DOI:
10.1039/C7SC04723A
(Edge Article)
Chem. Sci., 2018,
9, 754-759
Dual vicinal functionalisation of heterocycles via an interrupted Pummerer coupling/[3,3]-sigmatropic rearrangement cascade†
Received
1st November 2017
, Accepted 17th November 2017
First published on 17th November 2017
Abstract
A dual vicinal functionalisation cascade involving the union of heterocycles and allyl sulfoxides is described. In particular, the approach provides efficient one-step access to biologically relevant and synthetically important C3 thio, C2 carbo substituted indoles. The reaction operates under mild, metal free conditions and without directing groups, via an interrupted Pummerer coupling of activated allyl sulfoxides, generating allyl heteroaryl sulfonium salts that are predisposed to a charge accelerated [3,3]-sigmatropic rearrangement.
Introduction
Functionalised heterocycles constitute one of the most important families of molecules in chemistry. In particular, indoles are amongst the most common heterocyclic motifs found in biologically active compounds.1 Indoles that are substituted with sulfur at C3 and carbon at C2 display rich activity as biological probes,2 and in therapeutic areas such as atherosclerosis,3 HIV,4 and cancer5 amongst many others, and are also valuable building blocks for synthesis.6–8 Vicinal sulfur and carbon substitution has also proved to be of value in other heterocyclic systems (Scheme 1A).
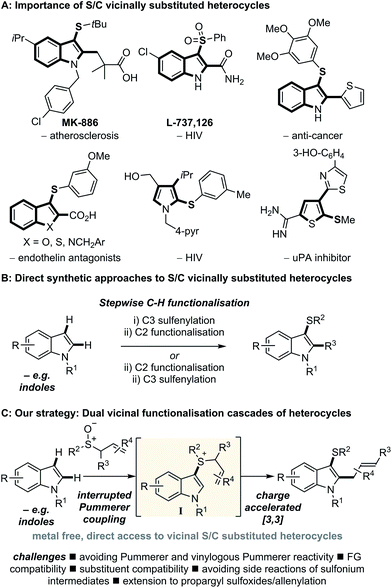 |
| Scheme 1 Vicinal S/C substituted heterocycles: important molecular architectures (A); stepwise C–H functionalisation strategy illustrated for indole (B); and, our approach utilising a dual vicinal functionalisation cascade, again, illustrated using indole. | |
Direct functionalisation of heterocycles is conceptually the most straightforward approach to decorated heterocycles and arguably the most attractive.9 For example, in considering indoles, the introduction of sulfur and carbon-containing groups by direct functionalisation9 has involved introduction of sulfur at C3 and a carbon-based group at C2 (or vice versa) in a stepwise fashion (Scheme 1B). Sulfenylation at C3 of indole is possible,10 however, direct regioselective introduction of a carbon based group at C2 requires highly basic organometallics11 or the use of expensive transition metals. Whilst transition metal catalysed regioselective C2 arylation, alkenylation12 and alkylation13 of indoles is possible, allylation at C2 requires superfluous directing groups.14 In addition, these stepwise approaches reduce overall process efficiency.15 An attractive alternative, direct functionalisation route to C3 thio, C2 carbo indoles would involve a cascade sequence, operating under mild and metal free conditions, in which the C3 sulfur and C2 carbo substituents are introduced in the same step via dual functionalisation of the readily accessible parent indole. However, a general process that constructs C3 thio, C2 carbo indoles in one synthetic operation from indoles was, until now, absent from the literature.
Herein, we report an efficient method for the construction of indoles bearing sulfur at C3 and a versatile allyl unit at C2, via dual vicinal functionalisation, enabled by an interrupted Pummerer coupling/[3,3]-sigmatropic rearrangement cascade (Scheme 1C).16,17 Importantly, the approach also extends to other important heterocycle classes. We employ readily accessible allylic sulfoxides that, once activated, are excellent coupling partners for the sulfenylation of various heterocycles. The generated heteroaryl–allyl sulfonium salt intermediates I are predisposed to a charge accelerated [3,3]-sigmatropic rearrangement and deliver useful allyl groups to heterocycles under metal free conditions. An analogous process uniting propargyl sulfoxides and indoles delivers sulfenylated/allenylated heterocycles.
