DOI:
10.1039/D1PY01645H
(Minireview)
Polym. Chem., 2022,
13, 1648-1657
Chemical design and synthesis of macromolecular profluorescent nitroxide systems as self-reporting probes
Received
11th December 2021
, Accepted 4th February 2022
First published on 10th March 2022
Abstract
The objective of this mini-review article is to highlight derivatives of polymeric profluorescent nitroxides. Importantly, the limitations that restrict expansion of this class of polymers are discussed. The challenges of harsh conditions that lead to undesired side-reactions during polymerization or the necessity of orthogonal post–modification reactions are reviewed. Importantly, I discuss the challenges within this field, and suggest strategies for the advancement of polymeric profluorescent nitroxide derivatives.
1. Introduction
Material scientists like to highlight the numerous opportunities that exist for further development of materials. This approach is especially true for development of ‘smart’ polymeric structures that allows access to adaptive/responsive materials with self-healing, shape memory, or actuation behaviour. Indeed, scientists can make all types of material on-scale with controllable and remarkable properties. However, designing materials with autonomous self-reporting properties is much more challenging. Hence, self-reporting systems have been investigated exhaustively, with a vast range of applications: self-reporting aggregation of polymers1,2 over-healing and degradation of dynamic materials,3–6 drug delivery systems7 and in vitro biosensors8 amongst others. Predominantly, fluorescent probes have become indispensable tools for self-reporting imaging and analytics because of their non-invasive nature. Conversely, apart from permanently fluorescent labels, it is highly desirable to generate an emissive fluorophore from a non-fluorescent labelling agent (i.e., a latent fluorophore or profluorophore) upon completion of a targeted reaction. The use of a profluorophore gives a clear indication that a fluorescent labelling has been achieved at the desired location because an OFF-to-ON change in emission has occured. For instance, O'Reilly and coworkers have employed dibromomaleimide monomers to prepare profluorescent polymers. The latter had demonstrated chemico-fluorescent responsiveness mediated by thiol conjugation, which resulted in a switch of fluorescence emission.2,9 In another elegant work, Nolte and colleagues showed that profluorescent 3-azido coumarin-terminated polymers could undergo a reaction with an alkyne-functionalized protein to produce a strongly fluorescent triazole-linked conjugate.10 Of particular interest was the cleavage of a quenching ‘trimethyl lock’ from a rhodamine functional polymer by intracellular esterases to generate an OFF-to-ON change in emission.11 An intrinsically metal-free profluorescent stable polymeric system was developed with the excellent properties of polymeric micelles for drug delivery, moreover the drug carrier could be monitored over an extended period of time without requiring complex core–shell particles to protect the potentially toxic payload. In similar manner, the fluorescence emission can also be triggered by virtue of the labelling reaction, which is also the fluorophore-forming reaction (i.e., two non-fluorescent groups react to form a fluorophore). These are rarer examples because the afore-mentioned approach requires a reaction that generates a fluorophore which is supposed to be highly efficient. By all manner of means, the tetrazole-alkene/azirine-alkene cycloaddition results in emissive products (e.g., surfaces, single-chain nanoparticles), as demonstrated by Barner-Kowollik and colleagues, and thus the reported system has fulfilled the criteria for self-reporting behaviour.12–14
In an effort to design such self-reporting materials, interest has been drawn to the unique properties of paramagnetic nitroxides (Scheme 1).15 These stable free radicals quench the excited states of a fluorophore closely linked to the nitroxide moiety in a wide variety of non-covalent and flexibly tethered covalent systems. Therefore, a nitroxide tethered to a fluorophore is called a ‘profluorescent nitroxide’ because the fluorescence is suppressed until the radical moiety is trapped or undergoes redox chemistry to form a diamagnetic species. An in-depth literature survey has revealed the existence of various profluorescent nitroxide derivates15 which could be ‘switched on’ by converting the paramagnetic nitroxides into diamagnetic species (i.e., hydroxylamine) at variable rates in response to physiological reducing (e.g., ascorbate or ascorbate/glutathione)-, oxidizing- or radical-trapping agents, and a 2- to 3.5-fold increase in fluorescence emission was observed.15 Typically, these nitroxides show minimal toxicity in comparison with metal-based magnetic resonance imaging (MRI) contrast agents, which face critical toxicity concerns.3,16 Hence, this feature has been exploited for selective metal-free MRI of redox processes in vivo17–19 and in vitro20–24 along for analysis of oxidation-induced polymer degradation.25,26 Furthermore, depending on the chromophore moiety present within the profluorescent nitroxide species, the absorption and emission wavelengths of these probes can be tuned to match other specific fields of research and clinical applications.
