DOI:
10.1039/D0QO00567C
(Review Article)
Org. Chem. Front., 2020,
7, 2538-2575
Promising reagents for difluoroalkylation
Received
11th May 2020
, Accepted 6th July 2020
First published on 6th July 2020
Abstract
This review describes recent advances in difluoroalkylation reactions using different substrates. Generally speaking, RCF2 radical is generally proposed in the mechanism of these reactions. At present, the most used difluoroalkylation reagent is RCF2X, which can be reduced by metal, photocatalyst or bases to RCF2 radical. Various substrates such as alkenes, alkynes, amines and so on could well be applied to these difluoroalkylation reactions.
1. Introduction
Because of their special physicochemical properties, difluorinated compounds have an important role in agrochemicals, pharmaceuticals, and materials sciences.1–3 Therefore, the development of efficient and simple methods for the introduction of difluoroalkyl groups has received more and more attention in recent years.4–8 At present, various reagents such as HCF2Cl,9 BrCF2P(O)(OEt)2,10 TMSCF2Br,11,12 HFPO,13 CF3ZnBr2·CH3CN,5 FSO2CF2CO2H14 and so on4,15–18 are used for difluoroalkylation reactions. But some side reactions such as dimerization, protonation and other unknown byproducts accompany the difluoroalkylation reaction when some of these reagents especially metal species are employed. As early as 2001, the Chen group realized the fluoroalkylation of aromatic compounds with per(poly)fluoroalkyl chlorides mediated by sodium dithionite. The single-electron transfer (SET) mechanism was proposed for the fluoroalkylation reaction.18b Difluoroalkyl halides as a class of efficient and readily available reagents for difluoroalkylation reactions have become the most widely used in organic synthesis in recent years. In 2018, the Zhang group summarized transition-metal-catalyzed difluoroalkylation with difluoroalkyl halides via cross-coupling.4 According to the pathways by which the difluoroalkyl halides react, they classified these reactions into four categories: nucleophilic difluoroalkylation, electrophilic difluoroalkylation, radical difluoroalkylation and difluoromethylation by metal–difluorocarbene coupling. There have been some other reviews about difluoroalkylation (CF2R) reactions,4,6,19–22 and these reactions will not be described in this review. Besides metal-catalyzed difluoroalkylation reactions, difluoroalkylation reactions which are conducted under metal-free conditions also have made much progress in recent years, and these reactions will be described in this review. This review mainly describes the difluoroalkylation reactions reported in the last three years. According to the different substrates, we classify these difluoroalkylation reactions into eight types.
2. Difluoroalkylation with alkenes
2.1. Carbodifluoroalkylation with alkenes
2.2. Hydrodifluoroalkylation with alkenes
2.3. Heterodifluoroalkylation with alkenes
2.4. Other difluoroalkylation with alkenes
3. Difluoroalkylation with alkynes
3.1. Carbodifluoroalkylation with alkynes
3.2. Hydrodifluoroalkylation with alkynes
3.3. Heterodifluoroalkylation with alkynes
3.4. Other difluoroalkylation with alkynes
4. Difluoroalkylation with arene
5. Difluoroalkylation with alkyl C–H bond
6. Difluoroalkylation with isocyano
7. Difluoroalkylation with amine
8. Difluoroalkylation with C
N
9. Difluoroalkylation with C
O
2. Difluoroalkylation with alkenes
2.1. Carbodifluoroalkylation with alkenes
In the past few years, a significant renaissance of photocatalysis in the field of organic chemistry has been seen7,23–33 since the pioneer works reported by MacMillan34 and Yoon35 in 2008. With the assistance of visible light, RCF2X could serve as an important synthon for difluoroalkylation reactions in organic chemistry. The reduction of RCF2X in the presence of a photocatalyst was one of the most common strategies for the formation of RCF2 radical. Because of its high reactivity, RCF2 radical could react with various substrates to construct complicated molecules via difuloroalkylation reactions.36–41
In 2018, the Li group demonstrated that difluoroalkylated pyrrolo[1,2-α]indoles with a quaternary carbon center can be efficiently synthesized via difluoroalkylation and cyclization cascade reaction of N-(but-2-enoyl)indoles with BrCF2R (Scheme 1).42 In the presence of visible light, products with good to high yields were generated. A plausible reaction mechanism is outlined in Scheme 1. Initially, RCF2 radical was generated from the oxidation of excited photocatalyst [Ir*3+] with RCF2Br. The addition of RCF2 radical to 1 produced intermediate 2. Subsequently, intermediate 3 was formed from intramolecular radical cyclization of intermediate B on C2 of the indole ring. Then oxidation of intermediate 3 by the [Ir4+] complex occurred to deliver intermediate 4 and the [Ir3+] catalyst. Finally, deprotonation of this cationic intermediate 4 by base afforded the final product.
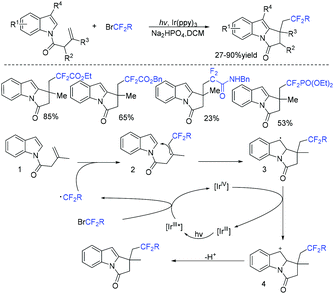 |
| Scheme 1 The difluoroalkylation/cyclization reaction of N-(but-2-enoyl)indoles. | |
This visible light induced radical addition/cyclization strategy could also be applied for the reaction of biaryl vinyl ethers with BrCF2CO2Et (Scheme 2).43 6-Fluoroalkylchromenes with moderate to good yields were obtained. Control experiments indicated that RCF2 radical might be involved in the mechanism, and a radical addition to the carbon–carbon double bond might occur prior to the cyclization process.
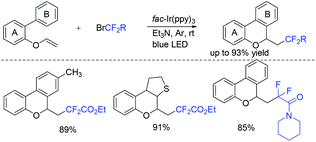 |
| Scheme 2 The radical addition/cyclization of biaryl vinyl ethers. | |
The copper-mediated difluoromethylenation of N-arylacrylamides with benzo-1,3-oxazolic difluoromethylbromide was described by the Jiang group (Scheme 3).44 RCF2 radical, which was generated from Cu(0)-mediated SET with RCF2Br, could add to the terminal C
C double bond of N-arylacrylamide and then underwent intramolecular radical cyclization to form the desired product.
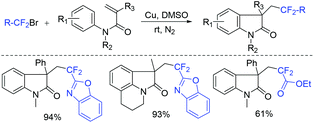 |
| Scheme 3 The copper-catalyzed difluoromethylenation of N-arylacrylamides. | |
In 2019, Feng and coworkers reported the cadmium sulfide quantum-dot-photocatalyzed cascade cyclization of ethyl chlorodifluoroacetate with unactivated olefins (Scheme 4).45 Control experiments showed that a radical intermediate was involved in this reaction. Visible-light-absorbing CdS QDs which could transform difluoromethyl chlorides to difluoromethyl radical played an important role in this strategy.
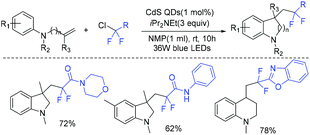 |
| Scheme 4 The cadmium sulfide quantum-dot-photocatalyzed cascade cyclization of unactivated olefins. | |
The Yang group found that CF2-containing benzoxepine derivatives can be synthesized from visible-light-induced external radical-triggered annulation.46 In addition, different products were obtained if the substituents on the nitrogen were different. A plausible mechanism is outlined in Scheme 5. Initially, the excited state [Ir(dtbbpy)(bpy)2PF6]* which was generated under visible-light irradiation was oxidized by BrCF2CO2Et to generate [Ir(IV)(dtbbpy)(bpy)2PF6]+ complex and RCF2 radical species 5. Subsequently, radical intermediate 6 was formed via sequential radical addition between RCF2 radical and the propenyl group of the substrate. Then intermediate 6 was oxidized by [Ir(IV)(dtbbpy)(bpy)2PF6]+ to form iminium intermediate 7 with the concurrent regeneration of [Ir(dtbbpy)(bpy)2PF6]. Finally, the corresponding product was generated from the hydrolyzation of iminium ion C by water (path a). Additionally, when nitrogen was monosubstituted iminium ion, the corresponding enamine was formed via the deprotonation and tautomerization reaction (path b).
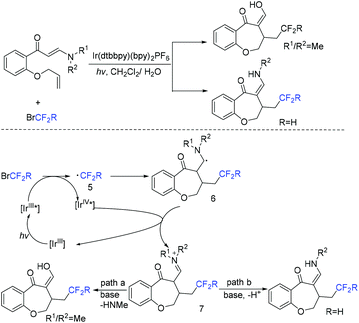 |
| Scheme 5 The synthesis of CF2-containing benzoxepine derivatives. | |
The Sun group found N-arylacrylamides could also react with BrCF2CO2R smoothly for assembly of difluoroalkylphenanthridine derivatives induced by visible light (Scheme 6).47 Besides, fluoroalkylating reagents CF3SO2Cl and BrCF2PO(OEt)2 were also well applicable to this reaction. Fluoroalkyl radical which was generated from oxidative quenching of the excited state PC* by the fluorine reagents was proposed in the mechanism. The subsequent intermolecular radical addition to the C
C double bond of N-arylacrylamides 8 afforded the radical intermediate 10, followed by intramolecular radical addition to the cyano group affording iminyl radical 11. Subsequently the homolytic aromatic substitution process, oxidation and deprotonation reaction occurred in sequence to afford the final products.
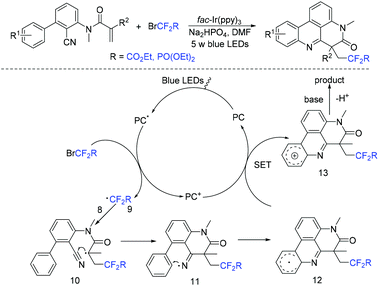 |
| Scheme 6 The assembly of difluoroalkylphenanthridine derivatives. | |
In 2018, the Shi group developed a facile method to construct diverse difluorinated quinoline-2,4-diones via Cu-catalyzed direct difluoromethylation of activated alkenes through a difluoromethyl radical addition/cyclization reaction (Scheme 7). It is noteworthy that visible-light photoredox catalysis was also effective for this reaction.48
 |
| Scheme 7 The synthesis of difluorinated quinoline-2,4-diones catalyzed by copper. | |
Chroman-4-ones are an important motif in medicinal compounds and biologically active molecules.49–51 In 2019, the Zhou group described a visible-light photoredox-catalyzed radical cascade cyclization of alkenyl aldehydes with BrCF2COR for the synthesis of various difluoroacetylated chroman-4-ones (Scheme 8).52 The addition reaction of fluoroalkyl radical 15 to 16 afforded the intermediate 17, which then underwent intramolecular cyclization to give the intermediate 18. Subsequently, 1,2-H shift of E resulted in intermediate 19. Then, intermediate 19 was oxidized by Ir(IV) to afford carbocation 20, which was converted to the desired product by deprotonation.
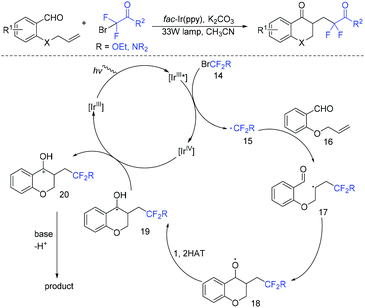 |
| Scheme 8 The cyclization of alkenyl aldehydes with BrCF2COR. | |
Difluoroacetic acids were also found to be good difluoroalkyl radical precursors for decarboxylative difluoroalkylation.53–57 In 2019, the Zhu group realized visible-light-induced acyldifluoroalkylation of alkenyl aldehydes with difluoroacetic acids (Scheme 9).58 PhI(OAc)2 should be added as the oxidant, which could react with the excited state Ir(III)* and difluoroacetic acids via SET process to give difluoromethyl radical.
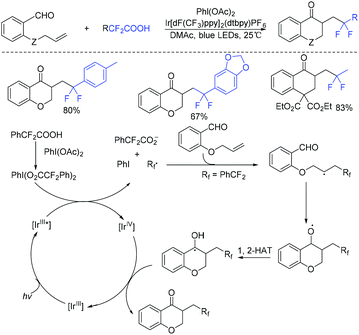 |
| Scheme 9 The acyldifluoroalkylation of alkenyl aldehydes with difluoroacetic acids. | |
The group of Tu and Jiang found that fluoroalkylated (Z)-1-indenones could be efficiently synthesized via hydrofluoroalkylation of 1,6-enynes with ethyl bromodifluoroacetate in the presence of photocatalysts.59 THF behaved as a hydrogen source in this transformation. The proposed mechanism is shown in Scheme 10. Oxidation of Ir*(III) by fluoroalkyl bromides resulted in fluoroalkyl radical 21. The addition of radical 21 to 1,6-enynes generated radical 22, which was followed by cyclization to produce vinyl radical 23. Finally, radical 23 abstracted hydrogen from THF to provide the desired product. In addition, fluoroalkyl and halo groups were all incorporated into 1-indenone skeleton when copper catalysis was used instead of photocatalysis.
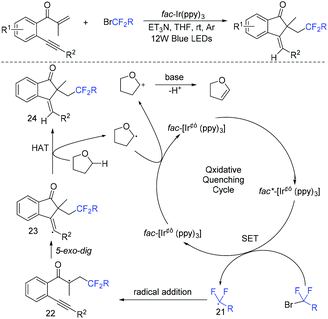 |
| Scheme 10 The synthesis of fluoroalkylated (Z)-1-indenones. | |
The Liang group found that 1,6-enynes were good substrates for difluoroalkylation with ICF2CO2Et (Scheme 11).60 In the absence of metal catalysts, two different difluoroalkylated cyclization products with various functional groups and high stereoselectivity were obtained under the condition that only base was used. The properties of the bases were key factors to give different difunctionalized cyclization products. The reaction of base with ICF2CO2Et to afford CF2CO2Et radical via EDA complex was suggested in the catalytic cycle.
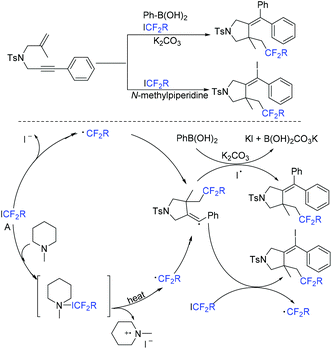 |
| Scheme 11 The difluoroalkylation of 1,6-enynes with ICF2CO2Et. | |
The palladium-catalyzed three-component cascade difluoroalkylation and arylation of 1,6-enynes with ethyl difluoroiodoacetate and phenylboronic acid was realized by the Zhang group (Scheme 12).61 The products can be converted into CF2-containing benzofurans, benzothiophenes and indoles through an Fe(OTf)3-catalyzed isomerization. Reduction of ICF2CO2Et with Pd(0) to form CF2CO2Et radical, transmetalation process between the intermediate 27 and phenylboronic acid process were proposed in the reaction mechanism.
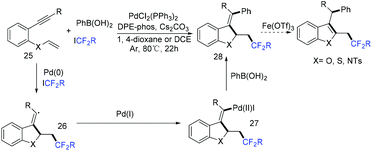 |
| Scheme 12 The synthesis of fluoroalkylated (Z)-1-indenones. | |
Difluoroacyl heterocyclic compounds have been found in various biologically active compounds and agrochemicals.62,63 Much effort has been devoted to these compounds.64–68 In 2019, the copper-mediated radical cyclization of naphthalenyl iododifluoromethyl ketones with olefins was demonstrated by the Wu group (Scheme 13),69 providing difluoroacyl compounds with moderate yields. Mechanistic investigations implied that difluorinated radical intermediate which was generated from the reduction of RCF2I by Cu(0) was involved in the reaction pathway.
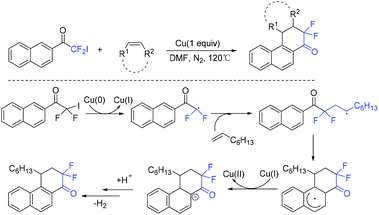 |
| Scheme 13 The copper-mediated radical cyclization of naphthalenyl iododifluoromethyl ketones. | |
In 2019, the Wang group described the copper/B2pin2-catalyzed difluoroalkylation of methylenecyclopropanes with bromodifluorinated acetates and acetamides (Scheme 14).70 Various substrates reacted well in this system. Both the catalyst and an inert atmosphere are necessary for the success of this reaction. A tandem radical process involving ring-opening/intramolecular cyclization was proposed in the catalytic cycle.
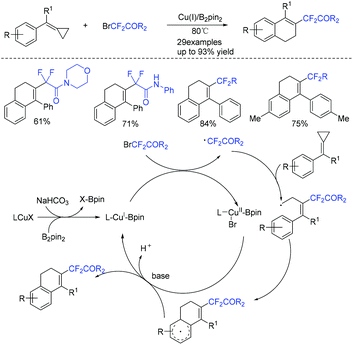 |
| Scheme 14 The copper/B2pin2-catalyzed difluoroalkylation of methylenecyclopropanes. | |
The first difunctionalization of unactivated alkenes through desulfonylation-initiated distal alkenyl migration was reported by the Wang group. Previously unknown 3,3-difluoro-5-styrylpiperidin-2-one derivatives bearing a quaternary stereocenter can be efficiently synthesized using this method (Scheme 15).71 A radical mechanism was suggested for this transformation. Initially, fluoroalkyl radical 29 and Cu(II) species are generated from the oxidation of Cu(I) species by BrCF2R3 through a SET process. Then fluoroalkyl radical 29 selectively attacks the less sterically hindered terminal alkene to give the transient alkyl radical 30. The addition reaction of the RCF2 radical 30 to the internal double bond occurred to generate the cyclized radical 31. Radical 31 will undergo rapid desulfonylation to form the key N-centered radical 32. Finally, an annulation proceeded rapidly and delivered the products 3,3-difluoro-5-styrylpiperidin-2-ones.
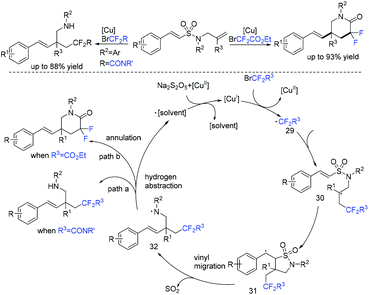 |
| Scheme 15 The difunctionalization of unactivated alkenes through desulfonylation. | |
A novel photocatalytic 1,2-heterocycle migration reaction of allylic alcohols with BrCF2CO2Et was described by the Noel group, affording various β-difluorinated α-aryl heterocyclic ketones (Scheme 16).72 The application of continuous flow allowed shorter reaction times, higher selectivity and opportunities to scale the chemistry. The addition of CF2CO2Et radical generated from the oxidation of fac-[Ir(ppy)3]* to the olefin generated intermediate 33. Then 1,2-heterocycle migration of 33 resulted in 35, which was further converted to the desired product via oxidation. The Cu2O-catalyzed phosphonyldifluoromethylation and ethoxycarbonyldifluomethylation of allylic alcohols through a radical 1,2-aryl migration was also realized by the Xiong group (Scheme 17).73
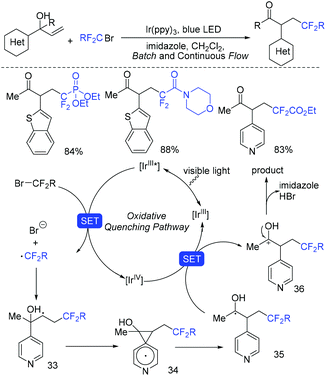 |
| Scheme 16 The photocatalytic difluoromethylation of allylic alcohols. | |
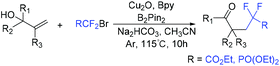 |
| Scheme 17 The Cu2O-catalyzed difluoromethylation of allylic alcohols. | |
Without the need of photocatalyst, a light-mediated Truce–Smiles arylative rearrangement mediated by an organic base was described by the group of Duong and Greaney (Scheme 18).74 This transformation simultaneously introduced an aryl ring and a difluoroacetate moiety across unactivated alkenes. A radical mechanism was proposed for this reaction. Different from previous reports, CF2CO2Et radical could be generated from the reduction of BrCF2CO2Et by TEMDA induced by visible light. The addition reaction of CF2CO2Et radical to alkenes 37 resulted in radical 38. Then, Truce–Smiles aryl shift with extrusion of SO2 and hydrogen atom transfer occurred in sequence to afford the desired product.
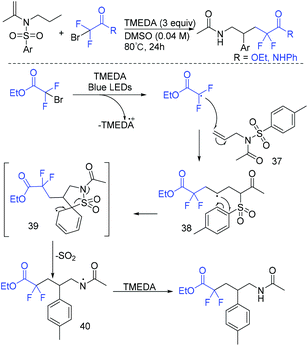 |
| Scheme 18 The light-mediated arylative rearrangement. | |
The visible-light-mediated tandem radical difluoroalkylation and alkynylation of unactivated alkenes with BrCF2COR was realized by the Zhu group (Scheme 19).75 However, an aliphatic alkynyl substrate was not suitable for this transformation because of the rearrangement of the starting material and undesired decomposition. The C–C bond activation via intramolecular 1,4-alkynyl migration was involved in the mechanism.
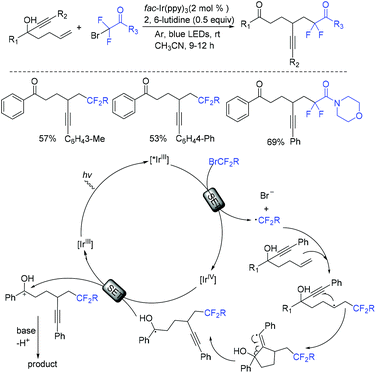 |
| Scheme 19 The light-mediated difluoroalkylation and alkynylation of unactivated alkenes. | |
Another organic base-promoted difluoroalkylation reaction of 1,4-enynes with ICF2CO2Et was realized by yje Liang group.76 Various functional groups were compatible with this reaction. A possible mechanism is outlined in Scheme 20. The direct interaction of N-methylpiperidine with ICF2CO2Et was proposed to form CF2CO2Et radical. Alkynyl radical migration of 41 was also proposed in the mechanism.
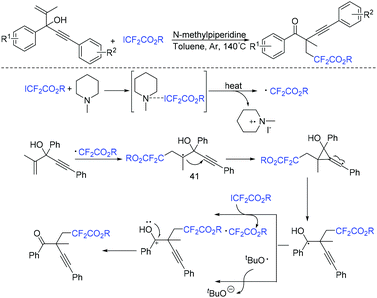 |
| Scheme 20 The base-promoted difluoroalkylation reaction of 1,4-enynes with ICF2CO2Et. | |
The first intermolecular alkynyl-difluoroalkylation of unactivated alkenes via visible-light-induced three-component reaction of difluoroalkyl halides, unactivated alkenes and alkynyl sulfones was realized by the Zhu group (Scheme 21).77 This reaction provides a simple and efficient route to the construction of synthetically valuable β-difluoroalkylated alkynes. The reaction of Ir*III and RfX occurred to form difluoroalkyl radical, which was followed by addition to alkenes to afford intermediate II. Then the addition of intermediate II to alkynyl sulfones and β-elimination occurred in sequence to produce the final product. It is worthwhile to note that Et3N plays multiple roles such as a base and a reductant in this reaction. Additionally, Ir(II)/Ir(III) pathway was also possible for this transformation.
