DOI:
10.1039/D2RA07902J
(Paper)
RSC Adv., 2023,
13, 11457-11463
Efficient dispersive solid-phase extraction of methylprednisolone from exhaled breath of COVID-19 patients†
Received
11th December 2022
, Accepted 28th March 2023
First published on 12th April 2023
Abstract
In the current study, bismuth ferrite nano-sorbent was synthesized and utilized as a sorbent for the dispersive solid-phase extraction of methylprednisolone from exhaled breath samples. The size and morphology of the nano-sorbent were characterized by X-ray diffraction analysis and scanning electron microscopy. Following its desorption with acetonitrile, methylprednisolone was quantified by a high-performance liquid chromatography-ultraviolet detector. Factors affecting the extraction of methylprednisolone were optimized. Under optimized experimental conditions, a linear relationship between the analytical signals and methylprednisolone concentration was obtained in the range of 0.001–0.2 μg mL−1 for exhaled breath condensate samples and 0.002–0.4 μg per filter for filter samples. A pre-concentration factor of 6.4-fold, corresponding to an extraction recovery of 96.0%, was achieved. The validated method was applied for the determination of methylprednisolone in real samples taken from the exhaled breath of COVID-19 patients under mechanical ventilation.
1. Introduction
Methylprednisolone (Fig. 1) is a Food and Drug Administration (FDA)-approved medicine used to treat allergic diseases, arthritis, asthma exacerbations, long-term asthma maintenance, acute multiple sclerosis exacerbations, and as an anti-inflammatory and immunosuppressive agent. It belongs to the systemic corticosteroid drug class. This activity discusses methylprednisolone's indications, action, and contraindications as a helpful medication in the treatment of a variety of endocrine, inflammatory, immunologic, hematologic, and respiratory problems.1 In the treatment of COVID-19 patients, a low dose of methylprednisolone was reported to have lower short-term mortality and less need for intensive care unit (ICU) admission and mechanical ventilation, and increased 28 days ventilator-free days without increasing the risk of secondary infections, but it may slightly prolong the duration of viral shedding.2 Therefore, monitoring its concentration in the biological samples will be critical for therapeutic drug monitoring purposes.
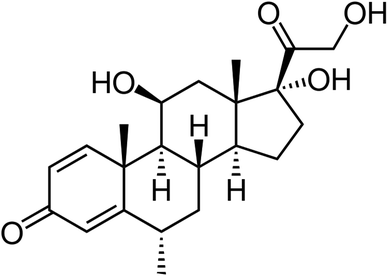 |
| Fig. 1 Molecular structure of methylprednisolone. | |
Some reported methods in the literature for methylprednisolone determination were high-pressure liquid chromatography (HPLC)-orbitrap high-resolution mass spectrometry,3 HPLC-UV,4 liquid chromatography with tandem mass spectrometry (LC-MS/MS),5 voltammetry6 and spectrophotometry.7 On the other hand, various biological samples such as plasma,8 urine,9 serum,10 and central nervous tissue11 were also used as body fluids for its measurements. Before quantitative or qualitative analysis with such analytical instruments, a sample pretreatment step is highly recommended. The main aims of the pretreatment methods are extraction from sample matrices, which mostly interfere with analyte analysis, and pre-concentration to put the analyte concentration in the detectable ranges. Dispersive solid phase extraction (DSPE) is a commonly used extraction technique coupled with chromatographic methods.12 The contemporary improvement on the traditional solid phase extraction (SPE) method is becoming more popular due to its exceptional simplicity, quick extraction time, low solvent cost, excellent effectiveness, and wide applicability.13 Materials used as sorbents provide effectiveness and selectivity for the extraction process. Among various reported sorbents,14,15 nano-sorbents with magnetic properties have emerged as an effective material for manipulating with a magnetic field. A well-known multiferroic material with simultaneous magnetic and electric ordering is bismuth ferrite (BiFeO3-type material),16 which was selected herein as a nano-sorbent for DSPE of methylprednisolone in the exhaled breath of COVID-19 patients under mechanical ventilation.