Results and discussion
Dual vicinal functionalisation of indoles
We18 and others19 have previously shown that aryl allyl sulfonium salts, formed from aryl sulfoxides and allyl silanes, enable the ortho allylation of aryl sulfoxides.20,21 We postulated that, provided alternative Pummerer processes could be avoided, an interrupted Pummerer coupling22 of activated allyl sulfoxides with indoles, would allow direct access to indole–allyl sulfonium salts I that are predisposed to [3,3]-sigmatropic rearrangement and therefore C2 allylation, accomplishing the dual vicinal functionalisation cascade of indoles in one straightforward synthetic operation.23
We began our investigation with unprotected N–H indole 1a (R = R1 = H) and methyl allyl sulfoxide 2a (Scheme 2). Upon treatment of sulfoxide 2a with trifluoroacetic anhydride (TFAA) in the presence of indole (1a) and K3PO4, we were pleased to observe exclusive C3 sulfenylation. Addition of indole to the activated allyl sulfoxide exclusively occurred at sulfur and not at the α- or γ-carbons of the sulfoxonium salt (cf.II, Scheme 5) as might be expected in the classic and vinylogous Pummerer reactions.16 The subsequent [3,3]-sigmatropic rearrangement of the generated sulfonium salt (cf.I) was facile, and resulted in C2 allylation and formation of C3 thio, C2 allyl indole 3a in high yield (88%).
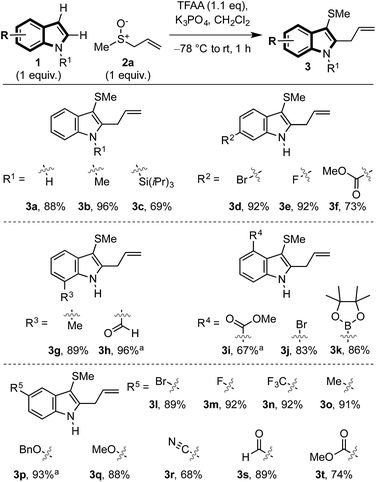 |
| Scheme 2 Exploring the scope of indoles in the dual vicinal functionalisation cascade. a 1.5 equivalents of sulfoxide and 1.6 equivalents of TFAA were used. | |
In exploring the scope of the reaction, we found that N-methyl- (3b) and the more hindered N-triisopropyl silyl indole (3c) were also compatible with the process. Indoles bearing substituents at all positions of the benzo ring were also amenable to cascade difunctionalisation (3d–t), including those bearing versatile substituents such as halides (3d,e,j,l,m), esters (3f,i,t), aldehydes (3h,s), trifluoromethyl (3n), ether (3p,q), nitrile (3r) and boronic ester (3k). In all cases, the expected products were obtained in good to excellent yield (67–96%).
We then examined the scope of allylic sulfoxide 2 in the dual functionalisation of indoles (Scheme 3). A variety of groups attached to sulfur of the allylic sulfoxide 2 were amenable to the process, such as alkyl (3b,u–w), including the more hindered isopropyl (3v), allyl (3w) and phenyl (3x). Interestingly, in the case of phenyl allyl sulfoxide (3x) where [3,3]-sigmatropic rearrangement of the intermediate sulfonium salt (cf.I) might result in allylation of either indole or phenyl rings, allylation occurred exclusively on the indole moiety.
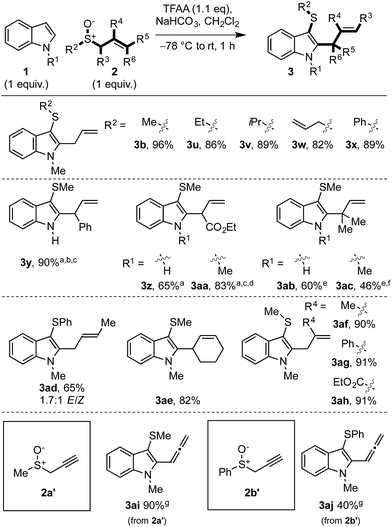 |
| Scheme 3 Exploring the scope of the allylic sulfoxide in the dual functionalisation cascade of indoles. a The corresponding E-allylic sulfoxides were used (i.e. R6 = H). b3y/4y = 4.8 : 1. c Yield determined by 1H-NMR. d3aa/4aa = 3 : 1. e Heated at 60 °C, 30 min. f3ac/4ac = 3.3 : 1. g Heated at 60 °C, 1 h. Regioisomer ratios determined from 1H-NMR spectra of the crude reaction mixture. | |
The substitution along the allyl portion of the allylic sulfoxide 2 was next investigated. When γ-substituted allyl sulfoxides were employed in the cascade, products 3y–ac were obtained in high yield. In the cases using N–Me indole enroute to 3aa and 3ac, a minor regioisomeric product, C2 thio C3 allyl indole 4, was also formed (see Scheme 5 and related discussion). Pleasingly, α- and β-substituted allyl sulfoxides gave exclusively the desired C3 thio, C2 allyl cascade products (cf.3ad–ah). Due to the mild conditions employed, internalisation of the alkene was not observed in these or any other cases. Finally, the use of propargyl sulfoxides in the cascade sequence, in place of allyl sulfoxides, delivered allenylated indoles 3ai and 3aj in 90% and 40% yield, respectively. Notably, previously attempted Pummerer-type allenylations using aryl and heteroaryl sulfoxides and allenylsilanes had proved unsuccessful.21h,i
