Persistent, mobile, and toxic plastic additives in Canada: properties and prioritization†
Received
4th March 2022
, Accepted 25th September 2022
First published on 26th September 2022
Abstract
The hazards of many plastic additives on human and environmental health are well documented. However, little emphasis has been put on plastic additives that are persistent, mobile, and toxic (PMT) rather than persistent, bioaccumulative, and toxic. Due to their high mobility and stability, it is unlikely that wastewater treatment plants will effectively remove PMT plastic additives. Herein, an in silico analysis was performed to (1) assess the retention of PMT plastic additives registered for use in Canada in wastewater treatment plants; and (2) determine whether their physical–chemical properties and structural features can be used as identifiers for PMT plastic additives with particularly low retention. We identified 124 PMT plastic additives of which 52% had less than 20% removal from wastewater treatment based on predictions using the model SimpleTreat. Log
Kaw, log
Kow/Dow, and log
Koc/Doc ranges were defined that are indicative of low retention PMT plastic additives. Furthermore, it was found that non-halogenated PMT plastic additives that contain nitrogen are most likely to be poorly retained in wastewater treatment plants. The results of this study provide screening and prioritization criteria, as well as a suspect list for PMT plastic additives.
Environmental significance
Persistent, Mobile and Toxic (PMT) substances have been recognised as having the potential to impact environmental and human health. However, there are significant challenges associated with their identification, quantification, and assessment in the environment given the amount of potential PMT substances and the diversity of chemistries and properties they encompass. Here we present a method to identify and prioritize PMT substances based on their physical–chemical property space, structural identifiers, use category, and in silico retention in wastewater treatment plants – with a focus on PMT plastic additives registered for use in Canada. The results can be used by the scientific community, regulators, and industry alike to screen chemicals for potential PMT characteristics.
|
1. Introduction
With the rise in global plastic pollution, plastic-associated contaminants have become an increasing threat to the environment and human health.1–7 Plastics consist of two main components: an organic polymer matrix serving as the main plastic structure, and additives used to impart specific physical–chemical properties.8 The most common types of these additives are functional additives (e.g., antioxidants, flame retardants, plasticizers), colorants, fillers, and reinforcement agents.9,10 Their contribution to the plastic (per mass) can range from less than one percent to fifty percent or more of the total plastic composition.10,11
Many functional plastic additives are not covalently bound to the polymer they are added to.8 This means that these compounds can be released from the plastic polymer over time and enter the surrounding environment.8,10 This leaching into the environment is concerning as many functional organic plastic additives have been associated with health effects such as different cancers, endocrine disruption, adverse effects to reproduction and development, as well as general toxicity in a variety of organisms.1,2,12,13
Given their potential toxicity and detection in the environment, some plastic additives have been identified as potential contaminants of concern or even as persistent organic pollutants (POPs).13 Nevertheless, many hazardous plastic additives are not restricted.4,13 The current regulations in Canada under the Canadian Environmental Protection Act (CEPA) control the emissions of many hydrophobic plastic additives, if they meet the regulatory criteria for persistence, bioaccumulation potential, and toxicity (PBT).14 Moreover, plastic pollution has become a regulatory priority for Canada leading to the creation of a comprehensive “Plastic Science Agenda”15 as well as the “Zero Plastic Waste Initiative”.16 There is a regulatory safeguard in Canada against the environmental risk of many plastic additives that are bioaccumulative but not for plastic additives that are persistent, mobile in water, and toxic (PMT).17,18
PMT substances are toxic polar organic compounds that are persistent in water and can therefore pose a long-term risk to the aquatic environment.17 Due to their polarity, generally low volatility, and low potential to sorb to organic matter or particles, emitted PMT substances remain in water or can penetrate groundwater aquifers and threaten the quality of drinking water sources.17,19,20 Moreover, the high environmental stability of PMT substances can enable them to undergo long-range water-based transport into remote environments such as the Arctic Ocean.18 The German Environmental Protection Agency (UBA) has released the following criteria for the identification of PMT substances:19
A substance is considered persistent (P) if (a) the degradation half-life in marine water at 9 °C is higher than 60 days, (b) the degradation half-life in fresh or estuarine water at 12 °C is higher than 40 days, (c) the degradation half-life in marine sediment at 9 °C is higher than 180 days, (d) the degradation half-life in fresh or estuarine water sediment at 12 °C is higher than 120 days, or (e) the degradation half-life in soil at 12 °C is higher than 120 days.19
A substance is considered mobile (M) if the lowest experimental organic carbon–water coefficient log
Koc over the pH range of 4–9 is less than 4.0.19
A substance is considered toxic (T) if it meets any of the criteria for toxicity set out in Annex XIII, 1.1.3 of the European Registration, Evaluation, Authorisation and Restriction of Chemicals (REACH) regulatory framework. These criteria are (a) the long-term no-observed effect concentration (NOEC) or EC10 for marine or freshwater organisms is less than 0.01 mg L−1, (b) the substance meets the criteria for classification as carcinogenic (category 1A or 1B), germ cell mutagenic (category 1A or 1B), or toxic for reproduction (category 1A, 1B, or 2), or (c) there is other evidence of chronic toxicity.19
Because PMT substances are highly mobile in water, wastewater treatment plants (WWTPs) are not expected to retain PMT substances and thus release them into the aquatic environment.20 PMT substances entering closed and semi-closed water cycles may even concentrate over time, with dilution being the only process able to reduce concentrations.17 However, the identities and potential retention of different PMT substances in WWTPs are currently unknown. In this research, we aimed to (A) identify PMT substances used in Canada, (B) identify which of those were plastic additives, (C) model their retention in WWTPs, and (D) outline physical–chemical properties that can be used as screening criteria to identify them.
2. Methods
2.1 Developing a list of PMT plastic additives registered for use in Canada
A comprehensive list of PMT plastic additives registered for use in Canada was developed based on the PMT criteria and PMT suspect list published by UBA.19 This “UBA PMT list” (available on the Norman Network, https://www.norman-network.net) was combined and compared to the Canadian Domestic Substances List (DSL) using CAS numbers to create a list of PMT substances registered for use in Canada (Table S1†). This list was then compared to the PlasticMAP database published by Wiesinger et al.8 that contains over 10
000 plastic monomers, additives, and processing aids to create a list of PMT plastic additives registered for use in Canada (Table S2†). Physical–chemical property data for PMT substances was collected from Arp et al.21 as well as the CompTox Chemistry Dashboard by the US Environmental Protection Agency22 for property data not available in the Arp et al. publication.