Non-volatile compounds could be exhaled as small droplets of lung lining fluid. These droplets could be trapped as the condensed phase was collected using a cold trap,17 which is called exhaled breath condensate (EBC).18 The next procedure to trap the droplets is their adsorption on the filters placed in the expiratory circuit of the mechanical ventilators. The filters were used to avoid microbial contamination in the ICU.
The treated samples prepared with the proposed method were injected into the HPLC-UV in the optimized condition for methylprednisolone detection. This study was conducted following our previous work on EBC to provide some new evidence for confirming the acceptability of exhaled breath as a possible alternate sample type for medication monitoring with the aim of early diagnostics or follow-up processes.
2. Experimental section
2.1. Reagents and solutions
All used materials were in the analytical grade, and deionized water was obtained from Ghazi Pharmaceutical Co. (Tabriz, Iran). Acetonitrile and hydrochloric acid were purchased from Scharlau (Barcelona, Spain). Methanol (99.9%), acetone (99%), tetrahydrofuran (THF, 98%), iron(III) chloride hexahydrate (FeCl3·6H2O), bismuth(III) nitrate pentahydrate (Bi(NO3)3·5H2O, 98%), sodium dihydrogen phosphate, sodium acetate, and sodium hydroxide acetic acid (99%) were provided by Merck Co. (Darmstadt, Germany).
2.2. Apparatus
A Knauer HPLC instrument (Berlin, Germany) with a K-1001 HPLC pump, a BioTech degasser, a sample loop (20 μL), and a K-2600 ultraviolet detector was employed in the isocratic mode for the analysis. The chromatographic data processing was performed by employing the Chromgate software (version 3.1). The column was a C18 Xbridge analytical column (5 μm × 250 mm × 4.6 mm) from Waters Co. (Ireland), and the mobile phase consisted of acetonitrile–H2O–tetrahydrofuran (45
:
54.98
:
0.02 v/v) at a flow rate of 1.0 mL min−1. The column oven temperature was adjusted to 25 °C. The UV detector was set at 254 nm.
Powder X-ray diffraction (XRD) data were recorded within the 2θ range of 20–70° using a Siemens diffractometer with filtered Cu-Kα radiation at 35 kV. A scanning electron microscope (SEM) model MIRA III (TESCAN, Czech Republic) was employed to investigate the shape and size of the synthesized nanoparticles. An electronic analytical balance model AB204-S (Mettler Toledo, Greisensee, Switzerland), an ultrasonic bath (Alex machine, Istanbul, Turkey), and a centrifuge model D-37520 (Sigma, Osterode, Germany) were utilized in the current work.
2.3. Preparation of the bismuth ferrite nanoparticles
The bismuth ferrite nanoparticles (BiFeO3 NPs) were synthesized according to a previous report.19 In summary, 4.85 g L−1 of Bi (NO3)3·5H2O and FeCl3·6H2O dissolved in 126.02 g L−1 HNO3 were transferred to a beaker, and 3.0 g of tartaric acid was added to the above solution. The mixture was heated under stirring on a stirrer, and after evaporation of the liquid, the temperature was kept at 160 °C for 1 h. The obtained green powder was calcinated at 600 °C for 2 h and then used for the extraction procedure.
2.4. General procedure
Typically, 0.003 g BiFeO3 NPs were added to 2.0 mL of the diluted sample solution spiked with methylprednisolone in the adjusted pH with phosphate buffer in 7.5. The mixture was sonicated for 10 min to complete the DSPE procedure. Then, the supernatant was separated by an external magnet. Finally, 0.3 mL acetonitrile as an eluent phase was added into the microtube containing sorbent, and the mixture was again sonicated for 10 min. After separation by centrifuging at 10
000 rpm for 10 minutes, 20 μL of the eluent phase was injected into the HPLC-UV, and the methylprednisolone chromatogram was recorded at 254 nm.
