DOI:
10.1039/C9QI01589B
(Review Article)
Inorg. Chem. Front., 2020,
7, 839-852
Inorganic planar π-conjugated groups in nonlinear optical crystals: review and outlook
Received
5th December 2019
, Accepted 13th January 2020
First published on 13th January 2020
Abstract
Nonlinear optical (NLO) materials have been playing significant roles in laser science and technology for converting laser wavelengths to spectral regions where normal lasers operated poorly. Inorganic planar π-conjugated groups possessing both large second harmonic generation (SHG) responses and optical anisotropy are one of the most desirable fundamental building units (FBUs) for NLO materials. Up to now, inorganic planar π-conjugated groups including [BO3], [B3O6], [CO3], [NO3], [HxC3N3O3] (x varying from 0 to 3), [BS3], [B3S6], [HgSe3], [AgSe3], and [B2P5] units are reported as NLO-active groups, which strongly enhance the structural diversity of NLO materials. In this review, the NLO materials containing inorganic planar π-conjugated groups are classified according to their shortest transparent regions, i.e. deep-ultraviolet (DUV, λ < 200 nm), ultraviolet (UV, 200 nm < λ < 380 nm), visible (VIS, 380 nm < λ < 780 nm), and infrared (IR, λ > 780 nm) regions. On the basis of available experimental and calculated data, the structure–property relationships and potential applied wave ranges for different inorganic planar π-conjugated groups are discussed.
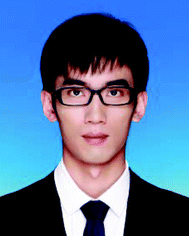 Pifu Gong | Pifu Gong received his B.S. in Physics from Shandong Normal University and then obtained his Ph.D. in Condensed Matter Physics from Technical Institute of Physics and Chemistry (TIPC), Chinese Academy of Sciences (CAS) in 2017. His current research focuses on developing new nonlinear-optical materials. |
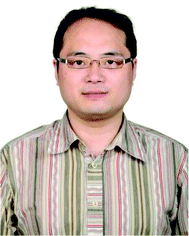 Zheshuai Lin | Zheshuai Lin obtained his Ph.D. from Fujian Institute of Research on the Structure of Matter, CAS, in 2002. Since 2008, he has been working as a professor at TIPC, CAS. His research into nonlinear-optical crystals employs a variety of modeling techniques spanning analytical and quantum mechanics. |
Introduction
As important photoelectric functional materials, nonlinear optical (NLO) crystals have played unique roles in laser frequency conversion technology, which can efficiently expand the spectral regions of common laser sources.1 Owing to their excellent performances, NLO crystals in all-solid-state laser systems have been widely applied in industrial processing, semiconductor manufacturing, photolithography, military confrontation, noninvasive medical diagnostics and signal communications.2–5 After making continuous effort for decades, many useful NLO crystals, such as KBe2BO3F2 (KBBF), β-BaB2O4 (BBO), LiB3O5 (LBO), KTiOPO4 (KTP), AgGaS2 (AGS), etc. have been developed and applied for laser frequency conversion in the deep ultraviolet (DUV), ultraviolet (UV), visible (VIS) and infrared (IR) regions.6–11 Meanwhile, great progress has been made on the research of structure–property relationships, which reveal the deterministic effects of the NLO-active units on the NLO performances.12–17
To realize laser frequency conversion, a NLO crystal must satisfy several fundamental but rigorous requirements, including a wide transparent window for an operating wave range, large second harmonic generation (SHG) response, and sufficient birefringence to achieve phase matching.18,19 All of them are closely related to the fundamental building units (FBUs). On the basis of structure–property relationships, planar π-conjugated groups, second-order Jahn–Teller (SOJT) distorted [MQ6] (M = Ti, Nb, Ta, Mo, W, Te, etc.; Q = O, S, Se, Te) groups, distorted polyhedra centered by stereochemically active long pair (SALP) cations (Pb, Bi, Sb, I, etc.), rare-earth cation centered polyhedra, and metal element centered [MQ4] (M = Zn, Al, In, Ga, Ge, etc.; Q = O, S, Se, Te) tetrahedra are regarded as NLO-active units.20–23 Among them, the planar π-conjugated groups with large SHG responses and optical anisotropy are one of the most desirable FBUs for NLO materials and many materials have achieved practical laser frequency conversion in DUV and UV regions using these groups.
Up to now, the reported planar π-conjugated NLO-active groups include [BO3], [B3O6], [CO3], [NO3], [HxC3N3O3] (x varying from 0 to 3), [BS3], [B3S6], [HgSe3], [AgSe3], and [B2P5] units. [BO3] and [B3O6] possessing π43 and π99 configurations (where the subscript is the number of atoms constructing the π-conjugated group and the superscript is the number of π-electrons constructing the π-orbitals), respectively, are the most common FBUs for DUV and UV NLO borates. In 2018, Prof. Zhao reviewed the role of cations in [BO3]-based NLO materials and Prof. Pan provided a comprehensive review of fluorooxoborates, which provide a better understanding of the structure–property relationships in NLO borates.24,25 [CO3] (π44) and [NO3] (π45) have similar structural configurations to [BO3], but provide more π electrons on the delocalized π orbitals, which results in larger SHG responses. Our group also systematically reviewed the NLO carbonates and nitrates and investigated the relationship between the SHG responses and the arrangements of the planar π-conjugated NLO-active groups inside.26,27 In 2017, our group pointed out that the [HxC3N3O3] (π99) groups could serve as NLO-active units for their similar electronic and structural configuration to the [B3O6] units.28 After that, dozens of hydro-cyanurates and cyanurates are reported, which strongly promote the development of related fields.29–32 Very recently, the no-oxygen-containing planar π-conjugated units, i.e. [BS3] (π43), [B2S6] (π99), [HgSe3] (π42), [AgSe3] (π41), and [B2P5] (π75), have been reported as NLO-active units and have attracted much attention in the exploration of related IR NLO materials. All these reported planar π-conjugated NLO-active groups can achieve both large SHG responses and optical anisotropy, which make them the most desirable FBUs for NLO materials.
Although some reviews have been reported for certain kinds of planar π-conjugated groups, a systematic review covering all the planar NLO-active groups is absent.33–36 In this work, all the kinds of inorganic planar π-conjugated groups are covered and the related NLO materials are classified according to their shortest transparent regions, i.e. deep-ultraviolet (DUV, λ < 200 nm), ultraviolet (UV, 200 nm < λ < 380 nm), visible (VIS, 380 nm < λ < 780 nm), and infrared (IR, λ > 780 nm) regions. On the basis of available experimental and calculated data, the potential applied wave ranges for different inorganic planar π-conjugated groups are investigated. In addition, the structure–property relationships and further developments of the NLO materials containing planar π-conjugated groups are discussed.
2. DUV transparent NLO crystals containing planar π-conjugated groups
According to our survey, only the NLO materials with [BO3], [B3O6], [CO3] and [NO3] units possess the property of DUV transparency. In this section, the structures and main optical properties of the related DUV transparent NLO crystals are discussed based on their planar π-conjugated groups.
2.1 DUV transparent borate with planar [BO3] and [B3O6] units
The ABe2BO3F2 (A = Na, K, Rb, Cs, NH4, Tl) family is a classical series of NLO materials. To date, KBe2BO3F2 (KBBF) is still the solo practically usable DUV NLO crystal to generate coherent light shorter than 200 nm by a direct frequency doubling method. This unique ability is attributed to its short UV cutoff edge (146 nm), large SHG coefficient (d11 = 0.47 pm V−1), and moderate birefringence (0.088 at 400 nm). However, KBBF suffers a strong layering growth tendency for the weak F−–K+ electrostatic attraction between the adjacent [Be2BO3F2]∞ layers, which strongly hinders its commercialization. To enhance the interlayer forces, NH4+ cations are introduced to replace the K+ cations in KBBF. NH4Be2BO3F2 (ABBF) possesses the same structure as KBBF, but introduces the hydrogen bonds between the layers.37 As per analysis, ABBF exhibits comparable optical properties to KBBF, such as a short UV cutoff edge (153 nm), large SHG response (1.2 times that of KH2PO4, i.e. 1.2 × KDP), and moderate birefringence (0.078 at 400 nm). Ultimately, when A-site cations are cut off to overcome the layering tendency of KBBF, γ-Be2BO3F (γ-BBF) was obtained. γ-BBF crystallizes in the non-centrosymmetric (NCS) trigonal space group R32.37 Its powder SHG response is found to be 2.3 times that of KDP. To evaluate its bandgap and birefringence, first-principles calculations are performed and the results reveal that γ-BBF possesses a large bandgap (about 8.56 eV), a moderate birefringence (0.105 at 532 nm), and a large SHG coefficient (d11 = 0.67 pm V−1). According to the refractive index dispersion equations, the calculated shortest phase matching edges for ABBF and γ-BBF are 173.9 nm and 146 nm, respectively. Therefore, both ABBF and γ-BBF can be used as new DUV NLO materials (Fig. 1).
