DOI:
10.1039/C7QO00817A
(Research Article)
Org. Chem. Front., 2018,
5, 222-225
Facile synthesis of carbo- and heterocycles via Fe(III)-catalyzed alkene hydrofunctionalization†
Received
9th September 2017
, Accepted 30th September 2017
First published on 10th October 2017
Abstract
A facile synthesis of carbo- and heterocycles via Fe(III)-catalyzed alkene hydrofunctionalization has been developed. Various alkenes bearing a leaving group could convert to alkyl radicals through hydrogen atom transfer (HAT), and then serve as cyclization dipoles for accessing carbo- and heterocycles. The synthetic utility was further demonstrated by the synthesis of tetracyclic carbocycles from isopregol.
Introduction
In the past few decades, the transition-metal-catalyzed alkene hydrofunctionalization has been an effective and distinct approach for the formation of C–X (X = C, N, O, S, F) bonds (Scheme 1A).1 Halpern first revealed the convincing evidence of the radical mechanism for the Mn(III)-catalyzed hydrogenation of α-methylstyrene, and hydrogen atom transfer (HAT) was found to be involved in this process.2 Recently, Shenvi elucidated the HAT mechanism more clearly, and further applied this in the ipso-radical Smiles–Truce rearrangement and synergistic catalysis with nickel.3 Meanwhile, Carreira developed the Co(II)-catalyzed hydrofunctionalization of unactivated alkenes with electrophiles like TsCN, TsN3, TsCl, and sulfonyl oximes to forge C–C, C–N, and C–Cl bonds.4 Herzon and coworkers also developed the Co(II)-catalyzed hydrofunctionalization of alkenes and applied it to the modification of heterocycles.5 With respect to the Fe-catalysis, Mukaiyama reported the seminal work on alkene hydroamination to access amines.6 Recently, Boger reported the Fe-catalyzed alkene hydrocyanation and hydroamination protocol,7 and Baran established Fe-catalyzed alkene hydrofunctionalization through conjugate addition, hydroamination and hydromethylation.8 Our group investigated the Fe(III)-catalyzed alkene hydrostyrenylation, hydrohydrazonation and hydroalkylation methods.9 Notably, the utility of alkene hydrofunctionalization has also been demonstrated in the total synthesis of natural products, such as picrotoxinin, gibberellic acid, retenone, and quinine.10
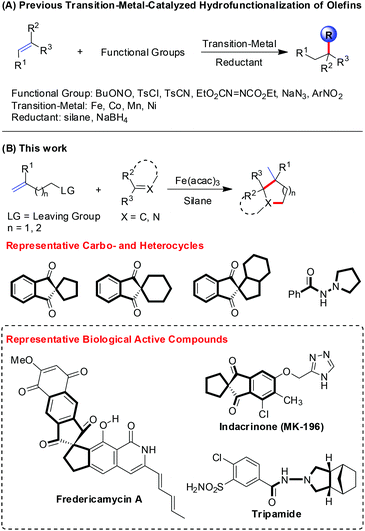 |
| Scheme 1 Fe-Catalyzed alkene hydrofunctionalization. | |
Saturated carbo- and heterocycles are important motifs in natural products and pharmaceuticals.11 Therefore, numerous methods have been developed for rapid access to these compounds. Pronin established Fe-catalyzed alkene hydrofunctionalization/polycyclization to construct a tricyclic core and further achieve the concise synthesis of paxilline indole diterpenes.12 Meanwhile, Maimone presented a four-step synthesis of the antimalarial cardamom peroxide via a crucial step of Mn(III)-catalyzed alkene hydroperoxidation.13 These indicated that radical alkene hydrofunctionalization is indeed a flexible and efficient strategy in the construction of saturated carbo- and heterocycles.
Pursuing our interests in the alkene hydrofunctionalization and heterocycle synthesis,14 we hypothesized that the alkenes bearing a leaving group could probably act as potential cyclization components, since the alkenes could convert to nucleophilic alkyl radicals in the presence of a Fe(III)-catalyzed HAT system, and the presence of an innate leaving group would constitute itself to be a cyclization dipole. Herein, we would like to report a facile synthesis of carbo- and heterocycles via the Fe(III)-catalyzed hydrofunctionalization of alkenes (Scheme 1B).