2.5. Collection of samples
The used sample in the current study was exhaled breath, which was collected in two forms of EBC and adsorbed on the ventilator filter. EBC samples were collected using a lab-made cooling trap system17 and directly used for the extraction procedure. The methylprednisolone on the filters was eluted with 2 mL of water, pH adjusted with a phosphate buffer at 7.5, and then employed for the procedures explained in the previous section. Sample donors signed a consent form approved by the Ethics Committee of the National Institute for Medical Research Development (approved No. IR.NIMAD.REC.1400.044).
3. Results and discussion
3.1. Characterization of the BiFeO3 NPs
To verify the crystallinity and phase purity of the prepared nanoparticles, XRD analysis was performed, and the relevant pattern is shown in Fig. 2. According to the literature,19 the appearance of distinct 2θ peaks at 2θ = 22 °(100), 31 °(110), 37 °(111), 41 °(200), 51 °(210), and 59 °(211) was ascribed to lattice planes of BiFeO3. Furthermore, these diffraction peaks of the sample prepared at 600 °C can be indexed to rhombohedral BiFeO3 (JCPDS No.71-2494). To obtain further evidence for the successful synthesis and to obtain information on surface morphology and particle size, SEM analysis was performed. Fig. 3, which shows the image at a magnification of 200 nm, reveals the spherical-shaped particles with a particle size of <40 nm for BiFeO3 NPs.
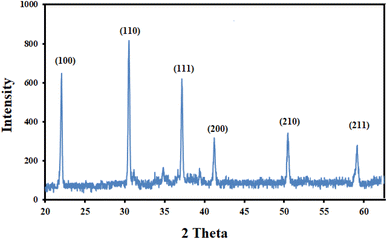 |
| Fig. 2 The XRD pattern of BiFeO3 NPs and its diffraction peaks. | |
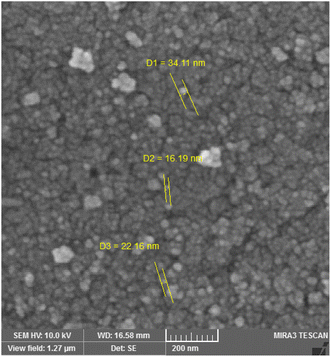 |
| Fig. 3 SEM image of BiFeO3 NPs at the magnification of 200 nm. | |
3.2. Optimization of the experimental conditions
3.2.1. Optimization of chromatographic condition. In order to find appropriate conditions for methylprednisolone determination by HPLC-UV, the composition and flow rate of the mobile phase were investigated. It should be noted that the only available system in our lab is in isocratic mode, and we tried various mobile phases in this mode. For this purpose, several mobile phases including (a) 30
:
70
:
2 v/v of acetonitrile–H2O–acetic acid, (b) 35
:
65
:
2 v/v of acetonitrile–H2O–acetic acid, (c) 40
:
60
:
2 v/v of acetonitrile–H2O–acetic acid, (d) 30
:
70
:
5 v/v of acetonitrile–H2O–acetic acid, (e) 50
:
50
:
2 v/v of acetonitrile–H2O–acetic acid, (f) 50
:
50 v/v of acetonitrile-H2O, (g) 45
:
54.98
:
0.02 v/v of acetonitrile–H2O–THF, and (h) 45
:
54.96
:
0.04 v/v of acetonitrile–H2O–THF were investigated and a good peak vision of methylprednisolone concerning both the shape and symmetry demand was obtained in the 45
:
54.98
:
0.02 v/v of acetonitrile–H2O–THF. The related chromatograms are given in Fig. 1S† in the ESM file, and the peak properties such as asymmetry coefficient, retention factor, and column efficiency are listed in Table 1S.† The results shown in the table also confirmed the good performance of 45
:
54.98
:
0.02 v/v of acetonitrile–H2O–THF as an optimum mobile phase for the isocratic analysis mode of methylprednisolone.Furthermore, the flow rate was changed in the range of 0.8–1.5 mL min−1 and the best peak was observed at a flow rate of 1.0 mL min−1. A typical chromatogram of methylprednisolone in the EBC sample under optimized conditions is shown in Fig. 4.
