Reduction in insect attachment ability by biogenic and non-biogenic ZnO nanoparticles
Received
17th August 2023
, Accepted 27th September 2023
First published on 16th October 2023
Abstract
Nanomaterials can represent an environmentally safe method to control different insect pests. The present study investigated the effect of biogenic and non-biogenic zinc oxide nanoparticles (ZnO-NPs) on the attachment ability of the Southern green stink bug, Nezara viridula, a major agricultural pest. The experiments were conducted on glass surfaces treated with different concentrations of ZnO-NPs, and the attachment ability of adult males of N. viridula was measured through traction force experiments. The results showed that both biogenic and non-biogenic ZnO-NPs reduced the attachment ability of N. viridula, with a significant decrease in traction force observed at concentrations of 12.5 mg L−1 and above. SEM analyses revealed that biogenic and non-biogenic ZnO-NPs aggregated on the attachment devices of N. viridula, including the pulvilli, the hairy pad and claws, disrupting the attachment mechanism. This study suggests that ZnO-NPs, particularly biogenic ZnO-NPs obtained from plant extract, have potential as a natural and eco-friendly pest control agent to reduce insect attachment and damage to crops.
Environmental significance
Phytophagous insects cause considerable damage to plants, heavily reducing crop production. Insect pests are controlled mainly by insecticides, which present health and environmental issues. The ability to firmly adhere to plant surfaces along the whole life cycle is of pivotal importance for insect survival and reproductive success. In this context, the development of antiadhesive coatings can represent an alternative method to reduce insect pest impact in agriculture. Here we demonstrate that both biogenic and non-biogenic ZnO-nanoparticles can reduce the attachment ability of the green stinkbug Nezara viridula, a major pest worldwide. ZnO-NPs aggregate on the insect attachment devices (pulvilli, hairy pad and claws), disrupting the insect attachment mechanism. This study may help to develop sustainable, nontoxic methods that can be alternatives to insecticides.
|
Introduction
Nearly half of the approximately 1 million described insect species are phytophagous insects,1 and many represent insect pests that cause considerable damage to plants, with crop losses of about 18 and 16%.2 The need to control pest insects in agriculture to prevent crop diseases or damage led to the development of synthetic organic insecticides, mainly represented by organophosphates, carbamates, synthetic pyrethroids, and neonicotinoids.3 Despite their beneficial impact on crop production, these chemicals can have acute and chronic effects on humans if they reach the trophic chain when reaching and accumulating in non-target organisms, and they can also pose more than one risk to environmental health.4,5 Consequently, the indiscriminate use of pesticides can result in environmental pollution, the emergence of agricultural pests and pathogens, and the loss of biodiversity.
Alternative and eco-friendly strategies have been developed over the last few decades, and many studies and research are still on course to offer sustainable and less environmentally impacting solutions to face the above problems that plague cropping systems. Among the approaches to protect plants from insects and environmental biotic and abiotic stresses, inert powders (particle film technology) are regarded for the coating of plants with chemically inert mineral particles. In particular, they create a porous surface film that does not interfere with plant gas exchanges and can be easily removed after harvest (see the review in ref. 6). In addition, the possibility of reducing insect attachment capacity is exploited as a suitable way to decrease their potential damage to crops, thus reducing crop losses. For these reasons, innovative, nanostructured and also bio-inspired materials have been developed. They are still the subject of intense research as technological solutions needed in a changing global society to control and minimize the ability of insects to attach to plants.7
In this frame, recent studies have demonstrated that nanoparticles of kaolin, zeolite, and calcium carbonate can affect the attachment ability of the Southern green stinkbug Nezara viridula (Hemiptera: pentatomidae), one of the most relevant pentatomid insect pests in the world,8 to natural and artificial surfaces.9,10 It is to be emphasized that the ability to firmly adhere to the surface along the whole life cycle is of pivotal importance for insect survival and reproductive success. For this reason, insects have developed very efficient attachment devices located on their tarsi represented by claws and smooth or hairy pads, supplemented with fluids, which make them able to cope with different slopes and with substrates of varying roughness and wettability (e.g. ref. 11–20).
Within this frame, nanoscience and nanotechnologies are also trying to respond to the problems affecting agriculture by developing and producing nanomaterials (NMs) to improve the crop yield and productivity and to cope with losses caused by biotic and abiotic stresses, nutrient deficiency and environmental pollution.21 Among the different applications, nanostructured materials can control different pests (reviewed in ref. 22 and 23). In particular, NMs can be used directly as active pesticide agents or nanocarriers to deliver active compounds to target pests.24,25 The effectiveness of nanoparticles (NPs) for the purposes mentioned above lies in their small size (in the range of 1 to 100 nm for at least one dimension), surface area and unique electronic properties that allow them to interact with biological entities more efficiently than the same material in bulk form.26
NP synthesis can traditionally be carried out through physical and chemical methods, which are expensive and time-consuming and can impact the environment. However, NPs can also be synthesized using green synthesis approaches in water as solvent or biological extract from plants (biogenic synthesis).27,28 Regarding bio-fabricated metal oxide nanoparticles, the process is generally carried out by leaving a suitable biological extract to react with a metal saline precursor.21 Generally, the effectiveness of the biogenic synthesis depends on the composition of the biological entity used.21,29 In fact, some biomolecules in the extracts can act as capping agents, thus stabilizing and allowing the nanoparticulation processes to occur.21,30 In addition, the extract can also operate the bio-reduction of the metal precursor from an oxidized to a zero-valent state and allow NPs to nucleate.31
Among the bio-fabricated NPs, the biogenic zinc oxide nanoparticles (ZnO-NPs) are being extensively studied as they can be easily obtained using suitable plant extracts.27 In addition, ZnO-NPs are considered safer and less toxic than those obtained from other oxides.32 Regarding agriculture, some recent studies have shown that ZnO-NPs, if applied in appropriate dosages, can prompt beneficial effects in crops by stimulating growth and yield, improving nutrient acquisition and antioxidant defenses. In particular, two recent studies highlighted that biogenic ZnO-NPs prompted beneficial effects in maize seedlings and olive tree explants.21,29
Given the growing interest and promising prospects in using NMs for agricultural applications, the present study aimed to test the effect of ZnO-NPs on insect attachment ability on artificial surfaces. To date, while NPs of various types have been studied to contain crop-damaging insects by exploiting their direct toxic action, no study has instead proposed an approach to undermine the ability of insects to adhere to surfaces using ZnO-NPs. Therefore, for this purpose, biogenic ZnO-NPs and non-biogenic ZnO-NPs (green water synthesized) were obtained according to a previously published methodology21 and applied to artificial surfaces. Then, the problematic Southern green stinkbug N. viridula was investigated as the model insect, evaluating its ability to attach to artificial surfaces (glass) treated with different concentrations of the NPs mentioned above. To estimate the possible contamination effect of ZnO-NPs, we also studied the tarsal attachment devices of insects after walking on the treated surfaces.
