DOI:
10.1039/C6RA15945A
(Paper)
RSC Adv., 2016,
6, 70682-70690
Highly selective intramolecular addition of C–N and S–N bonds to alkynes catalyzed by palladium: a practical access to two distinct functional indoles†
Received
20th June 2016
, Accepted 18th July 2016
First published on 18th July 2016
Abstract
Palladium-catalyzed highly selective intramolecular addition of C–N and S–N bonds to alkynes has been achieved, and two distinct functional indoles were synthesized rapidly from the same set of substrates in a catalyst-controlled manner. This protocol features high atom economy, moderate to high yields and excellent selectivity, thus affording a practical approach to functional indoles.
Introduction
Diversity-oriented synthesis (DOS) represents rapid and convenient access to molecular complexity and diversity.1 It has received wide attention because of its high efficiency in constructing structurally complex and diverse molecules,2 which can improve the hit rates of drug screening.3 In particular, the intramolecular cyclization transformations of DOS substrates have become an important strategy to generate highly functionalized heterocyclic compounds.4 However, most of the reported cyclization processes are defined single chemical reactions, thus generally leading to single heterocyclic products. This is in part attributed to the challenge of achieving high levels of chemoselectivity.5 Therefore, the power of DOS would be reinforced if the same materials could be diverged to different heterocyclic products. It is fascinating but also challenging to synthesize distinct sets of heterocyclic compounds from the same set of substrates in a highly selective manner, providing greater flexibility and efficiency.6 In our ongoing efforts to develop novel convenient and efficient approaches to synthesize valuable heterocyclic compounds, we herein report the rapid and highly selective synthesis of two distinct functional indoles from the same set of substrates in a catalyst-controlled manner.
Transition metal-catalyzed addition of X–Y (X, Y = H, B, C, N, O, Si, S, Cl, Se, etc.) bonds to alkynes forms an important strategy for the functionalization of carbon–carbon triple bonds.7–12 Particularly, the intramolecular addition of X–Y bonds to alkynes has become an efficient strategy to synthesize functional cyclic compounds such as indoles,8 indenes,9 indenones,10 benzofurans,11 benzothiophenes,12 benzosiloles,13 oxindoles,14 isoxazoles,15 furans,16 pyrroles,17 pyrrolidinones,14a,b azetidin-2-ones,18 piperidin-2-ones,14b,c,18 azepan-2-ones14b,18 and 1,2-oxaborolanes.19 Despite the remarkable achievements made, however, among the most reported methods, one specific metal only catalyzed the intramolecular addition of one specific kind of X–Y bond to alkynes with the migration of one specific kind of functional group. Based on our previous research,8c,d in the present work, we realized the highly selective intramolecular addition of C–N and S–N bonds to alkynes using the same metal palladium with different oxidation states, and the allyl group and sulfonyl group20 can display as migrating groups when employing Pd(0) and Pd(II) catalysts, respectively (Scheme 1). In our catalytic system, two distinct kinds of functional indoles were synthesized rapidly and selectively from the same set of substrates in a catalyst-controlled manner. To the best of our knowledge, this is the first report of selective intramolecular addition of different X–Y bonds to alkynes catalyzed by the same metal to furnish distinct heterocyclic compounds from the same substrates.