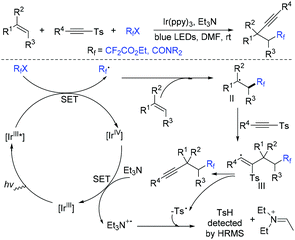 |
| Scheme 21 The alkynyl-difluoroalkylation of unactivated alkenes. | |
As is well known, allenes are highly reactive substrates and play an important role in organic chemistry.78,79 A nickel-catalyzed 1,4-carbofluoroalkylation of 1,3-enynes with boronic acids and fluoroalkyl halides to access structurally diverse fluoroalkylated allenes was reported by the Wang group (Scheme 22).80 The use of nickel to generate fluoroalkyl radicals from BrCF2CO2Et (rate-determining step) and reductive elimination were proposed for this reaction.
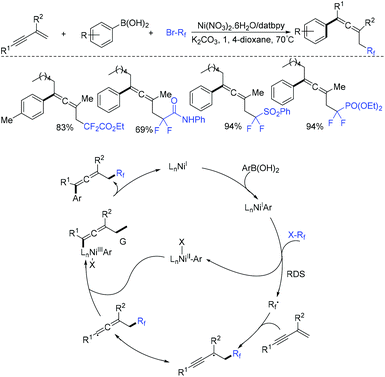 |
| Scheme 22 The nickel-catalyzed 1,4-carbofluoroalkylation of 1,3-enynes. | |
Besides 1,3-enynes, alkenes are also good substrates to react with boronic acids and fluoroalkyl halides, producing γ-arylation of carbonyl compounds. The Shu group found that the employment of copper and visible-light catalysis was essential for this reaction.81 The mechanism is shown in Scheme 23. The reduction of BrCF2CO2Et with excited Ir*(III) photocatalyst would give radical intermediate 42 and the oxidized photocatalyst Ir(IV). 42 would be trapped by alkenes to generate a new alkyl radical intermediate 43. In the presence of a base, the arylcopper intermediate 44 was delivered from the reaction of Cu(I) with aryl boronic acid, which was followed by recombination with 43 to give 45. Then, 45 would undergo reductive elimination to give the final product with the concurrent regeneration of Ir(III) and Cu(I) species.
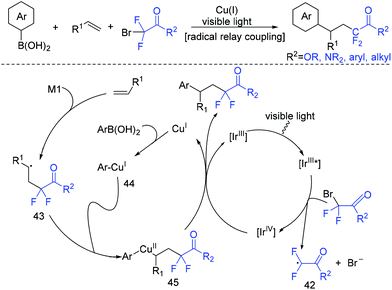 |
| Scheme 23 The process of γ-arylation of carbonyl compounds. | |
In 2019, the perfluoroalkylative pyridylation of alkenes via 4-cyanopyridineboryl radicals was developed by the Li group (Scheme 24).82 Density functional theory calculations and experimental studies suggested that the 4-cyanopyridineboryl radicals generated from 4-cyanopyridine and B2(pin)2 played an important role in the catalytic cycle, which not only activates C–X bond homolysis but also serves as a pyridine precursor. The selective cross-coupling of the resulting alkyl radicals generated from the addition reaction of RCF2 radicals to alkenes and 4-cyanopyridineboryl radicals delivered the corresponding product with a quaternary carbon center.
 |
| Scheme 24 The perfluoroalkylative pyridylation of alkenes. | |
In 2019, another three-component reaction of fluoroalkyl halides, alkenes and arenes was reported by the Liang group.83 An unprecedented three-component direct C–H addition was achieved in the challenging meta-selective fashion catalyzed by Ru. Control experiments and computational analyses were carried out to investigate the mechanism. The plausible catalytic cycle is outlined in Scheme 25. Initially, carboxylate-assisted C–H ruthenation occurred to give 46, which was followed by the reaction with fluoroalkyl halide and alkenes via SET to afford 47 and radical 48. Subsequently, the newly formed radical bond added to the para-position of the C–Ru bond, leading to species 49, which was followed by rearomatization to give ruthenacycle 50. Finally, the meta-functionalization product was delivered by demetalation, with the release of ruthenium(II) complex.
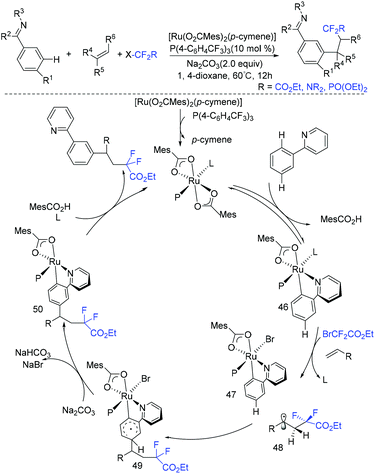 |
| Scheme 25 The three-component reaction of fluoroalkyl halides, alkenes and arenes. | |
In 2018, the Li group found that the 1,2-alkylarylation reaction of styrenes, α-carbonylalkyl bromides and N,N-disubstituted anilines could be conducted in the presence of photoredox and copper cooperative catalysis (Scheme 26).84 In addition to Friedel–Craftss alkylation, this reaction provided a new access for the C–H alkylation of N,N-disubstituted anilines with high para-selectivity. Carbonylalkyl bromides such as primary, secondary and tertiary α-bromoalkyl ketone esters, malonic esters and cycloalkane were all good partners for this reaction. The product with 79% yield was obtained when BrCF2CO2Et was employed. CF2CO2Et radical which was generated from the reduction of BrCF2CO2Et with the excited state Ru(bpy)3*2+ was proposed in the mechanism.
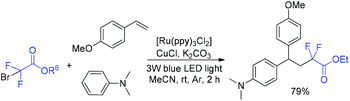 |
| Scheme 26 C(sp3)–Br/C(sp2)–H functionalization mediated by photoredox and copper cooperative catalysis. | |
Recently, the asymmetric three-component Minisci reaction of quinolines or pyridines with α-bromocarbonyl compounds and enamides mediated by dual photoredox and chiral Brønsted acid catalysis was presented by Studer and coworkers (Scheme 27).85 A range of valuable chiral γ-amino acid derivatives are obtained with good to excellent enantioselectivities. But when BrCF2CO2Et was used in this system, only 42% yields and 69
:
31 er value was isolated.
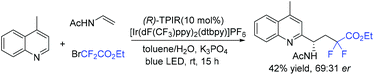 |
| Scheme 27 Alkenes for difluoromethyl radical addition. | |
The group of Li and Han demonstrated that N-heterocyclic carbene (NHC) could also catalyze radical difluoroalkylation of olefins.86 The dearomative difunctionalization of indoles could be readily achieved via this reaction. The mechanism is presented in Scheme 28. Firstly, the reaction of NHC catalyst, aldehyde and base occurred to give Breslow intermediate 51, which was followed by SET with fluoroalkyl reagent RCF2X to afford fluoroalkyl radical and a persistent ketyl radical 55. Subsequently, radical 54 generated from the addition of fluoroalkyl radical to styrene was trapped by radical 55 through a radical–radical cross-coupling pathway to form intermediate F. Finally, the fluoroketone product was obtained with the release of NHC.
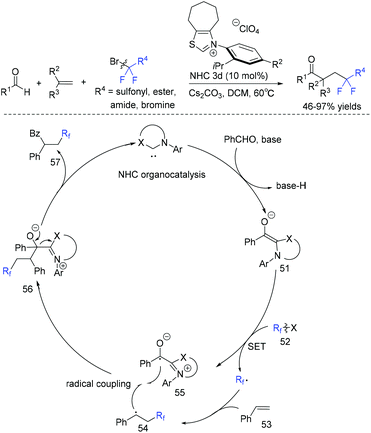 |
| Scheme 28 The N-heterocyclic carbene-catalyzed radical difluoroalkylation of olefins. | |
2.2. Hydrodifluoroalkylation with alkenes
In 2018, visible-light-induced 1,6-difunctionalizations of alkenes with BrCF2CO2Et were realized by the Nevado group (Scheme 29).87 The addition reaction of CF2CO2Et radical which was generated from the reduction of BrCF2CO2Et by [Ir]III* with double bond afforded a vicinal radical intermediate. Subsequently, a remote benzylic radical was generated via 1,5-HAT, which can be trapped with O- or C-nucleophiles to produce new Csp3–O and Csp3–Csp2 bonds at room temperature.
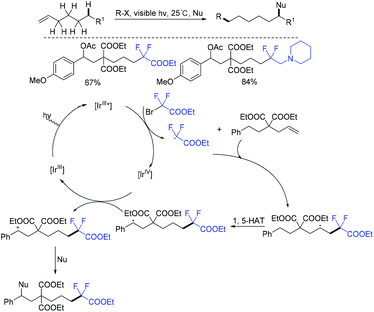 |
| Scheme 29 The visible-light-induced 1,6-difunctionalizations of alkenes. | |
In the same year, a series of difluoroalkylated ketones or aldehydes was obtained by the group of Luo and Cheng via Cu-catalyzed oxidation of alcohols with BrCF2CO2Et (Scheme 30).88 The catalytic oxidation of alcohols and difluoroalkylation of alkenes were concurrently realized in this reaction, enabling a highly efficient and attractive method for organic synthesis. Similarly, radical difluoroalkylation of alkenes, intramolecular 1,5- or 1,6-HAT, SET oxidation and deprotonation reactions were proposed in the mechanism.
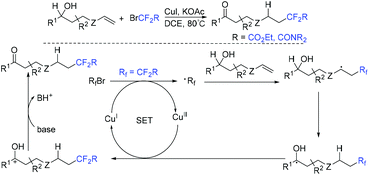 |
| Scheme 30 The Cu-catalyzed oxidation of alcohols with BrCF2CO2Et. | |
Besides alcohols, the group of Ma and Li demonstrated that difluoroalkylated ketones could also be synthesized via visible-light-mediated cascade remote oxyfluoroalkylation of alkenes employing dimethyl sulfoxide as the oxidant and solvent (Scheme 31).89 From the plausible mechanism we can see that the alkene fluoroalkylation-initiated remote benzyl C–H bond activation via 1,5-H migration in a highly controlled site-selective manner and Kornblum reaction with dimethyl sulfoxide as the oxidant played a key role in this reaction.
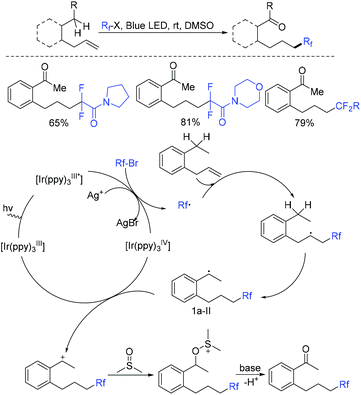 |
| Scheme 31 The Cu-catalyzed oxidation of alcohols with BrCF2CO2Et. | |
The group of Zhou and Chen realized the reaction of methylene-2-oxazolines with difluoroalkylating reagents via a photoredox strategy (Scheme 32).90 Difluoroalkylated oxazoles with diverse functionalities were prepared using this method. Tandem radical addition of CF2CO2Et radical and subsequent oxidative aromatization steps were proposed in the reaction mechanism.
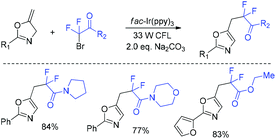 |
| Scheme 32 The difluoroalkylation of alkenes. | |
The photoredox-catalyzed reaction of α,β-dehydroamino acids and peptides with BrCF2CO2Et was demonstrated by the Mancheño group, providing various of non-natural amino acids and peptides (Scheme 33).91 Additionally, arylsulfonyl chlorides or various N-(acyloxy)phthalimides (NHPI esters) were also effective for this reaction. In the catalytic cycle, PC* was reduced by Hantzsch ester to form PC*− and HE-radical cation. Then PC*− reacted with BrCF2CO2Et to produce CF2CO2Et radical and the photocatalyst PC. Subsequently, the addition reaction of CF2CO2Et radical and the double bond of the α,β-dehydroamino acid resulted in radical I, which was followed by reduction and protonation reaction to provide the desired product.
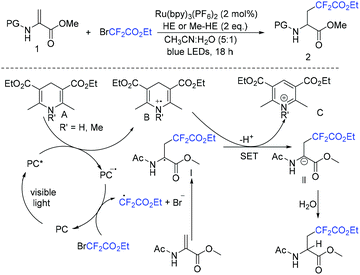 |
| Scheme 33 The photoredox-catalyzed reaction of α,β-dehydroamino acids and peptides with BrCF2CO2Et. | |
2.3. Heterodifluoroalkylation with alkenes
Due to its thermodynamic stability and kinetic inertness, it is challenging to utilize CO2 for organic synthesis. Many methods have been developed to harness CO2 for the synthesis of various organic compounds up to now.92–99 In 2018, a selective difluoroalkylation of allylamines with carbon dioxide via visible-light photoredox catalysis was developed by the Yu group.100 Various 2-oxazolidinones with difluoroalkyl groups were generated. A plausible mechanism is outlined in Scheme 34. Ru(I) which was generated from the reductive quenching of excited photocatalyst Ru(II)* by DABCO reduces BrCF2R to CF2R radical. Then carbamate 58 which was generated from 59 and CO2in situ reacted with CF2R radical to give benzylic radical 60. Then oxidation of 60 by excited Ru(II)* and intramolecular cyclization of 61 occurred in sequence to afford the final product 62.
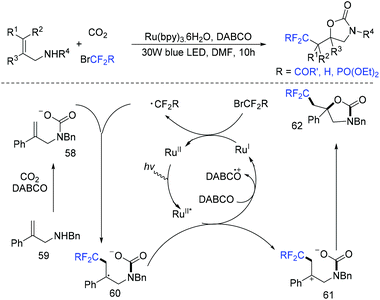 |
| Scheme 34 The difluoroalkylation of allylamines with carbon dioxide. | |
The cyclization of alkenyl carboxylic acids with difluoroalkyl reagents for the synthesis of difluoroalkylated lactones has been established in recent years. The Wu group developed a palladium-catalyzed reaction for the synthesis of difluoroalkylated lactones (Scheme 35a).101 In 2017, the iridium-catalyzed photoredox lactonization for the synthesis of difluoroalkylated lactones was realized by the Han group (Scheme 35b).102 In 2018, the group of Li realized the copper(I)-catalyzed difluoroalkylation of alkenyl carboxylic acids under mild reaction conditions (Scheme 36).103 The highly valued CF2-containing lactones were delivered in moderate to excellent yields. A radical-involved pathway was proposed for this catalytic reaction. Firstly, an initial SET from Cu(I)Ln 63 to RCF2Br occurred to generate RCF2 radical and Cu(II). Subsequently, the addition reaction of RCF2 radical to unsaturated carboxylic acid delivered the difluoroalkyl radical intermediate 64. Then, the carbocation intermediate 65 was formed by oxidation of 64, concurrently regenerating the active copper(I) catalyst. Finally, the desired product was obtained through an intramolecular nucleophilic attack (Scheme 36, path a). Another pathway involving the Cu(III) intermediate 66 followed by the reductive elimination under basic conditions to generate the final product was also possible (Scheme 36, path b). In the same year, another copper-catalyzed difluoroalkylation of alkenyl carboxylic acids for the synthesis of lactone was also reported by the Wang group (Scheme 37).104 A Cu(I)/Cu(II) mechanism was suggested for this transformation.
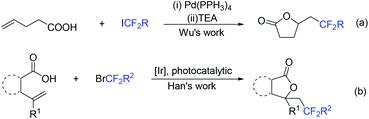 |
| Scheme 35 The cyclization of alkenyl carboxylic acids with difluoroalkyl reagents. | |
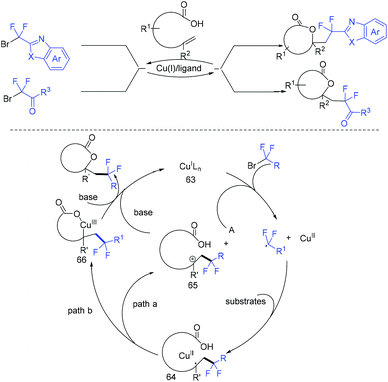 |
| Scheme 36 The copper-catalyzed cyclization of alkenyl carboxylic acids with difluoroalkyl reagents. | |
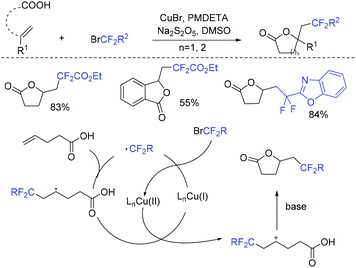 |
| Scheme 37 The copper-catalyzed cyclization of unsaturated carboxylic acids. | |
The Yang group demonstrated that bromodifluoromethylphosphonates are also good reagents for the construction of difluoroalkylated lactones (Scheme 38).105 The reaction of bromodifluoromethylphosphonates with alkenyl acids was smoothly carried out in the presence of visible light. Besides acids, alcohols and sulfonamides were also suitable for this system. CF2P-containing heterocycles can be obtained efficiently. Similar to the production process of RCF2 radical, the oxidative quenching process of the excited fac-[Ir(ppy)3]* with diisopropyl bromodifluoromethylphosphate via SET to afford CF2PO(OiPr)2 radical was involved in the reaction mechanism.
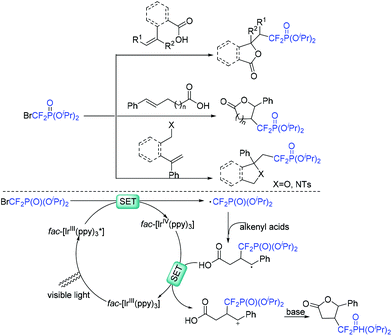 |
| Scheme 38 The reaction of bromodifluoromethylphosphonates with alkenyl acids. | |
Copper-catalyzed intramolecular oxydifluoroalkylation of hydroxyl-containing alkenes for the synthesis of various fluoroalkylated tetrahydrofurans was also described by the group of Wang and Guo.106 Besides ethyl bromodifluoroacetate, other functionalized difluoroalkylated bromides were also well employed in this system. The proposed mechanism of this reaction is shown in Scheme 39. First, RCF2 radical 67 and Cu(II) intermediate were generated from the oxidation of Cu(I) by ethyl bromodifluoroacetate. The addition reaction of radical 67 and alkenes occurred to generate intermediate 68, which was trapped by Cu(II) in the presence of base Na2CO3 to form intermediate 69. Then two possible intermediates 70 or 71 may be formed. The desired product can be generated from 70via nucleophilic attack of activated alcohol toward carbon cation species. Alternatively, intermediate 71 which undergoes reductive elimination could also deliver the desired product. These two pathways are possible simultaneously. In 2019, this group demonstrated that when amine-containing olefins were used as substrates instead of hydroxyl-containing alkenes, diverse difluorinated nitrogen-containing polycycles were obtained (Scheme 40).107 Oxidation of Cu(I) species by BrCF2CO2Et through a SET to afford an electrophilic RCF2 radical and Cu(II) species was also described in the proposed mechanism. Recently, the photoredox-catalyzed oxydifluoroalkylative cyclization of alkenes using RfI was also reported by the Luo group.108
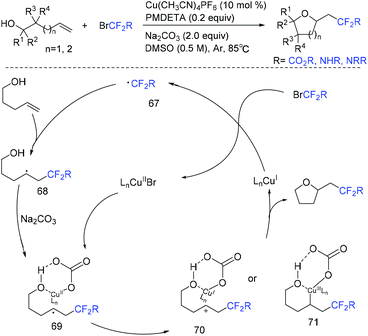 |
| Scheme 39 The copper-catalyzed intramolecular oxydifluoroalkylation of hydroxyl-containing alkenes. | |
 |
| Scheme 40 The synthesis of difluorinated nitrogen-containing polycycles. | |
Distal functional group migration was also observed in the reaction of alkenes with BrCF2CO2Et (Scheme 41).109 In combination with visible-light photocatalysis, difluorinated alkyl ketones were readily obtained with high yields. Besides heteroaryl group, imino, formyl, and alkynyl groups were also well tolerated in this system. Radical addition, functional group migration and oxidation reaction were proposed in the plausible mechanism.
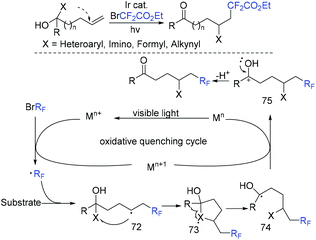 |
| Scheme 41 The synthesis of difluorinated alkyl ketones. | |
The Song group found that the copper/B2pin2 system was effective for difluoroalkylation-thiolation of arylalkenes (Scheme 42).110 B2pin2 as an organic reductant played an important role in this transformation, which enables the simultaneous installation of a C(sp3)–C(F2R) bond and a C(sp3)–S(R) bond by utilizing two electrophilic substrates. Radical trapping experiments suggested that the CF2CO2Et radical was involved in the reaction mechanism.
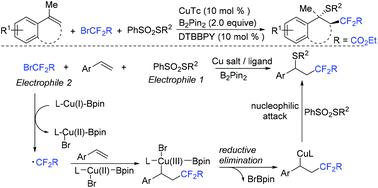 |
| Scheme 42 The light-mediated Truce–Smiles rearrangement. | |
The three-component difluoroalkylation–peroxidation of alkenes with difluorohaloactates and hydroperoxides catalyzed by Co(acac)2 was developed by the Li group (Scheme 43).111 Various β-peroxyldifluoroalkyl derivatives were accessed efficiently via this strategy. In addition, other halides were also tolerated in this reaction, giving the alkylation–peroxidation products. CF2CO2Et radical generated from the reduction of BrCF2CO2Et by Co(II) added to alkenes to form radical 76. Then tert-butylperoxy radical or cobalt tert-butylperoxy 77 which were generated from the reaction of cobalt(III) species with hydroperoxide react with radical 76 to afford the desired product.
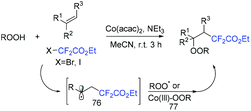 |
| Scheme 43 The three-component difluoroalkylation–peroxidation of alkenes with difluorohaloactates and hydroperoxides. | |
In 2019, the difluoroalkylation–thiolation of alkenes with BrCF2CO2Et and RSH mediated by iron was developed by the Cai group (Scheme 44).112 Csp3–Csp3 and Csp3–S bonds were simultaneously formed in this reaction. FeCl2 which can be used to activate thiols via Fe/S complex has been documented.113 A possible reaction mechanism was proposed as shown in Scheme 44. A radical intermediate 79 and an oxidized photocatalyst fac-Ir4+(ppy)3 were generated from the oxidation of fac-Ir3+(ppy)3* with BrCF2CO2Et. Then the radical 79 added to styrene 80, giving a benzyl radical intermediate 81. On the other hand, the reaction of Fe(II) with 4-methylbenzenethiol delivered an iron–sulfur intermediate 82 in the presence of a base, which was followed by recombination with 81 to produce intermediate 84. Finally, a SET occurred between 84 and fac-Ir4+(ppy)3, providing the desired product, along with the liberation of FeCl2. In the same year, the same group114 found that three-component difluoroalkylamination of alkenes with BrCF2CO2Et and amines could also be realized under similar reaction conditions (Scheme 45). Besides thiols and amines, alcohol was also found to be suitable for this type of reaction by the Nishikata group (Scheme 46).115 The copper-mediated radical and cation crossover mechanism was involved in the reaction pathway.