Dual vicinal functionalisation of other aromatic heterocycles
We also explored the dual vicinal functionalisation cascade of other important aromatic heterocycles14a since other S/C vicinally substituted systems also display biological activity (Scheme 1A)24 and our preliminary results are presented in Scheme 4. A variety of heterocycles including thiophene (6a–g), furan (6h) and pyrrole (6i–l) scaffolds, bearing various functionalities, afforded the desired C2 thio C3 allyl heterocycles. The use of triflic anhydride to activate the sulfoxide was necessary in these cases. In comparison with indole, the inherent nucleophilicity of the C2 position switched the regiochemistry of the initial sulfenylation to C2. However, pyrroles used in the formation of 6k and 6l also gave C3 thio, C2 allyl regioisomers. Finally, benzothiophene and benzofuran also underwent dual vicinal functionalisation (formation of 6m and 6n). As expected, compared to the analogous reactions of indoles, the processes were less efficient and showed decreasing selectivity for C3 sulfenylation. In fact, dual functionalisation of benzofuran gave the C2 thio C3 allyl isomer 6n as the major product.25
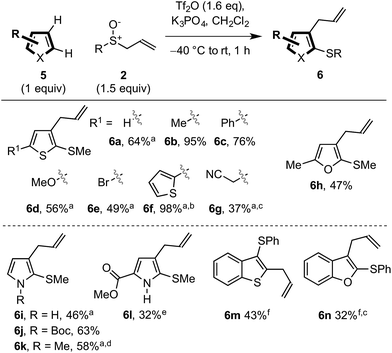 |
| Scheme 4 Preliminary results exploring other heterocycles in the dual vicinal functionalisation cascade. a using Na2CO3 instead of K3PO4. b 2 equivalents of sulfoxide and 2.2 equivalents of Tf2O were used. c Yield determined by 1H-NMR. d6k/C3 thio, C2 allyl pyrrole = 1 : 1.6. e6l/C3 thio, C2 allyl pyrrole = 1.5 : 1. f −78 °C to rt, overnight. | |
Proposed mechanism
Based on previous work18,19 and experimental observations, we propose the following mechanism for the dual functionalisation cascade (Scheme 5A). Activation of the allylic sulfoxide 2 with TFAA generates sulfoxonium salt II, which is trapped by indole through the inherently nucleophilic C3 position to form sulfonium salt I.22a–c Formation of sulfonium I is key to the prevention of over functionalisation since it is deactivated towards further electrophilic aromatic substitution, thus precluding further sulfenylation. In the case of sulfonium I generated enroute to 3z and 3aa, where the subsequent [3,3]-rearrangement was slow, a single C3 indolyl sulfonium product was observed by 1H-NMR (i.e. C2 sulfonium salts were not observed). Subsequent charge accelerated [3,3]-sigmatropic rearrangement17–21 in I accomplishes the second functionalisation event and delivers the desired C3 thio, C2 allyl indoles 3.
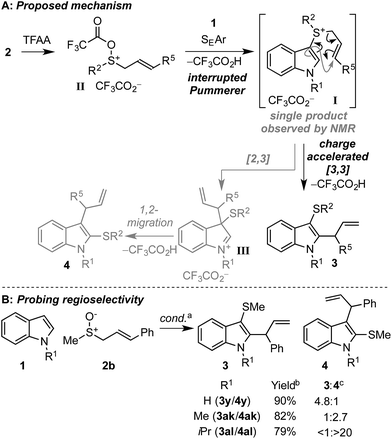 |
| Scheme 5 Proposed mechanism of the dual functionalisation cascade of indoles (A) and probing the effect of N-substitution for the selective generation of C3 thio, C2 allyl indole 3 or C2 thio, C3 allyl indole 4 (B). a Conditions as in Scheme 3. b Combined yield of 3 + 4. c Regioisomer ratios determined by 1H-NMR of the crude reaction mixture. | |
In some cases where γ-substituted allyl sulfoxides 2 were employed, the C2 thio, C3 allyl indoles 4 were also formed.26 We propose that in these cases, the [3,3]-sigmatropic rearrangement is disfavoured on steric grounds (vide infra) and instead a [2,3]-sigmatropic rearrangement of allyl sulfonium salt I27 forms intermediate III, which then undergoes facile sulfur migration to C2, likely via an episulfonium ion intermediate.28 Since C2 thio, C3 carbo indoles also have interesting biological activity, perhaps most notably in the amatoxins and phallotoxins found in several poisonous mushrooms,29 we further investigated this interesting facet of the method. Postulating that varying the sterics at nitrogen of indole would affect the relative rates of [3,3] and [2,3] rearrangements leading to different ratios of 3 and 4 respectively, we studied the reactivity of N–H, N–Me, and N–iPr indoles with γ-phenyl substituted allyl sulfoxide 2b (Scheme 5B). Pleasingly, when N–H indole 1a was employed, the selectivity for 3y was significantly increased (3y/4y = 4.8