Results and discussion
We commenced our study by investigating 2-benzylidene-1H-indene-1,3(2H)-dione (1a) and 3-methylbut-3-en-1-yl-4-methylbenzenesulfonate (2a). Initially, we carried out the reaction using Fe(acac)3 as the catalyst and PhSiH3 as the reductant in ethanol at 60 °C (Table 1, entry 1). Gratifyingly, a cyclized indanedione-fused spirocyclopentane product 3a was observed and isolated in 16% yield, and the low yield was probably due to the poor solubility of 1a in EtOH. This encouraged us to further optimize the reaction conditions. When the more lipophilic 4-bromo-2-methylbut-1-ene (2b) was used, the reaction afforded 2a in 66% yield (entry 2). When the solvent was changed to THF and 2 equivalents of ethanol were added, we were pleased to find that the yield of 3a could be dramatically improved to 82% (entry 3). Moreover, when the reaction was subjected to Boger's conditions at room temperature, the reaction was shut down and no product was observed (entry 4). The silane survey showed that EtSiH3 and (EtO)2MeSiH were inferior (entries 5 and 6). In addition, either increasing the amount of Fe(acac)3 to 1 equivalent or decreasing to 10% equivalent would diminish the yield (entries 7–9). Surprisingly, when the amount of PhSiH3 was increased, the yield was reduced to 46% (entry 10), while decreasing the temperature to 40 °C would also lead to a lower yield (entry 11).
Table 1 Reaction optimizationa
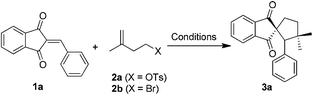
|
Entry |
Fe(III) |
Silanes |
Solvent |
T
|
Yieldb (%) |
Reaction conditions: 1a (0.2 mmol), 2 (0.4 mmol), Fe(III) (30 mol%), silane (0.2 mmol), EtOH (0.4 mmol) in 2 mL solvent under argon for 6 h.
Isolated yields are given.
2a (0.4 mmol).
2b (0.4 mmol).
Fe(III) (10 mol%).
Fe(III) (50 mol%).
Fe(III) (100 mol%).
Silane (0.4 mmol).
|
1c |
Fe(acac)3 |
PhSiH3 |
EtOH |
60 |
16 |
2d |
Fe(acac)3 |
PhSiH3 |
EtOH |
60 |
66 |
3d |
Fe(acac)3 |
PhSiH3 |
THF |
60 |
82 |
4d |
Fe2ox3·6H2O |
NaBH4 |
EtOH |
r.t. |
— |
5d |
Fe(acac)3 |
Et3SiH |
THF |
60 |
— |
6d |
Fe(acac)3 |
(EtO)2MeSiH |
THF |
60 |
— |
7d,e |
Fe(acac)3 |
PhSiH3 |
THF |
60 |
39 |
8d,f |
Fe(acac)3 |
PhSiH3 |
THF |
60 |
80 |
9d,g |
Fe(acac)3 |
PhSiH3 |
THF |
60 |
69 |
10d,h |
Fe(acac)3 |
PhSiH3 |
THF |
60 |
46 |
11d |
Fe(acac)3 |
PhSiH3 |
THF |
40 |
34 |
With the optimized reaction conditions in hand, we set out to explore the substrate scope of this transformation (Table 2). Various substituted 2-arylidene-1H-indene-1,3(2H)-diones, including both the electron-withdrawing and electron-donating substituents in the aromatic ring, such as methyl, methoxy, halogen, cyano, trifluoromethyl, dimethylamino, ester, methoxy, and cyano, were well tolerated under these conditions to furnish the spirocycles in moderate to good yields (3a–3k), and the structure of 3b was confirmed by X-ray analysis.15 Moreover, the polysubstituted 2-arylidene-1H-indene-1,3(2H)-dione was also well applicable in this protocol to deliver the spirocyclic ketone in moderate yield (3l). Interestingly, 1-naphthanyl and heterocycles containing unsaturated diones, like furan and thiophene, were amenable to this protocol to generate the corresponding spirocyclic products in moderate to good yields (3m–3o). Next, a variety of bromoalkenes were tested to react with 2-arylidene-1H-indene-1,3(2H)-diones (Table 2). Both mono-substituted terminal alkenes and disubstituted terminal alkenes could engage in this process to furnish indanedione-fused spirocyclohexanes and spirocyclopentanes in moderate to good yields. For example, when 4-bromobut-1-ene (2c) was utilized in this cyclization, the process could afford the products (3p and 3q) in moderate yields with 1
:
1 dr value. The cyclization of 5-bromopent-1-ene (2d) and 5-bromo-2-methylpent-1-ene (2e) could also lead to the formation of spiro[cyclohexane-1,2′-indene]-1′,3′-diones (3r and 3s). Interestingly, the formation of 3r was diastereoselective, and the coupling constants of the two hydrogens placed in the two stereocenters were about 11.6 Hz, thus indicating that the hydrogens were antarafacial. Therefore, this method provides a rapid and distinct approach to these indanedione-fused carbocycles from readily available starting materials.