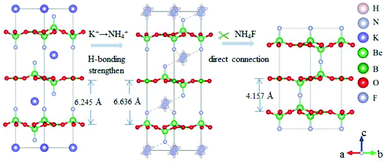 |
| Fig. 1 Structural evolution from KBBF, ABBF to γ-BBF. | |
Li4Sr(BO3)2 crystallizes in the monoclinic NCS space group Cc.38 As shown in Fig. 2, its structure is composed of 2D [SrBO3]∞ layers (Fig. 2b), which are further bridged by [BO3] units to construct the 3D framework of Li4Sr(BO3)2. Based on the grown single crystal (Fig. 2c), the UV cutoff edge is found to be 186 nm (corresponding to the bandgap of 6.69 eV). The powder SHG response is found to be 2 times that of KDP. According to the calculated results, Li4Sr(BO3)2 has a large SHG coefficient of 0.64 pm V−1 (d12) and a birefringence of 0.056 (at 532 nm), which agree well with the experimental results. Therefore, Li4Sr(BO3)2 is an attractive candidate for UV NLO applications.
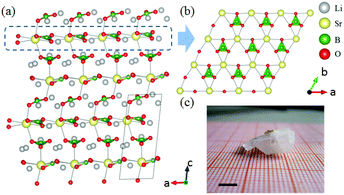 |
| Fig. 2 (a) The crystal structure, (b) the [SrBO3] layer, and (c) the as-grown crystal for Li4Sr(BO3)2. | |
NH4B4O6F is the first member of AB4O6F (A = NH4, Na, K, Rb, Cs) series NLO crystals.39–42 The compound crystallizes in the polar NCS space group Pna21. As shown in Fig. 3, three [BO3] and one [BO3F] connect together by sharing the O atoms to form the FBU of NH4B4O6F. These FBUs further construct the 2D [B4O6F]∞ layers extended along the a–c plane with the NH4+ cations inserted in the space between the layers. As shown by optical measurements, NH4B4O6F exhibits a wide DUV transparent range down to 156 nm (corresponding to the bandgap of 7.97 eV) and suitable birefringence (0.130 at 407 nm) that enable frequency doubling to 158 nm. The SHG response is found to be 2.5 times that of KDP. The calculated SHG coefficient (d33 = −1.19 pm V−1) and birefringence (0.117 at 1064 nm) show good agreement with the experimental results. All these properties clearly demonstrate that NH4B4O6F is an important NLO crystal for DUV applications.
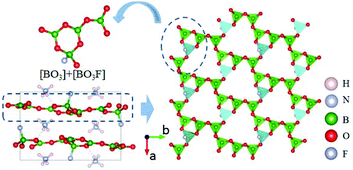 |
| Fig. 3 Crystal structural features of NH4B4O6F, whose FBU is composed of [BO3] and [BO3F]. | |
CsB4O6F also belongs to the AB4O6F (A = NH4, Na, K, Rb, Cs) series NLO crystals, but has a different structural type compared to NH4B4O6F.40 The compound crystallizes in the NCS space group Pna21. As shown in Fig. 4, the FBU of CsB4O6F consists of [B3O6] and [BO3F] units. These FBUs further connect together and construct the 2D [B4O6F]∞ layers of CsB4O6F. Optical measurements reveal that CsB4O6F exhibits a short cutoff edge (155 nm), a large SHG response (1.9 × KDP), and a suitable birefringence (0.127 at 400 nm) that enable frequency doubling down to 171.6 nm. The calculated SHG coefficient (d33 = 0.92 pm V−1) and birefringence (0.114 at 1064 nm) agree well with the experimental results. In addition, CsB4O6F possesses a more compact structure compared with KBBF, enabling the facile growth of large single crystals with a better growth habit.
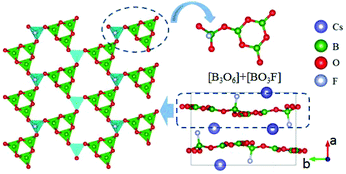 |
| Fig. 4 Crystal structural features of CsB4O6F, whose FBU is composed of [B3O6] and [BO3F]. | |
β-BaB2O4 (BBO) is a classic NLO crystal and has been widely used for laser frequency conversion.43 BBO crystallizes in the NCS space group R3c and its structure is shown in Fig. 5, which consists of isolated [B3O6] units and Ba cations. BBO possesses a large SHG coefficient of 1.6 pm V−1 (d22) and a large birefringence of 0.125 (at 404 nm) with a UV cutoff edge at 185 nm (corresponding to the bandgap of 6.72 eV). All these excellent properties guarantee its practical applications as a UV NLO crystal.
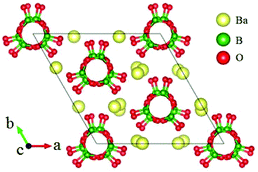 |
| Fig. 5 The crystal structure of β-BaB2O4. | |
2.2 DUV transparent carbonate with planar [CO3] units
KSrCO3F belongs to the ABCO3F (A = K, Rb, Cs; B = Mg, Ca, Sr, Ba) series NLO fluoride carbonates and crystallizes in the NCS space group P
m2.44–46 As shown in Fig. 6, its FBU consists of one [SrO6F2] polyhedron and three [CO3] units, which are connected together to form the 2D [SrCO3]∞ layer in the a–b plane as shown in Fig. 6b. These layers further construct the 3D framework of KSrCO3F by sharing the bridging F atoms with K atoms inserted in the space to balance the charges. Large, centimetre-sized single crystals of KSrCO3F have been grown by top seeded solution growth methods.47 Based on the obtained crystals, the main optical properties are determined. As per measurements, the UV absorption edge, birefringence and SHG coefficient are 195 nm (corresponding to the bandgap of 6.38 eV), 0.1117 (at 532 nm), and 0.50 pm V−1 (d22), respectively. Moreover, KSrCO3F possesses a large laser damage threshold (LDT) over 700 MW cm−2 under a 6 ns Nd:YAG laser operating at 15 Hz. All these properties indicate that KSrCO3F is an excellent UV NLO material.
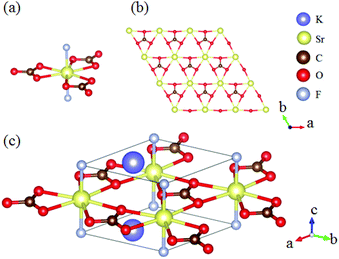 |
| Fig. 6 (a) The FBU, (b) [SrCO3] layer, and (c) the crystal structure of KSrCO3F. | |
Ca2Na3(CO3)3F crystallizes in the NCS space group Cm and its structure is shown in Fig. 7.48 Interestingly, the structure of Ca2Na3(CO3)3F can be described by the substitution of NLO-active [BO3] units for [CO3] units from YCa4O(BO3)3, which resulted in an optimal balance among the SHG coefficient, birefringence and UV transparency. As per measurements, Ca2Na3(CO3)3F has a large SHG response of 3 × KDP and a birefringence of 0.082 with the UV absorption edge located at 190 nm (corresponding to the bandgap of 6.53 eV). The calculated largest SHG coefficient and birefringence are about 0.5 pm V−1 (d12) and 0.072, respectively, which are in good agreement with the experimental results.
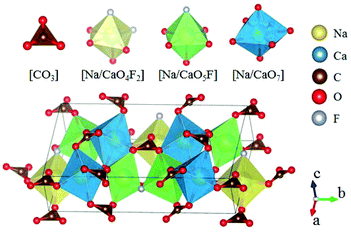 |
| Fig. 7 Crystallographic structure of Ca2Na3(CO3)3F. | |
2.3 DUV transparent nitrates with planar [NO3] units
Ba2(OH)3NO3 possesses a hexagonal NCS space group P
2m and its structure is shown in Fig. 8.49 In this compound, the Ba atoms are 9-fold coordinated with O atoms to form a [BaO3(OH)6] polyhedron, which is further connected with three [NO3] units to construct the 3D framework of Ba2(OH)3NO3. The optical measurements show that Ba2(OH)3NO3 has a large SHG response (about 4 times that of KDP) and a birefringence of 0.080 with the UV absorption edge shorter than 200 nm (corresponding to a bandgap of 6.22 eV). Theoretical calculations reveal that the SHG coefficient and birefringence of Ba2(OH)3NO3 are 2.41 pm V−1 and 0.082, respectively. More importantly, Ba2(OH)3NO3 exhibits good water-resistance, making this compound a promising UV NLO material.
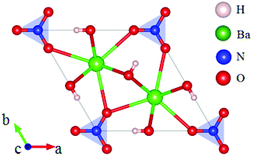 |
| Fig. 8 The crystal structure of Ba2(OH)3NO3. | |
Clearly, NLO borates, carbonates, and nitrates can show DUV transparency. For borates, the UV cutoff edges are about 180 nm, and those can be blue-shifted to 150 nm for fluorooxoborates. For carbonates, F ions must be introduced to achieve deep UV transmission. For nitrates, only two compounds, i.e. Ba2(OH)3NO3 and Sr2(OH)3NO3, are DUV transparent, while their UV cutoff edges are close to 200 nm. Currently, only borates can achieve the frequency conversion of DUV lasers, while carbonates and nitrates need to further reduce their phase matching wavelengths.
3. UV transparent NLO crystals containing planar π-conjugated groups
Besides the oxygen containing planar π-conjugated groups ([BO3], [B3O6], [CO3], [NO3], [HxC3N3O3]), [BS3] and [B3S6] can also exhibit transparency in the UV region. Meanwhile, the introduction of distorted polyhedra centered by SALP cations, rare-earth cation centered polyhedra, and metal element centered [MQ4] tetrahedra will affect their property of transparency. In this section, the related NLO crystals will be discussed.