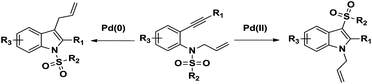 |
| Scheme 1 Selective intramolecular addition of C–N and S–N bonds to alkynes catalyzed by palladium. | |
Results and discussion
Screening experiments were performed employing 1a as the model substrate to optimize the intramolecular addition conditions for catalysts and solvents (Table 1). Initially, substrate 1a was subjected to Pd(0) catalysts such as Pd2(dba)3 and Pd(PPh3)4 in toluene at 90 °C for 8 h (entries 1 and 2). To our delight, the C–N addition product 2a was achieved when Pd(PPh3)4 was used as the catalyst, albeit with low yield (entry 2). An examination of the solvents revealed that the transformation was significantly influenced by the solvent used. Moderate yields (56–78%) were obtained in THF, DCE, DMF and DMSO (entries 3, 4, 8 and 9), a high yield (83%) was achieved in 1,4-dioxane (entry 5), and no desired product was detected in EtOH (entry 7). Pleasingly, 2a was exclusively afforded in CH3CN with almost quantitative yield (entry 6). Subsequently, optimization of the reaction conditions of Pd(II) catalyzed intramolecular addition of S–N bond to alkynes was carried out. Treatment of 1a with Pd(II) catalysts such as Pd(OAc)2 in CH3CN at 90 °C for 8 h provided the S–N addition product 3a in 16% yield (entry 10). Encouraged by this result, an investigation of other Pd(II) sources was conducted (entries 11–16). Among them, PdCl2(CH3CN)2 displayed the highest catalytic reactivity, providing product 3a with 92% yield (entry 16). In addition, screening of solvents revealed that CH3CN was the most suitable solvent for this reaction (entries 16–20). In this way, two distinct functional indoles were generated rapidly from the same set of substrates in a catalyst-controlled manner with high selectivity.
Table 1 Survey of the reaction conditions for the divergent transformations of 1a
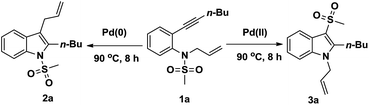
|
Entry |
Catalyst |
Solvent |
Yielda (%) |
2ab |
3ac |
Isolated yield. 1a (0.4 mmol), Pd(0) (0.04 mmol), and solvent (2.0 ml) under an argon atmosphere at 90 °C for 8 h. 1a (0.4 mmol), Pd(II) (0.04 mmol), and solvent (2.0 ml) under an argon atmosphere at 90 °C for 8 h. “—” means that product was not detected. |
1 |
Pd2(dba)3 |
Toluene |
Trace |
— |
2 |
Pd(PPh3)4 |
Toluene |
5 |
— |
3 |
Pd(PPh3)4 |
THF |
56 |
— |
4 |
Pd(PPh3)4 |
DCE |
62 |
— |
5 |
Pd(PPh3)4 |
1,4-Dioxane |
83 |
— |
6 |
Pd(PPh3)4 |
CH3CN |
99 |
— |
7 |
Pd(PPh3)4 |
EtOH |
0 |
— |
8 |
Pd(PPh3)4 |
DMF |
78 |
— |
9 |
Pd(PPh3)4 |
DMSO |
56 |
— |
10 |
Pd(OAc)2 |
CH3CN |
— |
16 |
11 |
PdCl2 |
CH3CN |
— |
47 |
12 |
PdCl2(dppf)2 |
CH3CN |
— |
0 |
13 |
(NH4)2PdCl4 |
CH3CN |
— |
11 |
14 |
Na2PdCl4 |
CH3CN |
— |
59 |
15 |
PdCl2(PhCN)2 |
CH3CN |
— |
84 |
16 |
PdCl2(CH3CN)2 |
CH3CN |
— |
92 |
17 |
PdCl2(CH3CN)2 |
Toluene |
— |
81 |
18 |
PdCl2(CH3CN)2 |
DCE |
— |
77 |
19 |
PdCl2(CH3CN)2 |
EtOH |
— |
0 |
20 |
PdCl2(CH3CN)2 |
DMSO |
— |
6 |
These selective conditions were then applied to synthesize a wide range of 3-allyl-1-sulfonyl-indoles (2) and 1-allyl-3-sulfonyl-indoles (3) from the same set of substrates (1). The results are summarized in Table 2. In general, the protocol was compatible with aliphatic and aromatic substituents at R1 and R2, as well as halogens, electron-donating and electron-withdrawing groups at R3, and all the indole products were obtained with high yields in a highly selective manner. In the case of Pd(PPh3)4 catalyzed intramolecular addition of C–N bond to alkynes, the protocol showed high yields (85–99%) for substrates with aliphatic and aromatic substituents at R1 (2a–2d). Pleasingly, the reactions of 1e–1f carrying functional groups such as ester and halogen at R1 afforded the corresponding products 2e–2f in excellent yields (90–92%). High yields (88–93%) were also achieved from 1g–1i with aliphatic and aromatic substituents at R2 (2g–2i). In addition, substrates bearing halogens (F, Cl, Br), electron-donating group (Me) and electron-withdrawing substituents (CN, CF3) at R3 furnished the corresponding products 2j–2o in high yields (86–92%). Similarly, for the PdCl2(CH3CN)2 catalyzed intramolecular addition of S–N bond to alkynes, the approach was compatible with aliphatic and aromatic substituents at R1 and R2 with moderate to high yields (3a–3i). The reactions of substrates having halogens (F, Cl, Br), electron-donating group (Me) and electron-withdrawing substituents (CN, CF3) at R3 also achieved the desired products 3j–3o in 77–87% yields.