Materials and methods
Insects
N. viridula adults and nymphs were collected in a field around Perugia (Umbria region, Italy) in June 2021 and reared in a controlled condition chamber (14 h photophase, temperature of 25 ± 1 °C; RH of 70 ± 10%) inside net cages (300 mm × 300 mm × 300 mm) (Vermandel, Hulst, The Netherlands). Nymphs and adults were fed with sunflower seeds (Helianthus annuus L.), pods of French bean (Phaseolus vulgaris L.) and cabbage leaves (Brassica oleracea L.). Only males were used for the experiments (while females were kept for the rearing), since no sexual dimorphism was previously observed in the attachment ability of males and females of N. viridula.33,34 As variations in attachment ability were highlighted in N. viridula adults according to the insect age,35 only males about ten days old were used.
Biogenic and non-biogenic ZnO-NPs
The synthesis of biogenic nanoparticles was conducted following a procedure previously published by Del Buono et al., 2021,21 using a hydroalcoholic extract obtained from an aquatic plant species, Lemna minor L., as a capping agent. In addition, the non-biogenic ZnO-NPs were obtained following the procedure described in the above-mentioned paper. The ZnO-NPs showed in the case of the biogenic synthesis a spherical shape and dimensions in the range of 10–20 nm, and in that of the green synthesis in water, a nano-lamellar structure and dimensions in the range of 20–100 nm (see all the characterization data reported in ref. 21).
Substrate preparation and characterization
Hydrophilic glass with a contact angle of 47.06 ± 3.37° (mean ± SD) was characterized in a previous study10 measuring the contact angle of water (Aqua Millipore, droplet size = 1 μL, sessile drop method) using a high-speed optical contact angle measuring instrument OCAH 200 (DataPhysics Instruments GmbH, Filderstadt, Germany). The glass (8 × 10 cm) treated with different biogenic and non-biogenic ZnO-NP suspensions in distilled water at different concentrations (6.25, 12.5, 25, 50, 100, 200 mg L−1) was prepared as follows:
10 ml of a suspension of biogenic and non-biogenic ZnO-NPs in distilled water at different concentrations (mg L−1) was distributed on glass (8 × 10 cm) delimited with an edge of dental wax (silicone elastomer President light body Coltène® Whaledent AG, Altstätten, Switzerland). Afterward, the surfaces were placed to dry in an oven at 30 °C until complete drying. The glass representing the control surface (untreated glass) was treated with 10 ml of distilled water.
The glass was observed under light microscopy and the distribution of NPs on treated glass was evaluated using the open-source image processing program ImageJ36 to verify the effective area covered by ZnO-NPs. The evaluated parameter was the coverage area (%).
Scanning electron microscopy and energy-dispersive X-ray microanalysis (EDX)
Small squares of treated glass were mounted on aluminum stubs using double-sided carbon tape and metalized with a thin layer of chromium (8 nm). Adult tarsi were dissected from adults anaesthetized with carbon dioxide. Samples were dried in an oven at 35 °C for 48 h. Afterwards, the samples were mounted on aluminum stubs using double-sided carbon tape and metalized with chromium. The samples were observed by field emission scanning electron microscopy with an FE SEM LEO 1525 (Zeiss) using back scattered electrons at 15 kV. The presence of biogenic and non-biogenic ZnO-NPs on tarsi was detected using back scattered electrons, which demonstrated compositional differences through contrast (brighter areas indicate the presence of biogenic or non-biogenic ZnO-NPs owing to their higher atomic number composition compared with the insect cuticle).
The quali–quantitative element composition in the tarsi of N. viridula was determined using the same microscope equipped with an energy-dispersive X-ray detector (EDX) (Bruker Quantax). The following parameters were applied: measurement time 5 min, accelerating voltage 15.00 kV, working distance 9 mm.
Traction force experiments
The experiments were performed using a traction force experimental set-up represented by a Biopac force tester (Biopac Systems Ltd, Goleta, CA, U.S.A.). Before each test, adults of N. viridula were weighed on a micro-balance (Phoenix Instruments). Experimental insects were anesthetized with carbon dioxide for 60 s and made incapable of flying by carefully gluing their forewings together using a small droplet of melted wax. One end of a fishing thread gel spun polyethylene (Berkley Spirit Lake, IA, U.S.A.), 0.02 mm in diameter and about 10 cm long, was fixed with a droplet of molten wax to the insect thorax. Before starting the experiments, the insects were left to recover for 30 min. The insect was attached to the force sensor by means of the thread and was allowed to move on the test substrate in a direction perpendicular to the force sensor (and parallel to the substrate) for 240 s. The Biopac force tester consisted of a force sensor FORT-10 (10 g capacity; World Precision Instruments Inc., Sarasota, FL, U.S.A.) connected to a data acquisition unit MP 160 (Biopac Systems Ltd, Goleta, CA, U.S.A.). Data were recorded using AcqKnowledge 5.0 software (Biopac Systems Ltd, Goleta, CA, U.S.A.). The force generated by the insect walking horizontally on the test substrates was measured. Force–time curves were used to evaluate the maximal pulling (traction) force produced by walking insects on the different test surfaces for each individual run. All the experiments were performed during the daytime at 25 ± 1 °C temperature and 60 ± 10% relative humidity.
N. viridula adult males were tested on different treated glass surfaces, according to the following sequence: untreated glass (glass before), treated glass (with biogenic or non-biogenic ZnO-NPs), untreated glass tested just after the test on treated glass (glass after 0 h) and untreated glass tested 24 h after the test on treated glass (glass after 24 h). The insects were tested on untreated glass after walking on treated glass at different time intervals to estimate the possible contamination effect of ZnO-NPs along with time. Seventy adult males of N. viridula were tested for each kind of ZnO-NPs. Force–time curves were used to evaluate the maximal traction force produced.