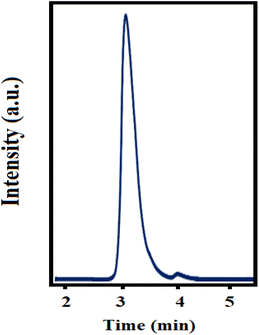 |
| Fig. 4 A typical chromatogram of methylprednisolone with the optimized conditions. | |
3.2.2. Optimization of the extraction conditions. Extraction (adsorption and desorption) parameters for the proposed extraction methods were optimized to reach a maximum recovery value. The experiments were repeated three times, and the averages of the results are reported here. The recovery percentage of methylprednisolone, which was considered an analytical signal, was computed using the amount of methylprednisolone eluted from the employed sorbents and its amount in the initial sample. The main parameters investigated in the optimization step were pH, sorbent amount, eluent type, eluent volume, and desorption and adsorption times. The influence of the pH of the donor sample was evaluated in the range of 4.0–9.5, and as can be seen from Fig. 5A, pH 7.5 showed a maximum value for methylprednisolone recovery. The reason for the high recovery in the basic medium was related to the electrostatic interaction between BiFeO3 NPs (pKa of ∼6.5)20,21 and methylprednisolone (pKa of 12.59).22 So, the pH of 7.5 adjusted with 10 μL of phosphate buffer 9.7 g L−1 was chosen for the next experiments. The effect of the sorbent amount on recovery values was also tested by varying the amount of the sorbent from 0.002–0.005 g. The maximum analytical signal was obtained at 0.003 g of the sorbent (Fig. 5B) and after this value, it remained constant. The type and volume of eluent are other parameters affecting the extraction processes. So, different eluents including acetonitrile, methanol, acetic acid/methanol (2
:
8 v/v), acetic acid/H2O (2
:
8 v/v), and acetic acid/acetonitrile (2
:
8 v/v) were tested for the desorption of the analyte from BiFeO3NPs sorbents. As can be seen from Fig. 5C, acetonitrile was selected as a suitable desorption solvent for BiFeO3NPs. In addition, 0.3 mL of the eluent solvent was sufficient to obtain a satisfactory recovery value (Fig. 5D). Moreover, the effect of adsorption and desorption times on the recovery values was also studied. For this purpose, adsorption and desorption times were changed to the range of 10–40 min. Fig. 5E and F demonstrate that 10 min is sufficient time for both adsorption and desorption procedures.
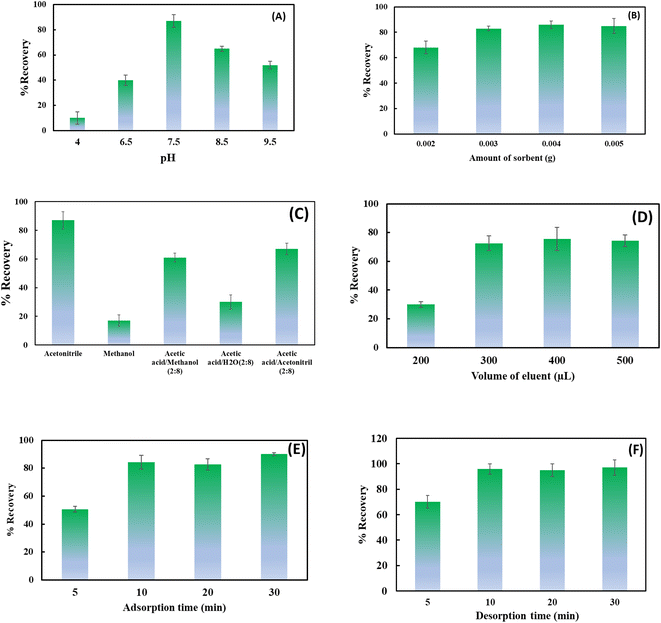 |
| Fig. 5 (A) The influence of pH; (B) sorbent amount; (C) eluent type; (D) eluent volume; (E) adsorption time and (F) desorption time on the methylprednisolone extraction. | |
3.3. Analytical figures of merit
The calibration graph for the proposed method was built by extracting methylprednisolone with different concentrations from spiked samples under optimum experimental conditions. A calibration plot was drawn for the EBC and ventilator filter samples. A linear relationship (R2 = 0.9977 for EBC and 0.9989 for the filter) between the peak area and methylprednisolone concentration was obtained within the concentration range of 0.001–0.2 μg mL−1 for EBC and 0.002–0.4 μg per filter for the filter samples (Fig. 6).