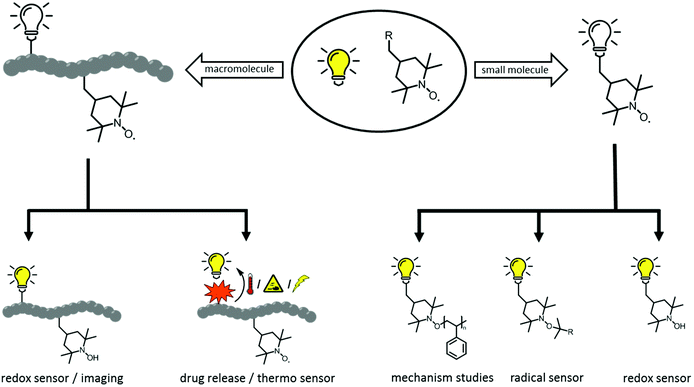 |
| Scheme 1 Versatile applications of small molecules and polymeric profluorescent nitroxide sensors. | |
Accordingly, this new area of profluorescent nitroxide sensors in which nitroxide radicals and fluorophores are covalently bound together (refer to Scheme 2) is of considerable interest. Responsive fluorescence appears to aid the design of advanced imaging probes and drug-delivery platforms that report their activation by altering the respective fluorescence.27 Unfortunately, small-molecule profluorescent nitroxide derivatives face disadvantages as a result of their: (i) low relaxivity compared with that of metals;25 (ii) very rapid in vivo reduction (in the order of minutes for most nitroxides).28–31 Nevertheless, nanostructured self-reporting agents that carry multiple reduction-resistant nitroxides could overcome these limitations.32–34
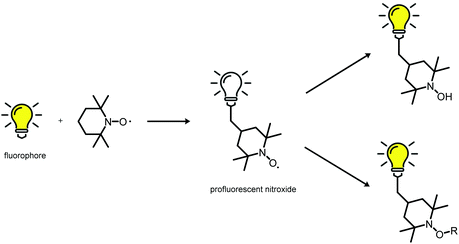 |
| Scheme 2 Fluorescence quenching and its restoration (schematic).15 | |
Indeed, the highly tuneable nature of the heterocyclic scaffold, the ease of introduction of spin labelling ‘handles’, and the relatively localised nature of the unpaired electron have made nitroxide radicals a research ‘hotspot’.35 Blinco et al.36 has reviewed in detail the chemistry and properties of nitroxides in general. They have also summarized the synthesis of the respective nitroxide radical polymers by reporting on the historical approach, the latest cutting-edge synthetic breakthroughs, and highlighting the most impactful applications. Hence, within the present mini-review, it is not aimed to catalogue the topics mentioned above, but instead it is targeted to describe the paradigm shifts in the development of functional profluorescent nitroxide macromolecular scaffolds applicable in biomedicine and material science. As such, the focus lies on the exciting and recently published works that address some of the most important problems that hinder the progress in the development of functional self-reporting profluorescent polymeric materials based on nitroxide derivatives. The examples within this review have been selected to show what is synthetically possible. Accordingly, it is first discussed how to include directly profluorescent nitroxide derivatives in a polymeric backbone. Next, the importance of ‘tailored’ polymers which fulfil a wide range of applications by adjustment of the composition, structure, as well as inherent chemical and physical properties, is discussed. Finally, a perspective on the future research towards the synthesis of profluorescent nitroxide-based polymer derivatives from fundamental and application perspectives is provided.
2. Macromolecular profluorescent nitroxides and their applications as self-reporting probes
The divide between ‘pure’ synthetic and ‘natural’ macromolecules continues to narrow in the pursuit of biologically relevant materials with increasingly complex functionality. Recent developments in biosynthetic polymer chemistry are allowing scientists to prepare, analyse and use polymeric systems in unprecedented ways. Refinements in controlled polymerization methods and bioconjugation strategies have enabled chemists to prepare ‘bespoke’ multifunctional materials with designed architectures. Critically, polymeric profluorescent systems are relatively less-investigated, but are quite an attractive subject of recent research.37,38 Intriguingly, a recent literature survey indicated that the potential of macromolecular nitroxide derivatives as new functional handles for use as self-reporting probes has not been reviewed critically. The several examples presented here, which are synthetically challenging, prompt initiatives for modifying the existing methods to develop simple and streamlined protocols.
2.1. Design of profluorescent nitroxide polymers via direct polymerization of functionalized monomers
A straightforward approach is to introduce the fluorophore and nitroxides directly through different functionalized monomers. This strategy adds the advantage of tuneable properties (e.g., solubility, biocompatibility and different topologies) thanks to addition of different comonomers.39 Nitroxide radicals are non-orthogonal to any radical polymerization process.40 Hence, they can be introduced directly only via metathesis polymerization, specifically ring-opening metathesis polymerization (ROMP), as revelaed in the examples shown below. Conversely, controlled radical-polymerization methods are usually are usually implemented for the polymerization of protected nitroxide species, or by employing suitable secondary amine monomers and subsequent oxidation by meta chloroperbenzoic acid (mCPBA), which is discussed in a separate sub-chapter.
Knall et al. employed ROMP to copolymerize a perylene bisimide-based norbornene with norbornenes bearing paramagnetic nitroxides and tertiary amines (acting as photoinduced electron transfer (PET) quenchers) to yield macromolecular PET probes and profluorescent polymers.39 The water solubility of the system was ensured by addition of oligo(ethylene glycol)-bearing monomers to create biocompatible sensors for concentrations of ascorbic acid down to 0.1 × 10−3 M (Scheme 3). By producing different polymer topologies, they also found out that the highest efficiency for quenching was when the fluorophore and nitroxide were covalently tethered within the same block in the closest proximity.39
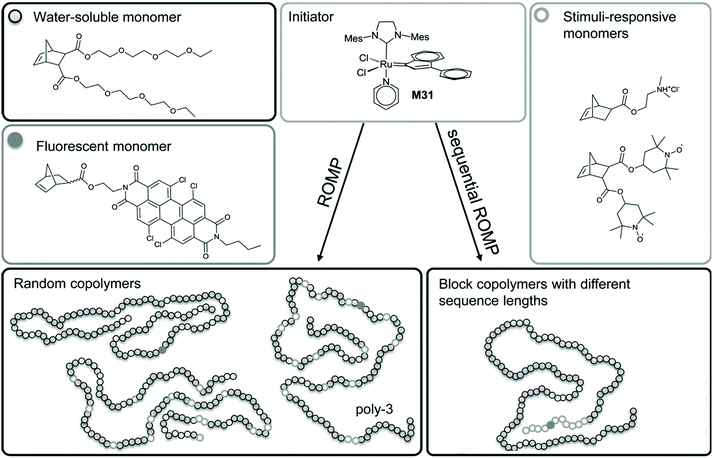 |
| Scheme 3 Synthetic strategy for water-soluble profluorescent nitroxide homopolymers, random copolymers and block copolymers. Reproduced with permission from ref. 39. Copyright, Wiley–VCH, 2013. | |