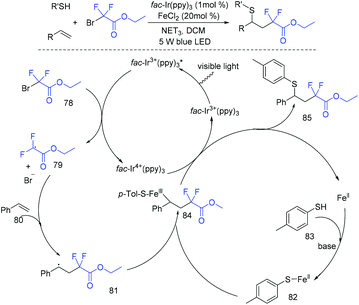 |
| Scheme 44 The difluoroalkylation–thiolation of alkenes with BrCF2CO2Et and RSH. | |
 |
| Scheme 45 The difluoroalkylation–thiolation of alkenes with BrCF2CO2Et and amines. | |
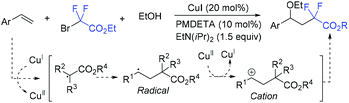 |
| Scheme 46 The difluoroalkylation–thiolation of alkenes with BrCF2CO2Et and alcohols. | |
1,4-Diazabicyclo[2.2.2]octane-bis(sulfur dioxide) adduct (DABSO) as an inexpensive and readily available reagent has been widely used in organic transformations.116–118 In 2019, Chen and coworkers achieved a zinc-mediated intermolecular reductive radical fluoroalkylsulfination of unsaturated C–C bonds with fluoroalkyl bromides and DABSO (Scheme 47).119 Alkenes and alkynes were all tolerated in this reaction, affording the product with moderate yields. Preliminary mechanistic investigations revealed that a sulfur dioxide anion radical species reduced by Zn from SO2 was involved in this reaction.
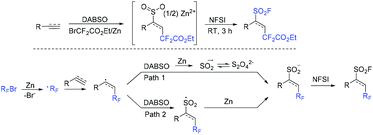 |
| Scheme 47 The difluoroalkylation–thiolation of alkenes with BrCF2CO2Et and alcohols. | |
Due to side reactions, nickel-catalyzed dialkylation of alkenes remains underdeveloped. In 2019, a nickel-catalyzed three-component difluoroalkylation–alkylation of N-vinyl-2-pyrrolidinone with difluoroalkyl bromides and dialkylzinc reagents was reported by the Zhang group (Scheme 48).120N-Vinyloxazolidinone and N-vinylacetamide can also be applied to this system. BrCF2COOEt was used for this reaction, the corresponding product being obtained in 51% yield. Two mechanisms initiated by Ni(I) or Ni(II) were possible for this reaction. As a key intermediate, radical 86 was generated in both mechanisms.
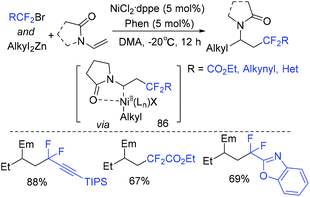 |
| Scheme 48 The difluoroalkylation–thiolation of alkenes with BrCF2CO2Et and alcohols. | |
The Shi group found that CF2CO2Et radical was also a good partner to react with alkenyl diboronate complexes (Scheme 49).121 In the presence of Ru photocatalyst, gem-bis(boryl)alkanes were formed from the reaction of alkenyl diboronate with diverse alkyl halides. Alkyl radicals added efficiently to the alkenyl diboronate complexes. Then the adduct radical anions undergo radical-polar crossover and 1,2-boryl-anion shift from boron to the α-carbon to afford the final product.
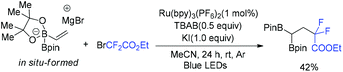 |
| Scheme 49 The reaction of alkenyl diboronate complexes with BrCF2CO2Et. | |
In 2018, the difluoroacetylation of alkenes using ethyl bromodifluoroacetate (BrCF2CO2Et) catalyzed by copper was reported by the Zhu group (Scheme 50).122 In the presence of a base, BrCF2CO2Et was reduced by the complex of CuI/B2Pin2 to generate CF2COOEt radical 91 along with Cu(II) 92. Then, radical 91 added to the terminal carbon of alkenes to give carbon radical intermediate E, which can be oxidized by 92, trapped by bromide ion to afford the desired product. Alternatively, intermediate 93 coupled with 92 to afford Cu(III) complex which was followed by reductive elimination to afford product with the release of Cu(I). This pathway was also possible.
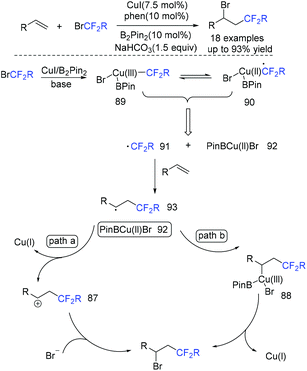 |
| Scheme 50 The copper-catalyzed difluoroacetylation of alkenes. | |
The He group demonstrated that organophosphine was a good catalyst for atom transfer radical addition of alkenes with ethyl iododifluoroacetate (Scheme 51).123 Substrate-controlled selectivity was observed in this difluoroacetylation. When styrenes were subjected to this system, a Heck-type difluoroalkylated product was furnished. Difluoroalkylated alkanes were obtained when alkenes were used. A noncovalent interaction between the phosphine catalyst and carbon–iodine bond was suggested for this reaction.
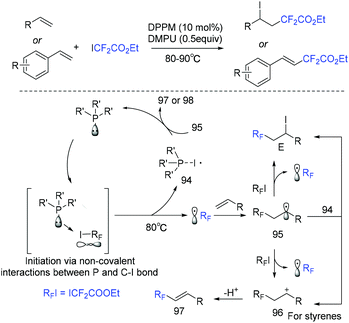 |
| Scheme 51 The organophosphine-catalyzed difluoroalkylation of alkenes. | |
The base-controlled selective (phenylsulfonyl)difluoromethylation of alkenes with PhSO2CF2I was observed by the Wang group (Scheme 52).124 Both atom transfer radical addition and Heck-type products could be obtained via simply tuning the organic or inorganic bases in the presence of visible light. The oxidative quenching of *Ru(bpy)2+ with PhSO2CF2I was proposed in the mechanism. Both types of products can be formed via two pathways.
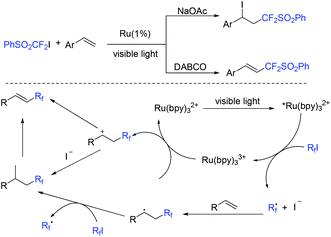 |
| Scheme 52 The (phenylsulfonyl)difluoromethylation of alkenes with PhSO2CF2I. | |
The photoredox-catalyzed oxydifluoroalkylation of alkenes with BrCF2CO2R using dimethyl sulfoxide (DMSO) as an oxidant was reported by Tang and coworkers (Scheme 53).125 From the proposed mechanism we can see that AgTFA as the additive assisted the formation of CF2CO2R radical via oxidative quenching of *Ir(ppy)3 with BrCF2CO2R. The final products were isolated via Kornblum oxidation of carbocation 100 with DMSO.
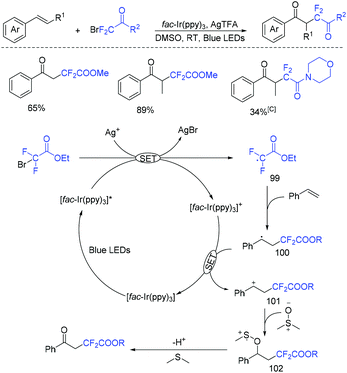 |
| Scheme 53 The photoredox-catalyzed oxydifluoroalkylation of alkenes with BrCF2CO2R using dimethyl sulfoxide. | |
2.4. Other difluoroalkylation with alkenes
The palladium-mediated vinylic C–H difluoroalkylation of ketene dithioacetals with BrCF2CO2Et was realized by the group of Wang and Zhu in 2018 (Scheme 54).126 Two SET processes were involved in the reaction mechanism. Owing to the unique structural characters of ketene dithioacetals, a Pd(0)/Pd(I) catalytic cycle was proposed for this reaction, which was different from the known palladium catalyzed Heck-type reaction of alkenes with fluoroalkyl bromides.
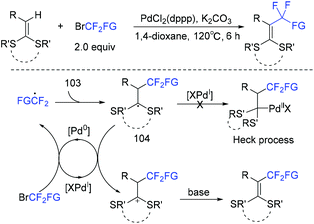 |
| Scheme 54 The palladium-mediated C–H difluoroalkylation of ketene dithioacetals. | |
Xu and coworkers reported a visible-light-induced cross-coupling reaction of para-quinone methides (p-QMs) with difluoroalkylating reagents (Scheme 55).127 A series of difluoroalkylated diarylmethane compounds with pharmaceutical potential was accessed. From the plausible mechanism we can see that the excited photocatalyst, *fac-Ir(ppy)3, was primarily quenched by p-QMs to afford the diarylmethane radical intermediates, which was followed by a radical–radical cross-coupling reaction with difluoroalkyl radicals to deliver the final product. Amine could react as reductant with both Ir(IV) and BrCF2CO2Et to produce Ir(III) and CF2CO2Et radical respectively.
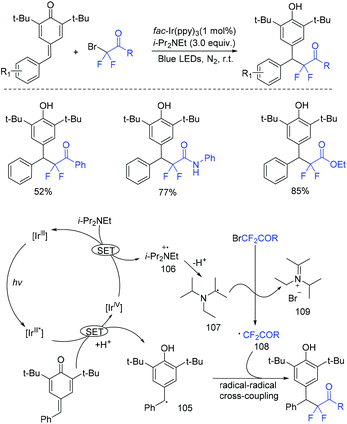 |
| Scheme 55 The visible-light-induced difluoroalkylation reaction of p-QMs. | |
Muñoz-Molina and Perez found that [Cp*RuCl(PPh3)2] complex showed good catalytic activity for the Heck-type alkenylation of alkyl bromides (Scheme 56).128 Tertiary, secondary, and primary alkyl bromides could all react smoothly in this reaction. The corresponding product could be obtained in 91% yield when BrCF2CO2Et was used. Hünig's base played an important role for this transformation. Preliminary mechanistic studies support the participation of alkyl radicals in the reaction. The mechanism is outlined in Scheme 56. Initially, the formation of carbon-centered radicals by a SET process was induced by the half-sandwich ruthenium(II) complex, which was followed by the anti-Markovnikov addition with the olefin to deliver another radical species. Then the new radical species reduced the ruthenium(III) center to ruthenium(II), with the release of intermediate 110. Finally, the desired product was obtained via base-induced elimination reaction. Additionally, the amine acts as a reductant to regenerate the Ru(II) catalyst from Ru(III).
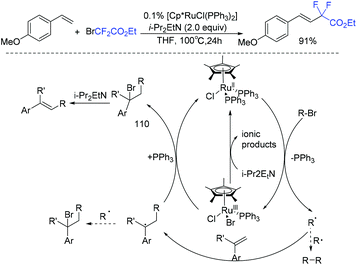 |
| Scheme 56 The Ru complex-catalyzed Heck-type alkenylation. | |
The visible-light-induced coupling of difluoromethyl halides with allyl sulfones for the synthesis of 4,4-difluoroalkenes was reported by the Ryu group (Scheme 57).129 With the help of catalytic Ru(bpy)3Cl2, diisopropylethylamine, and Hantzsch ester, the reaction proceed effectively within 2 h. In the catalytic cycle, Ru(I) was reduced by HEH and/or amine from the photoexcited Ru(II)* via a SET process. Then another SET process occurred between Ru(I) and BrCF2CO2Et to generate CF2CO2Et radical, which was followed by an addition reaction with C and β-fragmentation to furnish the product. In 2020, the Yao group found that the decarboxylative allylation of α,α-difluoroarylacetic acids with allyl sulfones can also be catalyzed by silver in water.130 Preliminary mechanistic investigations suggest that a radical process and β-elimination might be involved.
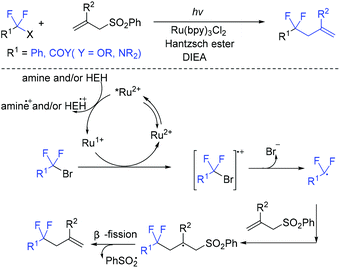 |
| Scheme 57 The visible-light-induced coupling of difluoromethyl halides with allyl sulfones. | |
The photoinduced decarboxylative difunctionalization of maleic anhydride with amine and fluorinated alkyl iodides was reported by the Zhang group (Scheme 58).131 A variety of fluorinated alkyl halides and amines reacted smoothly in this reaction. The difluoroalkyl radical which was generated from oxidative quenching reaction between fluorinated alkyl iodides and excited Ru(II)* was also possible in this reaction.
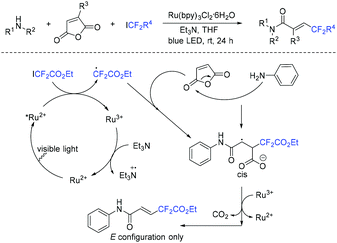 |
| Scheme 58 The photoinduced decarboxylative difunctionalization of maleic anhydride with amine and fluorinated alkyl iodides. | |
Coumarins are an important class of heterocyclic compounds which display a wide range of biological activities.132–135 In 2019, the same group found that difluoroalkylation of ortho-hydroxycinnamic esters with BrCF2CO2Et can proceed smoothly through a visible-light-induced cascade radical cyclization process (Scheme 59). A variety of 3-fluoroalkylated coumarins were successfully prepared under mild conditions. Similarly, RCF2 radical species was also proposed in the reaction cycle.136
 |
| Scheme 59 The difluoroalkylation of ortho-hydroxycinnamic esters. | |
3. Difluoroalkylation with alkynes
3.1. Carbodifluoroalkylation with alkynes
In 2015, the Fu group reported the visible-light-promoted aryldifluoroacetylation of alkynes with ethyl bromodifluoroacetate for the synthesis of 3-difluoroacetylated coumarins (Scheme 60).40 A wide range of functional groups was tolerated in this reaction. A tandem radical cyclization process which involved RCF2 radical was proposed for this reaction.
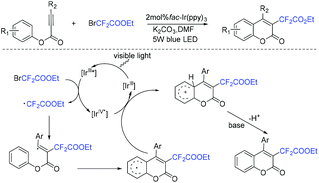 |
| Scheme 60 The visible-light-promoted aryldifluoroacetylation of alkynes with ethyl bromodifluoroacetate. | |
A mild and efficient photocatalytic protocol for the synthesis of difluoroacetylindenones via the reaction of 1,3-diarylpropynones with ethyl bromodifluoroacetate was established by Rastogi and coworkers (Scheme 61).137 The visible-light-catalyzed difluoroacetylation of 1,3-diarylpropynones followed by intramolecular radical cyclization was proposed for this reaction.
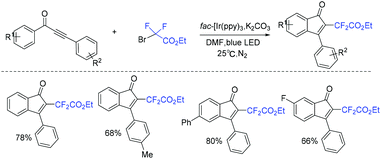 |
| Scheme 61 The synthesis of difluoroacetylindenones via the reaction of 1,3-diarylpropynones with ethyl bromodifluoroacetate. | |
In the presence of Ir(ppy)3 as photocatalyst, the group of Li and Hu found that N-benzyl-N-(2-ethynylaryl)amides were good partners to react with ethyl bromodifluoroacetate for the synthesis of substituted dibenzazepines (Scheme 62).138 Two carbon-centered functional groups are introduced across the C
C bond in a single reaction. The Xu group found that this reaction can also smoothly proceed in the presence of organic photocatalysts (cat-PMP) (Scheme 63).139 Fluoroalkyl radical which formed from the reduction of BrCF2CO2Et by excited-state cat-PMP* was proposed in the catalytic cycle. Then intermolecular addition of fluoroalkyl radical RCF2 to N-benzyl-N-(2-ethynylaryl)amides occurred to provide radical 112, which was followed by intramolecular addition to form intermediate 114. Intermediate 114 was oxidized by cat-PMP˙+ to afford 115. Finally, intermediate 115 undergoes deprotonation to furnish the desired product. On the other hand, another possible pathway (path b) was also possible for this reaction.
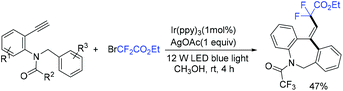 |
| Scheme 62 The synthesis of substituted dibenzazepines catalyzed by Ir(ppy)3. | |
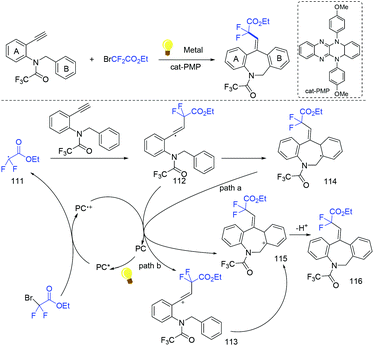 |
| Scheme 63 The synthesis of substituted dibenzazepines catalyzed by organic photocatalysts. | |
Copper-catalyzed cascade radical addition/dearomative spirocyclization of biaryl ynones to access difluoromethylated spiro[5.5]trienones was first realized by the Zhu group (Scheme 64).140 This reaction is useful for the construction of spiro compounds. When Ag was employed as catalyst, monofluoromethylated and phosphorated spiro[5.5]trienones were also obtained using diethylphosphite as substrate. Fluoroalkyl radical was proposed in the reaction mechanism. A vinyl radical 117 was formed via the addition of RCF2 radical to C–C triple bond, then 6-exo-trig cyclization occurred to afford intermediate 119 which is oxidized by Mn+1 to form oxocarbenium ion 120. After demethylation, the desired product was obtained.
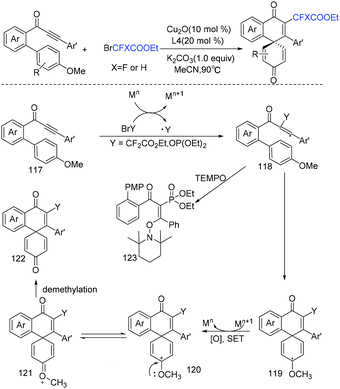 |
| Scheme 64 The copper-catalyzed cascade radical addition/dearomative spirocyclization of biaryl ynones. | |
In 2019, the group of Ackermann and Li demonstrated that cobalt was a good catalyst to promote the cyclization of fluoroalkyl radical with C–C triple bond (Scheme 65).141 Various fluorinated chromenes were synthesized via cobalt-catalyzed difluoroalkylation/Giese radical conjugate cyclization manifold with various alkyne-tethered cyclohexadienones and halogenated fluorinating reagents. Similar to copper catalyst, the Co catalyst could also reduce ethyl bromodifluoroacetate to produce difluoroalkyl radical. Subsequently, the addition of difluoroalkyl radical with alkynes resulted in alkenyl radical 127. Then intermediate 127 undergoes an intramolecular Giese radical conjugate addition to afford the key intermediate 128, which was followed by reduction by Zn, along with hydrolysis, providing the desired product, with the release of the active Co(I)Ln complex.
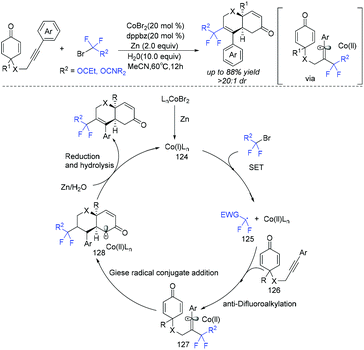 |
| Scheme 65 The cobalt-promoted cyclization of fluoroalkyl radical with C–C triple bond. | |
Cyclopropenes having unique rigid structure and variable reactivity have attracted much interest.142–145 In 2019, the Ma group146 reported the first highly enantioselective RhII-catalyzed cyclopropenation reaction of unactivated internal alkynes with a difluorodiazoethane reagent (PsDFA) (Scheme 66). A broad range of enantioenriched unsaturated three-membered carbocycles can be obtained smoothly. Subsequently, various reactions such as crosscouplings, hydrogenation, Diels–Alder reaction, and Pauson–Khand reaction could occur to demonstrate the synthetic utility of the strained carbocycles.
 |
| Scheme 66 The palladium-catalyzed difluoroalkylation reaction of N-propargylamides. | |
3.2. Hydrodifluoroalkylation with alkynes
The group of Wang and Zhao found that the hydrodifluoroalkylation of alkynes with ethyl bromodifluoroacetate could proceeded smoothly mediated by copper.147 Metabisulfite as reduction agent should be used for this reaction to suppress the homocoupling of terminal alkynes. It can be seen from Scheme 67 that CF2COOEt radical was generated from the reaction of BrCF2COOEt and Cu(I) via SET process. Then addition reaction with (phenylethynyl)copper 130, hydrogen abstraction from solvents and protonation by moisture occurred in sequence to give the final product.
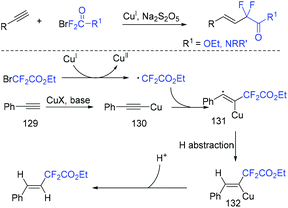 |
| Scheme 67 The copper-catalyzed hydrodifluoroalkylation of alkynes with ethyl bromodifluoroacetate. | |
3.3. Heterodifluoroalkylation with alkynes
A facile method for the synthesis of C2-tetrasubstituted indolin-3-ones via copper-catalyzed redox cycloisomerization of nonprefunctionalized nitroalkynes with BrCF2CO2Et was achieved by the Song group (Scheme 68).148 Using diboron as the reductant, this reaction proceeded in a one-pot protocol forming a fluorine-containing non-carbon quaternary center. One of the oxygens from NO2 was captured by B2pin2 with the aid of Na2CO3. Another oxygen in NO2 was transformed into C
O bond of C2-tetrasubstituted indolin-3-ones.
 |
| Scheme 68 The synthesis of C2-tetrasubstituted indolin-3-ones. | |
In 2019, the same group developed a visible-light-promoted cyclization of methylthiolated alkynones with BrCF2CO2Et for the synthesis of 2-substituted thiochromones.149 The desired products could be accessed with moderate to good yields via C(sp2)–S bond formation and C(sp3)–S cleavage. Besides CF2CO2Et group, phosphoryl, sulfenyl, and acyl groups are also well tolerated in this method. The reaction mechanism is depicted in Scheme 69. Firstly, the fluoroalkyl radical was generated from the corresponding conditions. Then the C–C triple bond in alkynones was attacked by fluoroalkyl radical to give vinyl radical 133. Finally, 6-exo-trig cyclization of 133 with the SMe moiety gave the desired product along with the release of methyl radical.
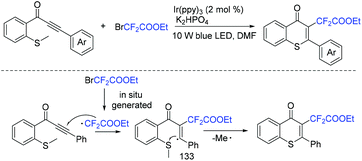 |
| Scheme 69 The cyclization of methylthiolated alkynones with BrCF2CO2Et for the synthesis of 2-substituted thiochromones. | |
The carbon radical intermediates generated from the electrophilic radical addition of CF2CO2Et radical with alkynes can be trapped by an oxygen nucleophile to form difluoro-substituted heterocycles.40,150,151 In 2018, a palladium-catalyzed difluoroalkylation reaction of N-propargylamides with ethyl iododifluoroacetate for the synthesis of difluoromethyl oxazoles and oxazolines was reported by the Liang group (Scheme 70).152 A Pd(0)/Pd(I)/Pd(II) cycle was proposed for the reaction mechanism. Additionally, perfluoroalkyl iodides were also suitable for the generation of perfluoroalkyl heterocycles.