Table 2 Divergent synthesis of two distinct indole compounds 2 and 3
Entry |
Yielda (%) |
2b |
3c |
Isolated yield. 1 (0.4 mmol), Pd(PPh3)4 (0.04 mmol), and CH3CN (2.0 mL) under an argon atmosphere at 90 °C for 8 h. 1 (0.4 mmol), PdCl2(CH3CN)2 (0.04 mmol), and CH3CN (2.0 mL) under an argon atmosphere at 90 °C for 8 h. |
1 |
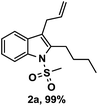 |
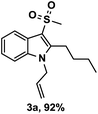 |
2 |
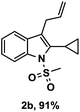 |
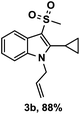 |
3 |
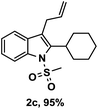 |
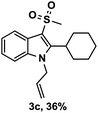 |
4 |
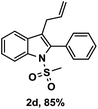 |
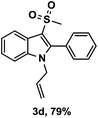 |
5 |
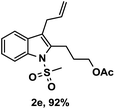 |
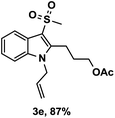 |
6 |
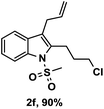 |
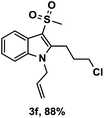 |
7 |
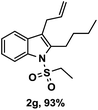 |
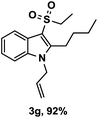 |
8 |
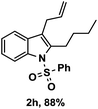 |
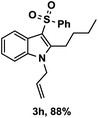 |
9 |
 |
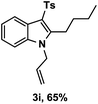 |
10 |
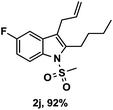 |
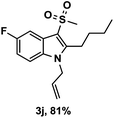 |
11 |
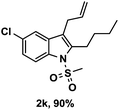 |
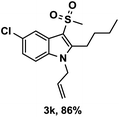 |
12 |
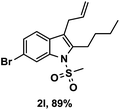 |
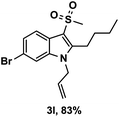 |
13 |
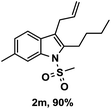 |
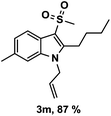 |
14 |
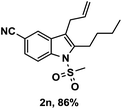 |
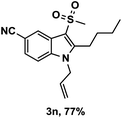 |
15 |
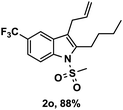 |
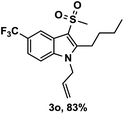 |
Thus, from the same set of substrates, two distinct functional indoles were prepared easily by simply switching the palladium catalyst. Our protocol exhibits valuable features of high selectivity, moderate to high yields and high atom economy. Such a divergent synthesis of functional indoles is evidently useful in diversity-oriented synthesis.
The chemical structures of compounds 2 and 3 were characterized and differentiated by comparing their 1H NMR spectra. The allyl hydrogens of compounds 2 generally display much lower chemical shifts than the allyl hydrogens of compounds 3. For example, the chemical shift of the allyl hydrogens (Ha) of compounds 2a is 3.44 ppm, while the chemical shift of the allyl hydrogens (Hb) of compounds 3a is 4.77 ppm (Fig. 1).
