DOI:
10.1039/C2RA20403G
(Communication)
RSC Adv., 2012,
2, 4065-4068
Room temperature synthesis of isoquino[2,1-a][3,1]oxazine and isoquino[2,1-a]pyrimidine derivatives via visible light photoredox catalysis†
Received
5th March 2012
, Accepted 12th March 2012
First published on 13th March 2012
Abstract
A novel and efficient construction of isoquino[2,1-a][3,1]oxazine and isoquino[2,1-a]pyrimidine frameworks has been realized by means of visible light photocatalytic reactions using air as the oxidant at room temperature. This strategy offers a direct and rapid access to two kinds of biologically important fused heterocycles in good to excellent yields.
Nitrogen- and oxygen-containing fused heterocycles are widely found in natural products as well as in pharmaceuticals and other fine chemicals.1 Among those compounds, the isoquino[2,1-a][3,1]oxazine and isoquino[2,1-a]pyrimidine derivatives have received much attention and such architectures have been considered as “privileged structures” for drug discovery.2 For instance, PD 102807 is an efficient selective M4 muscarinic receptor antagonist,2c,d whilst compound A has proven to be a selective acetylcholinesterase inhibitor with an IC50 (AChE) of 12 μM (Fig. 1).2e Driven by their rich biological activities, the development of efficient methods for the construction of these fused heterocyclic compounds is of widespread interest in synthetic organic chemistry. These unique structures are typically constructed by means of domino reactions and oxidative cyclization reactions.3 These processes either required a high reaction temperature3a,b or needed specific oxidants,3c–e and therefore have shown limited generality. Recently, the Seidel group disclosed an elegant method to synthesise isoquino[2,1-a]pyrimidine derivatives through an α-functionalization reaction of cyclic amines.4 Furthermore, Che and co-workers reported an intramolecular amination of sp2 and sp3 C–H bonds with aryl azides as the nitrogen source,5 providing an alternative route to these important heterocycles. Despite advances, the development of a versatile method, which can be applied to the construction of both isoquino[2,1-a][3,1]oxazine and isoquino[2,1-a]pyrimidine systems under benign conditions, is highly desirable and remains a challenge.
![Examples of biologically important molecules containing isoquino[2,1-a][3,1]oxazine and isoquino[2,1-a]pyrimidine motifs.](/image/article/2012/RA/c2ra20403g/c2ra20403g-f1.gif) |
| Fig. 1 Examples of biologically important molecules containing isoquino[2,1-a][3,1]oxazine and isoquino[2,1-a]pyrimidine motifs. | |
With inherent “green chemistry” features, the use of visible light to initiate organic transformations has attracted more and more attention.6 Various chemical reactions have been elegantly carried out under the irradiation of visible light.7–10 Among these transformations, the oxidation of tertiary amines to highly reactive iminium ions followed by further functionalizations has been well established.11 As part of our ongoing project on the synthesis of heterocyclic structures by visible light photoredox catalysis,12 we describe herein a new route to construct isoquino[2,1-a][3,1]oxazine and isoquino[2,1-a]pyrimidine frameworks through a photocatalytic oxidation/functionalization sequence (Scheme 1).
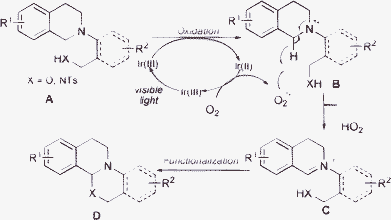 |
| Scheme 1 Photocatalytic oxidation/functionalization sequence. | |
Our studies began with the reaction of (2-(3,4-dihydroisoquinolin-2(1H)-yl)phenyl)methanol (1a) in the presence of 5.0 mol% Ir(ppy)2(dtb–bpy)PF6 under 36 W fluorescent light irradiation. As summarized in Table 1, the solvent investigation revealed that the reaction media had a pronounced effect on the reaction efficiency. DMF, DMSO, and CH3CN have been widely used in visible light photocatalytic transformation,7–12 but surprisingly they did not work well in our model reaction. The yield of the desired product 2a was basically less than 40% (Table 1, entries 1–3). Other solvents such as acetone (Table 1, entry 4), THF (Table 1, entry 5) and MeOH (Table 1, entry 6) have been examined. To our delight, 62% yield was achieved in the case of MeOH as the reaction media (Table 1, entry 6). When the reaction was performed in EtOH, it took longer to reach completion and gave a relatively lower efficiency (Table 1, entry 7, 58% yield). A significant improvement was observed when the concentration of the substrate was increased from 0.1 M to 0.3 M (62% to 73%, Table 1, entries 6 and 8), which might be attributed to the poor solubility of the product in MeOH. Further optimization showed that the catalyst loading could be decreased to 0.5 mol% without loss in the reaction yield (Table 1, entry 9).