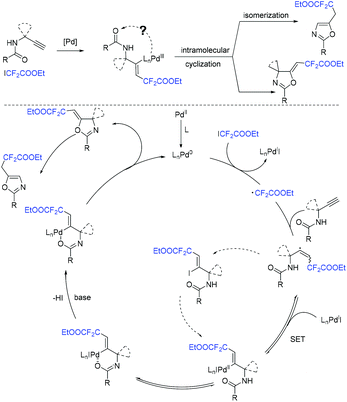 |
| Scheme 70 The palladium-catalyzed difluoroalkylation reaction of N-propargylamides. | |
An efficient cobalt-catalyzed difluoroalkylation of alkynes/alkenes for the construction of fluorinated hydrocarbons was described by Jacobi von Wangelin and coworkers.153 However, alkyl-substituted terminal alkynes were not effective in this system. This was the first cobalt-catalyzed reaction with high stereoselectivity. The proposed reaction mechanism is shown in Scheme 71. Different from related cobalt-catalyzed reductive couplings and Heck-type reactions, terminal halogen atom transfer mediated by Co catalyst was involved in the radical chain mechanism, which avoided the use of a stoichiometric sacrificial reductant. The RFCoIIIX complex which delivered the free RF radical and mediated the halogen atom transfer to the terminal vinyl radical played an important role in the catalytic cycle.
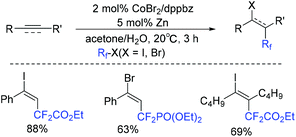 |
| Scheme 71 The cobalt-catalyzed difluoroalkylations of alkynes/alkenes. | |
In 2018, a palladium-mediated three-component reaction of alkynes with fluoroalkyl halides and diboron reagents was developed by the group of Zhu and Bai (Scheme 72).154a Both internal and terminal alkynes were tolerated in this reaction, providing the functionalized 1,2-fluoroalkylboronated alkenes with excellent regio- and stereoselectivity. In the catalytic cycle, a SET process was also proposed between fluoroalkyl halides and Pd(0) to afford RCF2 radical and Pd(I) species. Then the addition reaction of RCF2 radical and alkynes took place to give vinyl radical. Then oxidative addition and base-assisted transmetalation with B2pin2 delivering the alkenyl(boryl)palladium intermediate 137 and reductive elimination of 137 occurred successively to give the desired product (path a). Alternatively, path b featuring the direct reaction of vinyl radical I with Pd(I) was also possible. But this pathway was less likely. The palladium-catalyzed trans-fluoroalkylation–borylation of alkynes with fluoroalkyl iodides and B2pin2 was also realized by the Zhang group.154b
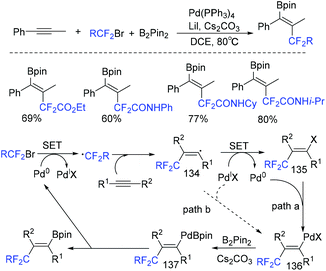 |
| Scheme 72 The palladium-mediated three-component reaction of alkynes with fluoroalkyl halides and diboron reagents. | |
In 2018, the Liang group found that air-stable SCF3 and SeCF3 reagents could act as free-radical initiators of ethyl iododifluoroacetate via reduction reaction (Scheme 73).155 In the presence of air-stable SCF3 and SeCF3 reagents, the difluoroalkylation reaction of alkynes with ethyl iododifluoroacetate proceeded smoothly. β-Proton elimination was successfully avoided. Besides alkynes, alkenes were also suitable in this reaction. A Cu(I)–Cu(II)–Cu(III) mechanism was described for this reaction.
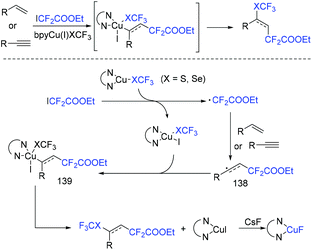 |
| Scheme 73 The copper-catalyzed difluoroalkylation reaction of alkynes with ethyl iododifluoroacetate. | |
A photocatalyst-free fluoroalkylation reaction of alkynes with fluoroalkyl iodides was developed by the He group (Scheme 74).156 It is worth noting that the noncovalent interactions between acetone and fluoroalkyl iodides could assist the formation of fluoroalkyl radical. Additionally, (hetero)arenes and alkenes were also tolerated in this transformation.
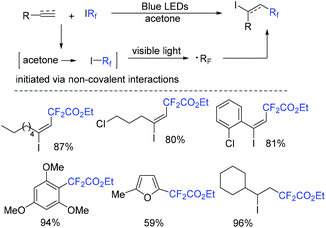 |
| Scheme 74 The photocatalyst-free fluoroalkylation reaction of alkynes with fluoroalkyl iodides. | |
3.4. Other difluoroalkylation with alkynes
In 2019, the visible-light-induced alkynyl-difluoroalkylation of alkynyl halides with fluoroalkyl halides to access a diverse range of fluoroalkylalkynes was described by the Cho group (Scheme 75).157 Controlled experiments suggested a radical mechanism was possible. SET from fac-Ir(ppy)3* to fluoroalkyl halide generated the oxidized photocatalyst fac-Ir(ppy)4 and the RF2 radical. The fac-Ir(ppy)4 could be reduced by TMEDA to form fac-Ir(ppy)3 complex. Then vinyl radical 141 was formed via the addition reaction of RCF2 radical and alkynyl halide, which could be converted to the final product via dehalogenation.
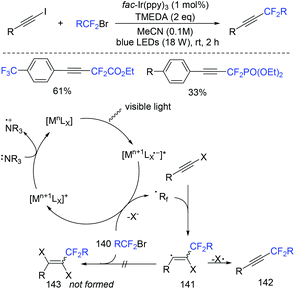 |
| Scheme 75 The visible-light-induced alkynyl-difluoroalkylation of alkynyl halides with fluoroalkyl halides. | |
In 2019, a palladium-catalyzed ligand-free difluoroalkylation of alkynes with iododifluoroacetones for the construction of difluorinated 1,3-enynes was developed by the Wu group (Scheme 76).158 The radical addition of RCOCF2 radical which was generated from reduction of iododifluoroacetones with Pd(PPh3)4 to alkynes and subsequent Sonogashira coupling with the same alkynes were involved in the reaction mechanism.
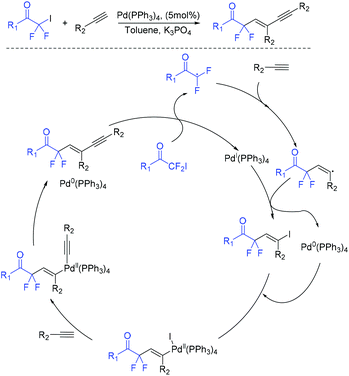 |
| Scheme 76 The construction of difluorinated 1,3-enynes. | |
4. Difluoroalkylation with arene
In 2018, an efficient para-selective C–H difluoromethylation of aromatic ketones catalyzed by tetrakis(triphenylphosphine)palladium(0) was developed by the Zhao group (Scheme 77).159 Additionally, several well-known drugs such as oxybenzone, ketoprofen, zaltoprofen, and propafenone were also well tolerated in this system. The role of AgF was unclear, it being likely to assist the palladium complex in activating the aromatic ring. A mechanistic study suggested that a highly para-selective difluoromethylation reaction may result from the palladium complex coordinated to the carbonyl group. SET between Pd(I) and BrCF2CO2Et took place to afford CF2CO2Et radical and Pd(II). Then CF2COOEt radical coupled with complex 144 which came from the coordination of Pd(II) intermediate with aromatic ketone to give intermediate 145. Subsequently, intermediate 145 was converted to the final product via SET process and aromatization, with the liberation of Pd(I) catalyst. Almost at the same time, similar work was described by the group of Xu and Lou (Scheme 78).160
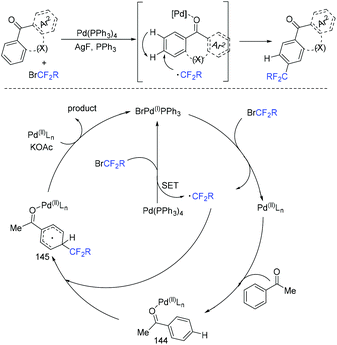 |
| Scheme 77 The para-selective C–H difluoromethylation of aromatic ketones. | |
 |
| Scheme 78 The para-selective C–H difluoromethylation of aromatic ketones. | |
In 2019, Li and coworkers found that para-selective C–H difluoroalkylation of aromatic carbonyls could also be induced by visible light.161 In the presence of Ir(ppy)3 as the catalyst and 1,10-phenanthroline as the additive, various products could be obtained efficiently. The proposed mechanism is shown in Scheme 79. Concurrently, irradiation of IrIII(ppy)3 resulted in excited IrIII(ppy)3*, which could be oxidized by BrCF2CO2Et via a SET process with the formation of CF2CO2Et radical and IrIV(ppy)3. Then the para-selective addition of CF2CO2Et radical to aryl carbonyls occurred to produce intermediate 146, which could be converted to radical anion intermediate 147 by the reverse process of deprotonation. Finally, intermediate 147 was oxidized by IrIV to give the product via rearomatization. In the same year, similar reactions of visible-light-induced difluoroalkylation of aromatic aldehydes were developed by the Xu group (Scheme 80).162
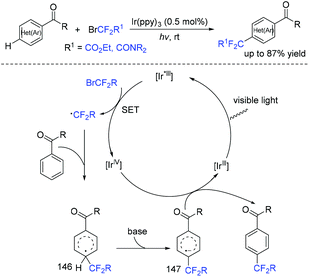 |
| Scheme 79 The para-selective C–H difluoromethylation of aromatic ketones. | |
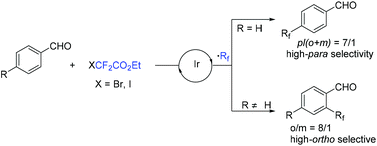 |
| Scheme 80 The para-selective C–H difluoromethylation of aromatic aldehydes. | |
Using ruthenium(II) as a catalyst, para-selective C–H difluoromethylation of ketoxime ethers was also realized by the Zhao group, providing the corresponding para-difluoromethylated products in moderate to good yield (Scheme 81).163 Mechanistic studies showed that chelation-assisted cycloruthenation may play an important role in the para selectivity of the difluoromethylation of ketoxime ethers. Different from Zhao's work, meta-C–H alkylation of arenes catalyzed by Ru was described by Ackermann in the presence of visible light (Scheme 82a).164 Both tertiary and secondary alkyl bromides were compatible with this reaction. When BrCF2CO2R was employed, the product was isolated in 48% yield. Recently, the Ru-catalyzed meta-C–H bond difluoroalkylation of 2-phenoxypyridines was realized by the Cui group (Scheme 82b).165
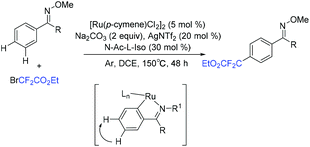 |
| Scheme 81 The para-selective C–H difluoromethylation of ketoxime ethers. | |
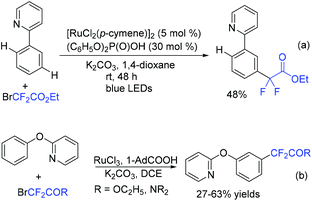 |
| Scheme 82 The meta-C–H alkylation of arenes catalyzed by Ru. | |
The ruthenium(II)-catalyzed para- and meta-selective C–H difluoroalkylation of aniline derivatives with BrCF2CO2Et was reported by the Liang group (Scheme 83).166 Mechanistic investigation implied that the key factor of the para-selective difluoroacetylation derived from the cycloruthenation that occurred at N–H and C–H simultaneously. The electronic effects of carboxylate ligands along with a base determined the selectivity. This reaction rendered readily the modification of anilines as well as the structural motifs of biologically active compounds. Notably, meta-substituted products were obtained when ClCF2CO2Et and BrCF2CO2Et were applied to this reaction.
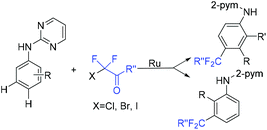 |
| Scheme 83 The ruthenium(II)-catalyzed para- and meta-selective C–H difluoroalkylation of aniline derivatives. | |
Use of Cu (Scheme 84a)167 and Ru (Scheme 84b)168 to catalyze difluoroalkylation of heteroarenes, including indoles, with BrCF2CO2R was also realized by several groups. However, a mixture of C-2 and C-3 difluoroalkylation was observed in these methods. Therefore, C-3 substituted indoles were usually applied to achieve selective C(2)–H difluoroalkylation. The Shi group realized copper-catalyzed C-2 difluoromethylation of indoles and pyrroles with BrCF2CO2Et. But a pyrimidyl group should be present as a directing group (Scheme 84c).169 In 2018, the nickel-catalyzed regioselective C(2)–H difluoroalkylation of C-3 unsubstituted indoles with BrCF2CO2R was successfully achieved by the Punji group (Scheme 85).170a Assistance of a directing group at the indoles was not necessary. A possible mechanism is described in Scheme 85. First, the Ni precursor might be reduced by Xantphos and base to active Ni(I) catalyst 148, which was followed by the reaction with BrCF2CO2R to afford intermediate 149. Then intermediate 149 underwent radical rebound to generate Ni(III) species 150, which was converted to intermediate 151via C(2)–H activation of indole.170b Finally, the product was formed via reductive elimination of 151, with the regeneration of the active catalyst. A parallel Ni(0)/Ni(II) path was also possible.
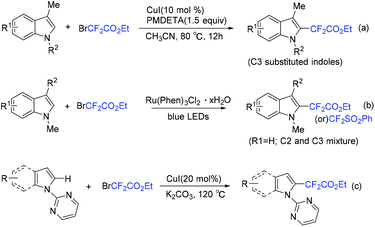 |
| Scheme 84 The difluoroalkylation of heteroarenes. | |
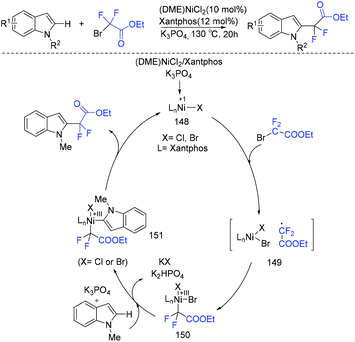 |
| Scheme 85 The nickel-catalyzed C(2)–H difluoroalkylation of C-3 unsubstituted indoles. | |
With the aid of DFT calculations, Noël and coworkers synthesized a series of substituted bithiophene derivatives as novel and inexpensive organic photocatalysts (Scheme 86).171 The activity of these photocatalysts was proved to be comparable with that of other commonly used organic or transition-metal photocatalysts in C–H functionalization of heteroarenes. Additionally, reaction proceeded in continuous-flow photomicroreactor affording the product with moderate to excellent yields within a few minutes. Both reductive and oxidative quenching pathways were proposed in the mechanism.
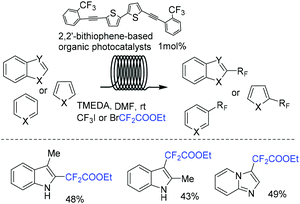 |
| Scheme 86 The visible-light-induced C–H functionalization of heteroarenes. | |
Imidazopyridines are important structures in numerous bioactive molecules and pharmaceuticals.172,173 Difluoroalkylation of imidazopyridines with BrCF2CO2Et and a Cu-based catalyst was reported by the Hajra group (Scheme 87a).174 In 2017, the Fu group175 demonstrated that an Ir photocatalyst was effective for this reaction (Scheme 87b). A variety of desired products with high functional group tolerance were obtained. CF2CO2Et radical derived from the reaction of BrCF2CO2Et and photoexcited state [fac-Ir(III)(ppy)3*] under light irradiation was proposed for this transformation. Besides imidazopyridines, benzo[d]isoxazoles were also proved to be suitable for Ir-photocatalyzed difluoroalkylation reaction.176 The first organophotoredox-catalyzed difluoromethylenephosphonation of imidazoheterocycles was reported by the Hajra group (Scheme 87c).177 Bis(pinacolato)diboron (B2pin2) played an important role for this reaction via activation of imidazoheterocycles. The Xu group demonstrated that visible-light-induced difluoroalkylation of imidazopyridines could also be realized in the absence of catalyst (Scheme 88).178 An energy transfer of bromodifluoroaryl ketones via visible light irradiation affording the excited intermediate bromodifluoroaryl ketones and a rapid C–Br bond homolysis to generate the difluoroacyl radical species I via excited intermediate bromodifluoroaryl ketones were proposed for this reaction.
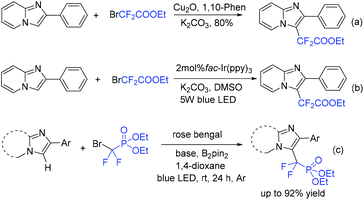 |
| Scheme 87 The difluoroalkylation of imidazopyridines. | |
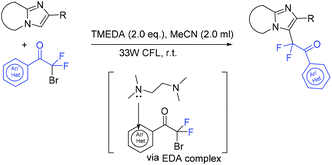 |
| Scheme 88 The visible-light-induced difluoroalkylation of imidazopyridines via EDA. | |
8-Aminoquinoline was also proved to be a good substrate for difluoroalkylation with difluoromethyl bromide reagent. For example, the Cu (Scheme 89a),179 Ni (Scheme 89b)180 and Ru (Scheme 89c)181 mediated difluoromethylation of 8-aminoquinoline has been achieved by the Wu, Wang and Zhao groups separately. In 2019, the visible-light-induced difluoroalkylation of 8-aminoquinolines at room temperature was realized by Sun and coworkers (Scheme 90).182 CF2CO2Et radical which was generated from oxidative quenching of excited Ir(ppy)3* by BrCF2CO2Et was proposed in this reaction.
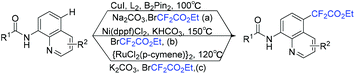 |
| Scheme 89 The Cu-, Ni-, Ru-catalyzed difluoroalkylation of 8-aminoquinolines. | |
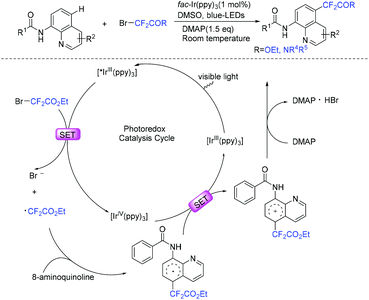 |
| Scheme 90 The visible-light-induced difluoroalkylation of 8-aminoquinolines. | |
Quinoxalin-2-one derivatives are useful molecules and appear in many bioactive molecules.183–186 In 2019, a copper-catalyzed direct C-3 difluoroacetylation of quinoxalinones with ethyl bromodifluoroacetate was demonstrated by Zhang and coworkers (Scheme 91).187 In 2019, the visible-light- and organoamine-cocatalyzed difluoroalkylation of quinoxalinones with functionalized difluoromethyl bromides was also realized by the Sun group (Scheme 92).188 Catalytic amount of DIPEA should be added for the success of this transformation. RCF2 radical generated from the reduction of RCF2Br was proposed for both of these reactions.
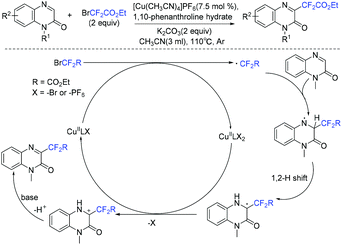 |
| Scheme 91 The copper-catalyzed direct C-3 difluoroacetylation of quinoxalinones. | |
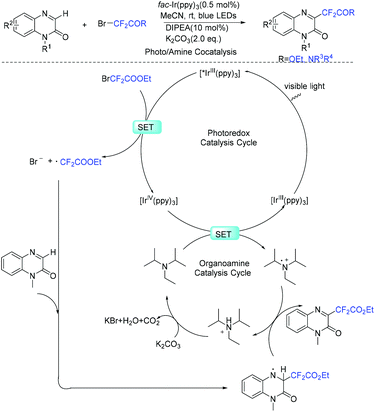 |
| Scheme 92 The visible-light- and organoamine-cocatalyzed difluoroalkylation of quinoxalinones. | |
In the same year, the decarboxylative difluoroarylmethylation of quinoxalin-2(1H)-ones with α,α-difluoroarylacetic acids was described by the Zhang group (Scheme 93).189 The CF2 group of α,α-difluoroarylacetic acids was crucial in this transformation. The decarboxylation process between α,α-difluorophenylacetic acid and (NH4)2S2O8 occurred to generate the Rf radical with the liberation of CO2. The Wan group demonstrated that difluoroarylacetic acids could also react well with other N-heteroarenes (Scheme 94).190 Using Ag catalyst, N-heteroarenes such as pyrazines, pyridine, quinoxaline, quinoline, and phenanthridine reacted smoothly in this reaction. The SET between difluoroacetic acid and Ag(II) which was generated from the oxidation of Ag(I) by (NH4)2S2O8 occurred to produce the corresponding nucleophilic aryldifluoromethyl radical.
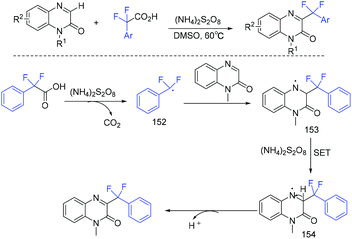 |
| Scheme 93 The decarboxylative difluoroarylmethylation of quinoxalin-2(1H)-ones. | |
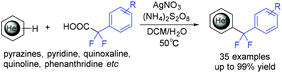 |
| Scheme 94 The decarboxylative difluoroarylmethylation of N-heteroarenes. | |
The first iron-catalyzed cross-coupling of Grignard reagents with fluoroalkyl halides was demonstrated by the Zhang group (Scheme 95).191 A diamine, in which a butylene was substituted at one carbon atom of the ethylene backbone in TMEDA, can improve the catalytic efficiency and suppress the side reactions of defluorination, because of the changes of iron complex from five-coordinate to more electron-deficient four-coordinate.
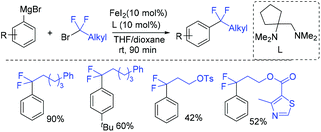 |
| Scheme 95 The cross-coupling of Grignard reagents with fluoroalkyl halides. | |
In 2019, the visible-light-induced difluoroalkylation of arenes with difluoroalkylation reagents (BrCF2CO2Et or BrCF2CONR1R2) was realized by the Liu group (Scheme 96).192 The in situ formation of cuprous photocatalyst from cuprous iodide, an imine ligand and a triaryl phosphine ligand was a key factor for this reaction. Difluoromethyl radicals which were generated via SET from the excited photocatalyst to difluoroalkylation reagents was suggested in the difluoroalkylation. In the same year, Hisaeda and coworkers found that these reactions can also be realized in the presence of cobalt catalyst (Scheme 97).193 Driven by controlled-potential electrolysis and visible-light irradiation in dimethyl sulfoxide, a wide range of electron-rich arenes and N-, O-, and S-containing heteroarenes reacted smoothly. Mechanistic studies revealed that a radical pathway mediated by homolytic cleavage of the cobalt(III) was involved in the mechanism. In the presence of triaryl phosphine as the catalyst, the visible-light-promoted difluoroalkylation reaction of arenes or heterocycles with difluoroalkyl iodide was presented by the Zhang group (Scheme 98).180 Mechanistic investigation implied that a radical-chain process that was initiated by an electron donor–acceptor complex formed from difluoroalkyl iodide and phosphine was involved in this reaction.