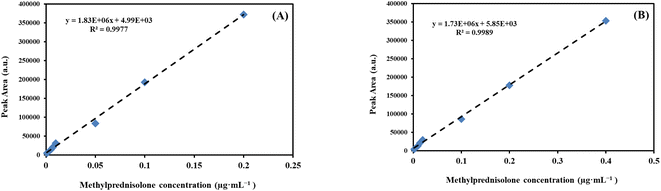 |
| Fig. 6 Calibration curve for methylprednisolone plotted in (A) EBC and (B) ventilator filter samples. | |
The limit of detection (LOD, which was calculated using a signal-to-noise ratio of three) was obtained as 0.0008 μg mL−1 for the developed method for EBC samples and 0.0016 μg per filter for filter samples. The relative standard deviations (%RSD) for inter-day and intra-day analyses (n = 5, methylprednisolone concentration 0.05 μg mL−1) were 3.6% and 7.3%, respectively. Furthermore, the pre-concentration factor and extraction recovery% of the validated method were 6.4 and 96%, respectively, which were calculated according to the below equations.
|
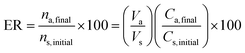 | (1) |
|
 | (2) |
where
ns, initial, and
na, final are the number of moles of methylprednisolone present in the sample solution (donor phase) and the number of moles of methylprednisolone present in the elution solution after extraction, respectively.
Cs, initial and
Ca, final are the initial and final methylprednisolone concentrations in the donor and elution solutions, respectively, whereas
Vs and
Va denote the donor and elution solution volumes, respectively.
The figures of merit of the proposed method were compared with other reported methods in the literature. As can be seen, the current method is comparable with other methods in a linear range and LOD point of view (Table 1).
Table 1 Analytical performance of the current method compared to other reported procedures for methylprednisolone determination
Method |
Sorbent |
Real sample |
Linear range (μg mL−1) |
LOD (μg mL−1) |
Ref. |
High performance liquid chromatography. Liquid chromatography-ion spray mass spectrometry. Liquid chromatography/tandem mass spectrometric. Differential pulse voltammetry. Square wave voltammetry. |
HPLCa-UV |
C18-functionalized magnetic silica nanoparticles |
Rat plasma |
0.1–4 |
0.01 |
8 |
HPLC-UV |
|
Human serum |
0.10–2.95 |
0.02 |
10 |
HPLC-UV |
Polyethylene glycol-modified magnetic carbon nanotubes |
Plasma |
0.01–20 |
0.005 |
23 |
HPLC-UV |
— |
Plasma, saliva, urine |
0.025–4 |
0.01 |
24 |
HPLC-IS-MSb |
— |
Human hair |
0.1–10 ng mg−1 |
0.04 ng mg−1 |
25 |
LC-MS/MSc |
— |
Plasma |
0.01–8.0 |
— |
26 |
DPVd |
— |
Pharmaceutical formulations, human serum and urine |
0.002–0.3 |
— |
6 |
SWVe |
|
Pharmaceutical dosages and human blood plasma |
0.0018–0.18 |
0.0016 |
27 |
HPLC-UV |
BiFO3 NPs |
EBC |
0.001–0.2 |
0.0008 |
This work |
3.4. Selectivity studies
The selectivity was studied in the presence of some co-administered drugs (acetaminophen, alprazolam, codeine, clonazepam, diazepam, oxazepam, and pantoprazole). As can be seen in Fig. 7, at the reported retention time for methylprednisolone, no significant overlap peak was observed. However, in the presence of pharmaceuticals with similar structures, including betamethasone, dexamethasone, and hydrocortisone, a significant overlapping was found, demonstrating that the synthesized sorbent could not distinguish between corticosteroids and could be used for the extraction of all of them. It is obvious that the co-administration of corticosteroids is not common in therapeutics, so this could not be considered a limitation of the proposed method.