Table 1 Optimisation of reaction conditionsa
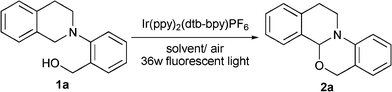
|
Entry |
Solvent |
Time (h) |
Yield (%)b |
Reaction conditions: 1a (0.3 mmol) and Ir(ppy)2(dtb–bpy)PF6 (5.0 mol%), solvent (3.0 mL), air and 36 W fluorescent light irradiation.
Yield of the isolated product.
1.0 mL MeOH was used.
0.5 mol% catalyst was used.
|
1 |
DMF |
8 |
16 |
2 |
DMSO |
3 |
37 |
3 |
CH3CN |
8 |
20 |
4 |
acetone |
10 |
38 |
5 |
THF |
4 |
19 |
6 |
MeOH |
7 |
62 |
7 |
EtOH |
24 |
58 |
8c |
MeOH |
12 |
73 |
9d |
MeOH |
13 |
72 |
After the optimal reaction conditions were established, we then investigated the scope of this photocatalytic oxidation/functionalization cascade. As highlighted in Table 2, this reaction appeared to be quite general with respect to structural variation of both aryl groups in the substrate. Incorporation of one or more alkyl and alkoxyl substituents at different positions on the substrate (e.g.1b–1f) revealed that electronic and steric modification of the substrate can be accomplished, and the reaction gave the corresponding products in 52–65% yields. As revealed in the reaction of 1g, we have successfully used an electron-deficient nucleophilic component, (2-(3,4-dihydroisoquinolin-2(1H)-yl)-5-nitrophenyl)methanol. Moreover, the reaction appears tolerant with respect to the steric contribution near the –OH group. Incorporation of a methyl group at the α position to the hydroxyl group can be realized (e.g.1h). It was noteworthy that the less reactive tertiary amine 1i was also a promising substrate to give the desired fused heterocycle in moderate yield (2i, 50%). Significantly, this methodology could be successfully extended to construct the isoquino[2,1-a]pyrimidine framework. The reactions of 1j–1m furnished the corresponding products in relatively high yield probably due to the higher nucleophilicity of –NHTs in the presence of tBuOK. Furthermore, the structure of product 2a was unambiguously established by X-ray crystallographic analysis.13
Table 2 Substrates scope examinationab
Reaction conditions: 1a–1i (0.3 mmol), Ir(ppy)2(dtb–bpy)PF6 (0.5 mol%), MeOH (1 mL), air and 36 W fluorescent light irradiation; 1j–1m (0.2 mmol), Ir(ppy)2(dtb–bpy)PF6 (0.5 mol%), tBuOK (2.0 equiv.), MeOH/DCM (1 mL + 1 mL), air and 36 W fluorescent light irradiation.
Yields given are of the isolated product.
determined by 1H NMR.
|
|
In order to demonstrate the preparative utility of this methodology, the reaction of 1k (1.02 g) was performed on a gram scale in the presence of 0.5 mol% of Ir(ppy)2(dtb–bpy)PF6 (Scheme 2, eqn 1). The reaction proceeded smoothly to afford the corresponding fused heterocycle 2k in 82% yield after 36 h. Perhaps more importantly, the metal catalyst could be successfully replaced by an inexpensive organic dye catalyst without loss on the reaction efficiency (Scheme 2, eqn 2).