3.1 UV transparent cyanurates with planar [HxC3N3O3] groups
LiCl(H3C3N3O3) crystallizes in the polar NCS space group R3m, as shown in Fig. 9.50,51 The structure is characterized by graphite-like quasi-2D infinite layers composed of [H3C3N3O3] units connected by a [LiO3Cl] tetrahedron (Fig. 9b and c). Interestingly, all the [H3C3N3O3] units are coplanar and parallel to one another, which is favorable for large SHG response. As per theoretical calculations, LiCl(H3C3N3O3) possesses a large SHG coefficient (d22 = 4.15 pm V−1) and strong optical anisotropy (Δn = 0.28 at 1064 nm) with a short UV transparent edge of 215 nm, thus showing an attractive prospect for future NLO applications in the solar-blind regions.
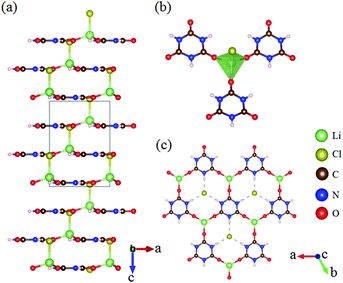 |
| Fig. 9 (a) The crystal structure, (b) FBU, and the [LiH3C3N3O3] layer of LiCl(H3C3N3O3). | |
β-Sr3(C3N3O3)2 crystallizes in the NCS space group R3c. Its crystal structure is shown in Fig. 10.28,52 β-Sr3(C3N3O3)2 is isostructural to the famous NLO crystal BBO, in which the [B3O6] is replaced by [C3N3O3]. As per measurements, β-Sr3(C3N3O3)2 has a larger powder SHG response than BBO. To provide a more detailed analysis, first-principles calculations were carried out and the results revealed that the compound has a large SHG coefficient (d22 = 3.93 pm V−1) and birefringence (0.36 at 1064 nm) with a UV cutoff edge of about 223 nm. All these optical properties clearly indicated that β-Sr3(C3N3O3)2 can serve as a UV NLO crystal.
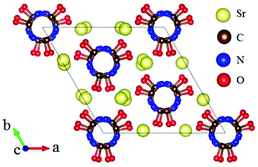 |
| Fig. 10 The crystal structure of β-Sr3(C3N3O3)2. | |
KLi(HC3N3O3)·2H2O crystallizes in the NCS space group Pna21 and its structure is shown in Fig. 11.53 In this compound, the hydro-isocyanurate [HC3N3O3] units connect with each other through [LiO4] to form a 1D chain, which further bridges via [HC3N3O3] units to construct an intricate 2D layer as shown in Fig. 11b. As per the determination of optical properties, KLi(HC3N3O3)·2H2O shows a large SHG response about 5.3 times that of KDP with a UV cutoff edge of 237 nm (corresponding to the bandgap of 5.23 eV). More importantly, a bulk single crystal with 10 mm × 15 mm × 6 mm in size was obtained. Based on the characterization of the single crystals, KLi(HC3N3O3)·2H2O exhibits a high LDT of 4.76 GW cm−2 and a large birefringence of 0.186 at 514 nm, which lead to the shortest phase-matching edge to 246 nm.
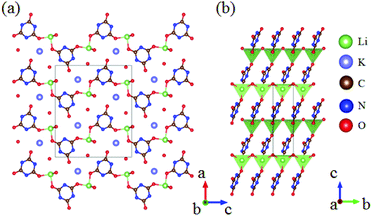 |
| Fig. 11 (a) Crystal structure of KLi(HC3N3O3)·2H2O; (b) layers of KLi(HC3N3O3)·2H2O consisting of (HC3N3O3) triangular units. | |
3.2 UV transparent borates with planar [BO3] units
RbZn2BO3Br2 belongs to the AZn2BO3X2 (A = K, Rb, NH4; X = Cl, Br) series NLO materials and only RbZn2BO3Br2 is discussed here.54 The framework of RbZn2BO3Br2 can be described by the tetrahedron substitution of [BeO3F] for [ZnO3Br] from KBBF as shown in Fig. 12. Optical measurements on RbZn2BO3Br2 show that this compound is phase-matchable with powder SHG response about 2.53 times that of KDP and its UV cutoff edge is located at 214 nm, corresponding to the bandgap of 5.79 eV. The first-principles calculations show that its largest SHG coefficient is 0.43 (d11) and reveal that the enhanced SHG response originates from the cooperative effect of coparallel [BO3] triangles and the distorted [ZnO3Br] tetrahedron. Moreover, this series of compounds exhibit a lighter layered growth habit than KBBF, which is beneficial for the research of crystal growth.
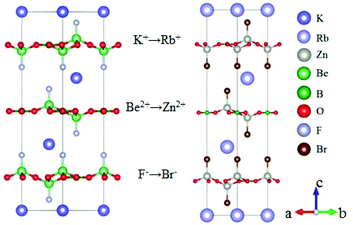 |
| Fig. 12 Structural evolution from KBBF to RbZn2BO3Br2. | |
Pb2BO3Cl crystallizes in the trigonal polar NCS space group P321 and exhibits a 2D layered structure as shown in Fig. 13.55 Pb2BO3Br and Pb2BO3I are isomorphic to this compound, which are not discussed here.56,57 As shown in Fig. 13b, the [BO3] units are parallelly arranged and connected with a neighbouring [PbO3] pyramid to construct the 2D infinite [PbBO3] layers in the a–b plane. Owing to the NLO favourable arrangement of [BO3], Pb2BO3Cl exhibits a large SHG response 9 times that of KDP. It also has a wide transparent window from the UV to mid-IR region with a bandgap of about 3.99 eV. The calculated largest SHG coefficient is about 7.2 pm V−1 (d11), which is larger than the experimental result. Its calculated birefringence is 0.12 at 1064 nm, which is in good agreement with the measured phase-matchability.
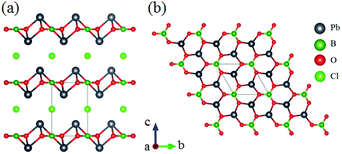 |
| Fig. 13 (a) The crystal structure and (b) the [PbBO3] layer of Pb2BO3Cl. | |
Cd4Y(BO3)3 belongs to the Cd4Re(BO3)3 (Re = Y, Gd, Lu) series NLO crystals and crystallizes in the monoclinic NCS space group Cm.58 There are three kinds of units ([BO3], [YO6], and [CdO6]) in this compound as shown in Fig. 14. In Cd4Y(BO3)3, these three kinds of units connect together by sharing corners and edges and construct the 3D framework. As per measurements, Cd4Y(BO3)3 has a moderate bandgap of 3.78 eV and a large SHG response 5.2 times that of KDP. Cd4Y(BO3)3 is also phase-matchable and has wide transparent regions ranging from UV to IR. The first-principles calculations also guaranteed the large SHG response of Cd4Y(BO3)3 (the calculated d12 = 4.99 pm V−1).
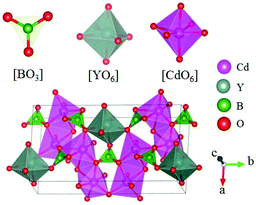 |
| Fig. 14 Crystal structure of Cd4Y(BO3)3. | |
3.3 UV transparent carbonate with planar [CO3] units
Na3Y(CO3)3 crystallizes in the NCS space group Ama2 and its structure is shown in Fig. 15.59 Its crystal structure can be described as alternate stackings of [Na(CO3)2]∞ and [Na2Y(CO3)2]∞ layers parallel to the b–c plane. SHG measurements show that Na3Y(CO3)3 has a large SHG response about 3.57 times that of KDP. It also exhibits wide transparent regions from UV to near IR with short UV cut-off edges at about 220 nm, corresponding to the bandgap of 5.59 eV. Theoretical calculation reveals that its largest SHG coefficient is 1.10 pm V−1 (d32). All the mentioned properties suggest that Na3Y(CO3)3 is a promising UV NLO crystal.
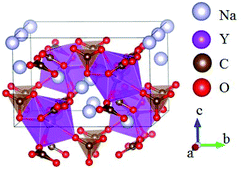 |
| Fig. 15 The crystal structure of Na3Y(CO3)3. | |
CsPbCO3F is isostructural to the aforementioned KSrCO3F. CsPbCO3F crystallizes in the NCS space group P
m2 and its structure is shown in Fig. 16.60 As per measurements, CsPbCO3F exhibits a smaller bandgap of 4.15 eV but a larger SHG response about 13.4 times that of KDP compared with KSrCO3F. The calculated SHG coefficient is about 5.1 pm V−1 (d22), which agrees well with the experimental result. As per the calculated results, it is the p–π interaction between Pb and [CO3] that resulted in the extremely large SHG efficiency.