Statistical analysis
In the traction force experiments, the maximal traction forces obtained for each insect on treated glass at different biogenic and non-biogenic ZnO-NP concentrations, untreated glass after 0 h, and untreated glass after 24 h were normalized by the corresponding maximal traction force obtained on untreated glass (glass before). The normalized traction force obtained on the different surfaces was analysed for each concentration with 1-way repeated measures ANOVA. To verify if the reduction of traction force during the measurement was time-dependent, the traction forces recorded every 60 s on surfaces treated with biogenic and non-biogenic ZnO-NPs with a concentration of 50 mg L−1 were compared with 1-way repeated measures ANOVA. The same test was used for comparison. For all the performed ANOVA, F-tests were used to assess the significance of the effects and their interactions. Multiple comparisons versus traction forces obtained on untreated glass were performed for significant factors using Dunnett's method.37 The Shapiro–Wilk test was used to verify the normality distribution of the data. The traction forces recorded in the first 60 s on untreated and treated surfaces with a concentration of 50 mg L−1 were compared using a t-test for dependent samples. The relationship between the percentage of glass surface covered by NPs and the different tested NP concentrations was tested using linear regression analysis.37 The 50% inhibitory concentration (IC50 values for the ZnO-NP concentration exhibiting antiadhesive properties were calculated using publicly available software on the AAT Bioquest website (https://www.aatbio.com/tools/ic50-calculator).
Results
The biogenic ZnO-NPs on glass (concentration of 200 mg L−1), visualized with SEM (Fig. 1a), show nanometric dimensions and a spherical shape, with aggregates of diameters ranging from 40 to 200 nm. In addition, the non-biogenic ZnO-NPs on glass (Fig. 1b) show nanometric dimensions ranging from 20 to 200 nm and a lamellar morphology with an irregular shape. Both NPs showed a clear tendency to aggregate following the application and drying on the surfaces investigated (Fig. 1).
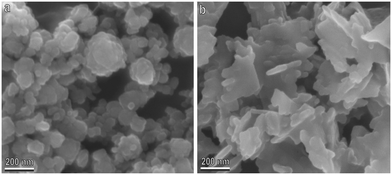 |
| Fig. 1 SEM images of biogenic ZnO-NPs (a) and non-biogenic ZnO-NPs (b). | |
The experiments to test the attachment ability of N. viridula adult males to different glass surfaces, untreated or treated with biogenic ZnO-NPs, were carried out according to the following sequence: untreated glass, treated glass, untreated glass after 0 h, and untreated glass after 24 h. The insect traction force on treated glass was significantly lower than that recorded on untreated glass, starting from a concentration of biogenic ZnO-NPs of 12.5 mg L−1. At a lower concentration (6.25 mg L−1), the insect attachment ability on treated glass was not significantly different from that recorded on glass (Fig. 2). In traction force experiments performed using N. viridula adults pulling on untreated glass at different time intervals after having walked on treated glass, i.e., after 0 h and after 24 h, there was no difference in the insect traction force recorded on untreated glass both after 0 and after 24 h and the initial insect traction force exerted by insects pulling on untreated glass (Fig. 2) (6.5 mg L−1: F = 1.79; d.f. = 3, 39; p = 0.173. 12.5 mg L−1: F = 17.50; d.f. = 3, 27; p < 0.001. 25 mg L−1: F = 64.33; d.f. = 3, 53; p < 0.001. 50 mg L−1: F = 45.80; d.f. = 3, 55; p < 0.001. 100 mg L−1: F = 39.69; d.f. = 3, 58; p < 0.001. 200 mg L−1: F = 49.01; d.f. = 3, 48; p < 0.001).
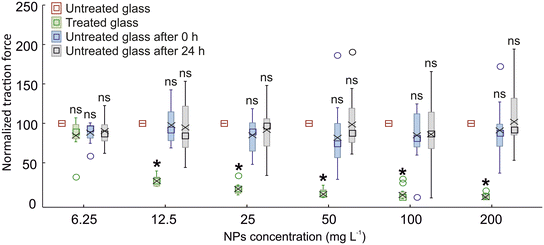 |
| Fig. 2 Normalized traction force obtained in traction force experiments with Nezara viridula pulling on untreated glass, treated glass at different biogenic ZnO-NP concentrations (mg L−1), untreated glass after 0 h, and untreated glass after 24 h. Boxplots show the interquartile range and the median (square), whiskers indicate the 1.5 × interquartile range, and X shows the arithmetic mean. Boxplots with an asterisk are significantly different from the untreated glass at p < 0.05, 1-way repeated measures ANOVA, Dunnett's method post hoc test. | |
The experiments to test the attachment ability of N. viridula adult males to different glass surfaces, untreated or treated with non-biogenic ZnO-NPs, were conducted according to the following sequence: untreated glass, treated glass, untreated glass after 0 h, and untreated glass after 24 h. The insect traction force on nanoparticle treated glass was significantly lower than that recorded on untreated glass, starting from a concentration of biogenic ZnO-NPs of 12.5 mg L−1. In contrast, at a lower concentration (6.25 mg L−1) the insect attachment ability on treated glass was not significantly different from that recorded on untreated glass (Fig. 3). In traction force experiments performed using N. viridula adults pulling on glass at different time intervals after having walked on treated surfaces, i.e., after 0 h and after 24 h, in insects tested immediately after walking on treated glass (untreated glass after 0 h), the traction force on glass was significantly lower than that recorded on untreated glass only at concentrations similar to or higher than 100 mg L−1. At lower concentrations, there was no significant difference in the traction force on glass before and after walking on a treated surface (Fig. 3). In insects tested 24 h after walking on treated glass (untreated glass after 24 h), there was no significant difference in the traction force on untreated glass before and after walking on treated glass at any concentration investigated (Fig. 3) (6.5 mg L−1: F = 1.18; d.f. = 3, 29; p = 0.343. 12.5 mg L−1: F = 19.45; d.f. = 3, 25; p < 0.001. 25 mg L−1: F = 43.55; d.f. = 3, ×54; p < 0.001. 50 mg L−1: F = 45.48; d.f. = 3, 53; p < 0.001. 100 mg L−1: F = 34.93; d.f. = 3, 54; p < 0.001. 200 mg L−1: F = 45.77; d.f. = 3, 51; p < 0.001).