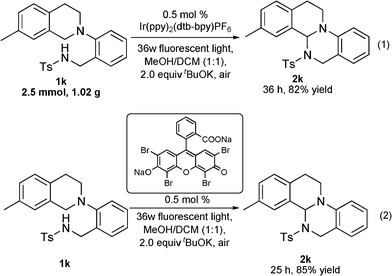 |
| Scheme 2 Gram scale and metal-free reactions. | |
The reaction of substrate 1n was performed under the standard conditions in an effort to synthesize structurally more complex fused heterocycle 2n, assuming the formation of two carbon–nitrogen bonds in a one-pot procedure. However, only one oxidation/functionalization product 2o was obtained in 56% yield after 64 h (Scheme 3).
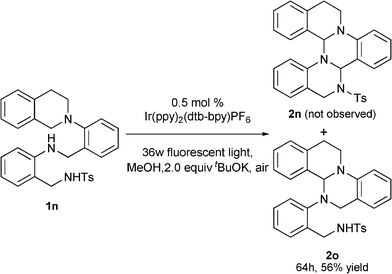 |
| Scheme 3 The reaction of substrate 1n. | |
Conclusions
In summary, we have developed a novel and efficient procedure to construct both isoquino[2,1-a][3,1]oxazine and isoquino[2,1-a]pyrimidine frameworks in good to excellent yields by means of a visible light photoredox catalysis strategy. It should be noted that the reaction features simple experimental procedures under very mild conditions. The application of this photoredox catalysis strategy to the synthesis of other biologically important heterocycles is currently underway in our laboratory.
Experimental section
General procedure for photocatalytic synthesis of isoquino[2,1-a][3,1]oxazine derivatives 2a–2i
To a 5.0 mL flask equipped with a magnetic stir bar was added substrates 1a–i (0.3 mmol), 0.5 mol% Ir(ppy)2(dtb–bpy)PF6 (1.37 mg, 0.0015 mmol) and MeOH (1.0 mL). After this, the solution was stirred in the open air at a distance of ∼5 cm from a 36 W fluorescent lamp at room temperature. The reaction was stopped at the indicated time (TLC) and the solvent was removed under reduced pressure. The crude product was purified by flash chromatography on silica gel (silica: 200–300; eluant: petroleum ether/ethyl acetate (10
:
1–5
:
1)) to provide pure products.
General procedure for photocatalytic synthesis of isoquino[2,1-a]pyrimidine derivatives 2j–m, 2o
To a 5.0 mL flask equipped with a magnetic stir bar was added substrates 1j–n (0.2 mmol), 0.5 mol% Ir(ppy)2(dtb–bpy)PF6 (0.913 mg, 0.001 mmol), tBuOK (44.8 mg, 0.4 mmol) and solvent (2.0 mL). After this, the solution was stirred in the open air at a distance of ∼5 cm from a 36 W fluorescent lamp at room temperature. Upon the completion of the reaction monitored by TLC, the solvent was removed under reduced pressure. The crude product was purified by flash chromatography on silica gel (silica: 200–300; eluant: petroleum ether/ethyl acetate (10
:
1–5
:
1)) to provide pure products.
Acknowledgements
We are grateful to the National Science Foundation of China (21072069 and 21002036) and the National Basic Research Program of China (2011CB808600) for support of this research.