2.2 Prioritizing PMT plastic additives based on their predicted removal efficiencies from WWTPs
The open access wastewater treatment model SimpleTreat 4.0 (ref. 23) was used to model the removal efficiencies of the PMT plastic additives from wastewater effluent to assess their potential for human and environmental exposure. SimpleTreat is a fugacity mass-balance model that predicts the removal of a chemical in a WWTP with conventional primary and secondary treatment as well as its partitioning into different media (air, sludge, effluent) during the treatment process using physical–chemical property data and information on biodegradation.23 For ionizable substances, SimpleTreat determines the fraction of the substance that is in acid/neutral/base form using the pKa of the substance and the pH that are input.23 The model only considers neutral molecules for partitioning through the air–water boundary, and as such, the ionic fraction of a substance is not considered for any type of volatilization. Consequently, the ionic fraction is only modeled based on the partitioning between the aqueous and sludge phases.23 For each substance, the following physical–chemical properties were retrieved from Arp et al.,21 CompTox Chemicals Dashboard, or the European Chemical Agency (ECHA) database (https://comptox.epa.gov/dashboard/dsstoxdb/batch_search, https://echa.europa.eu) and were inputted into SimpleTreat 4.0: molecular weight (g mol−1), octanol–water partition coefficient (Kow/Dow), organic carbon–water partition coefficient (Koc/Doc), water solubility (mg L−1), vapor pressure (Pa), Henry's law constant (Pa m3 mol−1), biodegradation half-life (days), and pKa for ionizable compounds. Dow, Doc, and water solubility were the minimum measured or predicted values at a pH between 4 and 10, while vapor pressure and Kaw values were measured or predicted at 298 K.28Kow/Dow values were only used whenever Koc/Doc values were not available. This was done in accordance with Arp et al.,21 who estimated log
Koc from log
Kow using the linear relationship between the two when an experimental log
Koc was unavailable. The default settings in SimpleTreat 4.0 were used for the ‘Mode of operation’ and ‘Emission scenario’.
The removal of PMT substances in wastewater treatment plants was modelled using two scenarios. For the first scenario, all PMT substances were classed as non-biodegradable based on their regulatory classification as “persistent”. This constitutes a ‘worst case scenario’ as a number of PMT substances on the UBA list are expected to be at least moderately degradable in wastewater treatment plants19 (Table S2†). For the second scenario, predicted or, where available, measured biodegradation half-lives (retrieved from CompTox) were used to model the removal efficiency of the PMT compounds (Table S3†).
Once the removal efficiencies were acquired (Table S3†) the PMT substances were ranked based on their estimated emissions via effluent (Table S4†). The ‘emission via effluent’ was used to group the PMT substances into four emission groups: <10%, 10–50%, 50–80%, and 80–100% emission. The 95% percentile ranges were calculated for each group to identify any overlapping groups.
Additionally, the partitioning of PMT plastic additives into sludge and air was investigated. The amount of PMT substances that partitioned into sludge was determined by taking the sum of the SimpleTreat output for percent elimination of the substance via primary and surplus sludge. The amount of PMT substances that partitioned into air was determined by taking the sum of the SimpleTreat output for percent elimination by volatilization and stripping (Table S3†).
2.3 Identifying the physical–chemical property space and use of PMT plastic additives
The physical–chemical property space of PMT plastic additives with high emission via effluent (80–100% emission) was investigated to identify the combination of log
Koc/Doc/Kow/Dow, log
Kaw, and vapor pressure that is indicative of PMT substances with low retention in WWTPs. Wherever available log
Koc/Doc were used. If multiple values were available for log
Koc/Doc/Kow/Dow (at pH 4 to 10), minimum values were used as a “worst-case scenario” with regards to potential mobility.21 Since log
Koc/Doc/Kow/Dow and water solubility are negatively correlated, only log
Koc/Doc/Kow/Dow was used in the analysis as there was more experimental data for it compared to water solubility. The resulting physical–chemical property space for the partitioning into air, sludge and effluent were combined to determine the dominant fate of the individual PMT plastic additives in WWTPs.
Additionally, the individual use functions of the identified PMT plastic additives were collected from the PlasticMAP published by Wiesinger et al.8 (Table S5†).
2.4 Statistical analysis and sensitivity analysis
Comparison and filtering of all data as well as the calculation of statistics and chemical space plots were conducted using Microsoft 356 excel for enterprise. Further data analysis and graphics were created using R Studio version 1.3.1093.
A sensitivity analysis based on the method by Morgan and Henrion24 was performed to assess the sensitivity of the total modelled emissions through effluent to the variability in input data. The sensitivity to a specific input parameter was tested by individually changing the median of the respective input parameter one at a time by 10% and calculating the impact on the total emission via the effluent. The sensitivity (S) of the emissions via effluent to an individual parameter was quantified using:
|  | (1) |
with
SEE defined as sensitivity of the emission
via the effluent; ΔEE defined as changes in the emission
via the effluent,
I as the initial input parameter value and Δ
I as the change in input parameter value.
2.5 Quality assurance
Given the high variability of physical–chemical property data amongst different sources and databases, collecting data from a single source was important to ensure consistency of the data. For this reason, the database ranking REACH-registered substances by their known or suspected persistency and mobility, published by Arp et al.,21 was used to collect nearly all the physical–chemical property data for this study (Table S2†). While this database does contain both experimental and modelled data, the aim was to transfer the consistency by which those data were collected to this study. Only two of the identified PMT plastic additives (CAS 156-60-0 and CAS 57-41-0) were not present in the Arp et al.21 database. For these substances, physical–chemical property data was collected from the CompTox Chemistry Dashboard22 or ECHA (https://echa.europa.eu). Biodegradation half-lives were collected from the CompTox Chemistry Dashboard22 for all substances included in this study.
3. Results
3.1 Estimated removal of PMT plastic additives from wastewater in relation to their properties
In total 208 of the 260 PMT substances identified by UBA are currently registered for use in Canada (Table S1†). One hundred and twenty-four (124) of these compounds (60%) were classified as plastic additives (Tables 1 and S2†). Among these, 50% contained nitrogen, 33% were halogenated, 13% contained sulphur, 4% contained phosphorus, and 3% contained silicon (Table S3†). The physical–chemical property ranges of the identified PMT plastic additives are presented in Table 1.
Table 1 Physical–chemical property range of PMT plastic additives registered for use in Canada, and 5th to 95th percentile property space for with PMT plastic additives ≥80% effluent emission, 50–80% effluent emission, 10–50% effluent emission, or ≤10% effluent emission from a modeled WWTP
Property range |
Total PMT plastic additives |
≥80% effluent emission |
50–80% effluent emission |
10–50% effluent emission |
≤10% effluent emission |
Log Kow/Dow (median) |
−11 to 9.9 (1.9) |
−3.9 to 4.1 (0.87) |
−7.1 to 4.4 (1.1) |
0.33 to 5.7 (2.8) |
1.4 to 4.7 (2.5) |
Log Koc/Doc (median) |
−8.3 to 4.0 (1.7) |
−3.7 to 3.1 (1.3) |
−0.32 to 3.7 (2.2) |
0.42 to 3.6 (2.4) |
0.60 to 3.2 (1.8) |
Log Kaw (median) |
−32 to 2.4 (−4.3) |
−16 to −2.3 (−6.3) |
−13 to −2.0 (−5.2) |
−6.2 to −0.54 (−1.3) |
−2.4 to 1.3 (−0.27) |
Water solubility (median) [mg L−1] |
4.2 × 10−5 to 3.3 × 1012 (3.3 × 103) |
0.043 to 1.9 × 1010 (5.8 × 103) |
3.7 to 6.7 × 108 (4.1 × 103) |
15 to 1.1 × 1010 (1.1 × 103) |
41 to 3.5 × 105 (1.3 × 103) |
Vapor pressure (median) [Pa] |
1.2 × 10−23 to 6.5 × 105 (3.0) |
8.1 × 10−20 to 2.2 × 103 (6.0 × 10−2) |
1.7 × 10−8 to 1.9 × 103 (0.20) |
1.4 × 10−3 to 2.2 × 104 (2.3 × 102) |
2.0 × 102 to 5.9 × 105 (1.3 × 104) |
The modelled emission via effluent for the 124 PMT plastic additives ranged from 0% to 100% with the majority of PMT plastic additives having median emissions via the effluent of over 75% (Fig. 1).