An intriguing example of a polymeric profluorescent nitroxide derivative with high topological complexity was demonstrated by Johnson and colleagues.41 They designed a redox-responsive branched-bottlebrush polymer for fluorescence imaging and MRI. Water-soluble nitroxide- and fluorophore-bearing monomers were polymerized, respectively, via ROMP. The employed fluorophore emitted near-infrared (NIR) light that could be used for NIR optical imaging (Scheme 4). The nitroxide derivatives partly acted as fluorescence quenchers and MRI contrast agents because they could alter the nuclear relaxation times of endogenous protons.42 Indeed, the former examples of functionalized polymers acted as contrast agents using only nitroxide radicals.8,42–45 Hence, the dual-sensor method employed in that work has not been known previously. The fluorescence quenching by nitroxides in the system decreased over time due to in vitro redox processes, which could therefore be observed as enhanced fluorescence emission and a decreased MRI contrast.41 Thus, entirely organic MRI systems garnered substantial interest due to their non-toxicity and biocompatibility compared with their metal–organic counterparts.16,46,47
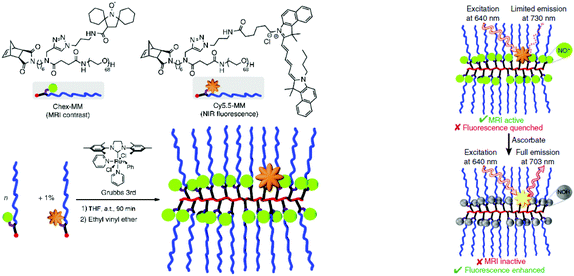 |
| Scheme 4 Structure of branched bottlebrush polymers bearing nitroxides and fluorophores (left). Concept of MRI and NIR imaging (right). Reproduced with permission from ref. 41. Copyright, Nature Publishing Group, 2014. | |
2.2. Design of profluorescent nitroxide polymers by post-polymerization functionalization
Post-polymerization modifications are highly versatile methods for the functionalization and modification of macromolecules. Accordingly, without changing the polymer backbone, the tailor-made manipulation of polymer properties is accomplished. In this regard, nitroxide-containing polymers have been prepared by polymerizing precursor monomers, mainly decorated with secondary amine groups, which were then oxidized into nitroxide radicals with a suitable oxidant (e.g., mCPBA). By adopting this strategy (i.e., conventional oxidative post-polymerization functionalization), profluorescent nitroxide polymers have been applied as a thermally triggered, self-reporting delivery system.48 Quite recently, our research team has incorporated thermo-releasable pyrene units in the polymer backbone by the copolymerization of 2,2-6,6-tetramethyl piperidine methacrylate (TMPM) with a tailor-made chromophore (i.e., pyrene-decorated monomer), with the ability to undergo hetero Diels–Alder chemistry. The nitroxide radicals were generated by the oxidative post-polymerization functionalization of TMPM via mCPBA, whereby pyrene fluorescence emission was quenched. In a final step, through the thermally triggered release of the pyrene, the nitroxide moieties in the resulting copolymer were of a sufficient distance to prevent a fluorescence quenching effect, and hence the fluorescence emission was restored (Scheme 5). The quenching and restoration of pyrene fluorescence was observed with the naked eye upon excitation by ultraviolet light. Therefore, this system can be employed as a sensor for the thermal field and as a self-reporting release system for fluorescent drug molecules (e.g., doxorubicin).49
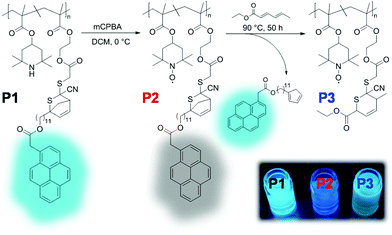 |
| Scheme 5 Synthetic strategy for preparation of a thermally triggered, self-reporting release system. Fluorescence restoration can be seen with the naked eye upon excitation by ultraviolet light (344 nm). Figure reproduced with permission from ref. 48. Copyright, Royal Society of Chemistry, 2017. | |
Another example, in which conventional oxidative post-polymerization functionalization is utilized, was highlighted by Blinco and colleagues. They compared the quenching efficiency and reducing behaviour of small-molecule profluorescent nitroxides and their macromolecular equivalents (Scheme 6).38 The synthesis of the small molecule was straightforward, but the nitroxide could not be introduced directly in the polymer backbone because the radical species would have interfered with the controlled radical polymerization that was employed. Therefore, the polymer was generated by polymerizing 2,2-6,6-tetramethyl piperidinyl methacrylate (TMPM) via atom-transfer radical polymerization (ATRP) and subsequent oxidation of the amine functional group using mCPBA.
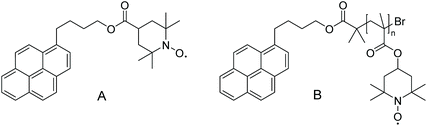 |
| Scheme 6 Molecular structure of the small-molecule profluorescent nitroxide (A) and macromolecular equivalent (B).38 | |
The performed photophysical investigations revealed a much higher quenching efficiency of the macromolecular probe compared with the small molecule, due to the high density of nitroxide moieties surrounding the fluorophore.42 Particularly, small-molecule fluorophores and nitroxides that were not covalently connected were mixed, and their quenching efficiency was compared with that of macromolecular profluorescent nitroxides. It was shown that profluorescent nitroxides in which the functional groups were bound together covalently provide efficient fluorescence quenching and thereby act as sensing probes.38
Post-polymerization modification of activated ester-containing polymers has emerged as an alternative and versatile route to equip polymers with numerous functional groups, including stable radicals. To meet the demand for construction of an efficient profluorescent nitroxide-based polymer, in 1993 Winnik et al. reported the first example of this type of post-polymerization approach by a substitution reaction of polymers derived from N-acryloxysuccinimide (NAS) monomers.50 Thus, as one of the earliest polymeric profluorescent nitroxides, pyrene- and nitroxide-bearing poly-(N-isopropylacrylamide) was designed in order to investigate the respective temperature dependent phase transition mechanism (Scheme 7).