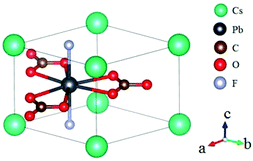 |
| Fig. 16 Crystallographic structure of CsPbCO3F. | |
KCdCO3F belongs to the ATCO3F (A = K, Rb; T = Zn, Cd) series NLO crystals and here only KCdCO3F is discussed.61 KCdCO3F crystallizes in the NCS space group P
c2 and its structure is shown in Fig. 17. In KCdCO3F, Cd is 5-fold coordinated with 3 O atoms and 2 F atoms and C atoms connect with O atoms to form [CO3] units. One [CdO3F2] connect with three [CO3] units and construct 2D layers, which are further linked by the bridging-F atoms to form the 3D framework of KCdCO3F. Interestingly, the [CO3] units in a certain layer are parallelly arranged but exhibit a rotation in two neighbouring layers. As per measurements, KCdCO3F possesses a large bandgap of 5.30 eV and a SHG response 4.58 times that of KDP. As per calculations, the SHG coefficient and birefringence are 1.99 pm V−1 (d22) and 0.11 at 1064 nm, respectively. The ATCO3F series NLO fluoride carbonates enrich the structural diversity of NLO carbonates and show great potential as UV NLO materials.
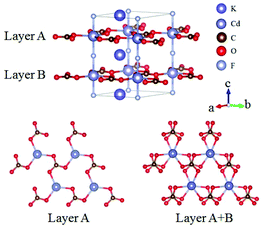 |
| Fig. 17 The crystal structure of KCdCO3F, in which the [CO3] units in neighbouring [CdCO3] layers exhibit a small rotation. | |
3.4 UV transparent nitrates with planar [NO3] units
Rb2Na(NO3)3 crystallizes in the orthorhombic polar NCS space group Pmc21, as shown in Fig. 18.62 In this compound, the Na atom is coordinated with 10 oxygen atoms, which are further connected with five [NO3] units to construct the FBUs. These FBUs connect together to form a 1D chain with the Rb atoms located between the chains. Rb2Na(NO3)3 possesses a large bandgap of about 4.74 eV and exhibits a large SHG response 5 times that of KDP. The calculated largest SHG coefficient (d31) is about 3.48 pm V−1, which agrees well with the experimental result.
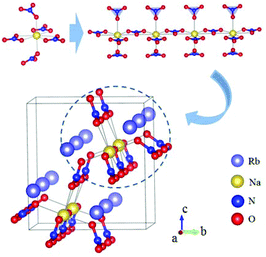 |
| Fig. 18 Crystallographic structure of Rb2Na(NO3)3. | |
Bi3TeO6OH(NO3)2 crystallizes in the NCS monoclinic space group P21 and its structure contains three kinds of NLO-active units, i.e. planar [NO3] units, distorted [TeO6] octahedron, and [Bi3O6H] groups with stereochemically active lone pair electrons, as shown in Fig. 19.63 In Bi3TeO6OH(NO3)2, the [TeO6] octahedron and [Bi3O6H] groups connect together to construct the 2D layers extended in the a–b plane with the [NO3] inserted between the layers. Due to the synergistic effects of these NLO-active units, Bi3TeO6OH(NO3)2 achieved a large SHG response about 3 times that of KDP. The measured bandgap of Bi3TeO6OH(NO3)2 is 3.59 eV, corresponding to the UV cutoff edge of 345 nm. Theoretical calculations reveal that the SHG coefficient and birefringence are d16 = −1.31 pm V−1 and 0.115 at 1064 nm, respectively. Interestingly, Bi3TeO6OH(NO3)2 is resistant to water, which provided an effective approach for practical nitrate NLO materials with high water-resistance.
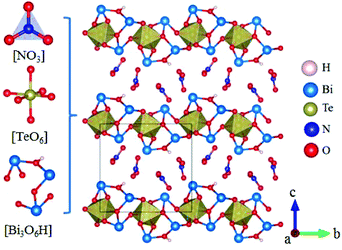 |
| Fig. 19 The crystal structure of Bi3TeO6OH(NO3)2. | |
3.5 UV transparent sulfides with planar [BS3] units
BaB2S4 crystallizes in the polar space group Cc and its structure is shown in Fig. 20.64 In BaB2S4, the planar [BS3] units and [BS4] tetrahedron are connected with each other by sharing corners to construct 1D infinite zigzag 1∞[B2S4] chains along the c-axis with 8-fold coordinated Ba cations located in the inter-chain. As per measurements, BaB2S4 exhibits a large bandgap of about 3.55 eV, due to which it can achieve a high LDT value during IR NLO applications, which can be preliminarily proven by powder LDT measurement (265 MW cm−2, 1064 nm, 10 ns, 10HZ). The powder SHG measurements show that the compound has a large SHG response about 0.7 times that of AgGaS2 with phase-matchability. The calculated largest SHG coefficient and birefringence are d12 = 8.22 pm V−1 and 0.11 at 2090 nm, respectively, which agree well with the experimental values. Moreover, BaB2S4 exhibits good air-stability, indicating its potential to become an IR NLO material.
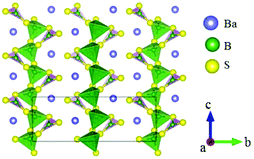 |
| Fig. 20 The crystal structure of BaB2S4. | |
LiBaB3S6 crystallizes in the NCS monoclinic space group Cc and its structure is shown in Fig. 21.20 As is shown, LiBaB3S6 contains [B3S6] anions formed by three corner-sharing [BS3] units with a six-membered [B3S3] ring inside. The metal cations are located between the anion units with Ba and Li 9- and 4-fold coordinated with sulfur atoms. Theoretical calculations demonstrate that LiBaB3S6 possesses a large bandgap (3.92 eV), thus indicating a high LDT value for mid-IR NLO applications. The birefringence is 0.343 at 1064 nm, which is favorable for achieving phase-matchability. However, the calculated SHG coefficients are rather small (d12 = 3.46 pm V−1) owing to the approximately centrosymmetric arrangement of [B3S6] units.
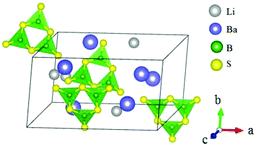 |
| Fig. 21 Crystallographic structure of LiBaB3S6. | |
As discussed above, the UV cutoff edges of NLO cyanurates are located at about 230 nm, indicating their applications in the solar-blind regions. The introduction of a polyhedron with SOJT and SALP cations will result in the red shift of the cutoff edges for borates, carbonates and nitrates. In addition, alkalis and alkaline-earth metals containing NLO sulphides with [BS3] and [B3S6] groups possess large bandgaps above 3.3 eV, which would lead to a large LDT for IR NLO applications.
4. VIS transparent NLO crystals containing planar π-conjugated groups
Ba3(BS3)(SbS3) crystallizes in the NCS space group P
2m and possesses a 0D structure constructed by isolated trigonal planar [BS3] units and discrete [SbS3] pyramids, as shown in Fig. 22.65 Based on the experimental measurements, Ba3(BS3)(SbS3) possesses a moderate bandgap of about 2.62 eV and is transparent in the range of 2.5–11 μm. The SHG response of Ba3(BS3)(SbS3) is three times as large as AgGaS2. The calculated largest NLO coefficient d21 is about 2.73 pm V−1 and the calculated birefringence is 0.05, which agree well with the powder SHG measurement.
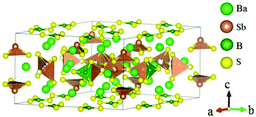 |
| Fig. 22 Crystallographic structure of Ba3(BS3)(SbS3). | |
A3VO(O2)2CO3 (A = K, Rb, Cs) is a new series of NLO carbonates. These three compounds are isostructural and crystallize in the NCS space group Cm.66–68 The structures are composed of distorted [VO(O2)2CO3] FBUs and charge balancing A+ cations as shown in Fig. 23. The measured bandgaps are 2.63 eV, 2.73 eV and 2.81 eV for the K-, Rb-, and Cs-containing compounds, respectively. Their powder SHG effects are 21, 20, and 23 times that of KDP, respectively. It should be noted that Cs2VO(O2)2CO3 showed the largest SHG response among the reported NLO carbonates. The calculated NLO coefficients and birefringence are listed in Table 1. As the theoretical calculations revealed, the [O2] anion groups and [CO3] in [VO(O2)2CO3] FBUs have made huge contributions to the NLO effects.