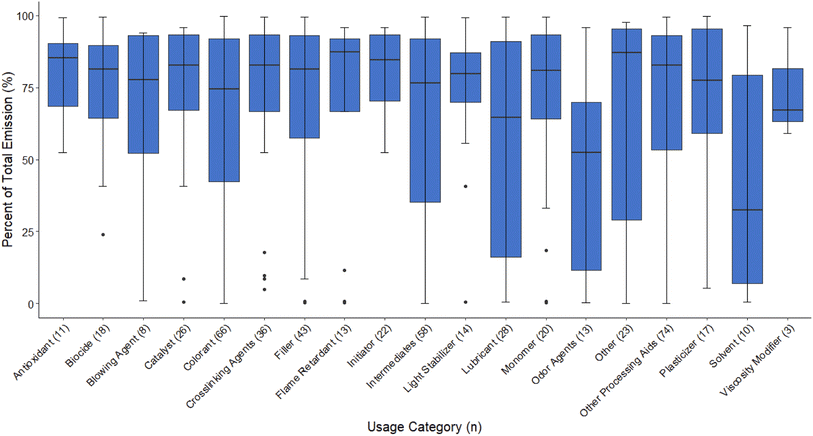 |
| Fig. 1 The distribution of effluent emission (as a percentage of total emission) of 124 PMT plastic additives, based on their use category. | |
There were 64 compounds (52% of the PMT plastic additives) with an 80–100% emission via effluent (Table 1). Of these, 26 (42%) were ionizable, 24 (38%) were neutral, and 14 were amphiprotic/polyprotic (Table S2†); 69% contained nitrogen, 19% contained sulphur, 23% were halogenated, and 3% contained phosphorus, and none contained silicon (Table S3†). There were 24 compounds with a 50–80% emission via effluent, 18 compounds with emissions via effluent between 10–50%, and 18 compounds with emissions via effluent of <10% (Table 1 and Fig. 2). Similar ranges for log
Koc/Doc/Kow/Dow and log
Kaw were observed for both experimental and predicted values (Fig. S2†).
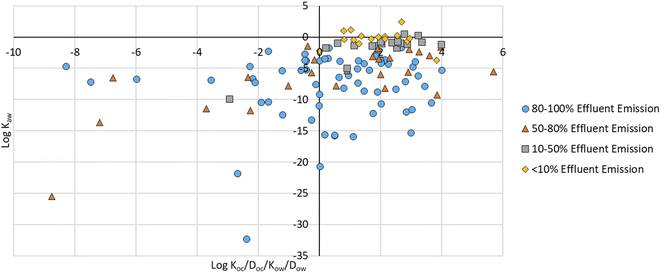 |
| Fig. 2 Log Koc/Doc/Kow/Dowversus log Kaw graph of 124 PMT plastic additives plotted according to their predicted % emission via effluent in a WWTP simulation. | |
In addition to the 64 compounds with ≥80% emission in the effluent, there were 21 compounds with ≥80% emission in the air, and three compounds with ≥40% partitioning into the sludge (Table 2).
Table 2 Physical–chemical property space (5th to 95th percentile) of PMT plastic additives registered for use in Canada with ≥80% emission through effluent or air from WWTP, or ≥40% partitioning into sludge
Property range |
≥80% emission via effluent |
≥80% emission via air |
≥40% partitioning into sludge |
Log Kow/Dow |
−3.9 to 4.1 |
1.4 to 4.4 |
5.7 to 6.0 |
Log Koc/Doc |
−3.7 to 3.1 |
0.51 to 3.1 |
3.9 to 4.0 |
Log Kaw |
−16 to −2.4 |
−2.2 to 1.1 |
−8.9 to −2.0 |
Water solubility [mg L−1] |
0.043 to 1.9 × 1010 |
47 to 6.7 × 104 |
0.65 to 1.8 × 104 |
Vapor pressure [Pa] |
8.1 × 10−20 to 2.2 × 103 |
56 to 5.8 × 105 |
1.7 × 10−4 to 3.7 × 10−3 |
3.2 Estimated removal of PMT plastic additives based on their use category
Use functions for the PMT plastic additives were taken from the PlasticMAP database published by Wiesinger et al.8 This database is composed of over 10
000 plastic monomers, additives, and processing aids, and was created by compiling and filtering information from 63 data sources. Wiesinger et al. assessed the quality of the information in this database based their origin, and entries were assigned an appropriate confidence level (low, medium, high). For the use function information gathered from the PlasticMAP database for the 124 PMT plastic additives in this study, 113 compounds (91%) had a high confidence score, 10 compounds had a medium confidence score, and one compound (N-methyl-benzenamine) had a low confidence score. Additionally, the 10 compounds with a medium confidence score had no defined use function, and as such, fell under the “other” category.
These use categories covered 101 of the 124 PMT plastic additives, with the remaining 23 compounds falling under the “other” category. Of these 101 PMT plastic additives with a known use, 89 fell into more than one category, with a total of 504 different entries when separated by use category (Table S5†). Most of the 101 PMT plastic additives fell into the ‘other processing aids’ (60%), ‘colorants’ (53%), and ‘intermediates’ (47%) use categories (Fig. S3†). This was true for PMT plastic additives in general as well as for PMT plastic additives with a high predicted emission through wastewater effluent (Fig. S3†). The use categories with the fewest PMT substances were ‘heat stabilizers’ and ‘antistatic agents’ with one substance each (Table S5†).
A majority of the use categories showed the greatest amount of PMT emissions to the effluent, followed by air, and lastly, partitioning into the sludge (Fig. 3). Odor agents and solvents had notably lower effluent emissions compared to the other categories (Fig. 3). While effluent emission was lower for odor agents compared to most other use categories, the median (53%) emission into effluent was still greater than that of air (32%) and sludge (0.14%), meaning effluent was the primary emission pathway. Solvents were the only use category in which air, rather than effluent, was the primary emission pathway (median 59%) (Fig. 3).