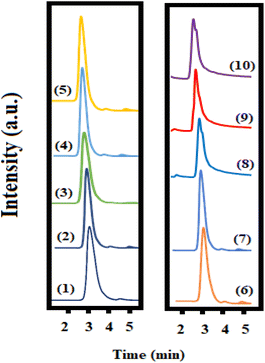 |
| Fig. 7 Selectivity studies for methylprednisolone determination in the presence of some co-administered drugs. (1) methylprednisolone, (2) methylprednisolone + pantoprazole, (3) methylprednisolone + oxazepam, (4) methylprednisolone + diazepam, (5) methylprednisolone + clonazepam, (6) methylprednisolone + alprazolam, (7) methylprednisolone + acetaminophen, (8) methylprednisolone + hydrocortisone, (9) methylprednisolone + dexamethasone, (10) methylprednisolone + betamethasone. | |
3.5. Real sample analysis
In order to investigate the applicability of the developed DSPE-HPLC-UV method for the determination of methylprednisolone in real samples, the method was employed for its extraction/determination from the ventilator filter samples of the patients receiving methylprednisolone treatment. The filter samples were provided during 24 h ventilation. The results of the analysis are summarized in Table 2.
Table 2 Details of the real samples and found concentration of methylprednisolone
No. |
Gender |
Age (year) |
Found concentration (μg per filter) |
1 |
Male |
64 |
0.022 ± 0.01 |
2 |
Male |
64 |
0.072 ± 0.03 |
3 |
Female |
72 |
0.070 ± 0.02 |
4 |
Male |
64 |
0.168 ± 0.05 |
5 |
Female |
72 |
0.132 ± 0.02 |
4. Conclusions
In this study, a DSPE-HPLC-UV based on BiFO3 NPs was validated for the determination of methylprednisolone in exhaled breath samples of COVID-19 patients. Exhaled breath is a simple biological sample that can be collected as EBC, or its non-volatile contents can be sediment on the ventilator filter. This work demonstrated that the DSPE method is a suitable approach to enhance the performance of methylprednisolone extraction from the biological samples with a pre-concentration factor of 6.4-fold and an extraction recovery of 96.0%, which showed promising results (low LOD of 0.0008 μg mL−1 and 0.001–0.2 μg. mL−1 for exhaled breath condensate and 0.002–0.4 μg per filter for the filter) in a short time for methylprednisolone analysis.
Conflicts of interest
The author(s) declare that they have no competing interests.
Acknowledgements
The research reported in this publication was supported by the Elite Researcher Grant Committee under grant number 4000353 from the National Institutes for Medical Research Development (NIMAD), Tehran, Iran.