:
1) relative to N–Me indole (3ak/4ak = 1
:
2.7). The trend of increased selectivity upon switching to the less sterically encumbered N–H indole 1a was also observed with γ-CO2Et and γ-dimethyl allyl sulfoxides, where the C3 thio C2 allyl regioisomers 3 were formed exclusively in both cases (cf.3zvs.3aa, and 3abvs.3ac, Scheme 3). Interestingly, upon utilising the more hindered N–iPr indole, C2 thio C3 allyl regioisomer 4al was obtained as the sole regioisomeric product.30
Scalability and iterative functionalisation
To further explore the synthetic capability of the dual functionalisation cascade products,31 we successfully accomplished gram scale reactions between diallyl sulfoxide (2c) and indoles 1a and 1b, which gave 3am (2.2 g) and 3w (1.7 g) in excellent yield (Scheme 6). In addition, products 3am and 3w were readily oxidised to indole–allyl sulfoxides 2d and 2e, which served as allyl sulfoxide units in the dual functionalisation cascade with other indoles to produce novel symmetrical (3an) and unsymmetrical bis indolyl sulfides (3ao, 3ap), a class of compounds that display antioxidant activity.32
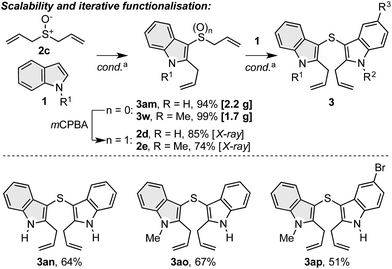 |
| Scheme 6 Scalability of the dual functionalisation cascade of indoles and iterative dual functionalisation cascades for the generation of novel bis indolyl sulfides. a Conditions as in Scheme 2. | |
Conclusions
In summary, we have described a dual vicinal functionalisation cascade of indoles that, without the need for superfluous directing groups or metals, provides efficient access to biologically relevant C3 thio, C2 carbo indoles under mild conditions. The process has also been applied to other heterocycles and to propargyl sulfoxide partners. The reaction operates via an interrupted Pummerer coupling between an activated sulfoxide partner and a heteroaromatic nucleophile, where the generated sulfonium salt formed then undergoes facile charge accelerated [3,3]-sigmatropic rearrangement and accomplishes the vicinal difunctionalisation of heterocycles in a single straightforward synthetic operation.
Conflicts of interest
There are no conflicts to declare.
Acknowledgements
We thank The University of Manchester (Lectureship to A. P. P.), EPSRC (PhD studentship to M. S. and Established Career Fellowship to D. J. P.), and Eli Lilly (PhD studentship to M. S.).
Notes and references
-
(a) V. Sharma, P. Kumar and D. Pathak, J. Heterocycl. Chem., 2010, 47, 491–502 CAS;
(b) N. A. Ali, B. A. Dar, V. Pradhan and M. Forooqui, Mini-Rev. Med. Chem., 2013, 13, 1792–1800 CrossRef PubMed.
-
(a) J.-I. Jun and L. F. Lau, Nat. Cell Biol., 2010, 12, 676–685 CrossRef CAS PubMed;
(b) J.-Y. Liu, J. Yang, B. Inceoglu, H. Qiu, A. Ulu, S.-H. Hwang, N. Chiamvimonvat and B. D. Hammock, Biochem. Pharmacol., 2010, 79, 880–887 CrossRef CAS PubMed.
- J. Jawien, M. Gajda, M. Rudling, L. Mateuszuk, R. Olszanecki, T. J. Guzik, T. Cichocki, S. Chlopicki and R. Korbut, Eur. J. Clin. Invest., 2006, 36, 141–146 CrossRef CAS PubMed.
-
(a) T. M. Williams,
et al.
, J. Med. Chem., 1993, 36, 1291–1294 CrossRef CAS PubMed;
(b) R. Silvestri,
et al.
, J. Med. Chem., 2003, 46, 2482–2493 CrossRef CAS PubMed;
(c) R. Ragno, M. Artico, G. D. Martino, G. L. Regina, A. Coluccia, A. D. Pasquali and R. Silvestri, J. Med. Chem., 2005, 48, 213–223 CrossRef CAS PubMed;
(d) R. Ragno,
et al.