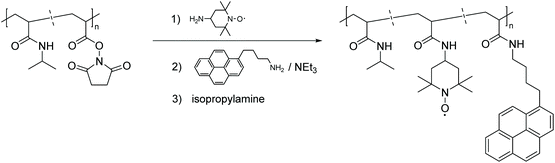 |
| Scheme 7 Synthetic strategy for implementation of nitroxide based radicals and a pyrene moiety in the PNIPAM backbone.50 | |
Implicitly, it was discovered that the polymer created intramolecular coils when dissolved in water, from which the more hydrophilic nitroxide moiety was excluded, in turn this resulted in lower quenching efficiency. When the polymer was dissolved in methanol, the hydrophobic groups did not aggregate, and the macromolecule became more flexible, allowing the pyrene and nitroxide radicals to interact. This assumption was confirmed by fluorescence measurements showing effective fluorescence quenching. The experiments also disclosed that as the aqueous solutions reached their lower critical solution temperature (LCST), the macromolecules coiled from expanded polymer chains into globules. Such collapsing behaviour disrupts the hydrophobic microdomains and increases the local concentration of isolated pyrenes and nitroxide groups, thereby enhancing the fluorescence quenching efficiency.50
In another example, Blinco et al. implemented a modular design towards macromolecular profluorescent nitroxides by employing orthogonal post-polymerization functionalization of polymeric precursors.37 In this approach, a glycidyl methacrylate derivative, which upon an oxirane ring-opening reaction employing sodium azide generated azide and alcohol functionalities in close proximity, was polymerized. By employing copper azide alkyne cycloaddition (CuAAC)-esterification reactions in a sequential manner, a fluorophore (e.g., 1-naphthalene and 9-anthracene) and nitroxide moiety (i.e., 4-carboxy-2,2,6,6-tetramethylpiperidine-N-oxyl (carboxy-TEMPO)) were installed independently in close proximity. Hence, a profluorescent polymeric material with unique properties and sensor advantages was obtained (Scheme 8).
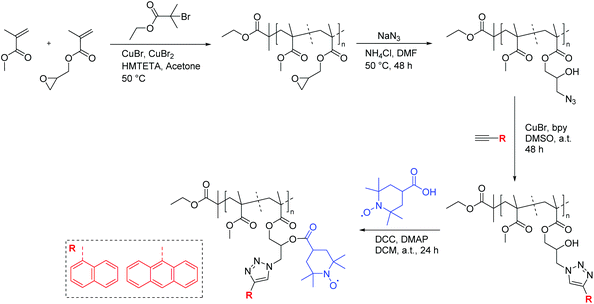 |
| Scheme 8 Synthetic strategy to obtain profluorescent nitroxide polymers by sequential post-polymerization functionalization reactions (i.e., CuAAC-esterification reactions).37 | |
Intriguingly, it was revealed that the specified polymer derivatives showed inherent sensing characteristics similar to those of their small-molecule counterparts to give rapid and efficient reactions, either with a reductant or with a radical source, to generate diamagnetic products. For instance, a polymer decorated with an anthracene chromophore and nitroxide moiety (Fig. 1) was shown to be an efficient sensor for carbon-centred free radicals. Accordingly, a degassed polymer solution with 2.5 eq. of azobis(isobutyronitril) (AIBN) was heated to 100 °C, and the fluorescence intensity was measured. A steady increase in fluorescence was observed that continued to increase until a maximum was reached after 180 min. Moreover, the comparison with an optically matched sample of the polymer revealed the fluorescence to be ∼89% of what would be expected from the parent fluorophore attached to the polymer backbone.37
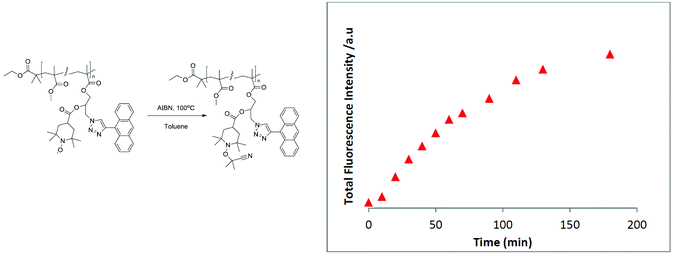 |
| Fig. 1 Evolution of total fluorescence over time when a polymer decorated with an anthracene chromophore and nitroxide moiety is heated at 100 °C in a degassed solution of toluene containing 2.5 equivalents of AIBN. Figure reproduced with permission from ref. 37. Copyright, Royal Society of Chemistry, 2015. | |
Recently, a profluorescent nitroxide probe based on the fluorescence quenching of doxorubicin in combination with a pH-labile hydrazide group was established as a pH-sensitive, self-reporting drug-release system.51 A biocompatible polymer based on tert-butyloxycarbonyl-hydrazidomethacrylate (bHMA), triethyleneglycol methacrylate (TEGMA) and tetramethylpiperidinyl methacrylate (TMPM) was synthesized via reversible addition–fragmentation transfer (RAFT) polymerization. Upon oxidation of the amine functional group, doxorubicin was incorporated in the polymer backbone. To investigate pH-sensitive release, glacial acetic acid was added to the reaction solution and the fluorescence was monitored over 7 days (Fig. 2). A very rapid release in the first 8 h and a continuous increase in the fluorescence of 50% was observed. The authors established a simple building block for nanocarrier systems whereby release could be visualized readily by fluorescence turn-on.
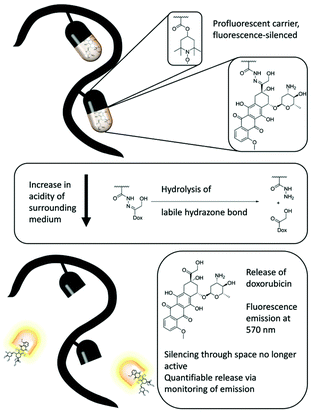 |
| Fig. 2 The concept of a polymeric profluorescent system that combines stable nitroxide radicals and doxorubicin and can effectively bind, release and report cancer therapeutics in fluorescence experiments. Figure reproduced with permission from ref. 51. Copyright, Royal Society of Chemistry, 2018. | |
Another self-reporting, profluorescent nitroxide polymer system was designed via a multi-component post-polymerization functionalization (i.e., the Ugi multicomponent reaction). This reaction was used to tether nitroxide (i.e., TEMPO) and a light-cleavable pyrene moiety in direct proximity to result in efficient fluorescence quenching (Fig. 3A and B).52 The self-reporting polymer scaffolds with profluorescent pyrene moieties reacted upon mild irradiation with visible light to undergo Norrish II elimination and release the acetylpyrene byproduct as a profluorescent reporting probe. This visible light-induced molecular release was monitored with the naked eye in a real time (Fig. 3C).