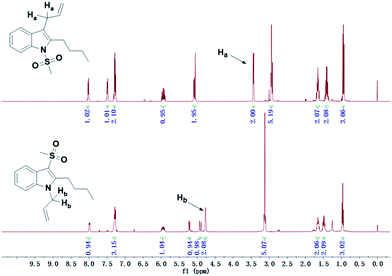 |
| Fig. 1 Structural characterization of compounds 2a and 3a. | |
The proposed catalytic cycle of Pd(0)-catalyzed intramolecular addition of C–N bond to alkynes was outlined in Scheme 2. Oxidative addition of the allyl amine group to the Pd(0) catalyst forms intermediate A1, then palladium-promoted ionization of the C–N bond offers intermediate B1, followed by nucleophilic attack of the nitrogen to the activated carbon–carbon triple bond, producing intermediate C1. Reductive elimination of the Pd(0) catalyst then gives the products.
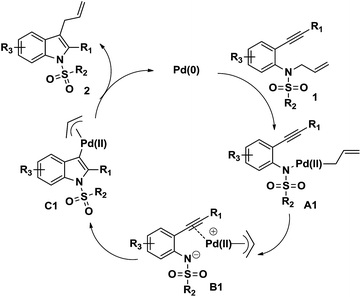 |
| Scheme 2 Proposed reaction mechanism of Pd(0)-catalyzed intramolecular addition of C–N bond to alkynes. | |
A plausible mechanism of Pd(II)-catalyzed intramolecular addition of S–N bond to alkynes was also proposed as shown in Scheme 3. Coordination of alkyne to the Pd(II) catalyst yields intermediate A2, followed by nucleophilic attack of nitrogen to the alkyne, affording intermediate B2. An intramolecular [1,3]-migration of the sulfonyl group then gives intermediate C2, which affords the products and regenerates the catalyst.
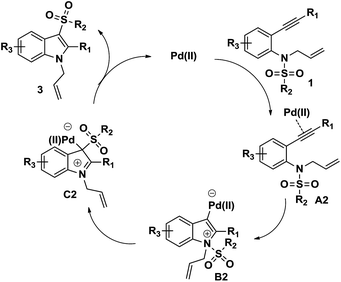 |
| Scheme 3 Proposed reaction mechanism Pd(II)-catalyzed intramolecular addition of S–N bond to alkynes. | |
Conclusions
We have developed a practical and efficient approach to synthesize two distinct functional indoles through the highly selective intramolecular addition of C–N and S–N bonds to alkynes catalyzed by palladium. The catalyst-controlled synthetic strategy features high atom economy, moderate to high yields and excellent selectivity. We anticipate that these functional indoles may find pharmaceutical applications after further investigations.
Experimental section
General information
The reagents were purchased from commercial suppliers and used without further purification. Analytical thin-layer chromatography (TLC) was performed on HSGF 254 (0.15–0.2 mm thickness), visualized by irradiation with UV light (254 nm). Column chromatography was performed using silica gel FCP 200-300. Melting points were measured with a micro melting point apparatus. Nuclear magnetic resonance spectra were recorded on a Brucker AMX-400 MHz instrument (TMS as IS). Chemical shifts were reported in parts per million (ppm, δ) downfield from tetramethylsilane. Proton coupling patterns were described as singlet (s), doublet (d), triplet (t), quartet (q), multiplet (m), and broad (br). Low and high-resolution mass were measured by the EI method with a Tsou-EI mass spectrometer.
General procedure for the preparation of 2
A long reaction tube (about fifteen centimeter length) equipped with a magnetic stir bar was charged with 1 (0.4 mmol), Pd(PPh3)4 (0.04 mmol), and CH3CN (2.0 ml) and capped with septa. Then the reaction tube was evacuated and backfilled with argon, and the process was repeated three times. After that, the reaction tube was kept in the pre-heated oil bath at 90 °C and stirred under reflux for 8 h. After removal of the solvent, the residue was purified by flash chromatography on silica gel (PE
:
EA = 16
:
1) to give the desired product 2.
