-C. Lee, H.-S. Lim, S.-W. Lee, K.-H. Yang, N.-K. Choi and S.-M. Kim, J. Microbiol., 2011, 49, 193–199 CrossRef CAS.
- P. Varijakshapanicker, S. McKune, L. Miller, S. Hendrickx, M. Balehegn, G. E. Dahl and A. T. Adesogan, Anim. Front., 2019, 9, 39–50 CrossRef.
- R. N. Zadoks, J. R. Middleton, S. McDougall, J. Katholm and Y. H. Schukken, J. Mammary Gland Biol. Neoplasia, 2011, 16, 357–372 CrossRef.
- M. Rhouma, J. M. Fairbrother, F. Beaudry and A. Letellier, Acta Vet. Scand., 2017, 59, 31 CrossRef.
- A. Ben Lagha, B. Haas, M. Gottschalk and D. Grenier, Vet. Res., 2017, 48, 22 CrossRef.
- R. Pieterse and S. D. Todorov, Braz. J. Microbiol., 2010, 41, 542–562 CrossRef.
- F. Diez-Gonzalez, Curr. Issues Intest. Microbiol., 2007, 8, 15–23 CAS.
- M. d. R. López-Cuellar, A.-I. Rodríguez-Hernández and N. Chavarría-Hernández, Biotechnol. Biotechnol. Equip., 2016, 30, 1039–1050 CrossRef.
- M. Torremorrell, in Current Therapy in Large Animal Theriogenology, ed. R. S. Youngquist and W. R. Threlfall, W.B. Saunders, Saint Louis, second edn, 2007, pp. 794–801, DOI:10.1016/B978-072169323-1.50109-4.
- G. Lebel, F. Piché, M. Frenette, M. Gottschalk and D. Grenier, Peptides, 2013, 50, 19–23 CrossRef CAS.
- S. Suda, A. Westerbeek, P. M. O'Connor, R. P. Ross, C. Hill and P. D. Cotter, Chem. Biol., 2010, 17, 1151–1160 CrossRef CAS.
- F. Crispie, J. Flynn, R. P. Ross, C. Hill and W. J. Meaney, Ir. Vet. J., 2004, 57, 652 CrossRef.
- K. Klostermann, F. Crispie, J. Flynn, W. J. Meaney, R. Paul Ross and C. Hill, J. Dairy Res., 2010, 77, 231–238 CrossRef CAS.
- A. Tosukhowong, T. Zendo, W. Visessanguan, S. Roytrakul, L. Pumpuang, J. Jaresitthikunchai and K. Sonomoto, Appl. Environ. Microbiol., 2012, 78, 1619–1623 CrossRef CAS.
- E. N. Abdelfatah and H. H. H. Mahboub, Int. J. Vet. Sci. Med., 2018, 6, 201–207 CrossRef.
- A. Tymoszewska, D. B. Diep, P. Wirtek and T. Aleksandrzak-Piekarczyk, Sci. Rep., 2017, 7, 8359 CrossRef.
- F. Villani, M. Aponte, G. Blaiotta, G. Mauriello, O. Pepe and G. Moschetti, J. Appl. Microbiol., 2001, 90, 430–439 CrossRef CAS.
- Y. Gao, D. Li, S. Liu and L. Zhang, Food Control, 2015, 50, 896–900 CrossRef CAS.
- A. A. Telke, K. V. Ovchinnikov, K. S. Vuoristo, G. Mathiesen, T. Thorstensen and D. B. Diep, Front. Microbiol., 2019, 10, 1–9 CrossRef.
- M. Georgalaki, E. Berghe, D. Kritikos, B. Devreese, J. Beeumen, G. Kalantzopoulos, L. De Vuyst and E. Tsakalidou, Appl. Environ. Microbiol., 2003, 68, 5891–5903 CrossRef.
- R. Pieterse, S. Todorov and L. Dicks, Braz. J. Microbiol., 2010, 41, 133–145 CrossRef.
- R. O. Benech, E. E. Kheadr, C. Lacroix and I. Fliss, J. Dairy Sci., 2003, 86, 1895–1909 CrossRef CAS.
- L. A. Ibarra-Sánchez, N. El-Haddad, D. Mahmoud, M. J. Miller and L. Karam, J. Dairy Sci., 2020, 103, 2041–2052 CrossRef.
- H. Khan, S. Flint and P.-L. Yu, Int. J. Food Microbiol., 2010, 141, 1–10 CrossRef CAS.
- Y. Alvarez, T. Sainz, C. Wacher, F. Fernández and E. Ponce-Alquicira, Science Against Microbial Pathogens: Communicating Current Research and Technological Advances, 2011, pp. 1331–1341 Search PubMed.
- A. Jofré, T. Aymerich, J. Monfort and M. Garriga, Eur. Food Res. Technol., 2008, 228, 159–162 CrossRef.
- A. Muñoz, M. Maqueda, A. Gálvez, M. Martínez-Bueno, A. Rodríguez and E. Valdivia, J. Food Prot., 2004, 67, 1517–1521 CrossRef.
- A. Muñoz, S. Ananou, A. Gálvez, M. Martínez-Bueno, A. Rodriguez, M. Maqueda and E. Valdivia, Int. Dairy J., 2007, 17, 760–769 CrossRef.
- M. Grande Burgos, R. Lucas, H. Abriouel, E. Valdivia, N. El Bakali, M. Maqueda, M. Martínez-Bueno, M. Martinez-Canamero and A. Gálvez, Int. J. Food Microbiol., 2006, 106, 185–194 CrossRef.