References
- A. Ocejo and R. Correa, in StatPearls, StatPearls Publishing, 2021 Search PubMed
. - S. Hong, H. Wang, Z. Zhang and L. Qiao, Steroids, 2022, 109022 CrossRef CAS PubMed
. - M. López-García, R. Romero-González and A. G. Frenich, J. Chromatogr. A, 2018, 1540, 21–30 CrossRef PubMed
. - N. Baimani, P. A. Azar, S. W. Husain, H. A. Panahi and A. Mehramizi, J. Chromatogr. A, 2018, 1571, 38–46 CrossRef CAS PubMed
. - D. Dibya, H. Dhiman, B. Anirbandeep, M. H. Sekhar and P. T. Kumar, J. Med. Pharm. Allied Sci., 2021, 10, 2740–2744 CrossRef
. - R. N. Goyal, N. Bachheti, A. Tyagi and A. K. Pandey, Anal. Chim. Acta, 2007, 605, 34–40 CrossRef CAS PubMed
. - O. J. Pozo, J. Marcos, X. Matabosch, R. Ventura and J. Segura, Rapid Commun. Mass Spectrom., 2012, 26, 541–553 CrossRef CAS PubMed
. - P. Yu, Q. Wang, X. Zhang, X. Zhang, S. Shen and Y. Wang, Anal. Chim. Acta, 2010, 678, 50–55 CrossRef CAS PubMed
. - G. Rodchenkov, V. Uralets and V. Semenov, J. Chromatogr. B Biomed. Appl., 1987, 423, 15–22 CrossRef CAS PubMed
. - M. D. Smith, J. Chromatogr. B, 1979, 164, 129–137 CrossRef CAS PubMed
. - P. McGinley, J. Braughler and E. Hall, J. Chromatogr. B, 1982, 230, 29–35 CrossRef CAS PubMed
. - G. Islas, I. S. Ibarra, P. Hernandez, J. M. Miranda and A. Cepeda, Int. J. Anal. Chem., 2017, 8215271 Search PubMed
. - P. Ścigalski and P. Kosobucki, Molecules, 2020, 25, 4869 CrossRef PubMed
. - M. Ghorbani, M. Aghamohammadhassan, H. Ghorbani and A. Zabihi, Microchem. J., 2020, 158, 105250 CrossRef CAS
. - H. Abdolmohammad-Zadeh, F. Morshedzadeh and E. Rahimpour, J. Pharm. Anal., 2014, 4, 331–338 CrossRef CAS PubMed
. - T. Gao, Z. Chen, Q. Huang, F. Niu, X. Huang, L. Qin and Y. Huang, Rev. Adv. Mater. Sci, 2015, 40, 97–109 CAS
. - A. Jouyban, M. Khoubnasabjafari, K. Ansarin and V. Jouyban-Gharamaleki, Breath sampling setup, Iranian patent 81363, 2013
. - E. Rahimpour, M. Khoubnasabjafari, V. Jouyban-Gharamaleki and A. Jouyban, Anal. Bioanal. Chem., 2018, 410, 6411–6440 CrossRef CAS PubMed
. - S. Ghosh, S. Dasgupta, A. Sen and H. Sekhar Maiti, J. Am. Ceram. Soc., 2005, 88, 1349–1352 CrossRef CAS
. - N. D. M. Ridzuan, N. H. M. Kaus, M. A. S. M. Lazim, T. Kobayashi, R. Adnan, M. Z. Othman and M. H. M. Kassim, J. Environ. Chem. Eng., 2020, 8, 104152 CrossRef
. - S.-M. Lam, Z. H. Jaffari, J.-C. Sin, H. Zeng, H. Lin, H. Li, A. R. Mohamed and D.-Q. Ng, J. Mol. Liq., 2021, 326, 115372 CrossRef CAS
. - https://go.drugbank.com/drugs/DB00959.
- P. Yu, H. Ma, Y. Shang, J. Wu and S. Shen, J. Chromatogr. A, 2014, 1348, 27–33 CrossRef CAS PubMed
. - S. M. Al-Habet and H. J. Rogers, J. Pharm. Sci., 1989, 78, 660–666 CrossRef CAS PubMed
. - V. Cirimele, P. Kintz, V. Dumestre, J. Goulle and B. Ludes, Forensic Sci. Int., 2000, 107, 381–388 CrossRef CAS PubMed
. - S. Siddiraju, M. L. L. Prasanth and T. Sirisha, Asian J. Pharm. Sci., 2016, 11, 459–468 CrossRef
. - R. N. Goyal, S. Chatterjee and A. R. S. Rana, Talanta, 2009, 80, 586–592 CrossRef CAS PubMed
.
|
This journal is © The Royal Society of Chemistry 2023 |