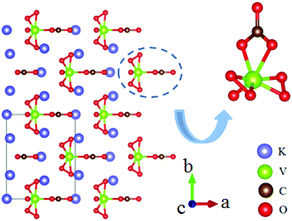 |
| Fig. 23 The crystal structure of A3VO(O2)2CO3. | |
Table 1 The main optical properties of the compounds containing the inorganic planar NLO-active groups
Compounds |
Space group |
Planar units |
Bandgap (eV) |
Exp. SHG response |
Largest SHG coefficient (pm V−1) |
Birefringence |
Ref. |
Calculated results by DFT methods.
|
DUV (Eg > 6.22 eV) |
γ-Be2BO3F |
R32 |
[BO3] |
8.56a |
2.3 × KDP |
d
11 = 0.67a |
0.105(@400 nm)a |
37
|
KBe2BO3F2 |
R32 |
[BO3] |
8.46 |
1.3 × KDP |
d
11 = 0.47 |
0.088(@400 nm) |
5,12
|
NH4Be2BO3F2 |
R32 |
[BO3] |
8.10 |
1.2 × KDP |
d
11 = 0.43 |
0.078(@400 nm) |
37
|
CsB4O6F |
Pna21 |
[B3O6] |
8.03 |
1.9 × KDP |
d
33 = 0.92a |
0.127(@400 nm) |
40
|
NH4B4O6F |
Pna21 |
[BO3] |
7.97 |
2.5 × KDP |
d
33 = −1.19a |
0.130(@407 nm) |
39
|
BaB2O4 |
R3c |
[B3O6] |
6.72 |
5.6 × KDP |
d
22 = 1.60 |
0.125(@404 nm) |
43
|
Li4Sr(BO3)2 |
Cc
|
[BO3] |
6.69 |
2 × KDP |
d
12 = 0.64a |
0.056(@532 nm)a |
38
|
Ca2Na3(CO3)3F |
Cc
|
[CO3] |
6.53 |
3 × KDP |
d
33 = 0.5a |
0.082(@589.6 nm) |
48
|
KSrCO3F |
P m2 |
[CO3] |
6.38 |
3.33 × KDP |
d
22 = 0.50 |
0.112(@532 nm) |
44,47
|
Ba2(OH)3NO3 |
P 2m |
[NO3] |
>6.22 |
4 × KDP |
d
22 = 2.41a |
0.080(@532 nm) |
49
|
UV (3.27 eV < Eg < 6.22 eV) |
RbZn2BO3Br2 |
R32 |
[BO3] |
5.79 |
2.53 × KDP |
d
11 = 0.43a |
— |
54
|
LiCl(H3C3N3O3) |
R3m |
[H3C3N3O3] |
5.76a |
— |
d
22 = 4.15a |
0.28(@800 nm)a |
50
|
Na3Y(CO3) |
Ama2 |
[CO3] |
5.59 |
3.57 × KDP |
d
32 = 1.10a |
— |
59
|
β-Sr3(C3N3O3)2 |
R3c |
[C3N3O3] |
5.57a |
>BBO |
d
22 = 3.93a |
0.36(@1064 nm)a |
28,52
|
KCdCO3F |
P c2 |
[CO3] |
5.30 |
4.58 × KDP |
d
22 = 1.99a |
0.11(@1064 nm)a |
61
|
KLi(HC3N3O3)·2H2O |
Pna21 |
[HC3N3O3] |
5.23 |
5.3 × KDP |
d
33 > 1.95 |
0.186(@514 nm) |
53
|
Rb2Na(NO3)3 |
Pmc21 |
[NO3] |
4.74 |
5 × KDP |
d
31 = 3.48 |
— |
62
|
CsPbCO3F |
P m2 |
[CO3] |
4.15 |
13.4 × KDP |
d
22 = 5.1a |
— |
60
|
Pb2BO3Cl |
P321 |
[BO3] |
3.99 |
9 × KDP |
d
11 = 7.2a |
0.12(@1064 nm)a |
55
|
LiBaB3S6 |
Cc
|
[B3S6] |
3.92a |
— |
d
12 = 3.46a |
0.343(@1064 nm)a |
20
|
Cd4YO(BO3)3 |
Cm
|
[BO3] |
3.78 |
5.2 × KDP |
d
12 = 4.99a |
|
58
|
Bi3TeO6OH(NO3)2 |
P21 |
[NO3] |
3.59 |
3 × KDP |
d
16 = −1.31a |
0.115(@1064 nm)a |
63
|
BaB2S4 |
Cc
|
[BS3] |
3.55 |
0.7 × AGS |
d
12 = 8.22a |
0.11(@2090 nm)a |
64
|
VIS (1.59 eV < Eg < 3.27 eV) |
Bi3TeBO9 |
P63 |
[BO3] |
3.23 |
20 × KDP |
d
33 = 5.1a |
— |
69
|
Cs3VO(O2)2CO3 |
Cm
|
[CO3] |
2.81 |
23 × KDP |
d
13 = 8.7a |
0.105(@1064 nm)a |
68
|
Rb3VO(O2)2CO3 |
Cm
|
[CO3] |
2.73 |
20 × KDP |
d
24 = 7.5 |
0.075(@1064 nm)a |
67
|
K3VO(O2)2CO3 |
Cm
|
[CO3] |
2.63 |
21 × KDP |
d
11 = 8.21 |
— |
66
|
Ba3(BS3)(SbS3) |
P 2m |
[BS3] |
2.62 |
3 × AGS |
d
21 = 2.73 |
0.050(static) |
65
|
IR (Eg < 1.59 eV) |
BaHgSe2 |
Pmc21 |
[HgSe3] |
1.56 |
1.5 × AGS |
d
33 = 39.87a |
0.147(@2090 nm)a |
70
|
Na2BP2 |
Pmc21 |
[B2P5] |
1.10 |
— |
d
12 = 57.14a |
0.68(@2090 nm)a |
73
|
Ag6HgSiSe6 |
Pmn21 |
[AgSe3] |
1.00 |
2 × AGS |
d
32 = 43.22a |
0.233(@2090 nm)a |
71
|
Ag6HgGeSe6 |
Pmn21 |
[AgSe3] |
0.92 |
0.5 × AGS |
d
32 = 21.36a |
0.207(@2090 nm)a |
71
|
Bi3TeBO9 crystallizes in the polar hexagonal space group P63 and its structure is shown in Fig. 24.69 This compound contains three kinds of NLO-active units, i.e. planar [BO3] units, a SOJT distorted [TeO6] octahedron, and a [BiO6] octahedron with stereochemically active lone pair electrons. These units connect with each other by sharing corners and edges to construct the 3D framework of Bi3TeBO9. Due to the synergistic effects of these units, Bi3TeBO9 has achieved the largest SHG responses 20 times that of KDP among the ever-reported borate NLO crystals. The bandgap of this compound is found to be 3.23 eV, which is located in the VIS region. In addition, theoretical calculations show that this compound has a larger NLO coefficient d33 of about 5.1 pm V−1 and reveal that such a large SHG response resulted from the synergistic effects of constituted NLO-active groups.
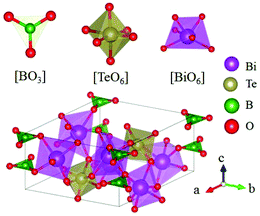 |
| Fig. 24 The crystal structure of Bi3TeBO9. | |
By introducing more than one bandgap-reducing units ([O2], [VOx] polyhedron, [TeO6] and [BiO6]), the UV transparent edges of borates and carbonates can be red shifted to the VIS region. Meanwhile, owing to the synergistic effects of multiple NLO-active units, the largest SHG responses of both NLO borates and carbonates are achieved, which will promote the exploration of materials with high laser frequency conversion efficiency in the visible spectrum region.
5. IR transparent NLO crystals containing planar π-conjugated groups
BaHgSe2 belongs to the orthorhombic NCS space group Pmc21 and its structure is shown in Fig. 25.70 In its structure, the Hg atoms have two different coordinated environments, where Hg1 atoms are coordinated to three Se atoms to form trigonal planar [HgSe3] units, while Hg2 atoms are bonded to two Se atoms to construct the [HgSe2] linear units. The [HgSe3] units connect with each other by sharing the bridging Se atoms and construct a 1D infinite chain along the a-axis, which will lead to large SHG responses. As per measurements, the bandgap of BaHgSe2 is about 1.56 eV and its SHG response is about 1.5 times that of AgGeS2. Moreover, the calculated largest SHG coefficient d33 is about 39.87 pm V−1 and the birefringence is 0.147 at 2090 nm. BaHgSe2 also exhibits good physicochemical stability, holding great promise for IR NLO applications.
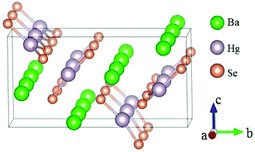 |
| Fig. 25 Crystallographic structure of BaHgSe2. | |
Ag6HgSiSe6 and Ag6HgGeSe6 possess similar structures and both of them crystallize in the NCS space group Pnm21, the FBUs and the structures of which are shown in Fig. 26 and 27, respectively.71 In Ag6HgSiSe6, [Ag/HgSe3] and [SiSe4] are connected alternately to form 1D [HgSiSe5] chains along the b-axis, which are further linked with each other's chains via [AgSe3] and [AgSe4] to construct the 3D framework of Ag6HgSiSe6. It should be noted that the planar [Ag/HgSe3] units are aligned completely parallel to each other in the 1D [HgSiSe5] chains, which would be the main origin of the large SHG responses of Ag6HgSiSe6. Ag6HgGeSe6 adopts a similar structure to that of Ag6HgSiSe6, but its [Ag/HgSe3] units degenerate into [Ag/HgSe2], thus reducing the second-order nonlinear polarizability of Ag6HgGeSe6. As per the measurements, the bandgaps of Ag6HgSiSe6 and Ag6HgGeSe6 are 1.00 eV and 0.92 eV, respectively. The measured powder SHG effects of these two compounds are 2 and 0.5 times that of AgGaS2, which agree well with the calculated SHG coefficients (d32 = 43.22 pm V−1 for Ag6HgSiSe6 and d32 = 21.36 pm V−1 for Ag6HgGeSe6). The calculated birefringence for Ag6HgSiSe6 and Ag6HgGeSe6 is 0.233 and 0.207 at 2090 nm, respectively. In addition, these two compounds are congruent-melting. All the mentioned features (such as strong SHG responses, valuable phase-matchable features, and congruent-melting thermal behaviour) make Ag6HgSiSe6 and Ag6HgGeSe6 promising IR NLO crystals for practical applications.