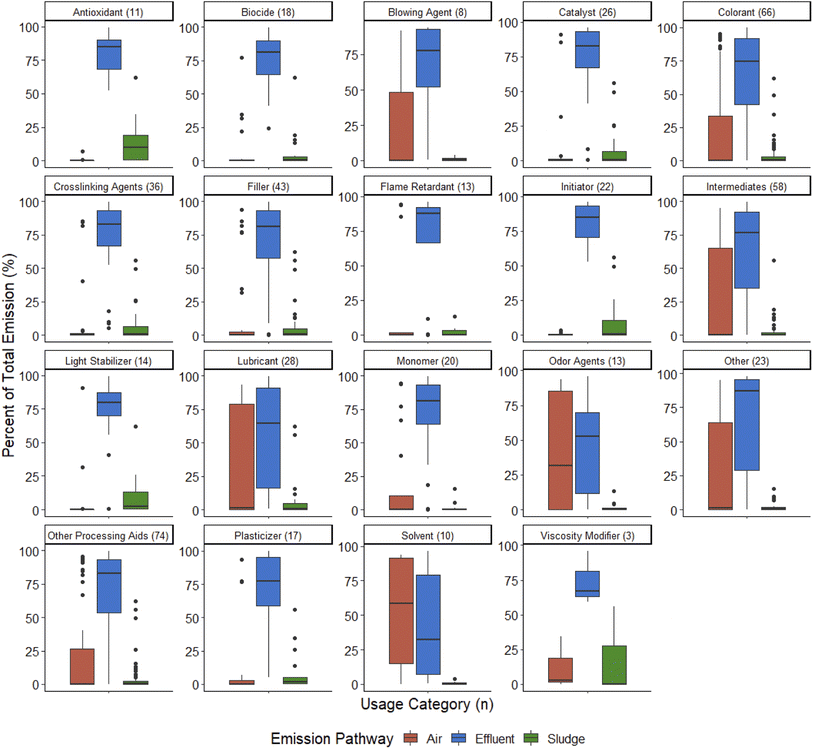 |
| Fig. 3 The distribution of emission data for the 124 PMT substances, based on the three emission pathways (air, effluent, sludge) from a theoretical WWTP and the known use categories of each substance. | |
For all other use categories, air was the second-most prevalent emission pathway, with emissions between 0 and 95% for 9 of the 19 use categories (Fig. S4†). These categories were blowing agents, colorants, intermediates, lubricants, monomers, odor agents, other processing aids, viscosity modifiers, and other. PMT plastic additive partitioning into sludge was low for PMT plastic additives from all use categories (Fig. S4†). Antioxidants had the highest median partitioning into sludge with 10% (Fig. 3).
Of the 124 PMT plastic additives, 23 fell under the “other” use category, as a usage function could not be identified for these substances. Despite this, they followed the same general pattern observed in the other use categories (effluent emission > air emission > sludge partitioning).
4. Discussion
In this study, we identified 124 PMT plastic additives registered for use in Canada with 64 substances with less than 20% retention in WWTP. Some of these low retention PMT plastic additives were known organic water contaminants, such as sulphanilic acid and dapsone,25,26 while others are new and emerging contaminants that have been scarcely studied, such as 1,3-di-o-tolylguanidine and trifluoromethanesulfonic acid (Table S2†). In previous research, Schulze et al.25 used an environmental emission score (E-score) calculation based on the annual tonnage and use characteristics of the substances to rank the likelihood of a persistent, mobile organic contaminant (PMOC) to be emitted into the environment. 61 of the 124 PMT plastic additives in this study were also ranked previously by Schulze et al.25 There were similarities between the relative ranks of the common substances in the two lists, such as sulphanilic acid, 1,4-diazabicyclo[2.2.2]octane and pigment yellow 83 ranking within the top 20% of both lists. With that being said, the relative ranks of many of the other substances did not align. This is likely due to the disparity in the methods by which the rankings of the two lists were performed. Since E-score focusses on annual tonnage, use characteristics, and the physical–chemical properties of a substance, the rankings done by Schulze et al. provide a more thorough assessment of emission potential. In contrast, the analysis performed in this study focusses on the partitioning and removal from a typical WWTP. As such, these rankings are complementary to one another and combining such ranking or scoring systems in the future may provide a more comprehensive list of substances for environmental screening and regulation.
Most of the top 20 ranked PMT plastic additives in this study had high PMOC and mobility scores (>3) based on the classification proposed by Arp et al.21 (Table S2†). However, there were some notable discrepancies, such as for organophosphate esters that were ranked as “unstable mobile organic compounds” in Arp et al.21 whereas many of them were ranked highly among the PMT substances in this analysis. The degradability of organophosphate esters has recently been questioned due to their prevalence in Arctic environments.18,27 Based on these findings, it has been suggested that organophosphate esters are PMOC substances rather than unstable mobile compounds.28 The differences in the respective rankings and the omission of many of the top 20 ranked PMT plastic additives in the environmental emission score calculation by Schulze et al.25 highlights the importance for combinations of prioritization mechanisms as well as specific local foci to understand and manage potential risks of emerging contaminants such as PMT plastic additives.
4.1 Prioritization of PMT plastic additives
The results of our research showed that PMT substances are used in Canada and 60% of them can be used as plastic additives. In an assessment of over 10
000 plastic additives, monomers, and processing aids used on the global market, Wiesinger et al.8 showed that only 57 were considered either PBT or very persistent and very bioaccumulative (vPvB). Conversely, we identified 124 plastic additives registered for use in Canada that meet or potentially meet the criteria for PMT. Considering that PMT substances can pose a risk to drinking water security17,29 and that PMT substances can be considered an equivalent level of concern to substances of very high concern (SVHCs) under REACH20 the use of PMT substances in Canada clearly merits further investigation. This is particularly important for the 64 PMT plastic additives with predicted emissions through wastewater effluent of >80%. These poorly retained that are persistent and toxic could pose a poorly reversible risk to drinking water security and the quality of receiving aquatic environments.
Considering the number of identified PMT plastic additives and range of uses, prioritization is key to address potential risks for human health and the environment from compounds that are most susceptible to pass through WWTPs and into surface and drinking waters. In Section 73 (1)30 under ‘Priority Substances and Other Substances’, the Canadian Environmental Protection Act (CEPA) states that substances should be categorized that “(a) may present, to individuals in Canada, the greatest potential for exposure; or (b) are persistent or bioaccumulative in accordance with the regulations, and inherently toxic to human beings or to non-human organisms, as determined by laboratory or other studies”. While mobility is not currently a parameter for prioritization, many PMT substances meet one or both criteria to some extent. Classifications like this in the CEPA, along with prioritization mechanisms based on the expected retention in WWTPs enable prioritization of PMTs for water quality monitoring and alterations to their use in industry.
4.1.1 Using the physical–chemical space to identify and prioritize future PMT plastic additives.
PMT plastic additives share common physicochemical properties (log
Kow/Dow/Koc/Doc, log
Kaw, and vapor pressure) that can be used to identify them. As expected, substances that were polar and non-volatile (log
Kow/Dow/Koc/Doc between −3.7 and 4.1, and log
Kaw between −16 and −2.3) were primarily emitted in the effluent, while those that were polar and volatile (log
Kow/Dow/Koc/Doc between 0.51 and 4.4, and log
Kaw between −2.2 and 1.1) were primarily emitted in the air, and those that were hydrophobic (log
Kow/Dow/Koc/Doc between 3.9 and 6.0) primarily partitioned into the sludge (Table S3†). Interestingly, substances primarily emitted in the effluent not only covered the low log
Kow/Dow/Koc/Doc values, but also contained several substances with higher log
Kow/Dow values up to a maximum of 4.4. This was likely due to the lack of log
Koc/Doc values for a 48 of the 124 PMT plastic additives, where log
Kow/Dow values had to be used, extending the property range above what would be expected (<4.0) (Fig. 4).