References
- For selective examples and reviews, see:
(a) M. D. McReynolds, J. M. Dougherty and P. R. Hanson, Chem. Rev., 2004, 104, 2239–2258 CrossRef CAS;
(b) F. Alonso, I. P. Beletskaya and M. Yus, Chem. Rev., 2004, 104, 3079–3159 CrossRef CAS;
(c) A. Deiters and S. F. Martin, Chem. Rev., 2004, 104, 2199–2238 CrossRef CAS;
(d) G. Zeni and R. C. Larock, Chem. Rev., 2004, 104, 2285–2309 CrossRef CAS;
(e) N. T. Patil and Y. Yamamoto, Chem. Rev., 2008, 108, 3395–3442 CrossRef CAS;
(f) G. Evano, N. Blanchard and M. Toumi, Chem. Rev., 2008, 108, 3054–3131 CrossRef CAS;
(g) Z. Jakopin and M. S. Dolenc, Curr. Med. Chem., 2010, 17, 651–671 CrossRef CAS;
(h) A. Diéguez-Vázquez, C. C. Tzschucke, W. Y. Lam and S. V. Ley, Angew. Chem., Int. Ed., 2008, 47, 209–212 CrossRef;
(i) A. R. Katritzky and S. Rachwal, Chem. Rev., 2010, 110, 1564–1610 CrossRef CAS.
-
(a) M. C. Olianas and P. Onali, Life Sci., 1999, 65, 2233–2240 CrossRef CAS;
(b) K. Kitaichi, J. C. Day and R. Quirion, Eur. J. Pharmacol., 1999, 383, 53–56 CrossRef CAS;
(c) C. Stoll, U. Schwarzwälder, S. Johann, G. Lambrecht, G. Hertting, T. J. Feuerstein and R. Jackisch, Neurochem. Res., 2003, 28, 413–417 CrossRef CAS;
(d) C. E. Augelli-Szafran, J. C. Jaen, D. W. Moreland, C. B. Nelson, J. R. Penvose-Yi and R. D. Schwarz, Bioorg. Med. Chem. Lett., 1998, 8, 1991–1996 CrossRef CAS;
(e) M. Decker, F. Krauth and J. Lehmann, Bioorg. Med. Chem., 2006, 14, 1966–1977 CrossRef CAS;
(f) M. Decker, Eur. J. Med. Chem., 2005, 40, 305–313 CrossRef CAS.
-
(a) K. Umetsu and N. Asao, Tetrahedron Lett., 2008, 49, 2722–2725 CrossRef CAS;
(b) I. Szatmári and F. Fülöp, Tetrahedron Lett., 2011, 52, 4440–4442 CrossRef;
(c) G. Kumaraswamy, A. N. Murthy and A. Pitchaiah, J. Org. Chem., 2010, 75, 3916–3919 CrossRef CAS;
(d) S. P. Stanforth, Tetrahedron, 2000, 56, 461–464 CrossRef CAS;
(e) K. Okimoto, T. Yoshida, M. Hoshi, K. Hottori, M. Komata, K. Numata and K. Tomozawa, Heterocycles, 2006, 68, 2563–2570 CrossRef.
-
(a) C. Zhang, C. K. De, R. Mal and D. Seidel, J. Am. Chem. Soc., 2008, 130, 416–417 CrossRef CAS;
(b) C. Zhang, S. Murarka and D. Seidel, J. Org. Chem., 2009, 74, 419–422 CrossRef CAS.
- Y.-G. Liu, J.-H. Wei and C.-M. Che, Chem. Commun., 2010, 46, 6926–6928 RSC.
- For recent reviews on photoredox catalysis, see:
(a) K. Zeitler, Angew. Chem., Int. Ed., 2009, 48, 9785–9789 CrossRef CAS;
(b) T. P. Yoon, M. A. Ischay and J. Du, Nat. Chem., 2010, 2, 527–532 CrossRef CAS;
(c) J. M. R. Narayanam and C. R. J. Stephenson, Chem. Soc. Rev., 2011, 40, 102–125 RSC;
(d) F. Teplý, Collect. Czech. Chem. Commun., 2011, 76, 859–917 CrossRef;
(e) J. W. Tucker and C. R. J. Stephenson, J. Org. Chem., 2012, 77, 1617–1622 CrossRef CAS.
- Selected recent articles from the MacMillan group, see:
(a) D. A. Nicewicz and D. W. C. MacMillan, Science, 2008, 322, 77–80 CrossRef CAS;
(b) P. V. Pham, D. A. Nagib and D. W. C. MacMillan, Angew. Chem., Int. Ed., 2011, 50, 6119–6122 CrossRef CAS;
(c) D. A. Nagib and D. W. C. MacMillan, Nature, 2011, 480, 224–228 CrossRef CAS;
(d) A. McNally, C. K. Prier and D. W. C. MacMillan, Science, 2011, 334, 1114–1117 CrossRef CAS.