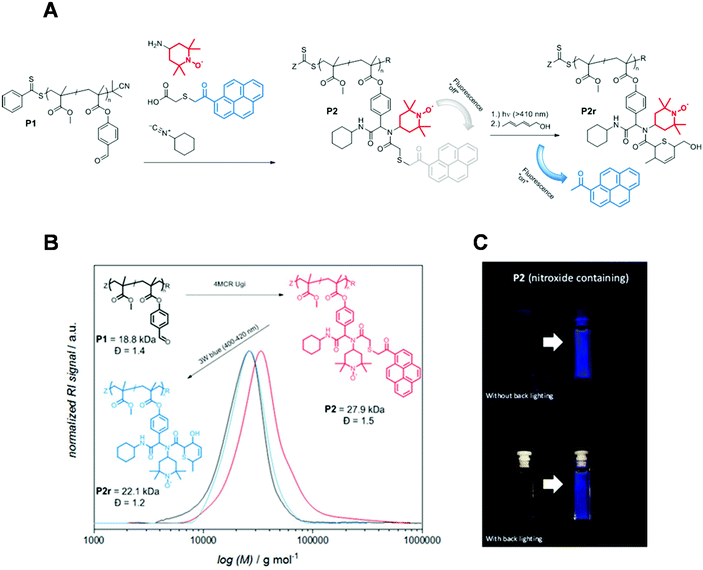 |
| Fig. 3 (A) 4-Amino TEMPO spin-silencing of the pyrene fluorophore upon multi-component polymerization functionalization of P1 to deliver P2 (schematic). Upon visible-light activation of P2, acetyl pyrene is released via a Norrish type-II mechanism to synthesize P2 accompanied with fluorescence activation. (B) SEC elugram demonstrating a shift to higher molecular weight upon multi-component post-polymerization ligation, followed by a shift to formation of a lower-molecular-weight polymer upon light-induced release of the acetyl pyrene derivative. (C) Photograph before and after irradiation of P2 demonstrating the fluorescence turn-on. Reproduced with permission from ref. 52. Copyright, Wiley–VCH, 2018. | |
2.3. Design of a profluorescent nitroxide polymer by electrostatic interaction
Tang and colleagues utilized an uncommon approach in which an anionic conjugated polymer was complexed with a cationic nitroxide derivative to create a profluorescent nitroxide sensor for the detection of free radicals or antioxidants.53 In other words, the anionic conjugated polymer poly{1,4-phenylene[9,9-bis(4-phenoxybutylsulfonate)]fluorene-2,7-diyl} (PFP-SO3−) and the nitroxide derivative (4-(trimethylammonium)-2,2,6,6-tetramethylpiperidine-1-oxyl iodide), CAT 1, formed complexes by electrostatic interactions. The fluorescence emission was quenched efficiently through a charge-transfer processes. Triggered either by the hydrogen abstraction from (±)-6-hydroxy-2,5,7,8-tetramethylchromane-2-carboxylic acid (trolox) or by the reduction process in the presence of antioxidants, such as ascorbic acid, the transformation of the paramagnetic nitroxide radical into diamagnetic hydroxylamine reversed the quenching. Therefore, the fluorescence of the anionic conjugated polymers was recovered (Scheme 9). This study clearly revealed that not all synthesized macromolecules can form complexes with the respective cation. Nevertheless, the quenching efficiency was sufficiently strong to enable the detection of antioxidants at a very low concentration (down to 50 nM).53
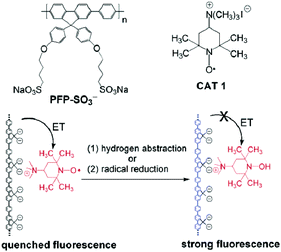 |
| Scheme 9 Visualization of the conjugated anionic polymer PFP-SO3−, cationic nitroxide derivative CAT 1, and the sensor mechanism employed in the design of a profluorescent nitroxide polymer by electrostatic interaction. Reproduced with permission from ref. 53. Copyright, American Chemical Society, 2006. | |
3. Conclusion and perspectives
The importance of profluorescent nitroxides (particularly polymeric profluorescent nitroxides) as versatile sensor materials in materials science is highlighted. Thus, a survey of previously reported polymeric profluorescent nitroxides has been provided. By using specific examples, it was shown that, due to the vast range of synthetic scopes of polymeric scaffolds, the biocompatibility of the profluorescent nitroxide polymer derivatives could be readily adjusted. Importnatly, with additional particular examples, it was emphasized that polymeric nitroxide derivatives appear to be excellent materials for biomedical purposes, such as dual-imaging techniques for MRI and self-reporting delivery systems.
Synthetically, future focus could involve creating a library of fluorophore and nitroxide monomers. If the design of a profluorescent nitroxide probe becomes completely modular, then simple interchange of the fluorophore and nitroxide units employing different monomer structures could be achieved to produce an ‘ideal’ probe tailored for each analytical purpose. Nevertheless, monomers decorated with nitroxide moieties require non-reactive covalent or non-covalent linkages to ensure that the observed fluorescence occurs through the transformation of the radical to paramagnetic species. In addition, diverse macromolecular topologies should be investigated by combining a certain monomer structure and topological configuration to ascertain optimal fitting sensors for the targeted application.