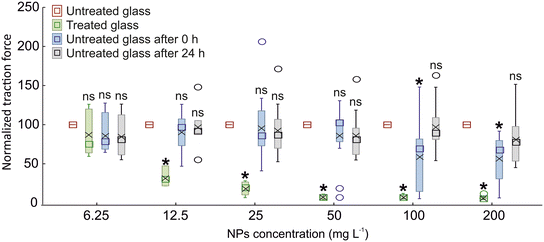 |
| Fig. 3 Normalized traction force obtained in traction force experiments with Nezara viridula pulling on untreated glass, treated glass at different non-biogenic ZnO-NP concentrations (mg L−1), untreated glass after 0 h, and untreated glass after 24 h. Boxplots show the interquartile range and the median (square), whiskers indicate the 1.5 × interquartile range, and X shows the arithmetic mean. Boxplots with an asterisk are significantly different from the untreated glass at p < 0.05, 1-way repeated measures ANOVA, Dunnett's method post hoc test. | |
The reduction in the traction force of N. viridula walking on glass treated with biogenic and non-biogenic Zn-NPs at different concentrations expressed as percentages in comparison with the traction force exerted by insects walking on untreated glass reached 70% at a concentration of 12.5 mg L−1 and remained high (around 90%) starting from a concentration of 50 mg L−1 (Fig. 4). The 50% inhibitory concentration (IC50) values for the concentration exhibiting antiadhesive properties related to insect attachment ability were very similar for biogenic and non-biogenic ZnO-NPs, and, in particular, it was 8.53 for biogenic ZnO-NPs and 9.03 for non-biogenic ZnO-NPs.
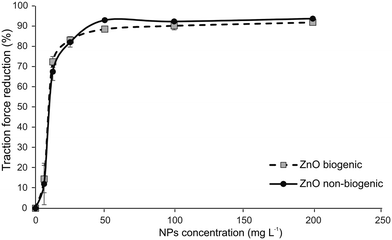 |
| Fig. 4 Reduction in the traction force of Nezara viridula walking on glass treated with different concentrations of biogenic and non-biogenic ZnO-NPs expressed as percentage in comparison with the traction force exerted on untreated glass. | |
The reduction of the traction force on treated surfaces (with a concentration of 50 mg L−1) was not significantly different during the duration of the traction force measurements both for biogenic (F = 2.45; d.f. = 3, 53; p = 0.0.79) and non-biogenic (F = 2.36; d.f. = 3, 45; p = 0.0.92) ZnO-NPs (Fig. 5). Moreover, in the first 60 s the traction forces recorded on biogenic (t = 8.79; d.f. = 13; p < 0.001) and non-biogenic (t = 10.62; d.f. = 12; p < 0.001) ZnO-NP treated surfaces were significantly lower than those recorded on untreated surfaces.
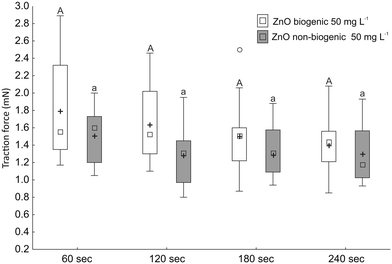 |
| Fig. 5 Traction force obtained every 60 s during the traction force experiments with Nezara viridula pulling on treated glass with ZnO-NPs at a concentration of 50 mg L−1. Boxplots show the interquartile range and the median (square), whiskers indicate the 1.5 × interquartile range, and X shows the arithmetic mean. Boxplots with the same letter are not significantly different at p < 0.05, 1-way repeated measures ANOVA. | |
The real distribution on the surface of NPs follows the increasing concentrations for both biogenic (F = 12.15; d.f. = 1, 5; p < 0.001), (Y = 0.1094 × X + 0.5736; P = 0.0001) and non-biogenic (F = 29.7; d.f. = 1, 5; p = 0.003), (Y = 0.1096 × X + 1.965; P = 0.0028) ZnO-NPs with a linear relationship (Fig. 6).
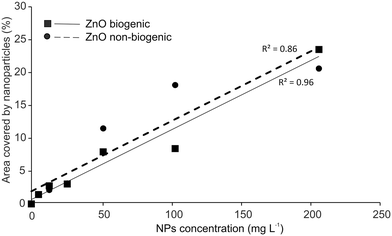 |
| Fig. 6 Linear relationship between the percentage of glass surface covered by NPs and the different tested NP concentrations. | |
The tarsal attachment organs of the adult of N. viridula are represented by smooth pulvilli, a pair of pretarsal claws and a basitarsal hairy pad. The latter bears on its ventral side a hairy pad with numerous adhesive setae (see ref. 38). The SEM observations of the tarsi of insects after they walked on glass treated with biogenic and non-biogenic ZnO-NPs reveal that at a concentration of 6.25 mg L−1 the attachment devices are not contaminated (Fig. 7a and f), while at a concentration of 100 mg L−1 both biogenic (Fig. 7b and d) and non-biogenic (Fig. 7g and i) ZnO-NPs aggregate on the distal portion of the claws, on the ventral surface of pulvilli and among the adhesive setae of the hairy pad. Furthermore, the EDX analysis confirms a high amount of Zn in these NPs (Fig. 7c, e, h and j).