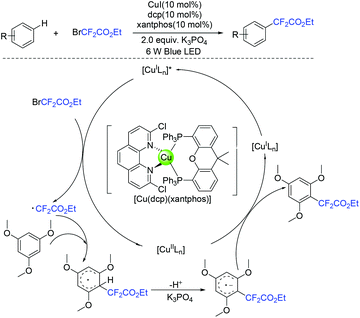 |
| Scheme 96 Visible-light-induced difluoroalkylation of arenes. | |
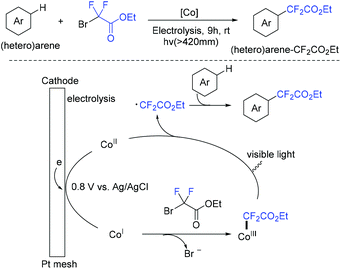 |
| Scheme 97 The Co-catalyzed difluoroalkylation of arenes. | |
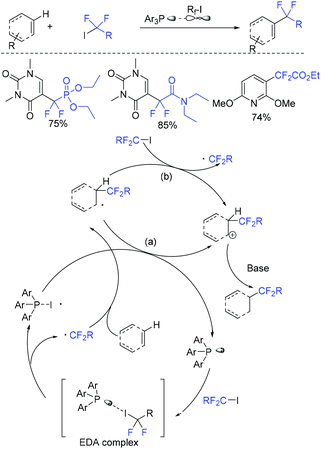 |
| Scheme 98 The triaryl phosphine-catalyzed difluoroalkylation of arenes. | |
An efficient method for the synthesis of 3,3-difluoro-2-oxindoles via copper/B2pin2-catalyzed C–H difluoroacetylation–cycloamidation of anilines with ethyl bromodifluoroacetate was realized by the Song group (Scheme 99).194 In this strategy, amino groups act as directing groups, providing ortho-difluoroacetylated products regioselectively. In the presence of a base, LCu(I)–Bpin species 155 was generated between LCuX(I) and B2pin2. Then BrCF2CO2R was reduced by species 155 to give a CF2CO2R radical. Subsequently CF2CO2R radical reacted with aniline to deliver intermediate 157 directed by amino groups. Finally, the 3,3-difluoro-2-oxindole derivatives were obtained after a SET pathway and intramolecular cycloamidation between ester and amino groups.
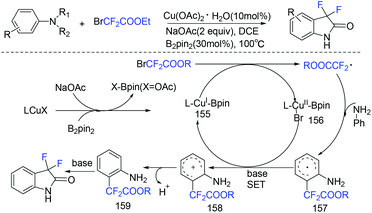 |
| Scheme 99 The copper/B2pin2-catalyzed C–H difluoroacetylation–cycloamidation of anilines. | |
In the same year, the Zhang group reported a visible-light-induced photoredox difluoromethylation–amidation of anilines with bromodifluoroacetate (Scheme 100).195 A broad range of difluorooxindoles was obtained via this process. However, aniline was not suitable for this system owing to the formation of a large amount of para-difluoroacetylated aniline. In the catalytic cycle, the reaction of bromodifluoroacetate and excited state of the photocatalyst *[Ir(ppy)3] was also proposed for this transformation to afford RCF2 radical. The Sun group found that 2-(1H-pyrrol-1-yl)anilines and 2-(1H-indol-1-yl)anilines were also good substrates to react with bromodifluoroacetate, providing various fluorinated pyrrolo[1,2-d]benzodiazepine derivatives.196
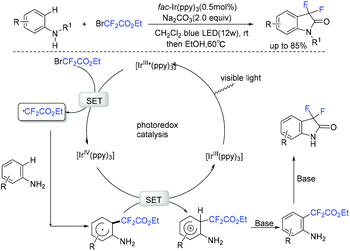 |
| Scheme 100 The visible-light-induced photoredox difluoromethylation–amidation of anilines. | |
Because of the steric disadvantage as well as entropy, the use of aryl-substituted anilines for this difluoromethylation transformation was challenging. The Li group realized the copper-catalyzed C–H [3 + 2] annulation of N-aryl- or alkyl-substituted anilines with bromodifluoroacetate (Scheme 101).197 The corresponding 3,3′-disubstituted oxindole products with moderate to good yields were assembled. In 2019, the Pd-catalyzed two-component C–H difluoroalkylation cyclization cascade reaction of 1-(2-aminophenyl)pyrrole derivatives was realized by the Liu group (Scheme 102).198 A variety of difluoroalkylated pyrrolobenzodiazepine derivatives were obtained in good yields. Two possible pathways which involved radical intermediates were proposed for this transformation.
 |
| Scheme 101 The copper-catalyzed annulation of N-substituted anilines. | |
 |
| Scheme 102 The copper-catalyzed annulation of N-substituted anilines. | |
5. Difluoroalkylation with alkyl C–H bond
Because of the high bond dissociation energy and lack of “active” HOMO or LUMO orbitals to interact with transition metal catalytic centers, it is more difficult to functionalize a C(sp3)–H bond.199–204 However, rapid progress has been achieved in recent years.133,205–210 Ketones are some of the most commonly used substrates for fluoroalkylation reactions.211,212 However, defluorination reactions often occur to form fluoroalkene compounds. In order to prevent defluorination reactions, tertiary ketones are usually necessary. In 2018, the group of Lu and Xiao described enantioselective radical difluoroalkylation reactions of β-ketoesters using an asymmetric photoredox and nickel catalysis cascade (Scheme 103).213 But the corresponding product was isolated with moderate yield and enantioselectivity (55% yield, 83
:
17 er) when BrCF2CO2R was used. In addition, perfluoroalkylation of β-ketoesters was also realized in this system. The reductive quenching of Ir(III)* with the Ni complex was proposed in the catalytic cycle. CF2CO2Et radical was generated from the reduction of XCF2CO2Et by Ir(II).
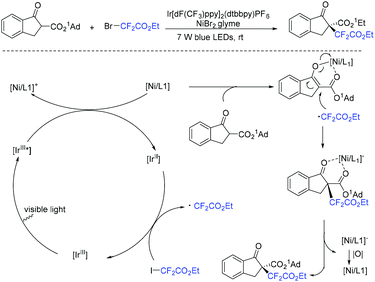 |
| Scheme 103 The difluoroalkylation reactions of β-ketoesters. | |
In 2018, the cobalt-catalyzed difluoroalkylation of tertiary aryl ketones for facile synthesis of quaternary alkyl difluorides was disclosed by the Wang group (Scheme 104).214 Preliminary mechanistic investigations indicated that a difluoroalkyl radical was involved in a Co(I)/Co(III) catalytic cycle. Zn metal could reduce the Co(II) to the catalytically active Co(I) species. Nickel was also found to be efficient for this type of reaction by the same group the following year (Scheme 105).215 But only tertiary aryl ketones were suitable for this difluoroalkylation reaction. A Ni(I)/Ni(III) catalytic cycle was proposed for this reaction. If a secondary ketone was employed, a tetrafluoroalkylated monofluoroalkene product was formed through defluorination.
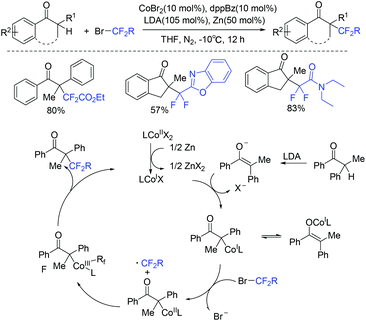 |
| Scheme 104 The cobalt-catalyzed difluoroalkylation of tertiary aryl ketones. | |
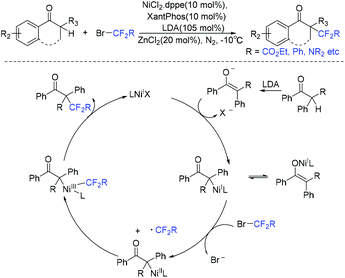 |
| Scheme 105 The nickel-catalyzed difluoroalkylation of tertiary aryl ketones. | |
In 2018, the methoxycarbonyldifluoromethylation of trimethylsilyl enol ethers and allyltrimethylsilanes with FSO2CF2CO2Me induced by visible light was developed by the Qing group (Scheme 106).216 Aromatic ketones and aliphatic ketones were all compatible with this transformation. The oxidative quenching of fac-IrIII(ppy)3* with FSO2CF2CO2Me took place to give CF2CO2Me radical and fac-IrIV(ppy)3 was proposed for this reaction. Additionally, fluoride anion could assist the desilylation of 161 to provide the target products.
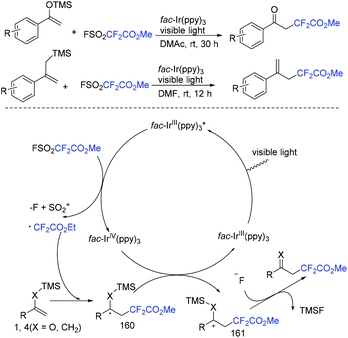 |
| Scheme 106 The methoxycarbonyldifluoromethylation of trimethylsilyl enol ethers. | |
6. Difluoroalkylation with isocyano
In 2013, the first visible-light-promoted isocyanide insertion was described by the Yu group (Scheme 107).217 Various 6-alkylated phenanthridine derivatives were obtained with good to excellent yields. Fluoroalkyl radical which was generated from the reduction of ethyl bromodifluoroacetate by Ir(ppy)3* added with isocyanide to provide radical 162. Subsequently, radical 162 underwent intramolecular hemolytic aromatic substitution to give radical intermediate 163. Then radical intermediate 163 was followed by oxidation and deprotonation reaction to release the desired product.
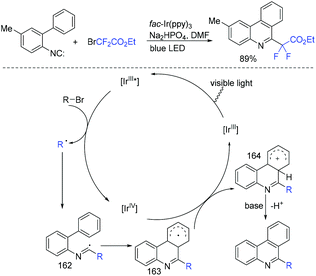 |
| Scheme 107 The visible-light-promoted isocyanide insertion. | |
The Song group realized the sodium sulfite-involved photocatalytic radical cascade cyclization of 2-isocyanoarylthioethers (Scheme 108).218 2-CF2-containing benzothiazoles can be efficiently prepared using this methodology. Different from previous reports, sodium sulfite should be added in the photocatalytic cycle to reduce IrIV into IrIII.
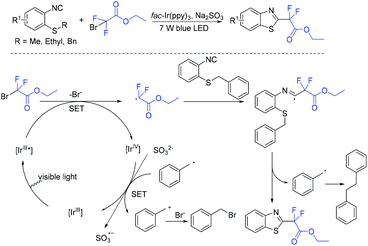 |
| Scheme 108 The photocatalytic radical cascade cyclization of 2-isocyanoarylthioethers. | |
A Cu/B2pin2 system-promoted cyclization reaction of vinyl isocyanides with bromodifluoroacetic derivatives for the synthesis of 1-difluoroalkylated isoquinolines was reported by the Shi group (Scheme 109).219 Preliminary mechanistic studies revealed that a tandem radical cyclization process was involved in this transformation. In addition, this transformation can also be induced by visible light.
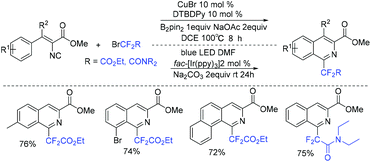 |
| Scheme 109 The Cu/B2pin2-promoted cascade cyclization of vinyl isocyanides. | |
7. Difluoroalkylation with amine
In 2018, the Song group found that BrCF2CO2Et played a dual role as a C1 synthon and a difluoroalkylating reagent in the reaction of BrCF2CO2Et with primary amines (Scheme 110).220 Difluorocarbene which was generated from the reaction of BrCF2CO2Et with Na2CO3via decarboxylation and debromination processes was involved in the catalytic cycle. Based on experimental observations and DFT calculations, they found that base played an active role in the formation of the key intermediate isocyanides generated from primary amines and difluorocarbene. The same group found that ethyl bromodifluoroacetate could also serve as a C1 synthon to access 2,4-disubstituted 1,3,5-triazines (Scheme 111).221 Both symmetric and unsymmetric 2,4-disubstituted 1,3,5-triazines could be obtained with only small amounts of toxic inorganic wastes generated. Based on experimental results, it was speculated that difluorocarbene (:CF2) was actually generated from BrCF2CO2Et with the assistance of base. Then difluorocarbene (:CF2) reacted with amidine to afford intermediate 165, which was defluorinated to imine intermediate 166 under basic conditions. Subsequently, intermediate 167 was generated from the reaction of intermediate 166 with another amidine. Finally the corresponding products were formed through intramolecular nucleophilic addition of 167 and elimination of one molecule of ammonia.
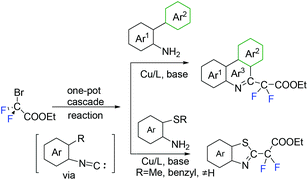 |
| Scheme 110 The reaction of BrCF2COOEt with primary amines. | |
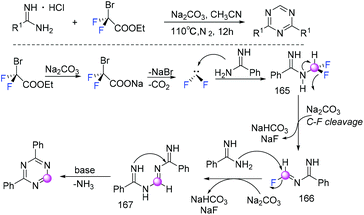 |
| Scheme 111 The synthesis of 2,4-disubstituted 1,3,5-triazines. | |
8. Difluoroalkylation with C
N
In 2016, the CuI (Scheme 112a)222 and Pd2(dba)3 (Scheme 112b)223 catalyzed difluoroalkylations of hydrazones were realized by the Monteiro group. The Ir (Scheme 112c)224 and Au (Scheme 112d)225 catalyzed difluoroalkylation of hydrazones induced by visible light was also presented by the Zhu and Hashmi groups separately. The Cu(II)/B2pin2-catalyzed difluoroalkylation of hydrazones with difluoroalkyl bromides using diboron as reductant was reported the Song group (Scheme 113).226 Both aromatic and aliphatic hydrazones were suitable for this reaction. A difluoroalkyl radical via a SET pathway was suggested in the reaction mechanism. Additionally, the catalytic diboron reagent was a key factor in this transformation.
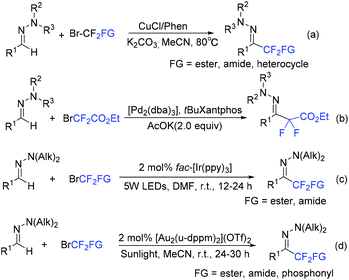 |
| Scheme 112 The difluoroalkylation of hydrazones catalyzed by various metals. | |
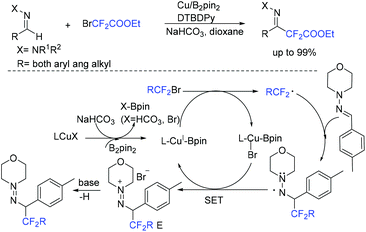 |
| Scheme 113 The Cu/B2pin2-catalyzed difluoroalkylation of hydrazones. | |
The direct three-component difluoroalkylation reaction between aldehydes, hydrazines, and halodifluoromethylated reagents catalyzed by visible-light organic photoredox was realized by Wang and coworkers (Scheme 114).227 A reductive quenching of photoexcited PC* species with the hydrazine in situ generated from aldehyde and hydrazine to form the strong reductant PC− and radical intermediate 168 was proposed for this reaction.
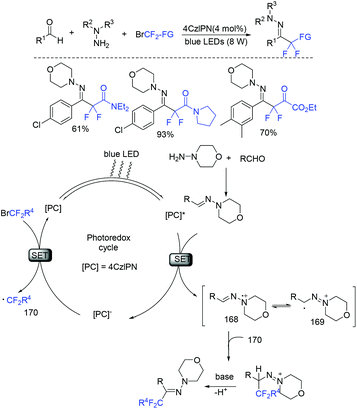 |
| Scheme 114 The three-component difluoroalkylation reaction between aldehydes, hydrazines and halodifluoromethylated reagents. | |
In 2019, a visible-light-induced difluoroalkylation of N,N-cyclicazomethine imine with BrCF2R was successfully realized by the Yang group (Scheme 115).228 Mechanistic investigations showed that a reductive radical–radical coupling pathway and oxidation of ascorbic acid with Ir(IV) to form ascorbyl radical were involved in the mechanism.
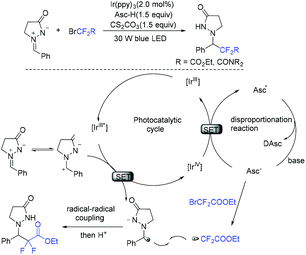 |
| Scheme 115 The difluoroalkylation of N,N-cyclicazomethine imine. | |
The first base-catalyzed enantioselective phosphonyldifluoromethylation was described by the Vilotijevic group (Scheme 116).229 By the use of diethyl(difluoro(trimethylsilyl)methyl)phosphonate reagent as a latent pronucleophile, the enantiomers of the product can be readily accessed. A kinetic resolution was involved in the reaction process. But the yield of the reaction was not very high.
 |
| Scheme 116 Enantioselective substitution of allylic fluorides. | |
9. Difluoroalkylation with C
O
In the past decade, tremendous efforts have been made in (phenylsulfonyl/arylthio)difluoromethylation of aldehydes.230–234 Among these reactions, the nucleophilic addition of PhSO2CF2− or PhSCF2− anion to carbonyl compounds (such as aldehydes) was the most popular method. In 2019, the Hu group found that TMSCF2Br was good partner for nucleophilic (phenylsulfonyl/arylthio)difluoromethylation of aldehydes and PhSO2Na(ArSNa) (Scheme 117).235 The preparation of PhSO2CF2X or PhSCF2X (X = H, Br, TMS) reagents was avoided in this three-component reaction. The in situ generation of difluorocarbene via the reaction of TMSCF2Br with a Lewis base activator (LB = PhSO2–, PhS–, or 174) was proposed in the reaction mechanism. Then the reaction of difluorocarbene with PhSO2Na(ArSNa) occurred to form the corresponding PhSO2CF2(PhSCF2) anions. Finally, the desired difluoromethylated products were obtained via nucleophilic addition of PhSO2CF2(PhSCF2) anions to aldehydes.
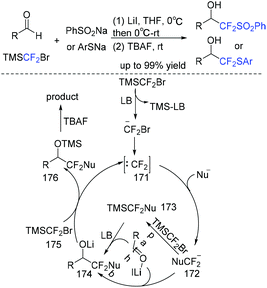 |
| Scheme 117 The (phenylsulfonyl)difluoromethylation of aldehydes. | |
10. Conclusions
The difluoroalklyation reaction has made much progress in the last few years. In this review, we summarized the advances of difluoroalklyation reactions in the last three years. The most commonly used difluoroalkylation reagent was RCF2X. Generally speaking, RCF2 radical which was usually generated from the reduction of RCF2X was the active intermediate in most cases. When alkenes or alkynes were used as substrates, reactions were usually initiated by radical addition to the carbon–carbon unsaturated bonds; then the final products can be isolated in different ways. Visible-light-induced and metal-catalyzed difluoroaklyations were all realized. Some electron-rich bases could also be used to induce the formation of RCF2 radical for these reactions via EDA complex. At present, the most efficient photocatalyst was metal [Ir]. In the absence of visible light, copper was proved to be one of the most effective catalysts in metal catalyzed difluoroalkylation. In consideration of green chemistry and cost efficiency, a cheap photocatalyst or even catalyst-free difluoroalkylation reaction is more desirable. Asymmetric difluoroalkylation is also worth further study. Additionally, multicomponent reaction for the introduction of RCF2 group also needs to be further explored. We anticipate that this review will open new avenues for the development of difluoroalkylation reactions.
Conflicts of interest
There are no conflicts to declare.
Acknowledgements
This work was supported by the National Natural Science Foundation of China (no. 21772107) and Shandong Province Key Research and Development Plan (no. 2019GSF108017). We also thank Cai-Zhen Ding, Yan-Li Wang and Hong-Di Yang for their useful help.
Notes and references
- A. Vulpetti and C. Dalvit, Fluorine local environment: from screening to drug design, Drug Discovery Today, 2012, 17, 890–897 CrossRef CAS PubMed.
- A. D. Dilman and V. V. Levin, Difluorocarbene as a Building Block for Consecutive Bond-Forming Reactions, Acc. Chem. Res., 2018, 51, 1272–1280 CrossRef CAS PubMed.
- T. Lasing, A. Phumee, P. Siriyasatien, K. Chitchak, P. Vanalabhpatana, K. K. Mak, C. Hee Ng, T. Vilaivan and T. Khotavivattana, Synthesis and antileishmanial activity of fluorinated rhodacyanine analogues: The ‘fluorine-walk’ analysis, Bioorg. Med. Chem., 2020, 28, 115187 CrossRef CAS PubMed.
- Z. Feng, Y. L. Xiao and X. G. Zhang, Transition-Metal (Cu, Pd, Ni)-Catalyzed Difluoroalkylation via Cross-Coupling with Difluoroalkyl Halides, Acc. Chem. Res., 2018, 51, 2264–2278 CrossRef CAS PubMed.
- W. Wang, Q. Yu, Q. Zhang, J. Li, F. Hui, J. Yang and J. Lü, Recent Progress on Difluoromethylation Methods, Chin. J. Org. Chem., 2018, 38, 1569–1585 CrossRef CAS.
- X. F. Tao, R. Sheng, K. Bao, Y. X. Wang and Y. X. Jin, Progress of Difluoromethyl Heteroaryl Sulfones as Difluoroalkylation Reagents, Chin. J. Org. Chem., 2019, 39, 2726–2734 CrossRef.
- A. Lemos, C. Lemaire and A. Luxen, Progress in Difluoroalkylation of Organic Substrates by Visible Light Photoredox Catalysis, Adv. Synth. Catal., 2019, 361, 1500–1537 CrossRef CAS PubMed.
- X. S. Hu, J. S. Yu and J. Zhou, Catalytic selective mono- and difluoroalkylation using fluorinated silyl enol ethers, Chem. Commun., 2019, 55, 13638–13648 RSC.
- J. Mizukado, Y. Matsukawa, H.-D. Quan, M. Tamura and A. Sekiya, Reactions of aliphatic fluoro-alcohols with CHClF2 at atmospheric pressure, J. Fluorine Chem., 2006, 127, 400–404 CrossRef CAS.
-
(a) R. M. Flynn and D. J. Burton, Synthetic and mechanistic aspects of halo-F-methylphosphonates, J. Fluorine Chem., 2011, 132, 815–828 CrossRef CAS;
(b) Z. Feng, F. Chen and X. Zhang, Copper catalyzed cross-coupling of iodobenzoates with bromozinc-difluorophosphonate, Org. Lett., 2012, 14, 1938–1941 CrossRef CAS PubMed.
- O. V. Fedorov, M. I. Struchkova and A. D. Dilman, Silicon Reagent with Functionalized Tetrafluoroethylene Fragments: Preparation and Coupling with Aldehydes, J. Org. Chem., 2016, 81, 9455–9460 CrossRef CAS.