Table 2 Synthesis of carbocyclesa
Standard conditions: 1 (0.2 mmol), 2 (0.4 mmol), Fe(acac)3 (30 mol%), PhSiH3 (0.2 mmol), EtOH (0.4 mmol) in 2 mL THF under argon for 6 h. Yields refer to isolated products; dr was identified by 1H NMR analysis.
|
|
Next, the synthetic utility was further applied to the synthesis of saturated carbocycles from isopregol (Scheme 2). Isopregol is a type of homoallylic alcohol natural product and can be easily converted to the cyclization precursor 2f. Under the standard conditions, various 2-arylidene-1H-indene-1,3(2H)-diones could participate in the cyclization with 2f to furnish the saturated tetracyclic carbocycles 4 in moderate yields. The dr value ranged from 1
:
1 to 1.13
:
1, and the structure of 4a was confirmed by X-ray analysis.16 The spirocyclic 1,3-indanedione derivatives represented privileged structural motifs in biological study. For example, fredericamycin A,17 indacrinone (MK-196)18 and tripamide19 were reported as antitumor, uricosuric-diuretic and antihypertensive compounds, respectively (Scheme 1C). Moreover, this Fe-catalyzed alkene hydrofunctionalization scope was also extended to the synthesis of heterocycles. For example, benzoyl hydrazine was generated in situ from benzoyl hydrazide and formaldehyde, and then used as a radical acceptor to cyclize with alkenes. Therefore, the 4-bromobut-1-ene (2c) and 5-bromopent-1-ene (2d) could be easily functionalized with this benzoyl hydrazine to furnish 1-aminopyrroline and 1-aminopiperidine derivatives in moderate to good yields (Scheme 2). These heterocycles represent important structural motifs in biologically interesting compounds. Therefore, this protocol provides a simple and direct access for the construction of these compounds.
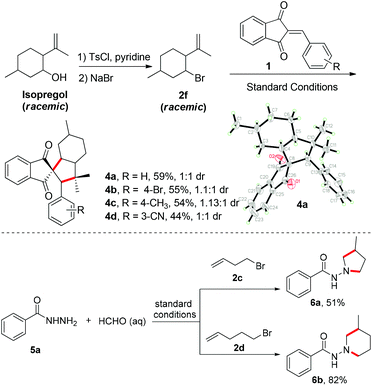 |
| Scheme 2 Synthesis of tetracyclic carbocycles and heterocycles. | |
Based on these results and the literature,5,8 a plausible mechanism is proposed in Fig. 1. At the beginning, Fe(III) is converted to Fe hydride species A upon the treatment of phenylsilane and ethanol. Then A undergoes hydrogen atom transfer (HAT) to alkene 1 to form Fe(II) species B and alkyl radical C. The addition of C to radical acceptor 1 would afford intermediate D, and the single-electron transfer between B and D delivers E and regenerates the Fe(III) catalyst. The final intramolecular nucleophilic reaction would furnish the carbocycles. With respect to the synthesis of heterocycles, the process was assumed to invoke the same mechanism.
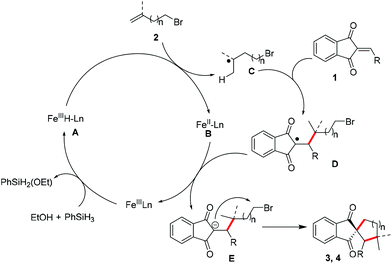 |
| Fig. 1 Proposed mechanism. | |
In summary, a Fe(III)-catalyzed alkene hydrofunctionalization for the synthesis of saturated carbo- and heterocycles has been developed. Various alkenes could cyclize with radical acceptors for the rapid assembly of carbo- and heterocycles with the features of mild reaction conditions, broad substrate scope and high efficiency.
Conflicts of interest
There are no conflicts to declare.
Acknowledgements
We are grateful for the financial support from the National Natural Science Foundation of China (no. 21472163).