- Selected recent articles from the Yoon group, see:
(a) Z. Lu, M. Shen and T. P. Yoon, J. Am. Chem. Soc., 2011, 133, 1162–1164 CrossRef CAS;
(b) A. E. Hurtley, M. A. Cimesia, M. A. Ischay and T. P. Yoon, Tetrahedron, 2011, 67, 4442–4448 CrossRef CAS;
(c) S.-S. Lin, M. A. Ischay, C. G. Fry and T. P. Yoon, J. Am. Chem. Soc., 2011, 133, 19350–19353 CrossRef CAS;
(d) J. Du, L. R. Espelt, I. A. Guzei and T. P. Yoon, Chem. Sci., 2011, 2, 2115–2119 RSC;
(e) E. L. Tyson, E. P. Farney and T. P. Yoon, Org. Lett., 2012, 14, 1110–1113 CrossRef CAS.
- Selected recent articles from the Stephenson group, see:
(a) C. Dai, J. M. R. Narayanam and C. R. J. Stephenson, Nat. Chem., 2011, 3, 140–145 CrossRef CAS;
(b) J. W. Tucker, J. M. R. Narayanam, P. S. Shah and C. R. J. Stephenson, Chem. Commun., 2011, 47, 5040–5042 RSC;
(c) J. D. Nguyen, J. W. Tucker, M. D. Konieczynska and C. R. J. Stephenson, J. Am. Chem. Soc., 2011, 133, 4160–4163 CrossRef CAS;
(d) L. Furst, J. M. R. Narayanam and C. R. J. Stephenson, Angew. Chem., Int. Ed., 2011, 50, 9655–9659 CrossRef CAS;
(e) J. W. Tucker and C. R. J. Stephenson, Org. Lett., 2011, 13, 5468–5471 CrossRef CAS.
- Selected articles from other groups, see:
(a) R. S. Andrews, J. J. Becker and M. R. Gagné, Angew. Chem., Int. Ed., 2010, 49, 7274–7276 CrossRef CAS;
(b) R. S. Andrews, J. J. Becker and M. R. Gagné, Org. Lett., 2011, 13, 2406–2409 CrossRef CAS;
(c) T. Maji, A. Karmakar and O. Reiser, J. Org. Chem., 2011, 76, 736–739 CrossRef CAS;
(d) M. H. Larraufie, R. Pellet, L. Fensterbank, J. P. Goddard, E. Lacôte, M. Malacria and C. Ollivier, Angew. Chem., Int. Ed., 2011, 50, 4463–4466 CrossRef CAS;
(e) Y. Su, L. Zhang and N. Jiao, Org. Lett., 2011, 13, 2168–2171 CrossRef CAS;
(f) J. B. Edson, L. P. Spencer and J. M. Boncella, Org. Lett., 2011, 13, 6156–6159 CrossRef CAS;
(g) D. Kalyani, K. B. McMurtrey, S. R. Neufeldt and M. S. Sanford, J. Am. Chem. Soc., 2011, 133, 18566–18569 CrossRef CAS;
(h) Y.-N. Cheng, J. Yang, Y. Qu and P.-X. Li, Org. Lett., 2012, 14, 98–101 CrossRef CAS;
(i) T. Courant and G. Masson, Chem.–Eur. J., 2012, 18, 423–427 CrossRef CAS;
(j) M. Zhu and N. Zheng, Synthesis, 2011, 2223–2236 CAS;
(k) S. Maity, M.-Z. Zhu, R. S. Shinabery and N. Zheng, Angew. Chem., Int. Ed., 2012, 51, 222–226 CrossRef CAS;
(l) X.-H. Ju, Y. Liang, P.-J. Jia, W.-F. Li and W. Yu, Org. Biomol. Chem., 2012, 10, 498–500 RSC;
(m) D. P. Hari, P. Schroll and B. Koönig, J. Am. Chem. Soc., 2012, 134, 2958–2961 CrossRef CAS;
(n) F. R. Bou-Hamdan and P. H. Seeberger, Chem. Sci., 2012 10.1039/c2sc01016j.