General procedure for the preparation of 3
A long reaction tube (about fifteen centimeter length) equipped with a magnetic stir bar was charged with 1 (0.4 mmol), PdCl2(CH3CN)2 (0.04 mmol), and CH3CN (2.0 mL) and capped with septa. Then the reaction tube was evacuated and backfilled with argon, and the process was repeated three times. After that, the reaction tube was kept in the pre-heated oil bath at 90 °C and stirred under reflux for 8 h. After removal of the solvent, the residue was purified by flash chromatography on silica gel (PE
:
EA = 8
:
1) to give the desired product 3.
Characterization data
3-Allyl-2-butyl-1-(methylsulfonyl)-1H-indole (2a). White solid (115.4 mg, yield 99%), mp 42–43 °C. 1H NMR (400 MHz, CDCl3) δ 8.07–7.99 (m, 1H), 7.52–7.46 (m, 1H), 7.33–7.26 (m, 2H), 6.00–5.88 (m, 1H), 5.12–5.03 (m, 2H), 3.44 (d, J = 6.0 Hz, 2H), 3.00–2.90 (m, 5H), 1.70–1.61 (m, 2H), 1.46–1.36 (m, 2H), 0.94 (t, J = 7.3 Hz, 3H); 13C NMR (126 MHz, CDCl3) δ 138.56, 136.48, 135.73, 130.84, 124.35, 123.70, 119.13, 118.27, 115.90, 114.65, 39.96, 32.95, 28.71, 26.01, 22.85, 13.97; EI-MS (m/z): 291 (M+); HRMS (EI) calcd for C16H21NO2S (M+) 291.1293, found: 291.1296.
1-Allyl-2-butyl-3-(methylsulfonyl)-1H-indole (3a). Yellow solid (107.2 mg, yield 92%), mp 65–66 °C. 1H NMR (400 MHz, CDCl3) δ 8.06–7.95 (m, 1H), 7.34–7.24 (m, 3H), 6.02–5.89 (m, 1H), 5.22 (d, J = 10.6 Hz, 1H), 4.91 (d, J = 17.0 Hz, 1H), 4.77 (d, J = 3.8 Hz, 2H), 3.17–3.08 (m, 5H), 1.71–1.60 (m, 2H), 1.54–1.45 (m, 2H), 0.96 (t, J = 7.2 Hz, 3H); 13C NMR (126 MHz, CDCl3) δ 146.26, 135.86, 132.00, 125.19, 123.19, 122.47, 119.71, 117.64, 110.39, 45.97, 45.81, 32.54, 24.47, 22.97, 13.89; EI-MS (m/z): 291 (M+); HRMS (EI) calcd for C16H21NO2S (M+) 291.1293, found: 291.1292.
3-Allyl-2-cyclopropyl-1-(methylsulfonyl)-1H-indole (2b). Pale yellow solid (100.2 mg, yield 91%), mp 84–85 °C. 1H NMR (400 MHz, CDCl3) δ 7.99 (d, J = 8.0 Hz, 1H), 7.49–7.43 (m, 1H), 7.32–7.24 (m, 2H), 6.04–5.92 (m, 1H), 5.06 (dd, J = 10.1, 1.6 Hz, 1H), 4.97 (dd, J = 17.1, 1.7 Hz, 1H), 3.56 (d, J = 5.6 Hz, 2H), 3.08 (s, 3H), 2.10–2.01 (m, 1H), 1.11–1.05 (m, 2H), 0.83–0.77 (m, 2H); 13C NMR (101 MHz, CDCl3) δ 138.33, 136.28, 135.79, 130.54, 124.68, 123.43, 119.41, 119.12, 115.91, 114.30, 40.62, 28.87, 8.51, 7.58; EI-MS (m/z): 275 (M+); HRMS (EI) calcd for C15H17NO2S (M+) 275.0980, found: 275.0978.