The range of applications could be extended to self-reporting dynamic systems (e.g., self-healing materials and nanoparticle formation) by employing nitroxide radical coupling reactions. Recently, the principle of profluorescent self-reporting systems has been applied to refoldable single-chain polymer nanoparticles via ultraviolet light-induced photo-click nitroxide radical coupling (folding) and mCPBA-mediated alkoxyamine fission (unfolding).54 As aforementioned, the medical applications of profluorescent self-reporting systems are highly promising. Hence, more effort can be directed in the development of novel release (e.g., pH- or light-triggered) systems in combination with profluorescent nitroxides. In summary, it could be postulated that multidisciplinary approaches and collaborations with neighbouring research fields will aid the development of more sophisticated self-reporting probes based on polymeric profluorescent nitroxide derivatives.
Conflicts of interest
There are no conflicts to declare.
Acknowledgements
I thank Professors P. Theato (KIT) and C. Barner-Kowollik (QUT) for their continued mentorship. I am grateful to C. W. Schmitt for the fruitful discussions on the topic.
H. M. acknowledges continued support from the Karlsruhe Institute of Technology (KIT).
This paper is dedicated to Professor Yusuf Yagci on the occasion of his 70th birthday.
References
- A. Noel, Y. P. Borguet and K. L. Wooley, Self-Reporting Degradable Fluorescent Grafted Copolymer Micelles Derived from Biorenewable Resources, ACS Macro Lett., 2015, 4, 645–650 CrossRef CAS PubMed.
- M. P. Robin, S. A. M. Osborne, Z. Pikramenou, J. E. Raymond and R. K. O'Reilly, Fluorescent Block Copolymer Micelles That Can Self-Report on Their Assembly and Small Molecule Encapsulation, Macromolecules, 2016, 49, 653–662 CrossRef CAS PubMed.
- K. Makyła, C. Müller, S. Lörcher, T. Winkler, M. G. Nussbaumer, M. Eder and N. Bruns, Fluorescent Protein Senses and Reports Mechanical Damage in Glass-Fiber-Reinforced Polymer Composites, Adv. Mater., 2013, 25, 2701–2706 CrossRef PubMed.
- A. M. Schenzel, N. Moszner and C. Barner-Kowollik, Self-Reporting Dynamic Covalent Polycarbonate Networks, Polym. Chem., 2017, 8, 414–420 RSC.
- N. Bruns and D. S. Clark, Self-Reporting Materials: Protein- Mediated Visual Indication of Damage in a Bulk Polymer, CHIMIA, 2011, 65, 245–249 CrossRef CAS PubMed.
- A. M. Schenzel, C. Klein, K. Rist, N. Moszner and C. Barner-Kowollik, Reversing Adhesion: A Triggered Release Self–Reporting Adhesive, Adv. Sci., 2016, 3, 1500361 CrossRef PubMed.
- M. P. Robin, A. B. Mabire, J. C. Damborsky, E. S. Thom, U. H. Winzer-Serhan, J. E. Raymond and R. K. O'Reilly, New Functional Handle for Use as a Self-Reporting Contrast and Delivery Agent in Nanomedicine, J. Am. Chem. Soc., 2013, 135, 9518–9524 CrossRef CAS PubMed.
- G. Rong, S. R. Corrie and H. A. Clark, In Vivo Biosensing: Progress and Perspectives, ACS Sens., 2017, 2, 327–338 CrossRef CAS PubMed.
- M. P. Robin, P. Wilson, A. B. Mabire, J. K. Kiviaho, J. E. Raymond, D. M. Haddleton and R. K. O′reilly, Conjugation-Induced Fluorescent Labeling of Proteins and Polymers Using Dithiomaleimides, J. Am. Chem. Soc., 2013, 135, 2875–2878 CrossRef CAS PubMed.
- J. Dirks, J. J. L. M. Cornelissen and R. J. M. Nolte, Monitoring Protein-Polymer Conjugation by a Fluorogenic Cu(I)-Catalyzed Azide-Alkyne 1,3-Dipolar Cycloaddition, Bioconjugate Chem., 2009, 20, 1129–1138 CrossRef PubMed.
- S. L. Mangold, R. T. Carpenter and L. L. Kiessling, Synthesis of Fluorogenic Polymers for Visualizing Cellular Internalization, Org. Lett., 2008, 10, 2997–3000 CrossRef CAS PubMed.
- M. Dietrich, G. Delaittre, J. P. Blinco, A. J. Inglis, M. Bruns and C. Barner-Kowollik, Photoclickable Surfaces for Profluorescent Covalent Polymer Coatings, Adv. Funct. Mater., 2012, 22, 304–312 CrossRef CAS.
- J. Willenbacher, K. N. R. Wuest, J. O. Mueller, M. Kaupp, H.-A. Wagenknecht and C. Barner-Kowollik, Photochemical Design of Functional Fluorescent Single-Chain Nanoparticles, ACS Macro Lett., 2014, 3, 574–579 CrossRef CAS.
- K. N. R. Wuest, H. Lu, D. S. Thomas, A. S. Goldmann, M. H. Stenzel and C. Barner-Kowollik, Fluorescent Glyco Single-Chain Nanoparticle-Decorated Nanodiamonds, ACS Macro Lett., 2017, 6, 1168–1174 CrossRef CAS.
- J. P. Blinco, K. E. Fairfull-Smith, B. J. Morrow and S. E. Bottle, Profluorescent Nitroxides as Sensitive Probes of Oxidative Change and Free Radical Reactions, Aust. J. Chem., 2011, 64, 373–389 CrossRef CAS.
- Y. Samuni and J. Gamson, Factors Influencing Nitroxide Reduction and Cytotoxicity In Vitro, Antioxid. Redox Signaling, 2004, 6, 587–595 CrossRef CAS PubMed.
- A. Rajca, Y. Wang, M. Boska, J. T. Paletta, A. Olankitwanit, M. A. Swanson, D. G. Mitchell, S. S. Eaton, G. R. Eaton and S. Rajca, Organic Radical Contrast Agents for Magnetic Resonance Imaging, J. Am. Chem. Soc., 2012, 134, 15724–15727 CrossRef CAS PubMed.