, J. Med. Chem., 2006, 49, 3172–3184 CrossRef CAS PubMed;
(e) Z. Zhao,
et al.
, Bioorg. Med. Chem. Lett., 2008, 18, 554–559 CrossRef CAS PubMed;
(f) V. Famiglini,
et al.
, J. Med. Chem., 2014, 57, 9945–9957 CrossRef CAS PubMed.
-
(a) G. De Martino,
et al.
, J. Med. Chem., 2006, 49, 947–954 CrossRef CAS PubMed;
(b) G. La Regina,
et al.
, J. Med. Chem., 2011, 54, 8394–8406 CrossRef CAS PubMed;
(c) G. La Regina,
et al.
, J. Med. Chem., 2013, 56, 123–149 CrossRef CAS PubMed;
(d) Q. Guan,
et al.
, Eur. J. Med. Chem., 2014, 87, 306–315 CrossRef CAS PubMed;
(e) M. P. Fortes, P. B. N. da Silva, T. G. da Silva, T. S. Kaufman, G. C. G. Militão and C. C. Silveira, Eur. J. Med. Chem., 2016, 118, 21–26 CrossRef CAS PubMed.
-
(a) M. Nuth, H. Guan, N. Zhukovskaya, Y. L. Saw and R. P. Ricciardi, J. Med. Chem., 2013, 56, 3235–3246 CrossRef CAS PubMed;
(b) L. M. Miller,
et al.
, J. Med. Chem., 2017, 60, 722–748 CrossRef CAS PubMed;
(c) J. A. Campbell,
et al.
, Bioorg. Med. Chem. Lett., 2004, 14, 4741–4745 CrossRef CAS PubMed;
(d) Y. Lamotte, P. Martres, N. Faucher, A. Laroze, D. Grillot, N. Ancellin, Y. Saintillan, V. Beneton and R. T. Gampe Jr, Bioorg. Med. Chem. Lett., 2010, 20, 1399–1404 CrossRef CAS PubMed;
(e) S. Daly, K. Hayden, I. Malik, N. Porch, H. Tang, S. Rogelj, L. V. Frolova, K. Lepthien, A. Kornienko and I. V. Magedov, Bioorg. Med. Chem. Lett., 2011, 21, 4720–4723 CrossRef CAS PubMed;
(f) T. Luker,
et al.
, Bioorg. Med. Chem. Lett., 2011, 21, 6288–6292 CrossRef CAS PubMed;
(g) J. H. Hutchinson,
et al.
, J. Med. Chem., 1993, 36, 2771–2787 CrossRef CAS PubMed.
- For examples, see ref. 6b, e–g.
- P. G. Gassman, T. J. v. Bergen, D. P. Gilbert and B. W. Cue, J. Am. Chem. Soc., 1974, 96, 5495–5508 CrossRef CAS.
- For selected reviews, see:
(a) I. V. Seregin and V. Gevorgyan, Chem. Soc. Rev., 2007, 36, 1173–1193 RSC;
(b) J. C. Lewis, R. G. Bergman and J. A. Ellman, Acc. Chem. Res., 2008, 41, 1013–1025 CrossRef CAS PubMed. For recent reviews focusing on the C–H functionalisation of indoles, see:
(c) L. Ping, D. S. Chung, J. Bouffard and S.-g. Lee, Chem. Soc. Rev., 2017, 46, 4299–4328 RSC;
(d) J.-B. Chen and Y.-X. Jia, Org. Biomol. Chem., 2017, 15, 3550–3567 RSC;
(e) C. B. Bheeter, L. Chen, J.-F. Soulé and H. Doucet, Catal. Sci. Technol., 2016, 6, 2005–2049 RSC.
- For selected examples, see:
(a) H. Qi, T. Zhang, K. Wan and M. Luo, J. Org. Chem., 2016, 81, 4262–4268 CrossRef CAS PubMed;
(b) F.-L. Yang and S.-K. Tian, Angew. Chem., Int. Ed., 2013, 52, 4929–4932 CrossRef CAS PubMed;
(c) D. Huang, J. Chen, W. Dan, J. Ding, M. Liu and H. Wu, Adv. Synth. Catal., 2012, 354, 2123–2128 CrossRef CAS;
(d) M. Tudge, M. Tamiya, C. Savarin and G. R. Humphrey, Org. Lett., 2006, 8, 565–568 CrossRef CAS PubMed;
(e) F. Xiao, H. Xie, S. Liu and G.-J. Deng, Adv. Synth. Catal., 2014, 356, 364–368 CrossRef CAS;
(f) C. J. Nalbandian, E. M. Miller, S. T. Toenjes and J. L. Gustafson, Chem. Commun., 2017, 53, 1494–1497 RSC;
(g) S. Vásquez-Céspedes, A. Ferry, L. Candish and F. Glorius, Angew. Chem., Int. Ed., 2015, 54, 5772 CrossRef PubMed;
(h) S. Song, Y. Zhang, A. Yeerlan, B. Zhu, J. Liu and N. Jiao, Angew. Chem., Int. Ed., 2017, 56, 2487–2491 CrossRef CAS PubMed.