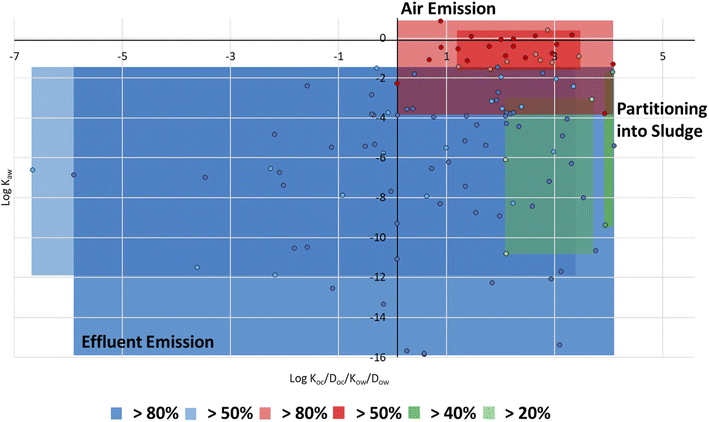 |
| Fig. 4 The partitioning space plot showing the dominant emission pathways (effluent, air, sludge) of 124 PMT plastic additives at a 90th percentile. | |
The observed property ranges can be used in the future for the initial screening of PMT substances entering WWTPs. The property ranges (Fig. 4) indicated that as a compound's percent emission via effluent increased, its water solubility increased (lower log
Koc/Doc) and volatility decreased (lower log
Kaw). Specifically, PMTs with a log
Koc/Doc between −3.7 and 3.1, and a log
Kaw between −16 and −2.4 at a 90th percentile were highly emitted in the effluent (80–100%). However, it is important to note that several PMT plastic additives were also modelled to be emitted to a considerable extent via air (Fig. 4).
While emission via air was expected for PMT plastic additives in use categories that have typically volatile compounds, such as solvents or odor agents, emission into air also occurred for PMT plastic additives in the lubricants, colorants, and intermediates use categories (Fig. S4†). Overall, 69% of the PMT plastic additives had ≥80% emissions from WWTP through either effluent or air (Table S3†) further highlighting the low retention of these compounds. The physical chemical property ranges that were identified as indicative for high emission PMT plastic additives through air, effluent, or 40% partitioning into sludge are presented in Table 2.
4.1.2 Prioritization based on structural identifiers and use categories.
In total, 62 of the PMT plastic additives contained nitrogen, 40 were halogenated, 16 contained sulphur, five contained phosphorus, and four contained silicon (Table S3†). There was a clear difference between common structural components in high emission PMT plastic additives compared to the overall PMT plastic additives. 69% of the high emission PMT plastic additives (80–100% emission) contained nitrogen, indicating that PMT plastic additives that contain nitrogen have a high potential to be released in the effluent from WWTPs (Table S3†). Halogenated compounds, on the other hand, only made up 23% of the high emission PMT substances, while they constituted 42% of the PMT plastic additives with <80% emissions through the effluent (Table S3†). The proportion of sulphur and phosphorus containing PMT plastic additives was similar between the high emission PMT plastic additives and the overall PMT plastic additives with <80% emissions via the effluent (Table S3†), indicating that the presence of these atoms does not have a strong impact on the ability of a PMT plastic additive to move through wastewater treatment plants. Silicon was only present in PMT plastic additives with <10% emission via the effluent. However, all of the silicon containing compounds had >80% predicted emissions via air, highlighting the importance of this emission pathway for certain chemicals (Table S3†).
The majority (88%) of PMT plastic additives fell under more than one use category (Table S5†). This is due to the diverse physical–chemical properties that PMT substances possess and speaks to the versatility and ubiquitous use of PMT plastic additives. All use categories apart from odor agents and viscosity modifiers had median modelled emission through effluent of >60% showing the widespread use of potential high-emission PMT plastic additives (Fig. 1). However, while some categories showed a wide spread of modelled emission, others contained substances where the modelled emissions via effluent exceeded 50% for all compounds apart from a few individual outliers (Fig. 1). These product categories with exclusively high-emission PMT plastic additives are antioxidants, crosslinking agents, flame retardants, initiators, light stabilizers, and viscosity modifiers (Fig. 1). Consistent with the overall PMT plastic additive results, the use categories with high-emission PMT plastic additives were dominated by nitrogen-containing compounds (Table S5†). Flame retardants and crosslinking agents were the only categories where halogenated compounds exceeded the amount nitrogen-containing compounds (Table S5†). The use categories provide a sense of what applications and industries the substances originate from, and are therefore an important measure for potential emission reduction efforts. Moreover, the combination of information on physical–chemical properties and use category can provide a valuable indication on whether a PMT plastic additive can be expected to consistently fall into the high-emission PMT plastic additive category.
4.2 Comparison with observational data on PMT plastic additives in WWTP
To assess the accuracy of the SimpleTreat model in predicting the removal of PMT plastic additives from a WWTP, the removal efficiencies of substances from this study were compared to removal efficiencies reported in the literature (Table S7†). The removal efficiencies for 18 substances in this study that were also reported in the literature were recorded and compared. Removal efficiencies reported in the literature were generally higher than the model results from the SimpleTreat analysis, but also highly variable with standard deviations ranging from 0.7 to 23% (Table S7†). There were six substances that had removal efficiencies within 25% of observational data reported in the literature (1H-benzotriazole, TCEP, TDCP, atrazine, diuron, and naphthalene), six within 50% the data reported in the literature (anthracene, TCPP, triclosan, TMDD, EDTA, and melamine), and six with a difference greater than 50% from the literature values (TBEP, benzothiazole, benzophenone-3, paracetamol, saccharin, and acenaphthene). The substances in each of these groups had no distinct differences in their physical chemical properties with similar median log
Kow/Dow (∼3), log
Koc/Doc (∼2), log
Kaw (∼−6), log vapor pressure (∼−3.5), and no common structural features.
While these results indicate that the SimpleTreat model might underestimate the removal of PMT substances in WWTPs, it is important to note that the literature values originate from a variety of studies, and thus, a variety of different WWTPs and regions. The removal mechanisms of many of these WWTPs may have been more advanced (e.g. including tertiary treatment) than the ‘typical’ primary and secondary WWTP modeled by SimpleTreat, which would explain some of the disparity between the literature values and those found in this study. Additionally, the WWTP influent and effluent collection methods varied greatly amongst the different literature studies, with some taking 24 hour composite samples over a single day or several days, and some taking grab samples (ref. S4–S5, S9, S14, S16 and S25†). Regardless, many of these studies still offer a valuable reference for the removal efficiencies of PMT plastic additives from WWTPs, especially in those studies that surveyed more than one WWTP or region (ref. S4, S5 and S9†). While the comparability of the SimpleTreat results was quite variable for the 18 substances, this is only a small number of the total PMT plastic additives investigated here, and as such, does not reflect the overall accuracy of the study. Further research should consider analyzing PMT substances under different WWTP scenarios/technologies in the model to determine whether the removal efficiencies are more reflective of their literature counterparts.