- X. Song, S. Tian, Z. Zhao, D. Zhu and M. Wang, Controlled Ring-Opening of Siloxydifluorocyclopropanes for Carbocyclization: Synthesis of Difluorocyclopentenones, Org. Lett., 2016, 18, 3414–3417 CrossRef CAS.
- J. Mizukado, Y. Matsukawa, H.-D. Quan, M. Tamura and A. Sekiya, Insertion reactions of difluorocarbene generated by pyrolysis of hexafluoropropene oxide to OH bond, J. Fluorine Chem., 2005, 126, 365–369 CrossRef CAS.
- K. Levchenko, O. P. Datsenko, O. Serhiichuk, A. Tolmachev, V. O. Iaroshenko and P. K. Mykhailiuk, Copper-Catalyzed O-Difluoromethylation of Functionalized Aliphatic Alcohols: Access to Complex Organic Molecules with an OCF2H Group, J. Org. Chem., 2016, 81, 5803–5813 CrossRef CAS PubMed.
- S. Krishnamoorthy, J. Kothandaraman, J. Saldana and G. K. S. Prakash, Direct Difluoromethylenation of Carbonyl Compounds by Using TMSCF3: The Right Conditions, Eur. J. Org. Chem., 2016, 4965–4969 CrossRef.
- Y. Zhao, W. Huang, J. Zheng and J. Hu, Efficient and Direct Nucleophilic Difluoromethylation of Carbonyl Compounds and Imines with Me3SiCF2H at Ambient or Low Temperature, Org. Lett., 2011, 13, 5342–5345 CrossRef CAS PubMed.
- Y. Arai, R. Tomita, G. Ando, T. Koike and M. Akita, Oxydifluoromethylation of Alkenes by Photoredox Catalysis: Simple Synthesis of CF2H-Containing Alcohols, Chem. – Eur. J., 2016, 22, 1262–1265 CrossRef CAS PubMed.
-
(a) K. Aikawa, H. Serizawa, K. Ishii and K. Mikami, Palladium-Catalyzed Negishi Cross-Coupling Reaction of Aryl Halides with (Difluoromethyl)zinc Reagent, Org. Lett., 2016, 18, 3690–3693 CrossRef CAS PubMed;
(b) X. T. Huang, Z. Y. Long and Q. Y. Chen, Fluoroalkylation of aromatic compounds with per(poly)fluoroalkyl chlorides initiated by sodium dithionite in DMSO, J. Fluorine Chem., 2001, 111, 107–113 CrossRef CAS.
-
(a) W. Liu, W. Yan, M. Paeth, S. Zacate and X. Zeng, Late-Stage Difluoromethylation of Aliphatic Carboxylic Acids with Copper Catalysis, Synlett, 2020, 745–749 CrossRef;
(b) C. Xu, W.-H. Guo, X. He, Y.-L. Guo, X.-Y. Zhang and X. Zhang, Difluoromethylation of (hetero)aryl chlorides with chlorodifluoromethane catalyzed by nickel, Nat. Commun., 2018, 9, 1170 CrossRef PubMed.
- X. Xiao, Z.-T. Zheng, T. Li, J.-L. Zheng, T. Tao, L.-M. Chen, J.-Y. Gu, X. Yao, J.-H. Lin and J.-C. Xiao, Recent Advances in Difluoromethylthiolation, Synthesis, 2020, 197–207 CAS.
- T. Koike and M. Akita, Recent progress in photochemical radical di- and mono-fluoromethylation, Org. Biomol. Chem., 2019, 17, 5413–5419 RSC.
-
(a) N. Levi, D. Amir, E. Gershonov and Y. Zafrani, Recent Progress on the Synthesis of CF2H-Containing Derivatives, Synthesis, 2019, 4549–4567 CAS;
(b) Z. Feng, Q.-Q. Min, X.-P. Fu, L. An and X. Zhang, Chlorodifluoromethane-triggered formation of difluoromethylated arenes catalysed by palladium, Nat. Chem., 2017, 9, 918–923 CrossRef CAS PubMed.
- T. Y. Shang, L. H. Lu, Z. Cao, Y. Liu, W. M. He and B. Yu, Recent advances of 1,2,3,5-tetrakis(carbazol-9-yl)-4,6-dicyanobenzene (4CzIPN) in photocatalytic transformations, Chem. Commun., 2019, 55, 5408–5419 RSC.
- Y. Chen, L.-Q. Lu, D.-G. Yu, C.-J. Zhu and W.-J. Xiao, Visible light-driven organic photochemical synthesis in China, Sci. China: Chem., 2018, 62, 24–57 CrossRef.
- G.-H. Li, Q.-Q. Han, Y.-Y. Sun, D.-M. Chen, Z.-L. Wang, X.-M. Xu and X.-Y. Yu, Visible-light induced cascade radical cyclization of sulfinic acids and o-(allyloxy)arylaldehydes towards functionalized chroman-4-ones, Chin. Chem. Lett., 2020 DOI:10.1016/j.cclet.2020.03.007.
- D. Q. Dong, L. X. Li, G. H. Li, Q. Deng, Z. L. Wang and S. Long, Visible-light-induced deoxygenative C2-sulfonylation of quinoline N-oxides with sulfinic acids for the synthesis of 2-sulfonylquinoline via radical reactions, Chin. J. Catal., 2019, 40, 1494–1498 CrossRef CAS.
- J. Hu, J. Wang, T. H. Nguyen and N. Zheng, The chemistry of amine radical cations produced by visible light photoredox catalysis, Beilstein J. Org. Chem., 2013, 9, 1977–2001 CrossRef PubMed.
- A. Banerjee, Z. Lei and M. Y. Ngai, Acyl Radical Chemistry via Visible-Light Photoredox Catalysis, Synthesis, 2019, 303–333 CAS.
- S. Yang, L. Wang, L. Wang and H. Li, Visible-Light Photoredox-Catalyzed Regioselective Sulfonylation of Alkenes Assisted by Oximes via [1,5]-H Migration, J. Org. Chem., 2020, 85, 564–573 CrossRef CAS PubMed.
- Q. Liu and L.-Z. Wu, Recent advances in visible-light-driven organic reactions, Natl. Sci. Rev., 2017, 4, 359–380 CrossRef CAS.
- X.-X. Meng, Q.-Q. Kang, J.-Y. Zhang, Q. Li, W.-T. Wei and W.-M. He, Visible-light-initiated regioselective sulfonylation/cyclization of 1,6-enynes under photocatalyst- and additive-free conditions, Green Chem., 2020, 22, 1388–1392 RSC.
- L.-Y. Xie, T.-G. Fang, J.-X. Tan, B. Zhang, Z. Cao, L.-H. Yang and W.-M. He, Visible-light-induced deoxygenative C2-sulfonylation of quinoline N-oxides with sulfinic acids, Green Chem., 2019, 21, 3858–3863 RSC.
- S. Peng, Y. Lin and W. He, Visible Light-Induced Aldehyde Reductive Minisci Reaction towards N-Heterocycles, Chin. J. Org. Chem., 2020, 40, 541–542 CrossRef.
- D. A. Nicewicz and D. W. C. MacMillan, Merging Photoredox Catalysis with Organocatalysis: The Direct Asymmetric Alkylation of Aldehydes, Science, 2008, 322, 77–80 CrossRef CAS PubMed.
- M. A. Ischay, M. E. Anzovino, J. Du and T. P. Yoon, Efficient Visible Light Photocatalysis of [2+2] Enone Cycloadditions, J. Am. Chem. Soc., 2008, 130, 12886–12887 CrossRef CAS PubMed.
- Z. Gu, H. Zhang, P. Xu, Y. Cheng and C. Zhu, Visible-Light-Induced Radical Tandem Aryldifluoroacetylation of Cinnamamides: Access to Difluoroacetylated Quinolone-2-ones And 1-Azaspiro[4.5]decanes, Adv. Synth. Catal., 2015, 357, 3057–3063 CrossRef CAS.
- W. Fu, M. Zhu, G. Zou, C. Xu and Z. Wang, Visible-Light-Mediated Radical Aryldifluoroacetylation ofN-Arylacrylamides to give Difluoroacetylated Oxindoles, Asian J. Org. Chem., 2014, 3, 1273–1276 CrossRef CAS.
- X. Sun and S. Yu, Visible-light-promoted iminyl radical formation from vinyl azides: synthesis of 6-(fluoro)alkylated phenanthridines, Chem. Commun., 2016, 52, 10898–10901 RSC.
- S. Tang, Y. L. Deng, J. Li, W. X. Wang, G. L. Ding, M. W. Wang, Z. P. Xiao, Y. C. Wang and R. L. Sheng, Synthesis of Perfluorinated Isoquinolinediones through Visible-Light-Induced Cyclization of Alkenes, J. Org. Chem., 2015, 80, 12599–12605 CrossRef CAS PubMed.
- W. Fu, M. Zhu, G. Zou, C. Xu, Z. Wang and B. Ji, Visible-light-mediated radical aryldifluoroacetylation of alkynes with ethyl bromodifluoroacetate for the synthesis of 3-difluoroacetylated coumarins, J. Org. Chem., 2015, 80, 4766–4770 CrossRef CAS PubMed.
- Z. Zhang, X. J. Tang and W. R. Dolbier Jr., Photoredox-Catalyzed Intramolecular Difluoromethylation of N-Benzylacrylamides Coupled with a Dearomatizing Spirocyclization: Access to CF2H-Containing 2-Azaspiro[4.5]deca-6,9-diene-3,8-diones, Org. Lett., 2016, 18, 1048–1051 CrossRef CAS PubMed.
- H. G. Huang, M. L. Yu, X. L. Su, P. Guo, J. Zhao, J. B. Zhou and Y. Li, Sustainable Radical Cascades to Synthesize Difluoroalkylated Pyrrolo 1,2-a indoles, J. Org. Chem., 2018, 83, 2425–2437 CrossRef CAS PubMed.
- Q. Deng, L. Tan, Y. Xu, P. Liu and P. Sun, Synthesis of 6-Fluoroalkyl 6 H-Benzo[c]chromenes via Visible-Light-Promoted Radical Addition/Cyclization of Biaryl Vinyl Ethers, J. Org. Chem., 2018, 83, 6151–6161 CrossRef CAS PubMed.
- T. Zhang, B. Chen, W. Wang, Q. Zhang, P. Wang, W. Wan, H. Deng, J. Hao and H. Jiang, Copper-Promoted Aryldifluoromethylenation of N-Arylacrylamides to 3-Benzo-diazolyldifluoromethylene-Substituted 2-Oxindoles, Asian J. Org. Chem., 2019, 8, 671–674 CrossRef CAS.
- J. L. Hu, T. J. Pu, Z. W. Xu, W. Y. Xu and Y. S. Feng, Cadmium Sulfide Quantum-Dot-Photocatalyzed Cascade Cyclization of Functionalized Difluoromethyl Chlorides with Unactivated Olefins, Adv. Synth. Catal., 2019, 361, 708–713 CrossRef CAS.
- H. Xiang, Q. L. Zhao, P. J. Xia, J. A. Xiao, Z. P. Ye, X. Xie, H. Sheng, X. Q. Chen and H. Yang, Visible-Light-Induced External Radical-Triggered Annulation To Access CF2-Containing Benzoxepine Derivatives, Org. Lett., 2018, 20, 1363–1366 CrossRef CAS PubMed.
- X. Liu, Z. Wu, Z. Zhang, P. Liu and P. Sun, Synthesis of trifluoroalkyl or difluoroalkyl phenanthridine derivatives via cascade reaction using an intramolecular cyano group as a radical acceptor under
photoredox catalysis, Org. Biomol. Chem., 2018, 16, 414–423 RSC.
- F. Ding, Y. Fang, Y. Jiang, K. Lin and L. Shi, Tandem Radical Cyclization for the Construction of Difluoro-Containing Oxindoles and Quinoline-2,4-diones, Chem.– Asian J., 2018, 13, 636–640 CrossRef CAS PubMed.
- T. C. McKee, H. R. Bokesch, J. L. McCormick, M. A. Rashid, D. Spielvogel, K. R. Gustafson, M. M. Alavanja, J. H. Cardellina and M. R. Boyd, Isolation and Characterization of New Anti-HIV and Cytotoxic Leads from Plants, Marine, and Microbial Organisms, J. Nat. Prod., 1997, 60, 431–438 CrossRef CAS PubMed.
- B. Lee, H. D. Basavarajappa, R. S. Sulaiman, X. Fei, S.-Y. Seo and T. W. Corson, The first synthesis of the antiangiogenic homoisoflavanone, cremastranone, Org. Biomol. Chem., 2014, 12, 7673–7677 RSC.
- L. Xiong, H. Hu, C.-W. Wei and B. Yu, Radical Reactions for the Synthesis of 3-Substituted Chroman-4-ones, Eur. J. Org. Chem., 2020, 1588–1597 CrossRef CAS.
- N. N. Zhou, M. X. Wu, M. Zhang and X. Q. Zhou, Visible-Light-Induced Difluoroacetylation of O-(Allyloxy)Aryl-Aldehydes: Access to Difluoroacetylated Chroman-4-ones, Asian J. Org. Chem., 2019, 8, 828–831 CrossRef CAS.
- X. Huang, Y. G. Zhang, C. S. Zhang, L. Zhang, Y. Xu, L. C. Kong, Z. X. Wang and B. Peng, The ortho-Difluoroalkylation of Aryliodanes with Enol Silyl Ethers: Rearrangement Enabled by a Fluorine Effect, Angew. Chem., Int. Ed., 2019, 58, 5956–5961 CrossRef CAS PubMed.
- C. Yu, N. Iqbal, S. Park and E. J. Cho, Selective difluoroalkylation of alkenes by using visible light photoredox catalysis, Chem. Commun., 2014, 50, 12884–12887 RSC.
- X. Li, S. Li, S. Sun, F. Yang, W. Zhu, Y. Zhu, Y. Wu and Y. Wu, Direct Decarboxylative Alkynylation of α,α-Difluoroarylacetic Acids under Transition Metal-Free Conditions, Adv. Synth. Catal., 2016, 358, 1699–1704 CrossRef CAS.
- X.-J. Tang and W. R. Dolbier Jr., Efficient Cu-catalyzed Atom Transfer Radical Addition Reactions of Fluoroalkylsulfonyl Chlorides with Electron-deficient Alkenes Induced by Visible Light, Angew. Chem., Int. Ed., 2015, 54, 4246–4249 CrossRef CAS PubMed.
- M. Zhang, W. Li, Y. Duan, P. Xu, S. Zhang and C. Zhu, Cascade Photoredox/Iodide Catalysis: Access to Difluoro-γ-lactams via Aminodifluoroalkylation of Alkenes, Org. Lett., 2016, 18, 3266–3269 CrossRef CAS PubMed.
- Y. L. Zhou, Z. M. Xiong, J. Y. Qiu, L. C. Kong and G. G. Zhu, Visible light photocatalytic acyldifluoroalkylation of unactivated alkenes for the direct synthesis of gem- difluorinated ketones, Org. Chem. Front., 2019, 6, 1022–1026 RSC.
- Z. J. Shen, S. C. Wang, W. J. Hao, S. Z. Yang, S. J. Tu and B. Jiang, Switching between Copper-Catalysis and Photocatalysis for Tunable Halofluoroalkylation and Hydrofluoroalkylation of 1,6-Enynes toward 1-Indenones, Adv. Synth. Catal., 2019, 361, 3837–3851 CrossRef CAS.
- M. Li, C. T. Wang, Y. F. Qiu, X. Y. Zhu, Y. P. Han, Y. Xia, X. S. Li and Y. M. Liang, Base promoted direct difunctionalization/cascade cyclization of 1,6-enynes, Chem. Commun., 2018, 54, 5334–5337 RSC.
- P. B. Zhang, C. Wang, M. C. Cui, M. S. Du, W. W. Li, Z. X. Jia and Q. Zhao, Synthesis of Difluoroalkylated Benzofuran, Benzothiophene, and Indole Derivatives via Palladium-Catalyzed Cascade Difluoroalkylation and Arylation of 1,6-Enynes, Org. Lett., 2020, 22, 1149–1154 CrossRef CAS PubMed.
- J. Wang, M. Sánchez-Roselló, J. L. Aceña, C. del Pozo, A. E. Sorochinsky, S. Fustero, V. A. Soloshonok and H. Liu, Fluorine in Pharmaceutical Industry: Fluorine-Containing Drugs Introduced to the Market in the Last Decade (2001–2011), Chem. Rev., 2014, 114, 2432–2506 CrossRef CAS PubMed.
- M. O. Anderson, J. Zhang, Y. Liu, C. Yao, P.-W. Phuan and A. S. Verkman, Nanomolar Potency and Metabolically Stable Inhibitors of Kidney Urea Transporter UT-B, J. Med. Chem., 2012, 55, 5942–5950 CrossRef CAS PubMed.
- M. Médebielle, Electrochemical addition of chlorodifluoroacetyl aromatic compounds to electron-rich olefinic substrates. A convenient synthesis of gem-difluoro heterocyclic compounds, Tetrahedron Lett., 1995, 36, 2071–2074 CrossRef.
- P. Hapiot and M. Médebielle, Electrochemically induced free-radical tandem cyclisation of chlorodifluoromethylated ketones: Application to the synthesis of gem-difluorinated heterocycles, J. Fluorine Chem., 2001, 107, 285–300 CrossRef CAS.
- C. Adouama, R. Keyrouz, G. Pilet, C. Monnereau, D. Gueyrard, T. Noël and M. Médebielle, Access to cyclic gem-difluoroacyl scaffolds via electrochemical and visible light photocatalytic radical tandem cyclization of heteroaryl chlorodifluoromethyl ketones, Chem. Commun., 2017, 53, 5653–5656 RSC.
- C. Qu, P. Xu, W. Ma, Y. Cheng and C. Zhu, A novel visible light mediated radical cyclization of enol lactones: a concise method for fluorinated polycyclic lactone scaffolds, Chem. Commun., 2015, 51, 13508–13510 RSC.
- C. Qu, Z. Wu, W. Li, H. Du and C. Zhu, Electron Catalytic Photochemical Cascade Carbodifluoroalkylation/Radical Cyclization of Methylene-2-oxazolines, Adv. Synth. Catal., 2017, 359, 1672–1677 CrossRef CAS.
- P. Peng, G. Z. Huang, Y. X. Sun, X. Wang, J. J. Wu and F. H. Wu, Copper-mediated cascade radical cyclization of olefins with naphthalenyl iododifluoromethyl ketones, Org. Biomol. Chem., 2019, 17, 6426–6431 RSC.
- C. Liu, Y. J. Yang, J. Y. Dong, M. D. Zhou, L. Li and H. Wang, Copper/B(2)pin(2)-Catalyzed Difluoroalkylation of Methylenecyclopropanes with Bromodifluorinated Acetates and Acetamides: One-Pot Synthesis of CF2-Containing Dihydronaphthalene Derivatives, J. Org. Chem., 2019, 84, 9937–9945 CrossRef CAS PubMed.
- X. Wang, J. Liu, Z. Yu, M. Guo, X. Tang and G. Wang, Desulfonylation-Initiated Distal Alkenyl Migration in Copper-Catalyzed Alkenylation of Unactivated Alkenes, Org. Lett., 2018, 20, 6516–6519 CrossRef CAS PubMed.
- X. J. Wei and T. Noel, Visible-Light Photocatalytic Difluoroalkylation-Induced 1, 2-Heteroarene Migration of Allylic Alcohols in Batch and Flow, J. Org. Chem., 2018, 83, 11377–11384 CrossRef CAS PubMed.
- L. Hu, Q. Deng, Y. Zhou, X. Zhang and Y. Xiong, Cu2O-catalyzed phosphonyldifluoromethylation of allylic alcohols through a radical 1,2-aryl migration, Tetrahedron, 2020, 76, 130949 CrossRef CAS.
- D. M. Whalley, H. A. Duong and M. F. Greaney, Alkene Carboarylation through Catalyst-Free, Visible Light-Mediated Smiles Rearrangement, Chem. – Eur. J., 2019, 25, 1927–1930 CrossRef CAS PubMed.
- J. Liu, W. P. Li, J. Xie and C. J. Zhu, Photoredox 1,2-dicarbofunctionalization of unactivated alkenes via tandem radical difluoroalkylation and alkynyl migration, Org. Chem. Front., 2018, 5, 797–800 RSC.
- M. Li, X. Y. Zhu, Y. F. Qiu, Y. P. Han, Y. Xia, C. T. Wang, X. S. Li, W. X. Wei and Y. M. Liang, Metal-Free Promoted CF2/CF3-Difunctionalization of Unactivated Alkenes, Adv. Synth. Catal., 2019, 361, 2945–2950 CrossRef CAS.
- W. W. Jin, M. C. Wu, Z. M. Xiong and G. G. Zhu, Visible-light induced three-component alkynyl-difluoroalkylation of unactivated alkenes, Chem. Commun., 2018, 54, 7924–7927 RSC.
- J. Ye and S. Ma, Conquering three-carbon axial chirality of allenes, Org. Chem. Front., 2014, 1, 1210–1224 RSC.
-
(a) J. Ye and S. Ma, Palladium-Catalyzed Cyclization Reactions of Allenes in the Presence of Unsaturated Carbon–Carbon Bonds, Acc. Chem. Res., 2014, 47, 989–1000 CrossRef CAS PubMed;
(b) J.-W. Gu, Q.-Q. Min, L.-C. Yu and X. Zhang, Tandem Difluoroalkylation-Arylation of Enamides Catalyzed by Nickel, Angew. Chem., Int. Ed., 2016, 55, 12270–12274 CrossRef CAS PubMed.
- K.-F. Zhang, K.-J. Bian, C. Li, J. Sheng, Y. Li and X.-S. Wang, Nickel-Catalyzed Carbofluoroalkylation of 1,3-Enynes to Access Structurally Diverse Fluoroalkylated Allenes, Angew. Chem., Int. Ed., 2019, 58, 5069–5074 CrossRef CAS PubMed.
- X. L. Lv, C. Wang, Q. L. Wang and W. Shu, Rapid Synthesis of gamma-Arylated Carbonyls Enabled by the Merge of Copper- and Photocatalytic Radical Relay Alkylarylation of Alkenes, Org. Lett., 2019, 21, 56–59 CrossRef CAS PubMed.
- J. Cao, G. Wang, L. Gao, H. Chen, X. Liu, X. Cheng and S. Li, Perfluoroalkylative pyridylation of alkenes via 4-cyanopyridine-boryl radicals, Chem. Sci., 2019, 10, 2767–2772 RSC.
- X. G. Wang, Y. Li, H. C. Liu, B. S. Zhang, X. Y. Gou, Q. Wang, J. W. Ma and Y. M. Liang, Three-Component Ruthenium-Catalyzed Direct Meta-Selective C-H Activation of Arenes: A New Approach to the Alkylarylation of Alkenes, J. Am. Chem. Soc., 2019, 141, 13914–13922 CrossRef CAS PubMed.