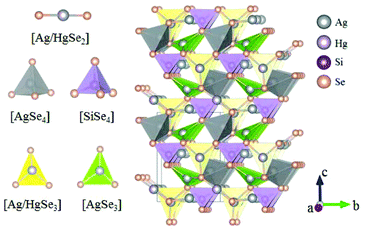 |
| Fig. 26 Crystallographic structure of Ag6HgSiSe6. | |
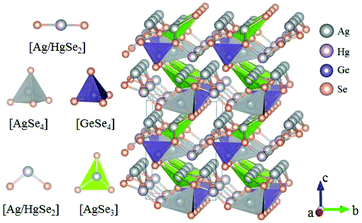 |
| Fig. 27 Crystallographic structure of Ag6HgGeSe6. | |
Na2BP2 was obtained by oxidative elimination reactions and it crystallizes in the Pmc21 space group (Fig. 28).72 In this compound, the B atoms are three coordinated with P atoms to construct the planar [BP3] triangles. Every two [BP3] units are bonded by a corner-sharing P atom and two bridging P atoms to form a [B2P5] 5-membered ring. These unique [B2P5] FBUs further connect with each other by sharing the exocyclic P atoms and construct a 1D infinite chain. All these chains are arranged along the a-axis with an inclined angle of ∼ 90° in the b–c plane between the adjacent chains. The bandgap of Na2BP2 is about 1.10 eV, indicating its shortwave cutoff edge located in the IR regions. Due to the absence of experimental data, the first principles calculations were carried out.73 As the results revealed, Na2BP2 possesses both the largest birefringence (Δn ∼ 0.68) and nonlinearity (d15 ∼ 57.14 pm V−1) among all known inorganic NLO materials containing planar NLO-active groups, which make [B2P5] a good NLO-active unit and inspire a new direction for designing high-performance NLO materials.
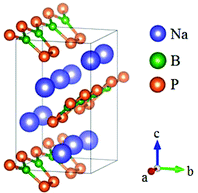 |
| Fig. 28 Crystallographic structure of Na2BP2. | |
In this section, IR NLO materials with planar [HgSe3], [AgSe3], and [B2P5] units are discussed. These crystals possess both huge SHG responses and birefringence, which make these units good candidates to achieve high conversion efficiency for IR NLO applications.
6. Conclusions
The main optical properties of the above-mentioned NLO materials containing inorganic planar NLO-active groups are listed in Table 1. All the mentioned NLO crystals exhibit large SHG responses and birefringence, indicating their potential in NLO applications. Specifically, the ordered arrangement of [BO3] units leads to the SHG response about 1.3 times that of KDP (KBBF), which could be enhanced to about 2 × KDP by modifying its density (AB4O6F series), and further enlarged to 20 × KDP (Bi3TeBO9) by introducing other NLO-active units. For NLO carbonates, the SHG responses are about 3 × KDP for the orderly arranged [CO3] units (KSrCO3F), which can further be enlarged to 23 × KDP (Cs3VO(O2)2CO3) by the synergistic effects with other kinds of NLO-active units. The orderly arranged [NO3] units lead to a response of about 4 × KDP, which is much larger than those of the [BO3] and [CO3] units. For the [HxC3N3O3] units, the SHG responses vary from 5 to 10 times that of KDP, which are dependent on their arrangement and density. For the no-oxygen-containing planar π-conjugated groups, the SHG responses are comparable to or even larger than that of AGS, which is suitable for IR NLO applications. In addition, most of the reported NLO materials containing inorganic planar NLO-active groups are phase-matchable, which indicated sufficient birefringence for practical applications.
To investigate the property of transparency of the planar π-conjugated groups, the distribution plots of the bandgaps of the involved NLO materials are drawn in Fig. 29. As is shown, only the bandgaps of [BO3] and [CO3] can cover the regions from VIS to DUV. [NO3] covers the UV and DUV regions, but its largest bandgap can only touch the DUV region, which makes NLO nitrates not suitable for DUV laser frequency conversion.27,74 The bandgaps of NLO cyanurates are mainly located at about 5.5 eV, which is beneficial for laser frequency conversion in the solar blind region. NLO materials containing [BS3] groups possess bandgaps covering the VIS and UV regions, which would lead to a large LDT for IR NLO applications. For the NLO compounds containing [MSe3] and [B2P5] units, the bandgaps are located in the IR region. Therefore, by summarising the property of transparency, the potential applied wave ranges of various planar π-conjugated groups are evaluated.
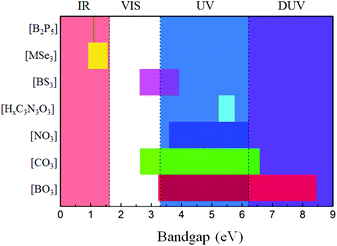 |
| Fig. 29 The distribution plot of bandgaps for the involved NLO materials with different planar π-conjugated groups. | |
In my opinion, inorganic planar NLO-active groups are one of the most desirable FBUs to construct NLO materials. Firstly, they possess large SHG responses and optical anisotropy, which are beneficial for NLO applications. Secondly, their bandgaps cover the whole wave ranges from DUV to IR, indicating that one can find suitable NLO materials with planar NLO-active groups for laser frequency conversion in a certain wave range. However, compared to the oxygen containing planar units, the kinds of no-oxygen containing planar units and the structural diversity of NLO compounds with these units are limited. For further development, NLO materials with new no-oxygen containing planar NLO-active groups should be considered.
Conflicts of interest
There are no conflicts to declare.
Acknowledgements
This work was supported by the NSFC (Grants No. 51802321, 51702330, and 51872297) and Fujian Innovation Academy (FJCXY18010201), CAS. ZL acknowledges the support from the Youth Innovation Promotion Association, Chinese Academy of Science.
Notes and references
- V. Petrov, Frequency down-conversion of solid-state laser sources to the mid-infrared spectral range using non-oxide nonlinear crystals, Prog. Quantum Electron., 2015, 42, 1–106 CrossRef
.
- M. Pushkarsky, A. Tsekoun, I. G. Dunayevskiy, R. Go and C. K. N. Patel, Sub-parts-per-billion level detection of NO2 using room-temperature quantum cascade lasers, Proc. Natl. Acad. Sci. U. S. A., 2006, 103, 10846–10849 CrossRef CAS PubMed
.
- N. Savage, Ultraviolet lasers, Nat. Photonics, 2007, 1, 83–85 CrossRef CAS
.
- N. Savage, Photodetectors, Nat. Photonics, 2007, 1, 730–731 CrossRef CAS
.
- C. T. Chen, G. L. Wang, X. Y. Wang and Z. Y. Xu, Deep-UV nonlinear optical crystal KBe2BO3F2-discovery, growth, optical properties and applications, Appl. Phys. B: Lasers Opt., 2009, 97, 9–25 CrossRef CAS
.
- D. Cyranoski, Materials science: China's crystal cache, Nature, 2009, 457, 953–955 CrossRef CAS PubMed
.
- Y. Zhou, G.-L. Wang, C.-M. Li, Q.-J. Peng, D.-F. Cui, Z.-Y. Xu, X.-Y. Wang, Y. Zhu, C.-T. Chen, G.-D. Liu, X.-L. Dong and X.-J. Zhou, Sixth harmonic of a Nd : YVO4 laser generation in KBBF for ARPES, Chin. Phys. Lett., 2008, 25, 963–965 CrossRef CAS
.
- C. T. Chen, B. C. Wu, A. D. Jiang and G. M. You, A new type ultraviolet SHG crystal: beta-BaB2O4, Sci. Sin., Ser. B, 1985, 28, 235–243 Search PubMed
.
- C. T. Chen, Y. C. Wu, A. D. Jiang, B. C. Wu, G. M. You, R. K. Li and S. J. Lin, New Nonlinear Optical Crystal: LiB3O5, J. Opt. Soc. Am. B, 1989, 6, 616–621 CrossRef CAS
.
- J. D. Bierlein and H. Vanherzeele, Potassium Titanyl Phosphat Properties and New Applications, J. Opt. Soc. Am. B, 1989, 6, 622–633 CrossRef CAS
.
- G. D. Boyd, H. Kasper and J. H. McFee, Linear and nonlinear optical properties of AgGaS2, CuGaS2, and CuInS2, and theory of wedge technique for measurement of nonlinear coefficients, IEEE J. Quantum Electron., 1971, QE 7, 563–573 CrossRef
.
-
C. Chen, T. Sasaki, R. Li, Y. Wu, Z. Lin, Y. Mori, Z. Hu, J. Wang, G. Aka, M. Yoshimura and Y. Kaneda, Nonlinear Optical Borate Crystals: Principals and Applications, Wiley-VCH press, Germany, 2012 Search PubMed
.
- Z. Lin, X. Jiang, L. Kang, P. Gong, S. Luo and M.-H. Lee, First-principles materials applications and design of nonlinear optical crystals, J. Phys. D: Appl. Phys., 2014, 47, 253001 CrossRef
.
- P. Gong, F. Liang, L. Kang, X. Chen, J. Qin, Y. Wu and Z. Lin, Recent advances and future perspectives on infrared nonlinear optical metal halides, Coord. Chem. Rev., 2019, 380, 83–102 CrossRef CAS
.