- Selected articles on photochemical oxidations of tertiary amines and their applications in synthesis, see:
(a) A. G. Condie, J. C. Gonzalez-Gomez and C. R. J. Stephenson, J. Am. Chem. Soc., 2010, 132, 1464–1465 CrossRef CAS;
(b) M. Neumann, S. Füldner, B. König and K. Zeitler, Angew. Chem., Int. Ed., 2011, 50, 951–954 CrossRef CAS;
(c) D. P. Hari and B. König, Org. Lett., 2011, 13, 3852–3855 CrossRef CAS;
(d) M. Rueping, C. Vila, R. M. Koenigs, K. Poscharny and D. C. Fabry, Chem. Commun., 2011, 47, 2360–2362 RSC;
(e) M. Rueping, S.-Q. Zhu and R. M. Koenigs, Chem. Commun., 2011, 47, 8679–8681 RSC;
(f) M. Rueping, D. Leonori and T. Poisson, Chem. Commun., 2011, 47, 9615–9617 RSC;
(g) M. Rueping, S.-Q. Zhu and R. M. Koenigs, Chem. Commun., 2011, 47, 12709–12711 RSC;
(h) D. B. Freeman, L. Furst, A. G. Condie and C. R. J. Stephenson, Org. Lett., 2012, 14, 94–97 CrossRef CAS;
(i) Q. Liu, Y.-N. Li, H.-H. Zhang, B. Chen, C.-H. Tung and L.-Z. Wu, Chem.–Eur. J., 2012, 18, 620–627 CrossRef CAS;
(j) Y.-H. Pan, S. Wang, C. W. Kee, E. Dubuisson, Y.-Y. Yang, K. P. Loha and C.-H. Tan, Green Chem., 2011, 13, 3341–3343 RSC;
(k) P. Kohls, D. Jadhav, G. Pandey and O. Reiser, Org. Lett., 2012, 14, 672–675 CrossRef CAS;
(l) Y. Miyake, K. Nakajima and Y. Nishibayashi, J. Am. Chem. Soc., 2012, 134, 3338–3341 CrossRef CAS.
-
(a) J. Xuan, Y. Cheng, J. An, L.-Q. Lu, X.-X. Zhang and W.-J. Xiao, Chem. Commun., 2011, 47, 8337–8339 RSC;
(b) Y.-Q. Zou, L.-Q. Lu, L. Fu, N.-J. Chang, J. Rong, J.-R. Chen and W.-J. Xiao, Angew. Chem., Int. Ed., 2011, 50, 7171–7175 CrossRef CAS;
(c) Y.-Q. Zou, J.-R. Chen, X.-P. Liu, L.-Q. Lu, R. L. Davis, K. A. Jørgensen and W.-J. Xiao, Angew. Chem., Int. Ed., 2012, 51, 784–788 CrossRef CAS.
- X-Ray crystal data for 2a CCDC 848932†: C16H15NO, M = 237.29, monoclinic, P21/c, P2ybc, a = 10.7911(19) Å, b = 8.3666(15) Å, c = 6.8985(12) Å, α = 90.00, β = 93.084(3), γ = 90.00, V = 621.93(19) Å3, Z = 2, T = 296(2), F000 = 264, final R indices [I > 2σ(I)]: R1 = 0.0595, wR2 = 0.1796, R indices (all data): R1 = 0.0637, wR2 = 0.1854.
Footnote |
† Electronic supplementary information (ESI) available: Substrates preparation, experimental procedures and compound characterisation data. CCDC reference number 848932. For ESI and crystallographic data in CIF or other electronic format see DOI: 10.1039/c2ra20403g |
|
This journal is © The Royal Society of Chemistry 2012 |
Click here to see how this site uses Cookies. View our privacy policy here.