- J. A. Joule, Sci. Synth., Knowl. Updates, 2010, 2, 327–334 Search PubMed.
- See ref. 9a for selected examples of C2 C–H arylation, alkenylation and cyanation of indoles.
- For selected examples of C2 C–H alkylation of indoles, see:
(a) W.-W. Chan, S.-H. Yeung, Z. Zhou, A. S. C. Chan and W.-Y. Yu, Org. Lett., 2010, 12, 604–607 CrossRef CAS PubMed;
(b) L. Furst, B. S. Matsuura, J. M. R. Narayanam, J. W. Tucker and C. R. J. Stephenson, Org. Lett., 2010, 12, 3104–3107 CrossRef CAS PubMed;
(c) D.-H. Lee, K.-H. Kwon and C. S. Yi, Science, 2011, 333, 1613–1616 CrossRef CAS PubMed;
(d) L. Jiao and T. Bach, J. Am. Chem. Soc., 2011, 133, 12990–12993 CrossRef CAS PubMed;
(e) C. S. Sevov and J. F. Hartwig, J. Am. Chem. Soc., 2013, 135, 2116–2119 CrossRef CAS PubMed;
(f) Z.-Q. Liu and Z. Li, Chem. Commun., 2016, 52, 14278–14281 RSC.
- For a recent review, of aryl C–H allylation, see:
(a) N. K. Mishra, S. Sharma, J. Park, S. Han and I. S. Kim, ACS Catal., 2017, 7, 2821–2847 CrossRef CAS. For selected examples, see:
(b) D.-G. Yu, T. Gensch, F. de Azambuja, S. Vásquez-Céspedes and F. Glorius, J. Am. Chem. Soc., 2014, 136, 17722–17725 CrossRef CAS PubMed;
(c) Y. Suzuki, B. Sun, K. Sakata, T. Yoshino, S. Matsunaga and M. Kanai, Angew. Chem., Int. Ed., 2015, 54, 9944–9947 CrossRef CAS PubMed;
(d) W. Liu, S. C. Richter, Y. Zhang and L. Ackermann, Angew. Chem., Int. Ed., 2016, 55, 7747–7750 CrossRef CAS PubMed;
(e) L. Kong, S. Yu, G. Tang, H. Wang, X. Zhou and X. Li, Org. Lett., 2016, 18, 3802–3805 CrossRef CAS PubMed;
(f) G. S. Kumar and M. Kapur, Org. Lett., 2016, 18, 1112–1115 CrossRef CAS PubMed.
- A related strategy for the synthesis of C3 thio, C2 carbo substituted indoles involves heterocycle construction to give C2 substituted systems followed by C3 sulfenylation, however, this approach suffers from poor step economy. For examples, see: ref. 5e and 6c.
- For recent reviews of Pummerer chemistry, see:
(a) L. H. S. Smith, S. C. Coote, H. F. Sneddon and D. J. Procter, Angew. Chem., Int. Ed., 2010, 49, 5832–5844 CrossRef CAS PubMed;
(b) S. Akai and Y. Kita, Top. Curr. Chem., 2007, 274, 35–76 CrossRef CAS;
(c) K. S. Feldman, Tetrahedron, 2006, 62, 5003–5034 CrossRef CAS;
(d) S. K. Bur and A. Padwa, Chem. Rev., 2004, 104, 2401–2432 CrossRef CAS PubMed.
- For a review of charge accelerated [3,3]-sigmatropic rearrangements of sulfonium salts, see: X. Huang, S. Klimczyk and N. Maulide, Synthesis, 2012, 175–183 Search PubMed.
-
(a) A. J. Eberhart, J. E. Imbriglio and D. J. Procter, Org. Lett., 2011, 13, 5882–5885 CrossRef CAS PubMed;
(b) A. J. Eberhart, C. Cicoira and D. J. Procter, Org. Lett., 2013, 15, 3994–3997 CrossRef CAS PubMed.
- S. Yoshida, H. Yorimitsu and K. Oshima, Org. Lett., 2009, 11, 2185–2188 CrossRef CAS PubMed.