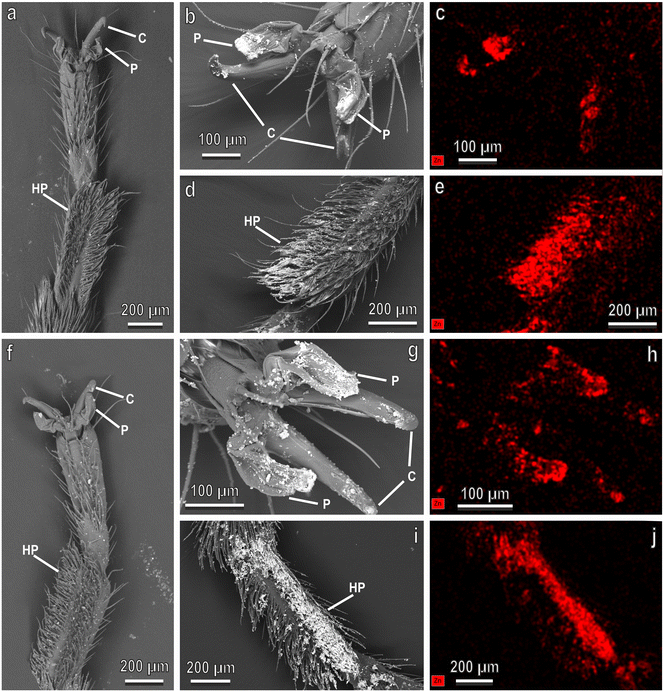 |
| Fig. 7 Ventral side of the tarsal attachment devices of Nezara viridula males represented by smooth pulvilli (P), a pair of pretarsal claws (C), and a basitarsal hairy pad (HP) just after they walked on glass treated with biogenic (a–e) and non-biogenic (f–j) ZnO-NPs at concentrations of 6.25 mg L−1 (a and f) and 100 mg L−1 (b–e and g–j), visualized with SEM (backscattered electrons) (a, b, d and f–i) and EDX analysis (c, e, h and j) highlighting in red the presence of Zn on the ventral surface of claws, pulvilli (c and h) and hairy pad (e and j). | |
Discussion
Concerning the need to overcome problems associated with conventional agriculture (pests, fertilizers, environmental pollution, phytopathogens, etc.), nanostructured materials are showing potential to become a new-age material. Some NPs can prompt substantial benefits in crops already at low concentrations, improving germination, photosynthesis, biomass production, chloroplast development and functionality and antioxidant defensive mechanisms.39–45 In this frame, zinc-based nanomaterials are attracting some interest, as this element is an essential micronutrient for plants.26 Recent studies have shown that ZnO-NPs can be used for treating crops, thus improving seed germination, plant vigor index, physiological and biochemical traits, and biomass production.42,46,47 In addition, the biogenic ZnO-NPs obtained from duckweed (the same used in the present study) revealed a beneficial effect in maize and olive tree explants.21,29 As for maize, the biogenic ZnO-NPs positively influenced the chlorophyll and carotenoid content and the cellular redox state, and stimulated biomass production. Concerning olive explants, ZnO-NPs stimulated the production of shoots, fresh and dry weight, and pigment and soluble protein contents, positively affecting total phenols and ROS scavenging activity.
The results presented in this study demonstrate that the attachment ability of the adult of N. viridula to glass surfaces can be significantly reduced by applying both biogenic and non-biogenic ZnO-NPs. The SEM images of the treated surfaces show that after the application, the biogenic ZnO-NPs maintained a spherical shape, according to ref. 21, but after drying, the nanostructured material tends to aggregate. The same happens for the non-biogenic ZnO-NPs, which showed a lamellar nanostructure but aggregation after the application and drying on the glass surface.
Furthermore, our SEM observations reveal that the reduction in attachment ability is due to the contamination of insect attachment devices with the ZnO-NPs. Indeed, the pulvilli and the hairy pad with adhesive setae of N. viridula are not contaminated at a concentration of 6.25 mg L−1 (not impairing the bug attachment ability), but at higher concentrations, both biogenic and non-biogenic ZnO-NPs aggregate on the distal portion of the claws, the ventral surface of pulvilli and among the adhesive setae of the hairy pad, interfering with adhesion of insects showing on their legs both smooth or hairy attachment devices. A similar effect in reducing insect attachment ability is induced by plant waxes, according to the contamination hypothesis.48,49 However, we cannot exclude some roles of ZnO-NPs in reducing insect adhesion owing to the absorption of the pad fluid due to the structured ZnO particles, as it happens for plant wax coverage.50
The concentration of both biogenic and non-biogenic ZnO-NPs required to significantly reduce the attachment ability of N. viridula to glass surfaces is around 12.5 mg L−1, and the reduction in attachment ability is very strong (about 70% reduction of the insect attachment ability), reaching 90% reduction of the initial force from a concentration of 50 mg L−1. Hypothesizing that N. viridula walks on an inclined plane, considering the maximum value of traction force recorded on treated surfaces at a concentration of 12.5 mg L−1, the insect is not able to attach to treated surfaces with an inclination ≥75° (α = arctan(F/m·g)). This means that ZnO-NP treatment can prevent the insect presence on different inclined natural surfaces such as leaves or fruits. Moreover, the reduction of the traction force on surfaces treated with ZnO-NPs was not different during 240 s of the traction test, thus suggesting that 1) attachment pad contamination is effective since the beginning of pad contact with the treated surface and does not increase during traction on treated glass surfaces and 2) insects cannot self-clean their attachment devices when walking on treated surfaces. The IC50 values for the concentration exhibiting antiadhesive properties related to insect attachment ability were very similar for biogenic and non-biogenic ZnO-NPs, indicating that the biogenic synthesis of the NPs did not significantly affect their anti-adhesive properties.
The results also show no difference in the insect traction force recorded on untreated glass after 0 and after 24 h, and the initial insect traction force exerted by insects pulling on untreated glass. This indicates that the ZnO-NPs do not have a residual effect on the insect attachment ability. This is probably due to the self-cleaning properties of the insect adhesive fluid, which plays an important role not only in increasing capillary forces allowing insect attachment ability51 but also in “washing-out” contaminating particles.52 Indeed measurements of the attachment ability of stick insects (smooth pads) and dock beetles (hairy pads) on glass after contamination with microspheres showed that insect pads recovered high levels of adhesion after only eight simulated steps.52 The only exception is represented by non-biogenic ZnO-NPs, which, starting from a concentration of 100 mg L−1, tend to significantly reduce the attachment ability of insects pulling on untreated glass after walking on treated glass. In any case, this reduction is no longer visible after 24 h. Such a slightly more persistent antiadhesive effect of the non-biogenic ZnO-NPs, compared to the biogenic NPs, is probably due to the different shapes of the NPs. Indeed, the lamellar shape of the non-biogenic NPs could be more difficult to remove from the insect attachment devices than the spherical ones typical of biogenic Zn-NPs.