1-Allyl-2-cyclopropyl-3-(methylsulfonyl)-1H-indole (3b). Brown oil (96.9 mg, yield 88%). 1H NMR (400 MHz, CDCl3) δ 8.12–8.03 (m, 1H), 7.34–7.22 (m, 3H), 6.05–5.91 (m, 1H), 5.27–5.18 (m, 1H), 5.02–4.97 (m, 2H), 4.94–4.88 (m, 1H), 3.19 (s, 3H), 2.03–1.95 (m, 1H), 1.30–1.21 (m, 2H), 1.07–1.00 (m, 2H); 13C NMR (101 MHz, CDCl3) δ 144.59, 135.38, 132.24, 125.15, 123.40, 122.44, 120.08, 117.29, 113.09, 110.45, 46.41, 45.69, 7.74, 6.69; EI-MS (m/z): 275 (M+); HRMS (EI) calcd for C15H17NO2S (M+) 275.0980, found: 275.0984.
3-Allyl-2-cyclohexyl-1-(methylsulfonyl)-1H-indole (2c). Pale yellow solid (120.6 mg, yield 95%), mp 72–73 °C. 1H NMR (400 MHz, CDCl3) δ 8.09–8.04 (m, 1H), 7.49–7.44 (m, 1H), 7.29–7.25 (m, 2H), 6.07–5.92 (m, 1H), 5.16–5.01 (m, 2H), 3.69–3.55 (m, 3H), 2.97 (s, 3H), 1.94–1.77 (m, 6H), 1.50–1.27 (m, 4H); 13C NMR (101 MHz, CDCl3) δ 142.13, 136.38, 135.84, 131.46, 124.33, 123.53, 118.91, 118.39, 116.11, 115.13, 40.38, 36.92, 32.39, 29.40, 27.16, 26.03; EI-MS (m/z): 317 (M+); HRMS (EI) calcd for C18H23NO2S (M+) 317.1449, found: 317.1444.
1-Allyl-2-cyclohexyl-3-(methylsulfonyl)-1H-indole (3c). Brown solid (45.7 mg, yield 36%), mp 62–63 °C. 1H NMR (400 MHz, CDCl3) δ 7.71 (d, J = 7.6 Hz, 1H), 7.49 (d, J = 8.2 Hz, 1H), 7.25–7.20 (m, 1H), 6.73 (s, 1H), 6.02–5.88 (m, 1H), 5.16 (d, J = 10.5 Hz, 1H), 4.86–4.75 (m, 3H), 3.13 (s, 3H), 2.70–2.61 (m, 1H), 2.04–1.98 (m, 2H), 1.91–1.86 (m, 2H), 1.83–1.75 (m, 2H), 1.60–1.51 (m, 2H), 1.45–1.36 (m, 2H); 13C NMR (126 MHz, CDCl3) δ 150.29, 137.73, 133.06, 129.63, 125.19, 120.68, 119.93, 116.92, 115.15, 96.72, 45.64, 43.89, 36.22, 33.60, 26.70, 26.13; EI-MS (m/z): 317 (M+); HRMS (EI) calcd for C18H23NO2S (M+) 317.1449, found: 317.1452.
3-Allyl-1-(methylsulfonyl)-2-phenyl-1H-indole (2d). White solid (105.9 mg, yield 85%), mp 128–130 °C. 1H NMR (400 MHz, CDCl3) δ 8.16 (dd, J = 7.2, 1.3 Hz, 1H), 7.63–7.60 (m, 1H), 7.49–7.35 (m, 7H), 6.03–5.91 (m, 1H), 5.11–5.01 (m, 2H), 3.36–3.31 (m, 2H), 2.79 (s, 3H); 13C NMR (101 MHz, CDCl3) δ 137.31, 137.10, 135.90, 131.09, 130.91, 130.75, 128.92, 127.86, 125.29, 124.21, 121.13, 119.93, 115.98, 115.56, 39.85, 28.88; EI-MS (m/z): 311 (M+); HRMS (EI) calcd for C18H17NO2S (M+) 311.0980, found: 311.0978.