- Z. Zhelev, R. Bakalova, I. Aoki, K.-I. Matsumoto, V. Gadjeva, K. Anzai and I. Kanno, Nitroxyl Radicals for Labeling of Conventional Therapeutics and Noninvasive Magnetic Resonance Imaging of Their Permeability for Blood-Brain Barrier: Relationship between Structure, Blood Clearance, and MRI Signal Dynamic in the Brain, Mol. Pharm., 2009, 6, 504–512 CrossRef CAS PubMed.
- R. C. Brasch, D. E. Nitecki, M. Brant-Zawadzki, D. R. Enzmann, G. E. Wesbey, T. N. Tozer, L. D. Tuck, C. E. Cann, J. R. Fike and P. Sheldon,
et al., Brain Nuclear Magnetic Resonance Imaging Enhanced by a Paramagnetic Nitroxide Contrast Agent: Preliminary Report Materials and Methods, Am. J. Roentgenol., 1983, 141, 1019–1023 CrossRef CAS PubMed.
- Y. Yang, Q. Zhao, W. Feng and F. Li, Luminescent Chemodosimeters for Bioimaging, Chem. Rev., 2013, 113, 192–270 CrossRef CAS PubMed.
- H.-Y. Ahn, K. E. Fairfull-Smith, B. J. Morrow, V. Lussini, B. Kim, M. V. Bondar, S. E. Bottle and K. D. Belfield, Two-Photon Fluorescence Microscopy Imaging of Cellular Oxidative Stress Using Profluorescent Nitroxides, J. Am. Chem. Soc., 2012, 134, 4721–4730 CrossRef CAS PubMed.
- N. B. Yapici, S. Jockusch, A. Moscatelli, S. R. Mandalapu, Y. Itagaki, D. K. Bates, S. Wiseman, K. M. Gibson, N. J. Turro and L. Bi, New Rhodamine Nitroxide Based Fluorescent Probes for Intracellular Hydroxyl Radical Identification in Living Cells, Org. Lett., 2012, 14, 50–53 CrossRef CAS PubMed.
- N. Medvedeva, V. V. Martin, A. L. Weis and G. I. Likhtenshtein, Dual Fluorophore-Nitronyl Probe for Investigation of Superoxide Dynamics and Antioxidant Status of Biological Systems, J. Photochem. Photobiol., A, 2004, 163, 45–51 CrossRef CAS.
- B. J. Morrow, D. J. Keddie, N. Gueven, M. F. Lavin and S. E. Bottle, A Novel Profluorescent Nitroxide as a Sensitive Probe for the Cellular Redox Environment, Free Radicals Biol. Med., 2010, 49, 67–76 CrossRef CAS PubMed.
- C. Coenjarts, O. García, L. Llauger, J. Palfreyman, A. L. Vinette and J. C. Scaiano, Mapping Photogenerated Radicals in Thin Polymer Films: Fluorescence Imaging Using a Prefluorescent Radical Probe, J. Am. Chem. Soc., 2003, 125, 620–621 CrossRef CAS PubMed.
- A. S. Micallef, J. P. Blinco, G. George, D. A. Reid, E. Rizzardo, S. H. Thang and S. E. Bottle, The Application of a Novel Profluorescent Nitroxide to Monitor Thermo-Oxidative Degradation of Polypropylene, Polym. Degrad. Stab., 2005, 89, 427–435 CrossRef CAS.
- M.-P. Chien, M. P. Thompson, E. C. Lin and N. C. Gianneschi, Fluorogenic Enzyme-Responsive Micellar Nanoparticles, Chem. Sci., 2012, 3, 2690–2694 RSC.
- R. M. Davis, A. L. Sowers, W. Degraff, M. Bernardo, A. Thetford, M. C. Krishna and J. B. Mitchell, A Novel Nitroxide Is an Effective Brain Redox Imaging Contrast Agent and in Vivo Radioprotector, Free Radicals Biol. Med., 2011, 51, 780–790 CrossRef CAS PubMed.
- H. Utsumi, K.-I. Yamada, K. Ichikawa, K. Sakai, Y. Kinoshita, S. Matsumoto and M. Nagai, Simultaneous Molecular Imaging of Redox Reactions Monitored by Overhauser-Enhanced MRI with N-Labeled Nitroxyl Radicals, Proc. Natl. Acad. Sci. U. S. A., 2006, 103, 1463–1468 CrossRef CAS PubMed.
- K.-I. Matsumoto, F. Hyodo, A. Matsumoto, A. P. Koretsky, A. L. Sowers, J. B. Mitchell and M. C. Krishna, High-Resolution Mapping of Tumor Redox Status by Magnetic Resonance Imaging Using Nitroxides as Redox-Sensitive Contrast Agents, Clin. Cancer Res., 2006, 12, 2455–2462 CrossRef CAS PubMed.
- F. Hyodo, K.-I. Matsumoto, A. Matsumoto, J. B. Mitchell and M. C. Krishna, Probing the Intracellular Redox Status of Tumors with Magnetic
Resonance Imaging and Redox-Sensitive Contrast Agents, Cancer Res., 2006, 66, 9921–9928 CrossRef CAS PubMed.
- C. S. Winalski, S. Shortkroff, R. V. Mulkern, E. Schneider and G. M. Rosen, Magnetic Resonance Relaxivity of Dendrimer-Linked Nitroxides, Magn. Reson. Med., 2002, 48, 965–972 CrossRef CAS PubMed.
- C. S. Winalski, S. Shortkroff, E. Schneider, H. Yoshioka, R. V. Mulkern and G. M. Rosen, Targeted Dendrimer-Based Contrast Agents for Articular Cartilage Assessment by MR Imaging 1, Osteoarthrotic Cartilage, 2008, 16, 815–822 CrossRef CAS PubMed.
- G. Francese, F. A. Dunand, C. Loosli, A. E. Merbach and S. Decurtins, Functionalization of PAMAM Dendrimers with Nitronyl Nitroxide Radicals as Models for the Outer-Sphere Relaxation in Dentritic Potential MRI Contrast Agents, Magn. Reson. Chem., 2003, 41, 81–83 CrossRef CAS.