In conclusion, this study suggests that biogenic and non-biogenic ZnO-NPs can be promising materials for developing anti-adhesive surfaces for insect control. Further studies are necessary to evaluate the antiadhesive properties of ZnO-NPs on natural surfaces such as plant leaves, where typical surface plant features such as waxes or trichomes can interfere with insect attachment ability34,53 and with the effectiveness of antiadhesive coatings as shown for kaolin particle films.9 We cannot also exclude the involvement of ZnO-NPs in insect oviposition and feeding deterrence or in reducing the insect survival rate and mating success, as highlighted for other nanoparticle films such as kaolin.54–63 Moreover, this study demonstrates that biogenic ZnO-NPs, synthesized using an extract from an invasive aquatic species, the duckweed, have potential as a non-toxic and environmentally friendly insect repellent. However, further studies are needed to evaluate their effectiveness against other insect species and to investigate their potential impacts on non-target organisms.
Ethical approval
This article does not contain any studies with human participants or animals (other than insects) performed by any of the authors.
Data availability
The datasets generated during and/or analysed during the current study are available from the corresponding author on reasonable request.
Author contributions
All authors conceived and designed the research. DD synthesized the NPs. MR and GS conducted the traction force experiments. MR and SP performed the SEM observations. GS analyzed the data. MR and DD wrote the manuscript. All authors read and approved the manuscript.
Conflicts of interest
The authors have no relevant financial or non-financial interests to disclose.
Acknowledgements
This work has been funded by the European Union – NextGenerationEU under the Italian Ministry of University and Research (MUR) National Innovation Ecosystem grant ECS00000041 – VITALITY. We acknowledge Università degli Studi di Perugia and MUR for support within the project Vitality. The authors are very grateful to Maddalena Lo Conte for her help in performing the experiments.
References
- S. H. Berlocher and J. L. Feder, Sympatric speciation in phytophagous insects: moving beyond controversy?, Annu. Rev. Entomol., 2002, 47, 773–815 CrossRef CAS PubMed.
- E.-C. Oerke, Crop losses to pests, J. Agric. Sci., 2006, 144, 31–43 CrossRef.
- J. E. Casida and G. B. Quistad, Golden age of insecticide research: past, present, or future?, Annu. Rev. Entomol., 1998, 43, 1–16 CrossRef CAS PubMed.
- D. Pimentel, Environmental and economic costs of the application of pesticides primarily in the United States, Environ. Dev. Sustain., 2005, 7, 229–252 CrossRef.
- D. A. Thompson, H. J. Lehmler, D. W. Kolpin, M. L. Hladik, J. D. Vargo, K. E. Schilling, G. H. LeFevre, T. L. Peeples, M. C. Poch, L. E. LaDuca, D. M. Cwiertny and R. W. Field, A critical review on the potential impacts of neonicotinoid insecticide use: current knowledge of environmental fate, toxicity, and implications for human health, Environ. Sci.: Processes Impacts, 2020, 22, 1315–1346 RSC.
- D. M. Glenn and G. J. Puterka, Particle films: A new technology for agriculture, Hortic. Rev., 2005, 31, 1–44 CAS.
- J. B. Bergmann, D. Moatsou, U. Steiner and B. Wilts, Bio-inspired materials to control and minimise insect attachment, Bioinspiration Biomimetics, 2022, 17, 051001 CrossRef PubMed.
- J. W. Todd, Ecology and behavior of Nezara viridula, Annu. Rev. Entomol., 1989, 34, 273–292 CrossRef.
- G. Salerno, M. Rebora, A. Kovalev, E. Gorb and S. Gorb, Kaolin nano-powder effect on insect attachment ability, J. Pest Sci., 2020, 93, 315–327 CrossRef.
- G. Salerno, M. Rebora, S. Piersanti, V. Saitta, A. Kovalev, E. Gorb and S. Gorb, Reduction in Insect Attachment Caused by Different Nanomaterials Used as Particle Films (Kaolin, Zeolite, Calcium Carbonate), Sustainability, 2021, 13, 8250 CrossRef CAS.
- W. Federle, M. Riehle, A. S. G. Curtis and R. J. Full, An integrative study of insect adhesion: Mechanics and wet adhesion of pretarsal pads in ants, Integr. Comp. Biol., 2002, 42, 1100–1106 CrossRef PubMed.
- L. Heepe, D. S. Petersen, L. Tölle, J. O. Wolff and S. N. Gorb, Sexual dimorphism in the attachment ability of the ladybird beetle Coccinella septempunctata on soft substrates, Appl. Phys. A: Mater. Sci. Process., 2017, 123, 34 CrossRef.
- C. Grohmann, A. Blankenstein, S. Koops and S. N. Gorb, Attachment of Galerucella nymphaeae (Coleoptera, Chrysomelidae) to surfaces with different surface energy, J. Exp. Biol., 2014, 217, 4213–4220 Search PubMed.
-
A. G. Peressadko and S. N. Gorb, Surface profile and friction force generated by insects, in First international industrial conference Bionik, ed. I. Boblan and R. Bannasch, Hannover Messe, Hannover, 2004 Search PubMed.
- P. Bußhardt, H. Wolfb and S. N. Gorb, Adhesive and frictional properties of tarsal attachment pads in two species of stick insects (Phasmatodea) with smooth and nubby euplantulae, Zoology, 2012, 115, 135–141 CrossRef PubMed.
- E. V. Gorb and S. N. Gorb, Attachment ability of females and males of the ladybird beetle Cryptolaemus montrouzieri to different artificial surfaces, J. Insect Physiol., 2020, 121, 104011 CrossRef CAS PubMed.
- M. Rebora, G. Salerno, S. Piersanti, E. V. Gorb and S. N. Gorb, Attachment devices and the tarsal gland of the bug Coreus marginatus (Hemiptera: Coreidae), Zoomorphology, 2021, 140, 85–102 CrossRef.
- G. Salerno, M. Rebora, S. Piersanti, E. Gorb and S. Gorb, Mechanical ecology of fruit-insect interaction in the adult Mediterranean fruit fly Ceratitis capitata (Diptera: Tephritidae), Zoology, 2020, 139, 125748 CrossRef PubMed.
- G. Salerno, M. Rebora, S. Piersanti, V. Saitta, E. Gorb and S. Gorb, Coleoptera claws and trichome interlocking, J. Comp. Physiol., A, 2023, 209, 299–312 CrossRef PubMed.
- J. Burack, S. N. Gorb and T. H. Büscher, Attachment Performance of Stick Insects (Phasmatodea) on Plant Leaves with Different Surface Characteristics, Insects, 2022, 13, 952 CrossRef PubMed.