4.3 Uncertainty of the modelled removal and limitations
4.3.1 Uncertainty.
A major source of uncertainty in this study was the use of predicted physical–chemical property data where experimental data was not available. Given that many of the PMT plastic additives investigated here remain largely unreported or under-studied, experimental data for several of the physical–chemical properties was unavailable, and predicted values had to be used instead. This led to uncertainty in the modelled removal – particularly with regards to the potential removal through biodegradation. The sensitivity analysis showed that altering Koc/Doc/Kow/Dow, Kaw, vapor pressure, or water solubility by 10% led to less than 10% change in the overall modeled emissions. Conversely, a 10% change in biodegradation half-live values led to changes in the modeled emissions of over 10% (Table S8†).
To evaluate the uncertainty based on biodegradation, two scenarios were investigated: one where no biodegradation was input for each substance, representing a ‘worst case scenario’ where no biodegradation occurs at all, and a second scenario where the experimental or predicted biodegradation half-lives for each substance were used. The differences in modeled effluent emission between these two scenarios ranged between 0.1 and 57% (median 4.9%), with 19 of the 124 PMTs having a difference greater than 20% between the two scenarios (Table S4†). While a median 4.9% difference does not represent a large difference between the two scenarios, it does lead to uncertainty about the magnitude of the physical–chemical parameters, including biodegradative half-lives, and how a large difference between the experimental and predicted values may have influenced the results. The sensitivity of the model's results to the highly variable and uncertain environmental half-lives explains some of the discrepancy between observed and modelled emissions for the analysed PMT substances and highlights the need for more experimental biodegradation data in different environments and for different wastewater treatment technologies.21,31
Despite this, the two scenarios and comparison with the literature showed a general agreement for the relative ranking of PMT substances with regards to their emissions. This shows that the relevant physical–chemical property ranges offer a valuable tool for PMT plastic additive screening and prioritization. In addition to the uncertainty introduced by the lack of available experimental property and monitoring data, there is an ongoing discussion on how to evaluate the mobility of chemicals, which means that the definition of what a “mobile” substance is might still change in the future.32 Log
Koc is generally considered a suitable measure for assessing the mobility of neutral organic compounds, as well as for some ionic compounds, because soil and sediment organic carbon (and its cation exchange properties) will rule the sorption for these compounds.33–35 With that said, log
Koc can be highly variable, especially for many ionic compounds, based on pH, ion exchange interactions with soil minerals, and weathering effects, to name a few.36–38 While a log
Koc value <4.0 over a pH range of 4–9 is the current metric for mobility assessment by UBA, discussions are underway to reassess the mobility criteria, namely reducing this log
Koc value from <4 to <3, as a log
Koc value <4 can include several substances which can be highly adsorbed, and thus unlikely to be mobile.32,33 Nevertheless, log
Koc serves as the best measure of mobility for a wide range of compounds. Given the variability and complexity in its measurement, experimental log
Koc values are often not available, and instead log
Kow or log
Dow (for ionizable substances), may be used as an analog to log
Koc. Although log
Kow and log
Dow do not account for the soil sorption properties that log
Koc does, they do provide a measure of the hydrophilicity of the compound and thereby its sorption properties.21,37
4.3.2 Limitations.
An important limitation of this study was that the substances evaluated were all known plastic additives registered for use on the Canadian Domestic Substance list that have been evaluated as persistent and toxic. This means that the study did not cover intermediates, precursors, or transformation products that have PMT properties. Likewise, substances that were not assessed as persistent were not included in the evaluation.
It is also important to note that SimpleTreat models the removal from a conventional WWTP with primary and secondary biological treatment. While this is representative for most conventional WWTPs in Europe and North America,23 the PMT removal in WWTP with tertiary treatment processes could be underestimated in the current analysis. Likewise, the PMT emissions are likely to be higher than what was estimated in this study if only primary treatment is applied. The limited availability of observational data also significantly limited our ability to validate the modelled removal of the analysed PMT substances. To enhance the overall assessment of PMT substances as potential environmental pollutants it is paramount to increase the availability of observational and experimental data on PMT properties and occurrence. The current shortness of experimental and monitoring data is due to a lack of previous regulatory and scientific focus on PMT substances as a group as well as substantial analytical challenges with regards to the detection and quantification of PMT substances. As emphasized in Reemtsma et al.,17 there is a significant gap between the typical physical–chemical property ranges amenable to common analysis techniques like reverse phase liquid chromatography (RPLC) and gas chromatography (GC), and the physical–chemical property ranges of PMT substances. Techniques like mixed-mode liquid chromatography (MMLC) and hydrophilic interaction liquid chromatography (HILIC) are beginning to show promise in analyzing these very polar substances, but many studies only apply this to a small library of PMT substances.39,40 This problem is extended further when considering the number of PMT transformation products that can arise from typical WWTP processes like ozonation, or natural transformations that can occur in the environment.26,29 These transformation products have the potential to be just as, if not more hazardous than their predecessors, further emphasizing the need for robust analytical methods that can identify and characterize such compounds in addition to the growing number of PMT substances.
5. Recommendations
Based on the findings of this study, the use, and emissions of PMT plastic additives and PMT substances in general merit further investigation and environmental monitoring. To understand the fate and potential risk of PMT substances for the environment, water quality, and consequently human health we consider research and development in the following areas as crucial:
5.1 Development of analytical methods for the identification of PMT substances
Sensitive and robust analytical methods play a key role in the future of researching and monitoring PMT substances. Additional research and analytical techniques, as well as standards are needed to enable the investigation of PMT substances as potential environmental contaminants.
5.2 Investigate the occurrence and dominant sources of PMT substances in the Canadian aquatic environment
The current study resulted in a ranked list of PMT plastic additives registered for use in Canada that are expected to have little to no retention in WWTPs. However, it is currently unknown to what extent these compounds are used and emitted. To ground-truth the in silico analysis presented here, the Canadian PMT plastic additive suspect list should be used as a target list for the screening of surface water, stormwater, and influent as well as effluent samples across different watersheds, including the Great Lakes. Such investigation from different geographic locations (from within and outside of Canada) would allow for further refinement of prioritization criteria for PMT plastic additives to be then included in regular monitoring efforts. In the future, the Canadian PMT plastic additive suspect list could be extended to include PMT plastic additives used outside of Canada, further PMT substances (that are not plastic additives) as well as PMT transformation products or more broadly PMOC substances.