- For reviews of related reactions, see:
(a) A. P. Pulis and D. J. Procter, Angew. Chem., Int. Ed., 2016, 55, 9842–9860 CrossRef CAS PubMed;
(b) H. Yorimitsu, Chem. Rec., 2017, 17, 1156 CrossRef CAS PubMed;
(c) A. Shafir, Tetrahedron Lett., 2016, 57, 2673–2682 CrossRef CAS.
- For related reactions involving other sulfoxides and coupling partners, see:
(a) T. Kobatake, S. Yoshida, H. Yorimitsu and K. Oshima, Angew. Chem., Int. Ed., 2010, 49, 2340–2343 CrossRef CAS PubMed;
(b) X. Huang and N. Maulide, J. Am. Chem. Soc., 2011, 133, 8510–8513 CrossRef CAS PubMed;
(c) X. Huang, M. Patil, C. Farés, W. Thiel and N. Maulide, J. Am. Chem. Soc., 2013, 135, 7312–7323 CrossRef CAS PubMed;
(d) T. Yanagi, S. Otsuka, Y. Kasuga, K. Fujimoto, K. Murakami, K. Nogi, H. Yorimitsu and A. Osuka, J. Am. Chem. Soc., 2016, 138, 14582–14585 CrossRef CAS PubMed;
(e) B. Peng, D. Geerdink, C. Farès and N. Maulide, Angew. Chem., Int. Ed., 2014, 53, 5462–5466 CrossRef CAS PubMed;
(f) D. Kaldre, B. Maryasin, D. Kaiser, O. Gajsek, L. González and N. Maulide, Angew. Chem., Int. Ed., 2017, 56, 2212–2215 CrossRef CAS PubMed;
(g) L. Shang, Y. Chang, F. Luo, J.-N. He, X. Huang, L. Zhang, L. Kong, K. Li and B. Peng, J. Am. Chem. Soc., 2017, 139, 4211–4217 CrossRef CAS PubMed;
(h) A. J. Eberhart and D. J. Procter, Angew. Chem., Int. Ed., 2013, 52, 4008–4011 (
Angew. Chem.
, 2013
, 125
, 4100–4103
) CrossRef CAS PubMed;
(i) A. J. Eberhart, H. J. Shrives, E. Álvarez, A. Carrër, Y. Zhang and D. J. Procter, Chem.–Eur. J., 2015, 21, 7428–7434 CrossRef CAS PubMed;
(j) H. J. Shrives, J. Fernández-Salas, C. Hedtke, A. P. Pulis and D. J. Procter, Nat. Commun., 2017, 8, 14801 CrossRef PubMed;
(k) J. A. Fernández-Salas, A. J. Eberhart and D. J. Procter, J. Am. Chem. Soc., 2016, 138, 790–793 CrossRef PubMed;
(l) B. Peng, X. Huang, L.-G. Xie and N. Maulide, Angew. Chem., Int. Ed., 2014, 53, 8718–8721 CrossRef CAS PubMed.
- For reactions of aryl or alkyl sulfoxonium salts with indoles, see:
(a) K. Hartke and D. Strangemann, Heterocycles, 1986, 24, 2399–2402 CrossRef CAS;
(b) V. G. Nenaidenko and E. S. Balenkova, Russ. J. Org. Chem., 2003, 39, 291–330 CrossRef CAS;
(c) J. A. Fernández-Salas, A. P. Pulis and D. J. Procter, Chem. Commun., 2016, 52, 12364–12367 RSC. For recent examples with other aromatic nucleophiles:
(d) P. Cowper, Y. Jin, M. D. Turton, G. Kociok-Kçhn and S. E. Lewis, Angew. Chem., Int. Ed., 2016, 55, 2564–2568 CrossRef CAS PubMed;
(e) D. Chen, Q. Feng, Y. Yang, X.-M. Cai, F. Wang and S. Huang, Chem. Sci., 2017, 8, 1601–1606 RSC;
(f) X. Huang, R. Goddard and N. Maulide, Angew. Chem., Int. Ed., 2010, 49, 8979–8983 CrossRef CAS PubMed.
- For a related reaction involving allyl sulfides, see:
(a) K. Tomita, A. Terada and R. Tachikawa, Heterocycles, 1976, 4, 733 CrossRef CAS;
(b) B. W. Bycroft and W. Landon, Chem. Commun., 1970, 967–968 RSC.