- D. Del Buono, A. Di Michele, F. Costantino, M. Trevisan and L. Lucini, Biogenic ZnO Nanoparticles Synthesized Using a Novel Plant Extract: Application to Enhance Physiological and Biochemical Traits in Maize, Nanomaterials, 2021, 11, 1270 CrossRef CAS PubMed.
- V. Ghormade, M. V. Deshpande and K. M. Paknikar, Perspectives for nano-biotechnology enabled protection and nutrition of plants, Biotechnol. Adv., 2011, 29, 792–803 CrossRef CAS PubMed.
- C. G. Athanassiou, N. G. Kavallieratos, G. Benelli, D. Losic, P. Usha Rani and N. Desneux, Nanoparticles for pest control: Current status and future perspectives, J. Pest Sci., 2018, 91, 1–15 CrossRef.
- K. Smith, D. A. Evans and G. A. El-Hiti, Role of modern chemistry in sustainable arable crop protection, Philos. Trans. R. Soc., B, 2008, 363, 623–637 CrossRef CAS PubMed.
- G. Benelli, R. Pavela, F. Maggi, R. Petrelli and M. Nicoletti, Commentary: making green pesticides greener? the potential of plant products for nanosynthesis and pest control, J. Cluster Sci., 2017, 28, 3–10 CrossRef CAS.
-
G. Qazi and F. A. Dar, Nano-agrochemicals: Economic Potential and Future Trends, in Nanobiotechnology in Agriculture. Nanotechnology in the Life Sciences, ed. K. Hakeem and T. Pirzadah, Springer, Cham, 2020, pp. 185–193 Search PubMed.
- P. Goswami and J. Mathur, Positive and negative effects of nanoparticles on plants and their applications in agriculture, Plant Sci. Today, 2019, 6, 232–242 CrossRef CAS.
- R. G. Saratale, G. D. Saratale, H. S. Shin, J. M. Jacob, A. Pugazhendhi, M. Bhaisare and G. Kumar, New insights on the green synthesis of metallic nanoparticles using plant and waste biomaterials: current knowledge, their agricultural and environmental applications, Environ. Sci. Pollut. Res., 2018, 25, 10164–10183 CrossRef CAS PubMed.
- L. Regni, D. Del Buono, M. Micheli, S. L. Facchin, C. Tolisano and P. Proietti, Effects of Biogenic ZnO Nanoparticles on Growth, Physiological, Biochemical Traits and Antioxidants on Olive Tree In Vitro, Horticulturae, 2022, 8, 161 CrossRef.
- S. Ying, Z. Guan, P. C. Ofoegbu, P. Clubb, C. Rico, F. He and J. Hong, Green synthesis of nanoparticles: Current developments and limitations, Environ. Technol. Innovation, 2022, 26, 102336 CrossRef CAS.
- T. M. Rabalao, B. Ndaba, A. Roopnarain and B. Vatsha, Towards a circular economy: The influence of extraction methods on photosynthesis of metallic nanoparticles and their impact on crop growth and protection, JSFA Reports, 2022, 2, 208–221 CrossRef.
- S. Ahmed, S. A. Chaudhry and S. Ikram, A review on biogenic synthesis of ZnO nanoparticles using plant extracts and microbes: a prospect towards green chemistry, J. Photochem. Photobiol., B, 2017, 166, 272–284 CrossRef CAS PubMed.
- G. Salerno, M. Rebora, E. Gorb, A. Kovalev and S. Gorb, Attachment ability of the southern green stink bug Nezara viridula (Heteroptera: pentatomidae), J. Comp. Physiol., A, 2017, 203, 601–611 CrossRef PubMed.
- G. Salerno, M. Rebora, E. Gorb and S. Gorb, Attachment ability of the polyphagous bug Nezara viridula (Heteroptera: pentatomidae) to different host plant surfaces, Sci. Rep., 2018, 8, 10975 CrossRef PubMed.
- G. Salerno, M. Rebora, S. Piersanti, Y. Matsumura, E. Gorb and S. Gorb, Variation of attachment ability of Nezara viridula (Hemiptera: pentatomidae) during nymphal development and adult aging, J. Insect Physiol., 2020, 127, 104117 CrossRef CAS PubMed.
- C. A. Schneider, W. S. Rasband and K. W. Eliceiri, NIH Image to ImageJ: 25 years of image analysis, Nat. Methods, 2012, 9, 671–675 CrossRef CAS PubMed.
-
StatSoft Inc, Statistica (data analysis software system), version 6, StatSoft Italia S.R.L., Vigonza, 2001 Search PubMed.
- M. Rebora, J. Michels, G. Salerno, L. Heepe, E. Gorb and S. Gorb, Tarsal attachment devices of the southern green stink bug Nezara viridula (Heteroptera: pentatomidae), J. Morphol., 2018, 279, 660–672 CrossRef CAS PubMed.
- S. Ambika and M. Sundrarajan, Antibacterial Behaviour of Vitex negundo Extract Assisted ZnO Nanoparticles against Pathogenic Bacteria, J. Photochem. Photobiol., B, 2015, 146, 52–57 CrossRef CAS PubMed.
- P. Acharya, G. K. Jayaprakasha, K. M. Crosby, J. L. Jifon and B. S. Patil, Green-Synthesized Nanoparticles Enhanced Seedling Growth, Yield, and Quality of Onion (Allium cepa L.), ACS Sustainable Chem. Eng., 2019, 7, 14580–14590 CrossRef CAS.
- J. A. Mazumder, E. Khan, M. Perwez, M. Gupta, S. Kumar, K. Raza and M. Sardar, Exposure of Biosynthesized Nanoscale ZnO to Brassica juncea Crop Plant: Morphological, Biochemical and Molecular Aspects, Sci. Rep., 2020, 10, 8531 CrossRef CAS PubMed.
- L. Sun, Y. Wang, R. Wang, R. Wang, P. Zhang, Q. Ju and J. Xu, Physiological, Transcriptomic, and Metabolomic Analyses Reveal Zinc Oxide Nanoparticles Modulate Plant Growth in Tomato, Environ. Sci.: Nano, 2020, 7, 3587–3604 RSC.