- M. J. Grande, R. Lucas, E. Valdivia, H. Abriouel, M. Maqueda, N. Ben Omar, M. Martínez-Cañamero and A. Gálvez, J. Food Prot., 2005, 68, 2085–2094 CrossRef CAS.
- M. Grande Burgos, R. Lucas, H. Abriouel, E. Valdivia, N. El Bakali, M. Maqueda, M. Martinez-Canamero and A. Gálvez, J. Appl. Microbiol., 2006, 101, 422–428 CrossRef.
- M. Grande Burgos, H. Abriouel, R. López, E. Valdivia, N. El Bakali, M. Martinez-Canamero and A. Gálvez, J. Food Prot., 2007, 70, 2339–2345 CrossRef.
- M. J. Grande, R. L. López, H. Abriouel, E. Valdivia, N. Ben Omar, M. Maqueda, M. Martínez-Cañamero and A. Gálvez, J. Food Prot., 2007, 70, 405–411 CrossRef CAS.
- S. Ananou, A. Baños, M. Maqueda, M. Martínez-Bueno, A. Gálvez and E. Valdivia, Food Control, 2010, 21, 478–486 CrossRef CAS.
- R. Lucas, M. J. Grande, H. Abriouel, M. Maqueda, N. Ben Omar, E. Valdivia, M. Martínez-Cañamero and A. Gálvez, Food Chem. Toxicol., 2006, 44, 1774–1781 CrossRef CAS.
- L. M. T. Dicks, S. D. Todorov, M. P. van der Merwe, A. Dalton and L. C. Hoffman, J. Food Saf., 2006, 26, 173–183 CrossRef CAS.
- M. Farías, A. Holgado and F. Sesma, J. Food Prot., 1994, 57, 1013–1015 CrossRef.
- M. T. García, R. Lucas, H. Abriouel, N. B. Omar, R. Pérez, M. J. Grande, M. Martínez-Cañamero and A. Gálvez, J. Appl. Microbiol., 2004, 97, 731–737 CrossRef.
- A. Lauková, S. Czikková, T. Dobránsky and O. Burdová, Food Microbiol., 1999, 16, 93–99 CrossRef.
- A. Laukova, G. Vlaemynck and S. Czikková, Folia Microbiol., 2001, 46, 157–160 CrossRef CAS.
- A. Laukova and P. Turek, Acta Sci. Pol., Technol. Aliment., 2011, 10, 423–431 CAS.
- M. Cabo, B. Torres, J. J. Rodríguez Herrera, M. Bernárdez and L. Pastoriza, J. Food Prot., 2009, 72, 515–523 CrossRef CAS.
- S. M. Devi, A. M. Ramaswamy and P. M. Halami, J. Food Sci. Technol., 2014, 51, 3325–3332 CrossRef CAS.
- F. Shi, Y. Wang, Y. Li and X. Wang, Biotechnol. Lett., 2016, 38, 1551–1557 CrossRef CAS.
- K. Makhloufi, A. Carré-Mlouka, P. Jean, C. Lombard, C. Van Reenen, L. Dicks and S. Rebuffat, PLoS One, 2013, 8, e70484 CrossRef CAS.
- Z. Yildirim, N. Oncul, M. Yıldırım and S. Karabiyikli, Acta Aliment., 2016, 45, 486–492 CrossRef CAS.
- P. Carlin Fagundes, F. Miceli de Farias, O. Cabral da Silva Santos, J. A. Souza da Paz, H. Ceotto-Vigoder, D. Sales Alviano, M. T. Villela Romanos and M. d. C. de Freire Bastos, Int. J. Food Microbiol., 2016, 237, 39–46 CrossRef CAS.
- C. Ollé Resa, J. Rosa and L. Gerschenson, Food Control, 2014, 35, 101–108 CrossRef.
- J. An, W. Zhu, Y. Liu, X. Zhang, L. Sun, P. Hong, Y. Wang, C. Xu, X. Defeng and H. Liu, Food Control, 2015, 51, 541–550 CrossRef.
- D. Field, N. Seisling, P. D. Cotter, R. P. Ross and C. Hill, Front. Microbiol., 2016, 7, 1713 Search PubMed.
- S. Ahmadi, M. Ghollasi and H. M. Hosseini, Microb. Pathog., 2017, 111, 193–197 CrossRef CAS.
- S. Preet and S. Pandey, Transl. Med., 2016, 06, 336–340 Search PubMed.
- M. De Kwaadsteniet, K. T. Doeschate and L. M. T. Dicks, Lett. Appl. Microbiol., 2009, 48, 65–70 CrossRef CAS.
- C. L. Lay, L. Dridi, M. G. Bergeron, M. Ouellette and I. l. Fliss, J. Med. Microbiol., 2016, 65, 169–175 CrossRef.
- D. Kruszewska, H.-G. Sahl, G. Bierbaum, U. Pag, S. O. Hynes and Å. Ljungh, J. Antimicrob. Chemother., 2004, 54, 648–653 CrossRef CAS.
- D. P. Twomey, A. I. Wheelock, J. Flynn, W. J. Meaney, C. Hill and R. P. Ross, J. Dairy Sci., 2000, 83, 1981–1988 CrossRef CAS.
- H. Chi and H. Holo, Curr. Microbiol., 2018, 75, 272–277 CrossRef CAS.
- R. Pieterse, S. D. Todorov and D. Leon M T, Braz. J. Microbiol., 2010, 41, 133–145 CrossRef.
|
This journal is © The Royal Society of Chemistry 2020 |
Click here to see how this site uses Cookies. View our privacy policy here.