5.3 Tools and data to conduct fate and transport and/or risk assessments of priority PMT substances
The lack of experimental physical–chemical property data as well as information on tonnages are substantial barriers to an effective fate and risk analysis of PMT plastic additives and PMT substances in general. More experimental data on physical–chemical properties should be collected and made available for further scientific and regulatory assessment of these compounds. Annual tonnage and specific use characteristics of a substance are especially important parameters that, if made available, would aid in further prioritization of PMT plastic additives, especially when implemented in a comprehensive ranking such as the E-score presented Schulze et al.25 In addition, in silico tools need to be developed or updated to include PMT substances in their applicability domain.
There is a clear need for greater emphasis on the development of experiments, models, and regulations for PMT plastic additives given their abundance and lack of current regulatory mechanisms. This is true in Canada as well as globally as persistent and mobile chemicals pose transboundary environmental and health risks that can only be addressed through integrated regulatory approaches and information exchange.41,42
Conflicts of interest
There are no conflicts to declare.
Acknowledgements
This research was supported by Environment and Climate Change Canada.
References
- M. MacLeod, H. P. H. Arp, M. B. Tekman and A. Jahnke, The global threat from plastic pollution, Science, 2021, 373, 61–65 CrossRef CAS.
- H. P. H. Arp, D. Kühnel, C. Rummel, M. Macleod, A. Potthoff, S. Reichelt, E. Rojo-Nieto, M. Schmitt-Jansen, J. Sonnenberg, E. Toorman and A. Jahnke, Weathering Plastics as a Planetary Boundary Threat: Exposure, Fate, and Hazards, Environ. Sci. Technol., 2021, 55, 7246–7255 CrossRef CAS PubMed.
- E. L. Teuten, J. M. Saquing, D. R. U. Knappe, M. A. Barlaz, S. Jonsson, A. Björn, S. J. Rowland, R. C. Thompson, T. S. Galloway, R. Yamashita, D. Ochi, Y. Watanuki, C. Moore, P. H. Viet, T. S. Tana, M. Prudente, R. Boonyatumanond, M. P. Zakaria, K. Akkhavong, Y. Ogata, H. Hirai, S. Iwasa, K. Mizukawa, Y. Hagino, A. Imamura, M. Saha and H. Takada, Transport and release of chemicals from plastics to the environment and to wildlife, Philos. Trans. R. Soc., B, 2009, 364, 2027–2045 CrossRef CAS PubMed.
- K. Gunaalan, E. Fabbri and M. Capolupo, The hidden threat of plastic leachates: A critical review on their impacts on aquatic organisms, Water Res., 2020, 184, 116170 CrossRef CAS PubMed.
-
C. Meyer and G. Ragutzki, KFKI Forschungsvorhaben Sedimentverteilung als Indikator für morphodynamische Prozesse (MTK 0591), 1999 Search PubMed.
- I. Katsikantami, S. Sifakis, M. N. Tzatzarakis, E. Vakonaki, O. I. Kalantzi, A. M. Tsatsakis and A. K. Rizos, A global assessment of phthalates burden and related links to health effects, Environ. Int., 2016, 97, 212–236 CrossRef CAS PubMed.
-
Environment and Climate Change Canada and Health Canada, Science Assessment of Plastic Pollution, 2020, pp. 32–49 Search PubMed.
- H. Wiesinger, Z. Wang and S. Hellweg, Deep Dive into Plastic Monomers, Additives, and Processing Aids, Environ. Sci. Technol., 2021, 55, 9339–9351 CrossRef CAS.
- J. N. Hahladakis, C. A. Velis, R. Weber, E. Iacovidou and P. Purnell, An overview of chemical additives present in plastics: Migration, release, fate and environmental impact during their use, disposal and recycling, J. Hazard. Mater., 2018, 344, 179–199 CrossRef CAS PubMed.
- C. M. Rochman, C. Brookson, J. Bikker, N. Djuric, A. Earn, K. Bucci, S. Athey, A. Huntington, H. McIlwraith, K. Munno, H. De Frond, A. Kolomijeca, L. Erdle, J. Grbic, M. Bayoumi, S. B. Borrelle, T. Wu, S. Santoro, L. M. Werbowski, X. Zhu, R. K. Giles, B. M. Hamilton, C. Thaysen, A. Kaura, N. Klasios, L. Ead, J. Kim, C. Sherlock, A. Ho and C. Hung, Rethinking microplastics as a diverse contaminant suite, Environ. Toxicol. Chem., 2019, 38, 703–711 CrossRef CAS PubMed.
-
I.-G. England, P. L. Sorensen, E. Hansen and N. H. Nilsson, Hazardous Substances in Plastic Materials TA 3017 2013 Prepared by COWI in Cooperation with Danish Technological Institute, Vejle, 2013 Search PubMed.
- B. Gewert, M. Macleod and M. Breitholtz, Variability in toxicity of plastic leachates as a function of weathering and polymer type: A screening study with the copepod nitocra spinipes, Biol. Bull., 2021, 240, 191–199 CrossRef CAS.
- C. M. Rochman, M. A. Browne, B. S. Halpern, B. T. Hentschel, E. Hoh, H. K. Karapanagioti, L.
M. Rios-Mendoza, H. Takada, S. Teh and R. C. Thompson, Classify plastic waste as hazardous, Nature, 2013, 494, 169–171 CrossRef CAS PubMed.
-
Government of Canada, Toxic substances list - Canada.ca, https://www.canada.ca/en/environment-climate-change/services/canadian-environmental-protection-act-registry/substances-list/toxic.html, accessed 7 January 2022 Search PubMed.
-
Environment and Climate Change Canada, Canada's plastics science agenda - Canada.ca, https://www.canada.ca/en/environment-climate-change/services/science-technology/canada-science-plastic-agenda.html, accessed 7 January 2022 Search PubMed.
-
Government of Canada, Zero plastic waste initiative - Canada.ca, https://www.canada.ca/en/environment-climate-change/services/environmental-funding/programs/zero-plastic-waste-initiative.html, accessed 7 January 2022 Search PubMed.
- T. Reemtsma, U. Berger, H. P. H. Arp, H. Gallard, T. P. Knepper, M. Neumann, J. B. Quintana and P. De Voogt, Mind the Gap: Persistent and Mobile Organic Compounds - Water Contaminants That Slip Through, Environ. Sci. Technol., 2016, 50, 10308–10315 CrossRef CAS.
- R. Sühring, M. L. Diamond, S. Bernstein, J. K. Adams, J. K. Schuster, K. Fernie, K. Elliott, G. Stern and L. M. Jantunen, Organophosphate Esters in the Canadian Arctic Ocean, Environ. Sci. Technol., 2021, 55, 304–312 CrossRef.
-
M. Neumann and I. Schliebner, Protecting the sources of our drinking water: The criteria for identifying persistent, mobile and toxic (PMT) substances and very persistent and very mobile (vPvM) substances under EU Regulation REACH (EC) No 1907/2006 | Umweltbundesamt, https://www.umweltbundesamt.de/publikationen/protecting-the-sources-of-our-drinking-water-the, accessed 7 January 2022 Search PubMed.