Notes and references
-
(a) T. E. Müller, K. C. Hultzsch, M. Yus, F. Foubelo and M. Tada, Chem. Rev., 2008, 108, 3795 CrossRef PubMed;
(b) M. Villa and A. Jacobi von Wangelin, Angew. Chem., Int. Ed., 2015, 54, 11906 CrossRef CAS PubMed;
(c) S. W. M. Crossley, C. Obradors, R. M. Martinez and R. A. Shenvi, Chem. Rev., 2016, 116, 8912 CrossRef CAS PubMed;
(d) X. S. Ma and S. B. Herzon, Chem. Sci., 2015, 6, 6250 RSC;
(e) J. Streuff and A. Gansäuer, Angew. Chem., Int. Ed., 2015, 54, 2 CrossRef PubMed.
- R. L. Sweany and J. Halpern, J. Am. Chem. Soc., 1977, 99, 8335 CrossRef CAS.
-
(a) S. W. Crossley, R. M. Martinez, S. Guevara-Zuluaga and R. A. Shenvi, Org. Lett., 2016, 18, 2620 CrossRef CAS PubMed;
(b) S. A. Green, J. L. M. Matos, A. Yagi and R. A. Shenvi, J. Am. Chem. Soc., 2016, 138, 12779 CrossRef CAS PubMed.
-
(a) J. Waser, H. Nambu and E. M. Carreira, J. Am. Chem. Soc., 2005, 127, 8294 CrossRef CAS PubMed;
(b) J. Waser, B. Gaspar, H. Nambu and E. M. Carreira, J. Am. Chem. Soc., 2006, 128, 11693 CrossRef CAS PubMed;
(c) B. Gaspar and E. M. Carreira, Angew. Chem., Int. Ed., 2007, 46, 4519 CrossRef CAS PubMed;
(d) B. Gaspar and E. M. Carreira, Angew. Chem., Int. Ed., 2008, 47, 5758 CrossRef CAS PubMed;
(e) B. Gaspar and E. M. Carreira, Am. Chem. Soc., 2009, 131, 13214 CrossRef CAS PubMed.
-
(a) X. Ma and S. B. Herzon, Chem. Sci., 2015, 6, 6250 RSC;
(b) X. Ma and S. B. Herzon, J. Org. Chem., 2016, 81, 8673 CrossRef CAS PubMed;
(c) X. Ma and S. B. Herzon, J. Am. Chem. Soc., 2016, 138, 8718 CrossRef CAS PubMed;
(d) X. Ma, H. Dang, J. A. Rose, P. Rablen and S. B. Herzon, J. Am. Chem. Soc., 2017, 139, 5998 CrossRef CAS PubMed.
-
(a) K. Kato and T. Mukaiyama, Chem. Lett., 1992, 1137 CrossRef CAS;
(b) T. Mukaiyama, S. Isayama, S. Inoki, K. Kato, T. Yamada and T. Takai, Chem. Lett., 1989, 449 CrossRef CAS.
-
(a) T. J. Barker and D. L. Boger, J. Am. Chem. Soc., 2012, 134, 13588 CrossRef CAS PubMed;
(b) E. K. Leggans, T. J. Barker, K. K. Duncan and D. L. Boger, Org. Lett., 2012, 14, 1428 CrossRef CAS PubMed.
-
(a) J. Gui, C.-M. Pan, Y. Jin, T. Qin, J. C. Lo, B. J. Lee, S. H. Spergel, M. E. Mertzman, W. J. Pitts, T. E. L. Cruz, M. A. Schmidt, N. Darvatkar, S. R. Natarajan and P. S. Baran, Science, 2015, 348, 886 CrossRef CAS PubMed;
(b) J. C. Lo, Y. Yabe and P. S. Baran, J. Am. Chem. Soc., 2014, 136, 1304 CrossRef CAS PubMed;
(c) J. C. Lo, J. Gui, Y. Yabe, C.-M. Pan and P. S. Baran, Nature, 2014, 516, 343 CrossRef CAS PubMed;
(d) H. T. Dao, C. Li, Q. Michaudel, B. D. Maxwell and P. S. Baran, J. Am. Chem. Soc., 2015, 137, 8046 CrossRef CAS PubMed.
-
(a) J. Zheng, J. Qi and S. Cui, Org. Lett., 2016, 18, 128 CrossRef CAS PubMed;
(b) J. Zheng, D. Wang and S. Cui, Org. Lett., 2015, 17, 4572 CrossRef CAS PubMed;
(c) Y. Shen, J. Qi and S. Cui, Org. Lett., 2016, 18, 2722 CrossRef CAS PubMed.