-
R. G. Hicks, H. Karoui, F. Le Moigne, O. Ouari and P. Tordo, Stable Radicals: Fundamentals and Applied Aspects of Odd-Electron Compounds, John Wiley & Sons, Ltd, Chichester, 2010 Search PubMed.
- K.-A. Hansen and J. P. Blinco, Nitroxide Radical Polymers – a Versatile Material Class for High-Tech Applications, Polym. Chem., 2018, 9, 1479–1516 RSC.
- E. M. Simpson, Z. D. Ristovski, S. E. Bottle, K. E. Fairfull-Smith and J. P. Blinco, Modular Design of Profluorescent Polymer Sensors, Polym. Chem., 2015, 6, 2962–2969 RSC.
- K. Hansen, K. E. Fairfull-smith, S. E. Bottle and J. P. Blinco, Development of a Redox-Responsive Polymeric Profl Uorescent Probe, Macromol. Chem. Phys., 2016, 217, 2330–2340 CrossRef CAS.
- K. Gallas, A.-C. Knall, S. R. Scheicher, D. E. Fast, R. Saf and C. Slugovc, A Modular Approach Towards Fluorescent pH and Ascorbic Acid Probes Based on Ring-Opening Metathesis Polymerization, Macromol. Chem. Phys., 2014, 215, 76–81 CrossRef CAS.
- J. Nicolas, Y. Guillaneuf, D. Bertin, D. Gigmes and B. Charleux, Nitroxide-Mediated Polymerization, Prog. Polym. Sci., 2013, 38, 63–235 CrossRef CAS.
- M. A. Sowers, J. R. McCombs, Y. Wang, J. T. Paletta, S. W. Morton, E. C. Dreaden, M. D. Boska, M. F. Ottaviani, P. T. Hammond and A. Rajca,
et al., Redox-Responsive Branched-Bottlebrush Polymers for in Vivo MRI and Fluorescence Imaging, Nat. Commun., 2014, 5, 5460–5469 CrossRef PubMed.
- J. Matko, N. Ohki and M. Edidin, Luminescence Quenching by Nitroxide Spin Labels in Aqueous Solution: Studies on the Mechanism of Quenching, Biochemistry, 1992, 31, 703–711 CrossRef CAS PubMed.
- X. Zhou and L. Ren, Building a Better Magnetic Resonance Imaging Contrast Agent Using Macromolecular Architecture, ACS Cent. Sci., 2017, 3, 820–822 CrossRef CAS PubMed.
- H. V. T. Nguyen, Q. Chen, J. T. Paletta, P. Harvey, Y. Jiang, H. Zhang, M. D. Boska, M. F. Ottaviani, A. Jasanoff and A. Rajca,
et al., Nitroxide-Based Macromolecular Contrast Agents with Unprecedented Transverse Relaxivity and Stability for Magnetic Resonance Imaging of Tumors, ACS Cent. Sci., 2017, 3, 800–811 CrossRef CAS PubMed.
- S. Garmendia, D. Mantione, S. Alonso-de Castro, C. Jehanno, L. Lezama, J. L. Hedrick, D. Mecerreyes, L. Salassa and H. Sardon, Polyurethane Based Organic Macromolecular Contrast Agents (PU-ORCAs) for Magnetic Resonance Imaging, Polym. Chem., 2017, 8, 2693–2701 RSC.
- R. B. Lauffer, Paramagnetic Metal-Complexes as Water Proton Relaxation Agents for NMR Imaging: Theory and Design, Chem. Rev., 1987, 87, 901–927 CrossRef CAS.
- S. Swaminathan, Nephrogenic Systemic Fibrosis, Gadolinium, and Iron Mobilization, N. Engl. J. Med., 2007, 357, 720–722 CrossRef CAS PubMed.
- S. H. Mutlu, C. W. Schmitt, N. Wedler-Jasinski, H. Woehlk, K. E. Fairfull-Smith, J. P. Blinco and C. Barner-Kowollik, Spin Fluorescence Silencing Enables an Efficient Thermally Driven Self-Reporting Polymer Release System, Polym. Chem., 2017, 8, 6199–6203 RSC.
- G. A. Husseini, S. Kanan and M. Al-Sayah, Investigating the Fluorescence Quenching of Doxorubicin in Folic Acid Solutions and Its Relation to Ligand-Targeted Nanocarriers, J. Nanosci. Nanotechnol., 2016, 16, 1410–1414 CrossRef CAS PubMed.
- F. M. Winnik’, M. F. Ottaviani, S. H. Bossman, W. Pan, M. Garcia-Canbay and N. J. Turro, Phase Separation of Poly (N-Isopropylacrylamide) in Water: A Spectroscopic Study of a Polymer Tagged with a Fluorescent Dye and a Spin Label, J. Phys. Chem., 1993, 97, 12998–13005 CrossRef.
- M. Eing, B. Olshausen, K. E. Fairfull-Smith, U. Schepers, C. Barner-Kowollik and J. P. Blinco, Reporting pH-Sensitive Drug Release via Unpaired Spin Fluorescence Silencing, Polym. Chem., 2018, 9, 499–505 RSC.
- M. Eing, B. T. Tuten, J. P. Blinco and C. Barner-Kowollik, Visible light activation of spin-silenced fluorescence, Chem. – Eur. J., 2018, 24, 12246–12249 CrossRef CAS PubMed.
- Y. Tang, F. He, M. Yu, S. Wang, Y. Li and D. Zhu, Radical Scavenging Mediating Reversible Fluorescence Quenching of an Anionic Conjugated Polymer: Highly Sensitive Probe for Antioxidants, Chem. Mater., 2006, 18, 3605–3610 CrossRef CAS.
- T. S. Fischer, S. Spann, Q. An, B. Luy, M. Tsotsalas, J. P. Blinco, H. Mutlu and C. Barner-Kowollik, Self-reporting and refoldable profluorescent single-chain nanoparticles, Chem. Sci., 2018, 9, 4696–4702 RSC.
|
This journal is © The Royal Society of Chemistry 2022 |
Click here to see how this site uses Cookies. View our privacy policy here.