- L. Kang, M. Zhou, J. Yao, Z. Lin, Y. Wu and C. Chen, Metal Thiophosphates with Good Mid-infrared Nonlinear Optical Performances: A First-Principles Prediction and Analysis, J. Am. Chem. Soc., 2015, 137, 13049–13059 CrossRef CAS PubMed
.
- F. Liang, L. Kang, Z. Lin, Y. Wu and C. Chen, Analysis and prediction of mid-IR nonlinear optical metal sulfides with diamond-like structures, Coord. Chem. Rev., 2017, 333, 57–70 CrossRef CAS
.
- L. Kang, F. Liang, X. Jiang, Z. Lin and C. Chen, First-Principles Design and Simulations Promote the Development of Nonlinear Optical Crystals, Acc. Chem. Res., 2019 DOI:10.1021/acs.accounts.9b00448
.
- L. Kang, D. M. Ramo, Z. Lin, P. D. Bristowe, J. Qin and C. Chen, First principles selection and design of mid-IR nonlinear optical halide crystals, J. Mater. Chem. C, 2013, 1, 7363–7370 RSC
.
- W. Yao, R. He, X. Wang, Z. Lin and C. Chen, Analysis of Deep-UV Nonlinear Optical Borates: Approaching the End, Adv. Opt. Mater., 2014, 2, 411–417 CrossRef CAS
.
- F. Liang, L. Kang, Z. Lin and Y. Wu, Mid-Infrared Nonlinear Optical Materials Based on Metal Chalcogenides: Structure-Property Relationship, Cryst. Growth Des., 2017, 17, 2254–2289 CrossRef CAS
.
- K. M. Ok, P. S. Halasyamani, D. Casanova, M. Llunell, P. Alemany and S. Alvarez, Distortions in octahedrally coordinated d0 transition metal oxides: A continuous symmetry measures approach, Chem. Mater., 2006, 18, 3176–3183 CrossRef CAS
.
- R. He, Z. S. Lin, M. H. Lee and C. T. Chen, Ab initio studies on the mechanism for linear and nonlinear optical effects in YAl3(BO3)4, J. Appl. Phys., 2011, 109, 103510 CrossRef
.
- R. Cong, Y. Wang, L. Kang, Z. Zhou, Z. Lin and T. Yang, An outstanding second-harmonic generation material BiB2O4F: exploiting the electron-withdrawing ability of fluorine, Inorg. Chem. Front., 2015, 2, 170–176 RSC
.
- Y. Shen, S. Zhao and J. Luo, The role of cations in second-order nonlinear optical materials based on pi-conjugated [BO3]3− groups, Coord. Chem. Rev., 2018, 366, 1–28 CrossRef CAS
.
- G. Han, Y. Wang, B. Zhang and S. Pan, Fluorooxoborates: Ushering in a New Era of Deep Ultraviolet Nonlinear Optical Materials, Chem. – Eur. J., 2018, 24, 17638–17650 CrossRef CAS PubMed
.
- L. Kang, S. Luo, H. Huang, N. Ye, Z. Lin, J. Qin and C. Chen, Prospects for Fluoride Carbonate Nonlinear Optical Crystals in the UV and Deep-UV Regions, J. Phys. Chem. C, 2013, 117, 25684–25692 CrossRef CAS
.
- X. Liu, P. Gong, Y. Yang, G. Song and Z. Lin, Nitrate nonlinear optical crystals: A survey on structure-performance relationships, Coord. Chem. Rev., 2019, 400, 213045 CrossRef CAS
.
- F. Liang, L. Kang, X. Zhang, M.-H. Lee, Z. Lin and Y. Wu, Molecular Construction Using (C3N3O3)3− Anions: Analysis and Prospect for Inorganic Metal Cyanurates Nonlinear Optical Materials, Cryst. Growth Des., 2017, 17, 4015–4020 CrossRef CAS
.
- X. Meng, F. Liang, K. Kang, J. Tang, T. Zeng, Z. Lin and M. Xia, Facile Growth of an Ultraviolet Hydroisocyanurate Crystal with Strong Nonlinearity and a Wide Phase-Matching Region from π-Conjugated (HC3N3O3)2− Groups, Inorg. Chem., 2019, 58, 11289–11293 CrossRef CAS
.
- X. Meng, F. Liang, J. Tang, K. Kang, Q. Huang, W. Yin, Z. Lin and M. Xia, Cs3Na(H2C3N3O3)4·3H2O: A Mixed Alkali-Metal Hydroisocyanurate Nonlinear Optical Material Containing pi-Conjugated Six-Membered-Ring Units, Eur. J. Inorg. Chem., 2019, 2791–2795 CrossRef CAS
.
- M. Xia, M. Zhou, F. Liang, X. Meng, J. Yao, Z. Lin and R. Li, Noncentrosymmetric Cubic Cyanurate K6Cd3(C3N3O3)4 Containing Isolated Planar pi-Conjugated (C3N3O3)3− Groups, Inorg. Chem., 2018, 57, 32–36 CrossRef CAS PubMed
.
- J. Lu, Y.-K. Lian, L. Xiong, Q.-R. Wu, M. Zhao, K.-X. Shi, L. Chen and L.-M. Wu, How To Maximize Birefringence and Nonlinearity of π-Conjugated Cyanurates, J. Am. Chem. Soc., 2019, 141, 16151–16159 CrossRef CAS PubMed
.
- M. Mutailipu, M. Zhang, Z. Yang and S. Pan, Targeting the Next Generation of Deep-Ultraviolet Nonlinear Optical Materials: Expanding from Borates to Borate Fluorides to Fluorooxoborates, Acc. Chem. Res., 2019, 52, 791–801 CrossRef CAS PubMed
.
- C. Wu, G. Yang, M. G. Humphrey and C. Zhang, Recent advances in ultraviolet and deep-ultraviolet second-order nonlinear optical crystals, Coord. Chem. Rev., 2018, 375, 459–488 CrossRef CAS
.
- Y. Pan, S.-P. Guo, B.-W. Liu, H.-G. Xue and G.-C. Guo, Second-order nonlinear optical crystals with mixed anions, Coord. Chem. Rev., 2018, 374, 464–496 CrossRef CAS
.
- T. T. Tran, H. Yu, J. M. Rondinelli, K. R. Poeppelmeier and P. S. Halasyamani, Deep Ultraviolet Nonlinear Optical Materials, Chem. Mater., 2016, 28, 5238–5258 CrossRef CAS
.
- G. Peng, N. Ye, Z. Lin, L. Kang, S. Pan, M. Zhang, C. Lin, X. Long, M. Luo, Y. Chen, Y.-H. Tang, F. Xu and T. Yan, NH4Be2BO3F2 and γ-Be2BO3F: Overcoming the Layering Habit in KBe2BO3F2 for the Next-Generation Deep-Ultraviolet Nonlinear Optical Materials, Angew. Chem., Int. Ed., 2018, 57, 8968–8972 CrossRef CAS PubMed
.
- S. Zhao, P. Gong, L. Bai, X. Xu, S. Zhang, Z. Sun, Z. Lin, M. Hong, C. Chen and J. Luo, Beryllium-free Li4Sr(BO3)2 for deep-ultraviolet nonlinear optical applications, Nat. Commun., 2014, 5, 4019 CrossRef CAS PubMed
.
- G. Shi, Y. Wang, F. Zhan, B. Zhang, Z. Yang, X. Hou, S. Pan and K. R. Poeppelmeier, Finding the Next Deep-Ultraviolet Nonlinear Optical Material: NH4B4O6F, J. Am. Chem. Soc., 2017, 139, 10645–10648 CrossRef CAS PubMed
.
- X. Wang, Y. Wang, B. Zhang, F. Zhang, Z. Yang and S. Pan, CsB4O6F: ACongruent-Melting Deep-Ultraviolet Nonlinear Optical Material by Combining Superior Functional Units, Angew. Chem., Int. Ed., 2017, 56, 14119–14123 CrossRef CAS PubMed
.
- B. Zhang, G. Shi, Z. Yang, F. Zhang and S. Pan, Fluorooxoborates: Beryllium-Free Deep-Ultraviolet Nonlinear Optical Materials without Layered Growth, Angew. Chem., Int. Ed., 2017, 56, 3916–3919 CrossRef CAS PubMed
.
- Z. Zhang, Y. Wang, B. Zhang, Z. Yang and S. Pan, Polar Fluorooxoborate, NaB4O6F: A Promising Material for Ionic Conduction and Nonlinear Optics, Angew. Chem., Int. Ed, 2018, 57, 6577–6581 CrossRef CAS PubMed
.
- J. Lin, M. H. Lee, Z. P. Liu, C. T. Chen and C. J. Pickard, Mechanism for linear and nonlinear optical effects in β-BaB2O4 crystals, Phys. Rev. B: Condens. Matter Mater. Phys., 1999, 60, 13380–13389 CrossRef CAS
.
- G. Zou, N. Ye, L. Huang and X. Lin, Alkaline-Alkaline Earth Fluoride Carbonate Crystals ABCO3F (A = K, Rb, Cs; B = Ca, Sr, Ba) as Nonlinear Optical Materials, J. Am. Chem. Soc., 2011, 133, 20001–20007 CrossRef CAS PubMed
.
- T. T. Tran, J. He, J. M. Rondinelli and P. S. Halasyamani, RbMgCO3F: A New Beryllium-Free Deep-Ultraviolet Nonlinear Optical Material, J. Am. Chem. Soc., 2015, 137, 10504–10507 CrossRef CAS PubMed
.