- For examples, see:
(a) A. Maria Almerico, F. Mingoia, P. Diana, P. Barraja, A. Montalbano, A. Lauria, R. Loddo, L. Sanna, D. Delpiano, M. Giovanna Setzu and C. Musiu, Eur. J. Med. Chem., 2002, 37, 3–10 CrossRef;
(b) T. J. Blacklock, P. Sohar, J. W. Butcher, T. Lamanec and E. J. J. Grabowski, J. Org. Chem., 1993, 58, 1672–1679 CrossRef CAS;
(c) A. M. Bunker, J. J. Edmunds, K. A. Berryman, D. M. Walker, M. A. Flynn, K. M. Welch and A. M. Doherty, Bioorg. Med. Chem. Lett., 1996, 6, 1367–1370 CrossRef CAS;
(d) R. Di Santo, R. Costi, M. Artico, G. Miele, A. Lavecchia, E. Novellino, A. Bergamini, R. Cancio and G. Maga, ChemMedChem, 2006, 1, 1367–1378 CrossRef CAS PubMed;
(e) N. L. Subasinghe, C. Illig, J. Hoffman, M. J. Rudolph, K. J. Wilson, R. Soll, T. Randle, D. Green, F. Lewandowski, M. Zhang, R. Bone, J. Spurlino, R. DesJarlais, I. Deckman, C. J. Molloy, C. Manthey, Z. Zhou, C. Sharp, D. Maguire, C. Crysler and B. Grasberger, Bioorg. Med. Chem. Lett., 2001, 11, 1379–1382 CrossRef CAS PubMed.
- This is in line with a reported sulfenylation of benzofuran. See ref. 10g.
- Products 3 and 4 could be differentiated based on HMBC NMR experiments. See ESI for full details.†.
- For examples of [2,3]-rearrangements of related allyl sulfonium salts, see:
(a) B. B. Snider and L. Fuzesi, Tetrahedron Lett., 1978, 19, 877–880 CrossRef;
(b) V. V. Veselovsky, V. A. Dragan and A. M. Moiseenkov, Tetrahedron Lett., 1988, 29, 6637–6640 CrossRef;
(c) G. Hu, J. Xu and P. Li, Org. Lett., 2014, 16, 6036–6039 CrossRef CAS PubMed.
- For reviews of sulfur 1,2-migration and episulfonium ion chemistry, see:
(a) D. J. Fox, D. House and S. Warren, Angew. Chem., Int. Ed., 2002, 41, 2462–2482 CrossRef CAS;
(b) A. W. Sromek and V. Gevorgyan, Top. Curr. Chem., 2007, 274, 77–124 CrossRef CAS. For examples of sulfur migration in indoles via similar intermediates, see:
(c) R. Plate and H. C. J. Ottenheijm, Tetrahedron, 1986, 4511–4516 CrossRef CAS;
(d) P. Hamel, J. Org. Chem., 2002, 67, 2854–2858 CrossRef CAS PubMed;
(e) T. Hostier, V. Ferey, G. Ricci, D. G. Pardoa and J. Cossy, Chem. Commun., 2015, 51, 13898–13901 RSC.
- H. E. Hallen, H. Luo, J. S. Scott-Craig and J. D. Walton, Proc. Natl. Acad. Sci. U. S. A., 2007, 104, 19097–19101 CrossRef CAS PubMed.
- Allyl migration in intermediate III could also deliver typical products 3. However, the proposed mechanism is consistent with the high migratory aptitude of ‘SR’ and the tendency of sulfonium salt intermediates similar to I to undergo facile 3,3-sigmatropic rearrangements.
- For recent reviews of desulfanylative cross-coupling type reactions involving sulfides as pseudo halides, see:
(a) K. Gao, S. Otsuka, A. Baralle, K. Nogi, H. Yorimitsu and A. Osuka, J. Synth. Org. Chem., Jpn., 2016, 74, 1119–1127 CrossRef CAS;
(b) D. H. Ortgies, A. Hassanpour, F. Chen, S. Woo and P. Forgione, Eur. J. Org. Chem., 2016, 408–425 CrossRef CAS;
(c) F. Pana and Z.-J. Shia, ACS Catal., 2014, 4, 280–288 CrossRef;
(d) L. Wang, W. Hea and Z. Yu, Chem. Soc. Rev., 2013, 42, 599–621 RSC.
- C. C. Silveira, S. R. Mendes, J. R. Soares, F. N. Victoria, D. M. Martinez and L. Savegnago, Tetrahedron Lett., 2013, 54, 4926–4929 CrossRef CAS.
Footnote |
† Electronic supplementary information (ESI) available: Full experimental details, NMR spectra, CCDC numbers for X-ray structures. CCDC 1570504, 1570698. For ESI and crystallographic data in CIF or other electronic format see DOI: 10.1039/c7sc04723a |
|
This journal is © The Royal Society of Chemistry 2018 |
Click here to see how this site uses Cookies. View our privacy policy here.