- X. Yong, Y. F. Han, Y. Li, R. J. Song and J. H. Li, Alkylarylation of styrenes via direct C(sp(3))-Br/C(sp(2))-H functionalization mediated by photoredox and copper cooperative catalysis, Chem. Commun., 2018, 54, 12816–12819 RSC.
- D. Zheng and A. Studer, Asymmetric Synthesis of Heterocyclic gamma-Amino-Acid and Diamine Derivatives by Three-Component Radical Cascade Reactions, Angew. Chem., Int. Ed., 2019, 58, 15803–15807 CrossRef CAS PubMed.
- J.-L. Li, Y.-Q. Liu, W.-L. Zou, R. Zeng, X. Zhang, Y. Liu, B. Han, Y. He, H.-J. Leng and Q.-Z. Li, Radical Acylfluoroalkylation of Olefins through N-Heterocyclic Carbene Organocatalysis, Angew. Chem., Int. Ed., 2020, 59, 1863–1870 CrossRef CAS PubMed.
- W. Shu, E. Merino and C. Nevado, Visible Light Mediated, Redox Neutral Remote 1,6-Difunctionalizations of Alkenes, ACS Catal., 2018, 8, 6401–6406 CrossRef CAS.
- J. Zhang, W. Jin, C. Cheng and F. Luo, Copper-catalyzed remote oxidation of alcohols initiated by radical difluoroalkylation of alkenes: facile access to difluoroalkylated carbonyl compounds, Org. Biomol. Chem., 2018, 16, 3876–3880 RSC.
- L. Li, H. Luo, Z. Zhao, Y. Li, Q. Zhou, J. Xu, J. Li and Y. N. Ma, Photoredox-Catalyzed Remote Difunctionalizations of Alkenes To Synthesize Fluoroalkyl Ketones with Dimethyl Sulfoxide as the Oxidant, Org. Lett., 2019, 21, 9228–9231 CrossRef CAS PubMed.
- G.-T. Song, C.-H. Qu, J.-P. Meng, Z.-G. Xu, C.-H. Zhou and Z.-Z. Chen, Photoredox catalytic cascade radical addition/aromatization of methylene-2-oxazolines: Mild access to C(sp3)-difluoro-oxazole derivatives, Tetrahedron Lett., 2019, 60, 151246 CrossRef CAS.
- T. Brandhofer and O. G. Mancheño, Versatile Ru-Photoredox-Catalyzed Functionalization of Dehydro-Amino Acids and Peptides, ChemCatChem, 2019, 11, 3797–3801 CrossRef CAS.
- A. Correa and R. Martín, Metal-Catalyzed Carboxylation of Organometallic Reagents with Carbon Dioxide, Angew. Chem., Int. Ed., 2009, 48, 6201–6204 CrossRef CAS PubMed.
- M. Börjesson, T. Moragas, D. Gallego and R. Martin, Metal-Catalyzed Carboxylation of Organic (Pseudo)halides with CO2, ACS Catal., 2016, 6, 6739–6749 CrossRef PubMed.
- S. Wang, G. Du and C. Xi, Copper-catalyzed carboxylation reactions using carbon dioxide, Org. Biomol. Chem., 2016, 14, 3666–3676 RSC.
- Q. Zhu, L. Wang, C. Xia and C. Liu, Recent Advance of Transition Metal-Catalyzed Direct C-H Bond Carboxylation with CO2, Chin. J. Org. Chem., 2016, 36, 2813–2821 CrossRef CAS.
- S. Zhang, X. Li and L.-N. He, Reductive Carboxylation of Unsaturated Hydrocarbons with Carbon Dioxide, Acta Chim. Sin., 2016, 74, 17–23 CrossRef CAS.
- W. Zhang, C. Guo and X. Lü, Ni-catalyzed direct carboxylation of unactivated alkyl electrophiles with carbon dioxide, Chin. J. Catal., 2016, 37, 215–217 CrossRef CAS.
- Y.-G. Chen, X.-T. Xu, K. Zhang, Y.-Q. Li, L.-P. Zhang, P. Fang and T.-S. Mei, Transition-Metal-Catalyzed Carboxylation of Organic Halides and Their Surrogates with Carbon Dioxide, Synthesis, 2018, 35–48 Search PubMed.
- X.-F. Wu and F. Zheng, Synthesis of Carboxylic Acids and Esters from CO2, Top. Curr. Chem., 2017, 375, 4 CrossRef PubMed.
- Z. B. Yin, J. H. Ye, W. J. Zhou, Y. H. Zhang, L. Ding, Y. Y. Gui, S. S. Yan, J. Li and D. G. Yu, Oxy-Difluoroalkylation of Allylamines with CO2 via Visible-Light Photoredox Catalysis, Org. Lett., 2018, 20, 190–193 CrossRef CAS PubMed.
- F. Xiao, F. Wu, X. Yang, Y. Shen and X. Shi, A convenient synthesis of fluoroalkylated γ-butyrolactones from polyfluoroalkyl iodides and 4-pentenoic acid catalyzed by Pd(PPh3)4, J. Fluorine Chem., 2005, 126, 319–323 CrossRef CAS.
- W. Sha, W. Zhang, S. Ni, H. Mei, J. Han and Y. Pan, Photoredox-Catalyzed Cascade Difluoroalkylation and Intramolecular Cyclization for Construction of Fluorinated γ-Butyrolactones, J. Org. Chem., 2017, 82, 9824–9831 CrossRef CAS PubMed.
- Y. Da, S. Han, X. Du, S. Liu, L. Liu and J. Li, Copper(I)-Catalyzed Oxydifluoroalkylation of Alkenes: A Route to Functionalization of Lactones, Org. Lett., 2018, 20, 5149–5152 CrossRef CAS PubMed.
- F. Y. Yuan, S. Zhou, Y. Y. Yang, M. J. Guo, X. Y. Tang and G. W. Wang, Copper catalyzed one-pot difluoroalkylation and lactonization of unsaturated carboxylic acids, Org. Chem. Front., 2018, 5, 3306–3309 RSC.
- Q. Yang, Q. Q. Lin, H. Y. Xing and Z. G. Zhao, Visible-light-mediated difluoromethylphosphonation of alkenes for the synthesis of CF2P-containing heterocycles, Org. Chem. Front., 2019, 6, 3939–3943 RSC.
- Y. Yang, F. Yuan, X. Ren, G. Wang, W. Zhao, X. Tang and M. Guo, Copper-Catalyzed Oxydifluoroalkylation of Hydroxyl-Containing Alkenes, J. Org. Chem., 2019, 84, 4507–4516 CrossRef CAS PubMed.
- X. Wang, M. Li, Y. Yang, M. Guo, X. Tang and G. Wang, One-pot Construction of Difluorinated Pyrrolizidine and Indolizidine Scaffolds via Copper-Catalyzed Radical Cascade Annulation, Adv. Synth. Catal., 2018, 360, 2151–2156 CrossRef CAS.
- J. Liao, L. Ouyang, Y. Lai and R. Luo, Photoredox-Catalyzed Oxy-/Aminofluoroalkylative Cyclization of Alkenes, J. Org. Chem., 2020, 85, 5590–5597 CrossRef CAS PubMed.
- J. J. Yu, D. P. Wang, Y. Xu, Z. Wu and C. Zhu, Distal Functional Group Migration for Visible-light Induced Carbo-difluoroalkylation/monofluoroalkylation of Unactivated Alkenes, Adv. Synth. Catal., 2018, 360, 744–750 CrossRef CAS.
- W. G. Kong, C. J. Yu, H. J. An and Q. L. Song, Copper-Catalyzed Intermolecular Reductive Radical Difluoroalkylation-Thiolation of Aryl Alkenes, Org. Lett., 2018, 20, 4975–4978 CrossRef CAS PubMed.
- Y. Chen, L. Li, Y. Ma and Z. Li, Cobalt-Catalyzed Three-Component Difluoroalkylation-Peroxidation of Alkenes, J. Org. Chem., 2019, 84, 5328–5338 CrossRef CAS PubMed.
- R. Xu and C. Cai, Three- component difluoroalkylation- thiolation of alkenes by iron- facilitated visible- light photoredox catalysis, Chem. Commun., 2019, 55, 4383–4386 RSC.
- K. S. Hagen, J. G. Reynolds and R. H. Holm, Definition of reaction sequences resulting in self-assembly of [Fe4S4(SR)4]2- clusters from simple reactants, J. Am. Chem. Soc., 1981, 103, 4054–4063 CrossRef CAS.
- R. Xu and C. Cai, Three-component difluoroalkylamination of alkenes mediated by photoredox and iron cooperative catalysis, Org. Biomol. Chem., 2019, 17, 8541–8545 RSC.
- Y. Murata, T. Shimada and T. Nishikata, Radical and Cation Crossover Reaction System Enables Synthesis of Complex Aliphatic Chains Possessing Functionalized Quaternary Carbons, Bull. Chem. Soc. Jpn., 2019, 92, 1419–1429 CrossRef CAS.
- G. H. Li, D. Q. Dong, Q. Deng, S. Q. Yan and Z. L. Wang, Copper-Catalyzed Deoxygenative C2-Sulfonylation of Quinoline N-Oxides with DABSO and Phenyldiazonium Tetrafluoroborates for the Synthesis of 2-Sulfonylquinolines via a Radical Reaction, Synthesis, 2019, 3313–3319 CAS.
- G. Qiu, L. Lai, J. Cheng and J. Wu, Recent advances in the sulfonylation of alkenes with the insertion of sulfur dioxide via radical reactions, Chem. Commun., 2018, 54, 10405–10414 RSC.
- S. Ye, G. Qiu and J. Wu, Inorganic sulfites as the sulfur dioxide surrogates in sulfonylation reactions, Chem. Commun., 2019, 55, 1013–1019 RSC.
- Y. Liu, Q. Lin, Z. Xiao, C. Zheng, Y. Guo, Q. Y. Chen and C. Liu, Zinc-Mediated Intermolecular Reductive Radical Fluoroalkylsulfination of Unsaturated Carbon-Carbon Bonds with Fluoroalkyl Bromides and Sulfur Dioxide, Chemistry, 2019, 25, 1824–1828 CrossRef CAS PubMed.
- C. Xu, Z.-F. Yang, L. An and X. Zhang, Nickel-Catalyzed Difluoroalkylation–Alkylation of Enamides, ACS Catal., 2019, 9, 8224–8229 CrossRef CAS.
- B. Zhao, Z. Li, Y. Wu, Y. Wang, J. Qian, Y. Yuan and Z. Shi, An Olefinic 1,2-Boryl-Migration Enabled by Radical Addition: Construction of gem-Bis(boryl)alkanes, Angew. Chem., Int. Ed., 2019, 58, 9448–9452 CrossRef CAS PubMed.
- D. K. Li, T. T. Mao, J. B. Huang and Q. Zhu, Copper-Catalyzed Bromodifluoroacetylation of Alkenes with Ethyl Bromodifluoroacetate, J. Org. Chem., 2018, 83, 10445–10452 CrossRef CAS PubMed.
- L. Zhao, Y. Huang, Z. Wang, E. Zhu, T. Mao, J. Jia, J. W. Gu, X. F. Li and C. Y. He, Organophosphine-Catalyzed Difluoroalkylation of Alkenes, Org. Lett., 2019, 21, 6705–6709 CrossRef CAS PubMed.
- J. Sheng, K. J. Bian, Y. M. Su, G. X. Liao, R. M. Duan, C. Li, Z. H. Liu and X. S. Wang, Visible light-mediated atom transfer radical addition to styrene: base controlled selective (phenylsulfonyl)difluoromethylation, Org. Chem. Front., 2020, 7, 617–621 RSC.
- L. X. Li, Y. N. Ma, M. Tang, J. Guo, Z. Yang, Y. Z. Yan, X. T. Ma and L. Tang, Photoredox-Catalyzed Oxydifluoroalkylation of Styrenes for Access to Difluorinated Ketones with DMSO as an Oxidant, Adv. Synth. Catal., 2019, 361, 3723–3728 CrossRef CAS.
- S. Tian, X. Song, D. Zhu and M. Wang, Alternative Palladium-Catalyzed Vinylic C–H Difluoroalkylation of Ketene Dithioacetals Using Bromodifluoroacetate Derivatives, Adv. Synth. Catal., 2018, 360, 1414–1419 CrossRef CAS.
- Y. N. Zhao, Y. C. Luo, Z. Y. Wang and P. F. Xu, A new approach to access difluoroalkylated diarylmethanes via visible-light photocatalytic cross-coupling reactions, Chem. Commun., 2018, 54, 3993–3996 RSC.
- J. M. Muñoz-Molina and P. J. Perez, Ruthenium-Catalyzed Heck-Type Alkenylation of Alkyl Bromides, J. Org. Chem., 2019, 84, 8289–8296 CrossRef PubMed.
- M. Uno, S. Sumino, T. Fukuyama, M. Matsuura, Y. Kuroki, Y. Kishikawa and I. Ryu, Synthesis of 4,4-Difluoroalkenes by Coupling of alpha-Substituted alpha,alpha-Difluoromethyl Halides with Allyl Sulfones under Photoredox Catalyzed Conditions, J. Org. Chem., 2019, 84, 9330–9338 CrossRef CAS PubMed.
- X. Li, R. Zhang, X. Zhang, P. Zhu and T. Yao, Silver-Catalyzed Decarboxylative Allylation of Difluoroarylacetic Acids with Allyl Sulfones in Water, Chem.– Asian J., 2020, 15, 1175–1179 CrossRef CAS PubMed.
- Y. W. Sun and G. Z. Zhang, Photoinduced Decarboxylative Amino-Fluoroalkylation of Maleic Anhydride, Chem. – Eur. J., 2020, 26, 419–422 CrossRef CAS PubMed.
- E. Sekino, T. Kumamoto, T. Tanaka, T. Ikeda and T. Ishikawa, Concise Synthesis of Anti-HIV-1 Active (+)-Inophyllum B and (+)-Calanolide A by Application of (−)-Quinine-Catalyzed Intramolecular Oxo-Michael Addition, J. Org. Chem., 2004, 69, 2760–2767 CrossRef CAS PubMed.
- P. Anand, B. Singh and N. Singh, A review on coumarins as acetylcholinesterase inhibitors for Alzheimer's disease, Bioorg. Med. Chem., 2012, 20, 1175–1180 CrossRef CAS PubMed.
- A. M. Musiliyu, S. C. John and M. O. F. Khan, A Review of Coumarin Derivatives in Pharmacotherapy of Breast Cancer, Curr. Med. Chem., 2008, 15, 2664–2679 CrossRef PubMed.
- S. H. Hao, L. X. Li, D. Q. Dong, Z. L. Wang and X. Y. Yu, Synthesis of coumarins derivatives via decarboxylative cross-coupling of coumarin-3-carboxylic acid with benzylic C(sp(3))-H bond, Tetrahedron Lett., 2018, 59, 4073–4075 CrossRef CAS.
- D. Song, C. M. Wang, Z. P. Ye, P. J. Xia, Z. X. Deng, J. A. Xiao, H. Y. Xiang and H. Yang, Visible-Light-Driven, Photoredox-Catalyzed Cascade of ortho-Hydroxycinnamic Esters To Access 3-Fluoroalkylated Coumarins, J. Org. Chem., 2019, 84, 7480–7487 CrossRef CAS PubMed.
- S. B. Nagode, A. K. Chaturvedi and N. Rastogi, Visible-light-catalyzed Tandem Difluoroacetylation–Intramolecular Cyclization of 1,3-Diarylpropynones: Access to Difluoroacetylated Indenones, Asian J. Org. Chem., 2017, 6, 453–457 CrossRef CAS.
- T. T. Zhang, M. J. Luo, F. Teng, Y. Li, M. Hu and J. H. Li, Photoredox Alkylarylation of N -Benzyl- N -(2-ethynylaryl)-Amides with α-Bromoalkyl Esters: Access to Dibenzazepines, Adv. Synth. Catal., 2019, 361, 4645–4650 CrossRef CAS.
- D. Liu, M. J. Jiao, X. Z. Wang and P. F. Xu, Metal-Free Visible-Light-Induced Construction of Difluoro-Containing Dibenzazepines, Org. Lett., 2019, 21, 4745–4749 CrossRef CAS PubMed.
- Y. Zhang, J. Zhang, B. Hu, M. Ji, S. Ye and G. Zhu, Synthesis of Difluoromethylated and Phosphorated Spiro[5.5]trienones via Dearomative Spirocyclization of Biaryl Ynones, Org. Lett., 2018, 20, 2988–2992 CrossRef CAS PubMed.
- S. Han, S. Liu, L. Liu, L. Ackermann and J. Li, Cobalt-Catalyzed Diastereoselective Difluoroalkylation/Giese Addition Domino Reactions, Org. Lett., 2019, 21, 5387–5391 CrossRef CAS PubMed.
- D. Y. Qian and J. L. Zhang, Gold-catalyzed cyclopropanation reactions using a carbenoid precursor toolbox, Chem. Soc. Rev., 2015, 44, 677–698 RSC.
- D. M. Patterson, L. A. Nazarova, B. Xie, D. N. Kamber and J. A. Prescher, Functionalized Cyclopropenes As Bioorthogonal Chemical Reporters, J. Am. Chem. Soc., 2012, 134, 18638–18643 CrossRef CAS PubMed.
- Y. Y. Yang, P. Antoni, M. Zimmer, K. Sekine, F. F. Mulks, L. Hu, L. M. Zhang, M. Rudolph, F. Rominger and A. S. K. Hashmi, Dual Gold/Silver Catalysis Involving Alkynylgold(III) Intermediates Formed by Oxidative Addition and Silver-Catalyzed C-H Activation for the Direct Alkynylation of Cyclopropenes, Angew. Chem., Int. Ed., 2019, 58, 5129–5133 CrossRef CAS PubMed.
- K. Chen, X. Y. Huang, S. B. J. Kan, R. K. Zhang and F. H. Arnold, Enzymatic construction of highly strained carbocycles, Science, 2018, 360, 71–75 CrossRef CAS PubMed.
- Z.-Q. Zhang, M.-M. Zheng, X.-S. Xue, I. Marek, F.-G. Zhang and J.-A. Ma, Catalytic Enantioselective Cyclopropenation of Internal Alkynes: Access to Difluoromethylated Three-Membered Carbocycles, Angew. Chem., Int. Ed., 2019, 58, 18191–18196 CrossRef CAS PubMed.
- X. Feng, X. Wang, H. Chen, X. Tang, M. Guo, W. Zhao and G. Wang, Copper-mediated regioselective hydrodifluoroalkylation of alkynes, Org. Biomol. Chem., 2018, 16, 2841–2845 RSC.
- W. Q. Fu and Q. Song, Copper-Catalyzed Radical Difluoroalkylation and Redox Annulation of Nitroalkynes for the Construction of C2-Tetrasubstituted Indolin-3-ones, Org. Lett., 2018, 20, 393–396 CrossRef CAS PubMed.
- J. Xu, F. Zhang, S. Zhang, L. Zhang, X. Yu, J. Yan and Q. Song, Radical Promoted C(sp(2))-S Formation and C(sp(3))-S Bond Cleavage: Access to 2-Substituted Thiochromones, Org. Lett., 2019, 21, 1112–1115 CrossRef CAS PubMed.
- Y. Zhang, S. Ye, M. Ji, L. Li, D. Guo and G. Zhu, Copper-Catalyzed Radical Cascade Difluoromethylation/Cyclization of 2-(3-Arylpropioloyl)benzaldehydes: A Route to Difluoromethylated Naphthoquinones, J. Org. Chem., 2017, 82, 6811–6818 CrossRef CAS PubMed.
- H.-L. Hua, B.-S. Zhang, Y.-T. He, Y.-F. Qiu, J.-Y. Hu, Y.-C. Yang and Y.-M. Liang, Copper-catalyzed difluoromethylation of propargylamide-substituted indoles: synthesis of mono- and bis-difluoromethylated indoloazepinone derivatives, Chem. Commun., 2016, 52, 10396–10399 RSC.
- J. W. Ma, Q. Wang, X. G. Wang and Y. M. Liang, Palladium-Catalyzed Cascade Difluoroalkylation/Cyclization of N-Propargylamides: Synthesis of Oxazoles and Oxazolines, J. Org. Chem., 2018, 83, 13296–13307 CrossRef CAS PubMed.
- G. Wu and A. Jacobi von Wangelin, Stereoselective cobalt-catalyzed halofluoroalkylation of alkynes, Chem. Sci., 2018, 9, 1795–1802 RSC.
-
(a) S. F. Wang, J. Zhang, L. C. Kong, Z. Tan, Y. H. Bai and G. G. Zhu, Palladium-Catalyzed anti-Selective Fluoroalkylboration of Internal and Terminal Alkynes, Org. Lett., 2018, 20, 5631–5635 CrossRef CAS PubMed;
(b) W.-H. Guo, H.-Y. Zhao, Z.-J. Luo, S. Zhang and X. Zhang, Fluoroalkylation–Borylation of Alkynes: An Efficient Method To Obtain (Z) Tri- and Tetrasubstituted Fluoroalkylated Alkenylboronates, ACS Catal., 2019, 9, 38–43 CrossRef CAS.
- B. S. Zhang, L. Y. Gao, Z. Zhang, Y. H. Wen and Y. M. Liang, Three-component difluoroalkylation and trifluoromethylthiolation/trifluoromethylselenolation of pi-bonds, Chem. Commun., 2018, 54, 1185–1188 RSC.
- T. Mao, M. J. Ma, L. Zhao, D. P. Xue, Y. B. Yu, J. W. Gu and C. Y. He, A general and green fluoroalkylation reaction promoted via noncovalent interactions between acetone and fluoroalkyl iodides, Chem. Commun., 2020, 56, 1815–1818 RSC.
- N. Iqbal, N. Iqbal, S. S. Han and E. J. Cho, Synthesis of fluoroalkylated alkynes via visible-light photocatalysis, Org. Biomol. Chem., 2019, 17, 1758–1762 RSC.
- X. Wang, J. Hu, J. Ren, T. Wu, J. Wu and F. Wu, Palladium-catalyzed one-pot construction of difluorinated 1,3-enynes from α,α,α-iododifluoroacetones and alkynes, Tetrahedron, 2019, 75, 130715 CrossRef CAS.
- G. Tu, C. Yuan, Y. Li, J. Zhang and Y. Zhao, A Ligand-Enabled Palladium-Catalyzed Highly para-Selective Difluoromethylation of Aromatic Ketones, Angew. Chem., Int. Ed., 2018, 57, 15597–15601 CrossRef CAS PubMed.
- Y. J. Mao, B. X. Wang, Q. Z. Wu, K. Zhou, S. J. Lou and D. Q. Xu, Pd-Catalyzed para-selective C-H difluoromethylation of aromatic carbonyls, Chem. Commun., 2019, 55, 2019–2022 RSC.
- J. Zhou, F. Wang, Z. Lin, C. Cheng, Q. Zhang and J. Li, Visible-Light-Induced para-Selective C(sp(2))-H Difluoroalkylation of Diverse (Hetero)aromatic Carbonyls, Org. Lett., 2020, 22, 68–72 CrossRef CAS PubMed.