-
(a) D. Bondar, J. Liu, T. Muller and L. A. Paquette, Org. Lett., 2005, 7, 1813 CrossRef CAS PubMed;
(b) J. Shi, G. Manolikakes, C. H. Yeh, C. A. Guerrero, R. A. Shenvi, H. Shigehisa and P. S. Baran, J. Am. Chem. Soc., 2011, 133, 8014 CrossRef CAS PubMed;
(c) J. Shi, H. Shigehisa, C. A. Guerrero, R. A. Shenvi, C. C. Li and P. S. Baran, Angew. Chem., Int. Ed., 2009, 48, 4328 CrossRef CAS PubMed;
(d) E. M. P. Silva, R. J. Pye, C. Cardin and L. M. Harwood, Synlett, 2010, 509 CAS;
(e) G. Liu and D. Romo, Angew. Chem., Int. Ed., 2011, 50, 7537 CrossRef CAS PubMed;
(f) M. A. A. Endoma-Arias and T. Hudlicky, Tetrahedron Lett., 2011, 52, 6632 CrossRef CAS;
(g) O. F. Jeker and E. M. Carreira, Angew. Chem., Int. Ed., 2012, 51, 3474 CrossRef CAS PubMed.
-
(a) Y. Monguchi, Y. Sawama and H. Sajiki, Heterocycles, 2015, 91, 239 CrossRef CAS;
(b) D. Antermite, L. Degennaro and R. Luisi, Org. Biomol. Chem., 2017, 15, 34 RSC;
(c) K. Biggadike, M. Ahmed, D. L. Ball, D. A. Dalmas Wilk and C. D. Edwards, J. Med. Chem., 2016, 59, 1711 CrossRef CAS PubMed;
(d) H. Cui, S. Peng, X. Gu, H. Chen, Y. He and W. Gao, Eur. J. Med. Chem., 2016, 111, 126 CrossRef CAS PubMed.
- D. T. George, E. J. Kuenstner and S. V. Pronin, J. Am. Chem. Soc., 2015, 137, 15410 CrossRef CAS PubMed.
- X. Hu and T. J. Maimone, J. Am. Chem. Soc., 2014, 136, 5287 CrossRef CAS PubMed.
-
(a) J. Zheng, Y. Zhang, Z. Deng and S. Cui, Adv. Synth. Catal., 2016, 358, 746 CrossRef CAS;
(b) S. Cui, Y. Zhang, D. Wang and Q. Wu, Chem. Sci., 2013, 4, 3912 RSC;
(c) Y. Zhang, D. Wang and S. Cui, Org. Lett., 2015, 17, 2494 CrossRef CAS PubMed;
(d) S. Cui, Y. Zhang and Q. Wu, Chem. Sci., 2013, 4, 3421 RSC;
(e) Y. Zhang, Q. Wu and S. Cui, Chem. Sci., 2014, 5, 297 RSC;
(f) Y. Zhang, J. Zheng and S. Cui, J. Org. Chem., 2014, 79, 6490 CrossRef CAS PubMed;
(g) J. Zheng, Y. Zhang and S. Cui, Org. Lett., 2014, 16, 3560 CrossRef CAS PubMed.
- CCDC 1491658.†.
- CCDC 1523563.†.
-
(a) R. C. Pandey, M. W. Toussaint, R. M. Stroshane, C. C. Kalita, A. A. Aszalos, A. L. Garretson, T. T. Wei, K. M. Byme, R. F. Geoghegan and R. J. White, J. Antibiot., 1981, 34, 1389 CrossRef CAS PubMed;
(b) R. Miosra, R. C. Pandey, B. D. Hilton, P. P. Roller and J. V. Silverton, J. Antibiot., 1987, 40, 786 CrossRef;
(c) D. L. Boger and I. C. Jacobson, J. Org. Chem., 1990, 55, 1919 CrossRef CAS;
(d) J. A. Wendt, P. J. Gauvreau and R. D. Bach, J. Am. Chem. Soc., 1994, 116, 9921 CrossRef CAS.
- B. A. Brooks, E. M. Blair, R. Finch and A. F. Lant, Br. J. Clin. Pharmacol., 1980, 10, 248 CrossRef.
- K. Nakamura, S. Hamano and T. Horie, J. Labelled Compd. Radiopharm., 1977, 2, 191 Search PubMed.
Footnote |
† Electronic supplementary information (ESI) available. CCDC 1491658 and 1523563. For ESI and crystallographic data in CIF or other electronic format see DOI: 10.1039/c7qo00817a |
|
This journal is © the Partner Organisations 2018 |
Click here to see how this site uses Cookies. View our privacy policy here.