- S. E. Hale, H. P. H. Arp, I. Schliebner and M. Neumann, Persistent, mobile and toxic (PMT) and very persistent and very mobile (vPvM) substances pose an equivalent level of concern to persistent, bioaccumulative and toxic (PBT) and very persistent and very bioaccumulative (vPvB) substances under REACH, Environ. Sci. Eur., 2020, 32(155) DOI:10.1186/s12302-020-00440-4.
- H. P. H. Arp, T. N. Brown, U. Berger and S. E. Hale, Ranking REACH registered neutral, ionizable and ionic organic chemicals based on their aquatic persistency and mobility, Environ. Sci.: Processes Impacts, 2017, 19, 939–955 RSC.
- A. J. Williams, C. M. Grulke, J. Edwards, A. D. McEachran, K. Mansouri, N. C. Baker, G. Patlewicz, I. Shah, J. F. Wambaugh, R. S. Judson and A. M. Richard, The CompTox Chemistry Dashboard: A community data resource for environmental chemistry, J. Cheminf., 2017, 9(61) DOI:10.1186/S13321-017-0247-6.
- J. Struijs, SimpleTreat 4.0: a Model to Predict Fate and Emission of Chemicals in Wastewater Treatment Plants Background Report Describing the Equations, RIVM Rapp, 2014, 601353005 Search PubMed.
-
M. Morgan, M. G. Henrion and M. Small, Uncertainty: A Guide to Dealing with Uncertainty in Quantitative Risk and Policy Analysis, Cambridge University Press, Cambridge, New York, 1992 Search PubMed.
- S. Schulze, D. Sättler, M. Neumann, H. P. H. Arp, T. Reemtsma and U. Berger, Using REACH registration data to rank the environmental emission potential of persistent and mobile organic chemicals, Sci. Total Environ., 2018, 625, 1122–1128 CrossRef CAS.
- S. Schulze, D. Zahn, R. Montes, R. Rodil, J. B. Quintana, T. P. Knepper, T. Reemtsma and U. Berger, Occurrence of emerging persistent and mobile organic contaminants in European water samples, Water Res., 2019, 153, 80–90 CrossRef CAS.
-
AMAP, POPs and Chemicals of Emerging Arctic Concern: Influence of Climate Change. Summary for Policy-makers, 2021 Search PubMed.
- T. F. M. Rodgers, J. W. Truong, L. M. Jantunen, P. A. Helm and M. L. Diamond, Organophosphate Ester Transport, Fate, and Emissions in Toronto, Canada, Estimated Using an Updated Multimedia Urban Model, Environ. Sci. Technol., 2018, 52, 12465–12474 CrossRef CAS PubMed.
- H. Rüdel, W. Körner, T. Letzel, M. Neumann, K. Nödler and T. Reemtsma, Persistent, mobile and toxic substances in the environment: a spotlight on current research and regulatory activities, Environ. Sci. Eur., 2020, 32, 1–11 CrossRef.
-
G. of Canada, Canadian Environmental Protection Act, 1999: part 5 - Canada.ca, https://www.canada.ca/en/environment-climate-change/services/canadian-environmental-protection-act-registry/publications/canadian-environmental-protection-act-1999/part-5.html#t6, accessed 1 February 2022 Search PubMed.
- C. Rücker and K. Kümmerer, Modeling and predicting aquatic aerobic biodegradation – a review from a user's perspective, Green Chem., 2012, 14, 875–887 RSC.
-
European Commission, in Ad Hoc Meeting of CARACAL PBT/vPvB/PMT/vPvM Criteria 30 September 2021, 2021, p. 9 Search PubMed.
- S. E. Hale, M. Neumann, I. Schliebner, J. Schulze, F. S. Averbeck, C. Castell-Exner, M. Collard, D. Drmač, J. Hartmann, R. Hofman-Caris, J. Hollender, M. de Jonge, T. Kullick, A. Lennquist, T. Letzel, K. Nödler, S. Pawlowski, N. Reineke, E. Rorije, M. Scheurer, G. Sigmund, H. Timmer, X. Trier, E. Verbruggen and H. P. H. Arp, Getting in control of persistent, mobile and toxic (PMT) and very persistent and very mobile (vPvM) substances to protect water resources: strategies from diverse perspectives, Environ. Sci. Eur., 2022, 34, 1–24 CrossRef.
- G. Bronner and K. U. Goss, Sorption of organic chemicals to soil organic matter: Influence of soil variability and ph dependence, Environ. Sci. Technol., 2011, 45, 1307–1312 CrossRef CAS PubMed.
- C. S. Helling, G. Chesters and R. B. Corey, Contribution of Organic Matter and Clay to Soil Cation-Exchange Capacity as Affected by the pH of the Saturating Solution, Soil Sci. Soc. Am. J., 1964, 28, 517–520 CrossRef CAS.
- H. C. Tülp, K. Fenner, R. P. Schwarzenbach and K. U. Goss, pH-dependent sorption of acidic organic chemicals to soil organic matter, Environ. Sci. Technol., 2009, 43, 9189–9195 CrossRef.
- G. Sigmund, H. P. H. Arp, B. M. Aumeier, T. D. Bucheli, B. Chefetz, W. Chen, S. T. J. Droge, S. Endo, B. I. Escher, S. E. Hale, T. Hofmann, J. Pignatello, T. Reemtsma, T. C. Schmidt, C. D. Schönsee and M. Scheringer, Sorption and Mobility of Charged Organic Compounds: How to Confront and Overcome Limitations in Their Assessment, Environ. Sci. Technol., 2022, 56, 4702–4710 CrossRef CAS PubMed.
- D. Loeffler, A. Hatz, D. Albrecht, M. Fligg, J. Hogeback and T. A. Ternes, Determination of non-extractable residues in soils: Towards a standardised approach, Environ. Pollut., 2020, 259, 113826 CrossRef CAS PubMed.
- S. Bieber, G. Greco, S. Grosse and T. Letzel, RPLC-HILIC and SFC with Mass Spectrometry: Polarity-Extended Organic Molecule Screening in Environmental (Water) Samples, Anal. Chem., 2017, 89, 7907–7914 CrossRef CAS.
- R. Montes, R. Rodil, R. Cela and J. B. Quintana, Determination of Persistent and Mobile Organic Contaminants (PMOCs) in Water by Mixed-Mode Liquid Chromatography-Tandem Mass Spectrometry, Anal. Chem., 2019, 91, 5176–5183 CrossRef CAS PubMed.
- Z. Wang, R. Altenburger, T. Backhaus, A. Covaci, M. L. Diamond, J. O. Grimalt, R. Lohmann, A. Schäffer, M. Scheringer, H. Selin, A. Soehl and N. Suzuki, We need a global science-policy body on chemicals and waste, Science, 2021, 371, 774–776 CrossRef CAS.
- B. Jin, C. Huang, Y. Yu, G. Zhang and H. P. H. Arp, The Need to Adopt an International PMT Strategy to Protect Drinking Water Resources, Environ. Sci. Technol., 2020, 54, 11651–11653 CrossRef CAS PubMed.
|
This journal is © The Royal Society of Chemistry 2022 |