- M. Adrees, Z. S. Khan, M. Hafeez, M. Rizwan, K. Hussain, M. Asrar, M. N. Alyemeni, L. Wijaya and S. Ali, Foliar Exposure of Zinc Oxide Nanoparticles Improved the Growth of Wheat (Triticum aestivum L.) and Decreased Cadmium Concentration in Grains under Simultaneous Cd and Water Deficient Stress, Ecotoxicol. Environ. Saf., 2021, 208, 111627 CrossRef CAS PubMed.
- N. Bilesky-José, C. Maruyama, T. Germano-Costa, E. Campos, L. Carvalho, R. Grillo, L. F. Fraceto and R. De Lima, Biogenic α-Fe2O3 Nanoparticles Enhance the Biological Activity of Trichoderma against the Plant Pathogen Sclerotinia sclerotiorum, ACS Sustainable Chem. Eng., 2021, 9, 1669–1683 CrossRef.
- C. Tolisano and D. Del Buono, Biobased: Biostimulants and biogenic nanoparticles enter the scene, Sci. Total Environ., 2023, 885, 163912 CrossRef CAS PubMed.
- D. Mishra, M. K. Chitara, S. Negi, J. Pal Singh, R. Kumar and P. Chaturvedi, Biosynthesis of Zinc Oxide Nanoparticles via Leaf Extracts of Catharanthus roseus (L.) G. Don and Their Application in Improving Seed Germination Potential and Seedling Vigor of Eleusine coracana (L.) Gaertn, Adv. Agric., 2023, 2023, 7412714 Search PubMed.
- V. N. Punitha, S. Vijayakumar, M. S. Alsalhi, S. Devanesan, M. Nilavukkarasi, E. Vidhya, S. P. Kumar and W. Kim, Biofabricated ZnO nanoparticles as vital components for agriculture revolutionization–a green approach, Appl. Nanosci., 2023 DOI:10.1007/s13204-023-02765-x.
- F. M. Borodich, E. V. Gorb and S. N. Gorb, Fracture behaviour of plant epicuticular wax crystals and its role in preventing insect attachment: A theoretical approach, Appl. Phys. A: Mater. Sci. Process., 2010, 100, 63–71 CrossRef CAS.
- E. V. Gorb and S. N. Gorb, Anti-adhesive effects of plant wax coverage on insect attachment, J. Exp. Bot., 2017, 68, 5323–5337 CrossRef CAS PubMed.
- E. V. Gorb, P. Hofmann, A. E. Filippov and S. N. Gorb, Oil adsorption ability of three dimensional epicuticular wax coverages in plants, Sci. Rep., 2017, 7, 45483 CrossRef CAS PubMed.
- J. H. Dirks and W. Federle, Fluid-based adhesion in insects – principles and challenges, Soft Matter, 2011, 7, 11047–11053 RSC.
- C. J. Clemente, J. M. R. Bullock, A. Beale and W. Federle, Evidence for self-cleaning in fluid-based smooth and hairy adhesive systems of insects, J. Exp. Biol., 2010, 213, 635–642 CrossRef PubMed.
-
E. V. Gorb and S. N. Gorb, Anti-adhesive surfaces in plants and their biomimetic potential, in Materials design inspired by nature: Function through inner architecture, ed. P. Fratzl, J. W. C. Dunlop and R. Weinkamer, RSC Smart Materials, The Royal Society of Chemistry, 2013, vol. 4, pp. 282–309 Search PubMed.
- A. L. Knight, T. R. Unruh, B. A. Christianson, G. J. Puterka and D. M. Glenn, Effects of kaolin-based particle films on oblique banded leafroller, Choristoneura rosaceana (Harris) (Lepidoptera Tortricidae), J. Econ. Entomol., 2000, 93, 744–749 CrossRef CAS PubMed.
- T. R. Unruh, A. L. Knight, J. Upton, D. M. Glenn and G. J. Puterka, Particle films for suppression of the codling moth (Lepidoptera: Tortricidae) in apple and pear orchards, J. Econ. Entomol., 2000, 93, 737–743 CrossRef CAS PubMed.
- T. E. Cottrell, B. W. Wood and C. C. Reilly, Particle film affects black pecan aphid (Homoptera: Aphididae) on pecan, J. Econ. Entomol., 2002, 95, 782–788 CrossRef PubMed.
- G. J. Puterka, D. M. Glenn and R. C. Pluta, Action of particle films on the biology and behavior of pear psylla (Homoptera: Psyllidae), J. Econ. Entomol., 2005, 98, 2079–2088 CrossRef PubMed.
- J. E. Barker, A. Fulton, K. A. Evans and G. Powel, The effects of kaolin particle film on Plutella xylostella behaviour and development, Pest Manage. Sci., 2006, 62, 498–504 CrossRef CAS PubMed.
- S. L. Lapointe, C. L. Mckenzie and D. G. Hall, Reduced oviposition by Diaprepes abbreviatus (Coleoptera: Curculionidae) and growth enhancement of citrus by Surround particle film, J. Econ. Entomol., 2006, 99, 109–116 CrossRef CAS PubMed.
- N. J. Bostanian and G. Racette, Particle film for managing arthropod pests, J. Econ. Entomol., 2008, 101, 145–150 CrossRef CAS PubMed.
- F. Tacoli, N. Mori, A. Pozzebon, E. Cargnus, S. Da Vià, P. Zandigiacomo, C. Duso and F. Pavan, Control of Scaphoideus titanus with natural products in organic vineyards, Insects, 2017, 8, 1–10 CrossRef PubMed.
- F. Tacoli, F. Pavan, E. Cargnus, E. Tilatti, A. Pozzebon and P. Zandigiacomo, Efficacy and mode of action of kaolin in the control of Empoasca vitis and Zygina rhamni (Hemiptera: Cicadellide) in vineyards, J. Econ. Entomol., 2017, 110, 1164–1178 CrossRef PubMed.
- F. Tacoli, E. Cargnus, F. Kiaeian Moosavi, P. Zandigiacomo and F. Pavan, Efficacy and mode of action of kaolin and its interaction with bunch-zone leaf removal against Lobesia botrana on grape-vines, J. Pest Sci., 2019, 92, 465–475 CrossRef.
|
This journal is © The Royal Society of Chemistry 2023 |
Click here to see how this site uses Cookies. View our privacy policy here.