- T. T. Tran, J. Young, J. M. Rondinelli and P. S. Halasyamani, Mixed-Metal Carbonate Fluorides as Deep-Ultraviolet Nonlinear Optical Materials, J. Am. Chem. Soc., 2017, 139, 1285–1295 CrossRef CAS
.
- W. Zhang and P. S. Halasyamani, Crystal growth and optical properties of a UV nonlinear optical material KSrCO3F, CrystEngComm, 2017, 19, 4742–4748 RSC
.
- M. Luo, Y. Song, C. Lin, N. Ye, W. Cheng and X. Long, Molecular Engineering as an Approach To Design a New Beryllium-Free Fluoride Carbonate as a Deep-Ultraviolet Nonlinear Optical
Material, Chem. Mater., 2016, 28, 2301–2307 CrossRef CAS
.
- X. Dong, L. Huang, Q. Liu, H. Zeng, Z. Lin, D. Xu and G. Zou, Perfect balance harmony in Ba2NO3(OH)3: a beryllium-free nitrate as a UV nonlinear optical material, Chem. Commun., 2018, 54, 5792–5795 RSC
.
- F. Liang, N. Wang, X. Liu, Z. Lin and Y. Wu, Co-crystal LiCl·(H3C3N3O3): a promising solar-blind nonlinear optical crystal with giant nonlinearity from coplanar π-conjugated groups, Chem. Commun., 2019, 55, 6257–6260 RSC
.
- O. Shemchuk, D. Braga, L. Maini and F. Grepioni, Anhydrous ionic co-crystals of cyanuric acid with LiCl and NaCl, CrystEngComm, 2017, 19, 1366–1369 RSC
.
- M. Kalmutzki, M. Stroebele, F. Wackenhut, A. J. Meixner and H. J. Meyer, Synthesis and SHG Properties of Two New Cyanurates: Sr3(O3C3N3)2 (SCY) and Eu3(O3C3N3)2 (ECY), Inorg. Chem., 2014, 53, 12540–12545 CrossRef CAS
.
- D. Lin, M. Luo, C. Lin, F. Xu and N. Ye, KLi(HC3N3O3)·2H2O: Solvent-drop Grinding Method toward the Hydro-isocyanurate Nonlinear Optical Crystal, J. Am. Chem. Soc., 2019, 141, 3390–3394 CrossRef CAS PubMed
.
- G. Yang, P. Gong, Z. Lin and N. Ye, AZn2BO3X2(A = K, Rb, NH4; X = Cl, Br): New Members of KBBF Family Exhibiting Large SHG Response and the Enhancement of Layer Interaction by Modified Structures, Chem. Mater., 2016, 28, 9122–9131 CrossRef CAS
.
- G. Zou, C. Lin, H. Jo, G. Nam, T.-S. You and K. M. Ok, Pb2BO3Cl: A Tailor-Made Polar Lead Borate Chloride with Very Strong Second Harmonic Generation, Angew. Chem., Int. Ed., 2016, 55, 12078–12082 CrossRef CAS PubMed
.
- M. Luo, Y. Song, F. Liang, N. Ye and Z. Lin, Pb2BO3Br: a novel nonlinear optical lead borate bromine with a KBBF-type structure exhibiting strong nonlinear optical response, Inorg. Chem. Front., 2018, 5, 916–921 RSC
.
- H. Yu, N. Z. Koocher, J. M. Rondinelli and P. S. Halasyamani, Pb2BO3I: A Borate Iodide with the Largest Second-Harmonic Generation (SHG) Response in the KBe2BO3F2 (KBBF) Family of Nonlinear Optical (NLO) Materials, Angew. Chem., Int. Ed., 2018, 57, 6100–6103 CrossRef CAS
.
- G. Zou, Z. Ma, K. Wu and N. Ye, Cadmium-rare earth oxyborates Cd4ReO(BO3)3 (Re = Y, Gd, Lu): congruently melting compounds with large SHG responses, J. Mater. Chem., 2012, 22, 19911–19918 RSC
.
- M. Luo, C. Lin, G. Zou, N. Ye and W. Cheng, Sodium-rare earth carbonates with shorite structure and large second harmonic generation response, CrystEngComm, 2014, 16, 4414–4421 RSC
.
- G. Zou, L. Huang, N. Ye, C. Lin, W. Cheng and H. Huang, CsPbCO3F: A Strong Second-Harmonic Generation Material Derived from Enhancement via p-pi Interaction, J. Am. Chem. Soc., 2013, 135, 18560–18566 CrossRef CAS
.
- G. Yang, G. Peng, N. Ye, J. Wang, M. Luo, T. Yan and Y. Zhou, Structural Modulation of Anionic Group Architectures by Cations to Optimize SHG Effects: A Facile Route to New NLO Materials in the ATCO3F (A = K, Rb; T = Zn, Cd) Series, Chem. Mater., 2015, 27, 7520–7530 CrossRef CAS
.
- G. Zou, C. Lin, H. G. Kim, H. Jo and K. M. Ok, Rb2Na(NO3)3: A Congruently Melting UV-NLO Crystal with a Very Strong Second-Harmonic Generation Response, Crystals, 2016, 6, 42 CrossRef
.
- S. Zhao, Y. Yang, Y. Shen, B. Zhao, L. Li, C. Ji, Z. Wu, D. Yuan, Z. Lin, M. Hong and J. Luo, Cooperation of Three Chromophores Generates
the Water-Resistant Nitrate Nonlinear Optical Material Bi3TeO6OH(NO3)2, Angew. Chem., Int. Ed., 2017, 56, 540–544 CrossRef CAS
.
- H. Li, G. Li, K. Wu, B. Zhang, Z. Yang and S. Pan, BaB2S4: An Efficient and Air-Stable Thioborate as Infrared Nonlinear Optical Material with High Laser Damage Threshold, Chem. Mater., 2018, 30, 7428–7432 CrossRef CAS
.
- Y.-Y. Li, B.-X. Li, G. Zhang, L.-J. Zhou, H. Lin, J.-N. Shen, C.-Y. Zhang, L. Chen and L.-M. Wu, Syntheses, Characterization, and Optical Properties of Centrosymmetric Ba3(BS3)1.5(MS3)0.5 and Noncentrosymmetric Ba3(BQ3)(SbQ3), Inorg. Chem., 2015, 54, 4761–4767 CrossRef CAS
.
- Y. Song, M. Luo, F. Liang, N. Ye and Z. Lin, The second-harmonic generation intensification derived from localization conjugated pi-orbitals in O22−, Chem. Commun., 2018, 54, 1445–1448 RSC
.
- G. Zou, H. Jo, S.-J. Lim, T.-S. You and K. M. Ok, Rb3VO(O2)2CO3: A Four-in-One Carbonatoperoxovanadate Exhibiting an Extremely Strong Second-Harmonic Generation Response, Angew. Chem., Int. Ed., 2018, 57, 8619–8622 CrossRef CAS
.
- G. Zou, Z. Lin, H. Zeng, H. Jo, S.-J. Lim, T.-S. You and K. M. Ok, Cs3VO(O2)2CO3: an exceptionally thermostable carbonatoperoxovanadate with an extremely large second-harmonic generation response, Chem. Sci., 2018, 9, 8957–8961 RSC
.
- M. Xia, X. Jiang, Z. Lin and R. Lit, “All-Three-in-One”: A New Bismuth-Tellurium-Borate Bi3TeBO9 Exhibiting Strong Second Harmonic Generation Response, J. Am. Chem. Soc., 2016, 138, 14190–14193 CrossRef CAS
.
- C. Li, W. Yin, P. Gong, X. Li, M. Zhou, A. Mar, Z. Lin, J. Yao, Y. Wu and C. Chen, Trigonal Planar [HgSe3]4− Unit: A New Kind of Basic Functional Group in IR Nonlinear Optical Materials with Large Susceptibility and Physicochemical Stability, J. Am. Chem. Soc., 2016, 138, 6135–6138 CrossRef CAS PubMed
.
- Z. Li, Y. Yang, Y. Guo, W. Xing, X. Luo, Z. Lin, J. Yao and Y. Wu, Broadening Frontiers of Infrared Nonlinear Optical Materials with π-Conjugated Trigonal-Planar Groups, Chem. Mater., 2019, 31, 1110–1117 CrossRef CAS
.
- K. E. Woo, J. Wang, J. Mark and K. Kovnir, Directing Boron-Phosphorus Bonds in Crystalline Solid: Oxidative Polymerization of P
B
P Monomers into 1D Chains, J. Am. Chem. Soc., 2019, 141, 13017–13021 CrossRef CAS PubMed
.
- P. Gong, S. Zhang, G. Song, X. Liu and Z. Lin, An Unprecedented Planar π-conjugated [B2P5]5− Group with Ultralarge Birefringence and Nonlinearity: an ab initio Study, Chem. Commun., 2020, 56, 643–646 RSC
.
- L. Huang, G. Zou, H. Cai, S. Wang, C. Lin and N. Ye, Sr2(OH)3NO3: the first nitrate as a deep UV nonlinear optical material with large SHG responses, J. Mater. Chem. C, 2015, 3, 5268–5274 RSC
.
|
This journal is © the Partner Organisations 2020 |
Click here to see how this site uses Cookies. View our privacy policy here.