- W. K. Tang, F. Tang, J. Xu, Q. Zhang, J. J. Dai, Y. S. Feng and H. J. Xu, Photocatalytic site-selective C-H difluoroalkylation of aromatic aldehydes, Chem. Commun., 2020, 56, 1497–1500 RSC.
- C. Yuan, L. Zhu, R. Zeng, Y. Lan and Y. Zhao, Ruthenium(II)-Catalyzed C-H Difluoromethylation of Ketoximes: Tuning the Regioselectivity from the meta to the para Position, Angew. Chem., Int. Ed., 2018, 57, 1277–1281 CrossRef CAS PubMed.
- P. Gandeepan, J. Koeller, K. Korvorapun, J. Mohr and L. Ackermann, Visible-Light-Enabled Ruthenium-Catalyzed meta-C-H Alkylation at Room Temperature, Angew. Chem., Int. Ed., 2019, 58, 9820–9825 CrossRef CAS PubMed.
- C. Jia, S. Wang, X. Lv, G. Li, L. Zhong, L. Zou and X. Cui, Ruthenium-Catalyzed meta-CAr–H Bond Difluoroalkylation of 2-Phenoxypyridines, Eur. J. Org. Chem., 2020, 1992–1995 CrossRef CAS.
- S. Cai, Y. Tian, J. Zhang, Z. Liu, M. Lu, W. Weng and M. Huang, Carbotrifluoromethylation of Allylic Alcohols via 1,2-Aryl Migration Promoted by Visible-Light-Induced Photoredox Catalysis, Adv. Synth. Catal., 2018, 360, 4084–4088 CrossRef CAS.
- X. Wang, S. Zhao, J. Liu, D. Zhu, M. Guo, X. Tang and G. Wang, Copper-Catalyzed C–H Difluoroalkylations and Perfluoroalkylations of Alkenes and (Hetero)arenes, Org. Lett., 2017, 19, 4187–4190 CrossRef CAS PubMed.
- Q. Lin, L. Chu and F.-L. Qing, Direct Introduction of Ethoxycarbonyldifluoromethyl-Group to Heteroarenes with Ethyl Bromodifluoroacetate via Visible-Light Photocatalysis, Chin. J. Chem., 2013, 31, 885–891 CrossRef CAS.
- S. Y. Yan, Z. Z. Zhang, Y. H. Liu, G. Liao, P. X. Li and B. F. Shi, Copper-Catalyzed C-H Ethoxycarbonyldifluoromethylation of Indoles and Pyrroles, Asian J. Org. Chem., 2018, 7, 1319–1322 CrossRef CAS.
-
(a) V. Soni, D. M. Sharma and B. Punji, Nickel-Catalyzed Regioselective C(2)-H Difluoroalkylation of Indoles with Difluoroalkyl Bromides, Chem. – Asian J., 2018, 13, 2516–2521 CrossRef CAS PubMed;
(b) L. Ackermann, B. Punji and W. Song, User-Friendly [(Diglyme)NiBr2]-Catalyzed Direct Alkylations of Heteroarenes with Unactivated Alkyl Halides through C
H Bond Cleavages, Adv. Synth. Catal., 2011, 353, 3325–3329 CrossRef CAS.
- C. Bottecchia, R. Martín, I. Abdiaj, E. Crovini, J. Alcazar, J. Orduna, M. J. Blesa, J. R. Carrillo, P. Prieto and T. Noël, De novo Design of Organic Photocatalysts: Bithiophene Derivatives for the Visible-light Induced C–H Functionalization of Heteroarenes, Adv. Synth. Catal., 2019, 361, 945–950 CrossRef CAS.
- X. Chen, W. Xu, K. Wang, M. Mo, W. Zhang, L. Du, X. Yuan, Y. Xu, Y. Wang and J. Shen, Discovery of a Novel Series of Imidazo[1,2-a]pyrimidine Derivatives as Potent and Orally Bioavailable Lipoprotein-Associated Phospholipase A2 Inhibitors, J. Med. Chem., 2015, 58, 8529–8541 CrossRef CAS PubMed.
- E.-G. Cecile and G. Alain, Recent Progress in the Pharmacology of Imidazo[1,2-a]pyridines, Mini-Rev. Med. Chem., 2007, 7, 888–899 CrossRef PubMed.
- S. Mishra, P. Mondal, M. Ghosh, S. Mondal and A. Hajra, Copper-catalyzed C–H
ethoxycarbonyldifluoromethylation of imidazoheterocycles, Org. Biomol. Chem., 2016, 14, 1432–1436 RSC.
- G. Yin, M. Zhu and W. Fu, Visible-light-induced photocatalytic difluoroacetylation of imidazopyridines via direct and regioselective C H functionalization, J. Fluorine Chem., 2017, 199, 14–19 CrossRef CAS.
- M. Q. Hua, F. Y. Mou, N. H. Wang, Y. Chen, H. Y. Xiong, Y. Huang, C. L. Lei and Q. Zhang, Visible-light-induced photocatalytic difluoroalkylation of 3-substituted benzo d isoxazoles via direct and regioselective C-H functionalization, Tetrahedron Lett., 2018, 59, 4449–4453 CrossRef CAS.
- M. Singsardar, S. Mondal, S. Laru and A. Hajra, Organophotoredox-Catalyzed C(sp(2))-H Difluoromethylenephosphonation of Imidazoheterocycles, Org. Lett., 2019, 21, 5606–5610 CrossRef CAS PubMed.
- C. H. Qu, G. T. Song, J. Xu, W. Yan, C. H. Zhou, H. Y. Li, Z. Z. Chen and Z. G. Xu, Merging Visible Light with Cross-Coupling: The Photochemical Direct C-H Difluoroalkylation of Imidazopyridines, Org. Lett., 2019, 21, 8169–8173 CrossRef CAS PubMed.
- S. Han, A. Liang, X. Ren, X. Gao, J. Li, D. Zou, Y. Wu and Y. Wu, Copper-catalyzed remote CH ethoxycarbonyldifluoromethylation of 8-aminoquinolines with bis(pinacolato)diboron as reductant, Tetrahedron Lett., 2017, 58, 4859–4863 CrossRef CAS.
- H. Chen, P. Li, M. Wang and L. Wang, Nickel-Catalyzed Site-Selective C–H Bond Difluoroalkylation of 8-Aminoquinolines on the C5-Position, Org. Lett., 2016, 18, 4794–4797 CrossRef CAS PubMed.
- C. Chen, R. Zeng, J. Zhang and Y. Zhao, Ruthenium-Catalyzed Difluoroalkylation of 8-Aminoquinoline Amides at the C5-Position, Eur. J. Org. Chem., 2017, 6947–6950 CrossRef CAS.
- H. Lu, D. Y. Wang and A. Zhang, Visible Light-Promoted Phosphine-Catalyzed Difluoroalkylation of Arenes and Heterocycles, J. Org. Chem., 2020, 85, 942–951 CrossRef CAS PubMed.
- S. Leilei, H. Wei, W. Jifeng, Z. Huaiyu, Z. Hua and L. Xun, Quinoxalinone as a Privileged Platform in Drug Development, Mini-Rev. Med. Chem., 2018, 18, 392–413 CrossRef PubMed.
- L.-Y. Xie, Y.-L. Chen, L. Qin, Y. Wen, J.-W. Xie, J.-X. Tan, Y. Huang, Z. Cao and W.-M. He, Visible-light-promoted direct C–H/S–H cross-coupling of quinoxalin-2(1H)-ones with thiols leading to 3-sulfenylated quinoxalin-2(1H)-ones in air, Org. Chem. Front., 2019, 6, 3950–3955 RSC.
- L.-Y. Xie, Y.-S. Bai, X.-Q. Xu, X. Peng, H.-S. Tang, Y. Huang, Y.-W. Lin, Z. Cao and W.-M. He, Visible-light-induced decarboxylative acylation of quinoxalin-2(1H)-ones with α-oxo carboxylic acids under metal-, strong oxidant- and external photocatalyst-free conditions, Green Chem., 2020, 22, 1720–1725 RSC.
- L.-Y. Xie, Y.-S. Liu, H.-R. Ding, S.-F. Gong, J.-X. Tan, J.-Y. He, Z. Cao and W.-M. He, C(sp2)–H/O–H cross-dehydrogenative coupling of quinoxalin-2(1H)-ones with alcohols under visible-light photoredox catalysis, Chin. J. Catal., 2020, 41, 1168–1173 CrossRef CAS.
- Y. W. Bao, Z. Wang, C. Chen, B. L. Zhu, Y. B. Wang, J. H. Zhao, J. Y. Gong, M. Y. Han and C. Liu, Palladium-catalyzed tandem cyclization of fluorinated imidoyl chlorides with 2-bromophenylboronic acid: Synthesis of 6-fluoroalkyl-phenanthridines, Tetrahedron, 2019, 75, 1450–1456 CrossRef CAS.
- C. Jin, X. H. Zhuang, B. Sun, D. Y. Li and R. Zhu, Merging Visible-Light Photoredox and Organoamine Catalysis for the C-3 Difluoroalkylation of Quinoxalin-2(1H)-Ones, Asian J. Org. Chem., 2019, 8, 1490–1494 CrossRef CAS.
- G. F. Hong, J. W. Yuan, J. H. Fu, G. Y. Pan, Z. W. Wang, L. R. Yang, Y. M. Xiao, P. Mao and X. M. Zhang, Transition-metal-free decarboxylative C3-difluoroarylmethylation of quinoxalin-2(1H)-ones with alpha,alpha-difluoroarylacetic acids, Org. Chem. Front., 2019, 6, 1173–1182 RSC.
- X. J. Xie, Y. F. Zhang, J. Hao and W. Wan, Ag-Catalyzed minisci C-H difluoromethylarylation of N-heteroarenes, Org. Biomol. Chem., 2020, 18, 400–404 RSC.
- L. An, Y. L. Xiao, S. Zhang and X. G. Zhang, Bulky Diamine Ligand Promotes Cross-Coupling of Difluoroalkyl Bromides by Iron Catalysis, Angew. Chem., Int. Ed., 2018, 57, 6921–6925 CrossRef CAS PubMed.
- J. Dai, W. Lei and Q. Liu, Visible-Light-Driven Difluoroalkylation of Aromatics Catalyzed by Copper, Acta Chim. Sin., 2019, 77, 911 CrossRef.
- M. J. Hossain, T. Ono, Y. Yano and Y. Hisaeda, Learning From Vitamin B-12-Mediated Reactions: Cobalt(III)-Carbon-Assisted Catalytic C-H Difluoroacylation of (Hetero)Arenes through Controlled-Potential Electrolysis, ChemElectroChem, 2019, 6, 4199–4203 CrossRef CAS.
- M. Ke and Q. Song, Copper/B2pin2-catalyzed C-H difluoroacetylation-cycloamidation of anilines leading to the formation of 3,3-difluoro-2-oxindoles, Chem. Commun., 2017, 53, 2222–2225 RSC.
- L. C. Yu, J. W. Gu, S. Zhang and X. Zhang, Visible-Light-Promoted Tandem Difluoroalkylation-Amidation: Access to Difluorooxindoles from Free Anilines, J. Org. Chem., 2017, 82, 3943–3949 CrossRef CAS PubMed.
- G. Lian, J. Li, P. Liu and P. Sun, Photoredox-Catalyzed Radical Cascade Reaction To Synthesize Fluorinated Pyrrolo[1,2-d]benzodiazepine Derivatives, J. Org. Chem., 2019, 84, 9322–9329 CrossRef CAS PubMed.
- A. Z. Cao, Y. T. Xiao, Y. C. Wu, R. J. Song, Y. X. Xie and J. H. Li, Copper-catalyzed C-H [3 + 2] annulation of N-substituted anilines with alpha-carbonyl alkyl bromides via C(sp(3))-Br/C(sp(2))-H functionalization, Org. Biomol. Chem., 2020, 18, 2170–2174 RSC.
- Y. Gao, C. P. Li, B. Xu and H. Liu, Rapid access to difluoroalkylated pyrrolobenzodiazepines via a Pd-catalyzed C-H difluoroalkylation/cyclization cascade reaction, Org. Chem. Front., 2019, 6, 410–414 RSC.
- H. Li, B.-J. Li and Z.-J. Shi, Challenge and progress: palladium-catalyzed sp3 C–H activation, Catal. Sci. Technol., 2011, 1, 191–206 RSC.
- K. M. Engle, T.-S. Mei, M. Wasa and J.-Q. Yu, Weak Coordination as a Powerful Means for Developing Broadly Useful C–H Functionalization Reactions, Acc. Chem. Res., 2012, 45, 788–802 CrossRef CAS PubMed.
- D. Q. Dong, X. Gao, L. X. Li, S. H. Hao and Z. L. Wang, Sulfonylation of C(sp(3))-H bond for synthesis of 2-sulfolmethyl azaarenes catalyzed by TBAI in water, Res. Chem. Intermed., 2018, 44, 7557–7567 CrossRef CAS.
- D. Q. Dong, S. H. Hao and Z. L. Wang, Functionalization of C(sp(3))-H Bonds Adjacent to Oxygen of Alcohols under Metal-free Conditions, Mini-Rev. Org. Chem., 2017, 14, 130–135 CrossRef CAS.
- S. H. Hao, L. X. Li, D. Q. Dong and Z. L. Wang, Direct construction of sulfenylated pyrazoles catalyzed by I-2 at room temperature, Chin. J. Catal., 2017, 38, 1664–1667 CrossRef CAS.
- Y.-Z. Li, B.-J. Li, X.-Y. Lu, S. Lin and Z.-J. Shi, Cross Dehydrogenative Arylation (CDA) of a Benzylic C
H Bond with Arenes by Iron Catalysis, Angew. Chem., Int. Ed., 2009, 48, 3817–3820 CrossRef CAS PubMed.
- X. Wan, Z. Ma, B. Li, K. Zhang, S. Cao, S. Zhang and Z. Shi, Highly Selective C–H Functionalization/Halogenation of Acetanilide, J. Am. Chem. Soc., 2006, 128, 7416–7417 CrossRef CAS PubMed.
- L. X. Li, D. Q. Dong, S. H. Hao and Z. L. Wang, Direct sulfonylation of pyrazolones with sodium sulfinates catalyzed
by TBAI in water, Tetrahedron Lett., 2018, 59, 1517–1520 CrossRef CAS.
- S. Q. Yan, D. Q. Dong, C. W. Xie, W. S. Wang and Z. L. Wang, Synthesis of Bicyclic ortho-Aminocarbonitrile Derivatives Catalyzed by 1,4-Diazabicyclo 2.2.2 octane, Chin. J. Org. Chem., 2019, 39, 2560–2566 CrossRef.
- D. Q. Dong, W. J. Chen, D. M. Chen, L. X. Li, G. H. Li, Z. L. Wang, Q. Deng and S. Long, Direct Synthesis of Sulfonated or Sulfenylated Pyrazolones Mediated by KIO3 and Their Anti -microbial Activity, Chin. J. Org. Chem., 2019, 39, 3190–3198 CrossRef.
- D. Q. Dong, G. H. Li, D. M. Chen, Y. Y. Sun, Q. Q. Han, Z. L. Wang, X. Xu and X. Yu, Chin. J. Org. Chem., 2020, 40, 1766–1771 CrossRef.
- M. Yang, B. Su, Y. Wang, K. Chen, X. Jiang, Y.-F. Zhang, X.-S. Zhang, G. Chen, Y. Cheng, Z. Cao, Q.-Y. Guo, L. Wang and Z.-J. Shi, Silver-catalysed direct amination of unactivated C–H bonds of functionalized molecules, Nat. Commun., 2014, 5, 4707 CrossRef CAS PubMed.
- Y. Li, J. Liu, S. Zhao, X. Du, M. Guo, W. Zhao, X. Tang and G. Wang, Copper-Catalyzed Fluoroolefination of Silyl Enol Ethers and Ketones toward the Synthesis of beta-Fluoroenones, Org. Lett., 2018, 20, 917–920 CrossRef CAS PubMed.
- W.-P. Li, Y.-C. Zhu, Y.-J. Zhou, H.-W. Yang and C.-J. Zhu, Visible light induced C-H monofluoroalkylation to synthesize 1,4-unsaturated compound, Tetrahedron, 2019, 75, 1647–1651 CrossRef CAS.
- J. Liu, W. Ding, Q. Q. Zhou, D. Liu, L. Q. Lu and W. J. Xiao, Enantioselective Di-/Perfluoroalkylation of beta-Ketoesters Enabled by Cooperative Photoredox/Nickel Catalysis, Org. Lett., 2018, 20, 461–464 CrossRef CAS PubMed.
- C. Li, Y. X. Cao, R. Wang, Y. N. Wang, Q. Lan and X. S. Wang, Cobalt-catalyzed difluoroalkylation of tertiary aryl ketones for facile synthesis of quaternary alkyl difluorides, Nat. Commun., 2018, 9, 4951 CrossRef PubMed.
- C. Li, Y. X. Cao, R. X. Jin, K. J. Bian, Z. Y. Qin, Q. Lan and X. S. Wang, Highly stereoselective nickel-catalyzed difluoroalkylation of aryl ketones to tetrasubstituted monofluoroalkenes and quaternary alkyl difluorides, Chem. Sci., 2019, 10, 9285–9291 RSC.
- W. Yu, Y. Ouyang, X. H. Xu and F. L. Qing, Visible Light-Induced Methoxycarbonyldifluoromethylation of Trimethylsilyl Enol Ethers and Allyltrimethylsilanes with FSO2CF2CO2Me, Chin. J. Chem., 2018, 36, 1024–1030 CrossRef CAS.
- H. Jiang, Y. Cheng, R. Wang, M. Zheng, Y. Zhang and S. Yu, Synthesis of 6-alkylated phenanthridine derivatives using photoredox neutral somophilic isocyanide insertion, Angew. Chem., Int. Ed., 2013, 52, 13289–13292 CrossRef CAS PubMed.
- Y. Yuan, W. Dong, X. Gao, X. Xie and Z. Zhang, Sodium Sulfite-Involved Photocatalytic Radical Cascade Cyclization of 2-Isocyanoaryl Thioethers: Access to 2-CF2/CF3-Containing Benzothiazoles, Org. Lett., 2019, 21, 469–472 CrossRef CAS PubMed.
- F. Ding, Y. Jiang, K. Lin and L. Shi, Tandem radical cyclization for the construction of 1-difluoroalkylated isoquinolines via Cu catalyzed and visible light-promoted pathways, Org. Biomol. Chem., 2018, 16, 1812–1815 RSC.
- X. X. Ma, S. Y. Mai, Y. Zhou, G. J. Cheng and Q. L. Song, Dual role of ethyl bromodifluoroacetate in the formation of fluorine-containing heteroaromatic compounds, Chem. Commun., 2018, 54, 8960–8963 RSC.
- X. X. Yu, Y. Zhou, X. X. Ma and Q. L. Song, Transition metal-free assembly of 1,3,5-triazines using ethyl bromodifluoroacetate as C1 source, Chem. Commun., 2019, 55, 8079–8082 RSC.
- A. Prieto, R. Melot, D. Bouyssi and N. Monteiro, C–H Difluoroalkylation of Aldehyde Hydrazones with Functionalized Difluoromethyl Bromides under Copper Catalysis, ACS Catal., 2016, 6, 1093–1096 CrossRef CAS.
- A. Prieto, R. Melot, D. Bouyssi and N. Monteiro, Palladium-Catalyzed C(sp2)–H Alkylation of Aldehyde-Derived Hydrazones with Functionalized Difluoromethyl Bromides, Angew. Chem., Int. Ed., 2016, 55, 1885–1889 CrossRef CAS PubMed.
- P. Xu, G. Wang, Y. Zhu, W. Li, Y. Cheng, S. Li and C. Zhu, Visible-Light Photoredox-Catalyzed C–H Difluoroalkylation of Hydrazones through an Aminyl Radical/Polar Mechanism, Angew. Chem., Int. Ed., 2016, 55, 2939–2943 CrossRef CAS PubMed.
- J. Xie, T. Zhang, F. Chen, N. Mehrkens, F. Rominger, M. Rudolph and A. S. Hashmi, Gold-Catalyzed Highly Selective Photoredox C(sp(2))-H Difluoroalkylation and Perfluoroalkylation of Hydrazones, Angew. Chem., Int. Ed., 2016, 55, 2934–2938 CrossRef CAS PubMed.
- M. Ke and Q. Song, Copper-Catalyzed C(sp(2))-H Difluoroalkylation of Aldehyde Derived Hydrazones with Diboron as Reductant, J. Org. Chem., 2016, 81, 3654–3664 CrossRef CAS PubMed.
- J. X. Li, L. Li, M. D. Zhou and H. Wang, Visible-light-promoted organic-dye-catalyzed three-component coupling of aldehydes, hydrazines and bromodifluorinated reagents, Org. Chem. Front., 2018, 5, 1003–1007 RSC.
- P. J. Xia, Z. P. Ye, D. Song, J. W. Ren, H. W. Wu, J. A. Xiao, H. Y. Xiang, X. Q. Chen and H. Yang, Photocatalytic reductive radical-radical coupling of N,N‘-cyclicazomethine imines with difluorobromo derivatives, Chem. Commun., 2019, 55, 2712–2715 RSC.
- Y. Zi, M. Lange and I. Vilotijevic, Enantioselective Lewis base catalyzed phosphonyldifluoromethylation of allylic fluorides using a C-silyl latent pronucleophile, Chem. Commun., 2020, 56, 5689–5692 RSC.
- G. K. S. Prakash and J. Hu, Selective Fluoroalkylations with Fluorinated Sulfones, Sulfoxides, and Sulfides, Acc. Chem. Res., 2007, 40, 921–930 CrossRef CAS PubMed.
- C. Ni, M. Hu and J. Hu, Good Partnership between Sulfur and Fluorine: Sulfur-Based Fluorination and Fluoroalkylation Reagents for Organic Synthesis, Chem. Rev., 2015, 115, 765–825 CrossRef CAS PubMed.
- J. Hu, Nucleophilic, radical, and electrophilic (phenylsulfonyl)difluoromethylations, J. Fluorine Chem., 2009, 130, 1130–1139 CrossRef CAS.
- T. Chatterjee, N. Iqbal, Y. You and E. J. Cho, Controlled Fluoroalkylation Reactions by Visible-Light Photoredox Catalysis, Acc. Chem. Res., 2016, 49, 2284–2294 CrossRef CAS PubMed.
- M. Pohmakotr, D. Soorukram, C. Kuhakarn and V. Reutrakul, Synthesis of gem-Difluoromethylenated Compounds Employing α,α-Difluoro-α-phenylsulfanyl-α-trimethylsilylmethane (PhSCF2SiMe3) as a gem-Difluoromethylene Building Block, Synlett, 2014, 2558–2573 CrossRef.
- Q. Xie, Z. Zhu, C. Ni and J. Hu, Nucleophilic (Phenylsulfonyl/arylthio)difluoromethylation of Aldehydes with TMSCF2Br: A Three-Component Strategy, Org. Lett., 2019, 21, 9138–9141 CrossRef CAS PubMed.
|
This journal is © the Partner Organisations 2020 |
Click here to see how this site uses Cookies. View our privacy policy here.