1-Allyl-3-(methylsulfonyl)-2-phenyl-1H-indole (3d). Pale yellow solid (98.4 mg, yield 79%), mp 122–123 °C. 1H NMR (400 MHz, CDCl3) δ 8.22–8.18 (m, 1H), 7.54–7.45 (m, 5H), 7.41–7.34 (m, 3H), 5.90–5.79 (m, 1H), 5.19 (dd, J = 10.4, 0.8 Hz, 1H), 4.90 (dd, J = 17.1, 0.8 Hz, 1H), 4.57–4.54 (m, 2H), 2.95 (s, 3H); 13C NMR (101 MHz, CDCl3) δ 144.05, 135.57, 132.26, 130.72, 130.13, 128.92, 128.36, 125.12, 123.89, 122.83, 120.61, 117.73, 113.03, 110.97, 46.71, 45.83; EI-MS (m/z): 311 (M+); HRMS (EI) calcd for C18H17NO2S (M+) 311.0980, found: 311.0986.
3-(3-Allyl-1-(methylsulfonyl)-1H-indol-2-yl)propyl acetate (2e). Colourless oil (123.4 mg, yield 92%). 1H NMR (400 MHz, CDCl3) δ 8.03–7.98 (m, 1H), 7.52–7.47 (m, 1H), 7.32–7.25 (m, 2H), 5.99–5.86 (m, 1H), 5.11–5.01 (m, 2H), 4.10 (t, J = 6.3 Hz, 2H), 3.46–3.42 (m, 2H), 3.06–3.00 (m, 2H), 2.93 (s, 3H), 2.06–1.99 (m, 5H); 13C NMR (101 MHz, CDCl3) δ 171.12, 136.71, 136.44, 135.39, 130.55, 124.59, 123.75, 119.22, 119.01, 115.97, 114.52, 63.75, 39.83, 29.37, 28.49, 22.78, 21.00; EI-MS (m/z): 335 (M+); HRMS (EI) calcd for C17H21NO4S (M+) 335.1191, found: 335.1196.
3-(1-Allyl-3-(methylsulfonyl)-1H-indol-2-yl)propyl acetate (3e). Yellow oil (116.7 mg, yield 87%). 1H NMR (400 MHz, CDCl3) δ 7.97 (dd, J = 6.9, 2.2 Hz, 1H), 7.34–7.25 (m, 3H), 6.01–5.88 (m, 1H), 5.23 (d, J = 10.4 Hz, 1H), 4.92 (d, J = 17.1 Hz, 1H), 4.82–4.74 (m, 2H), 4.18 (t, J = 6.2 Hz, 2H), 3.23–3.17 (m, 2H), 3.12 (s, 3H), 2.07–1.99 (m, 5H); 13C NMR (101 MHz, CDCl3) δ 171.13, 144.65, 135.84, 131.82, 124.96, 123.42, 122.58, 119.61, 117.73, 110.74, 110.43, 63.71, 45.83, 45.73, 29.31, 21.39, 21.01; EI-MS (m/z): 335 (M+); HRMS (EI) calcd for C17H21NO4S (M+) 335.1191, found: 335.1188.
3-Allyl-2-(3-chloropropyl)-1-(methylsulfonyl)-1H-indole (2f). White solid (112.3 mg, yield 90%), mp 52–53 °C. 1H NMR (400 MHz, CDCl3) δ 8.06–8.00 (m, 1H), 7.55–7.51 (m, 1H), 7.35–7.27 (m, 2H), 6.02–5.91 (m, 1H), 5.14–5.06 (m, 2H), 3.59 (t, J = 6.2 Hz, 2H), 3.50 (d, J = 6.0 Hz, 2H), 3.16–3.09 (m, 2H), 2.94 (s, 3H), 2.24–2.13 (m, 2H); 13C NMR (101 MHz, CDCl3) δ 136.57, 136.33, 135.50, 130.65, 124.75, 123.90, 119.59, 119.37, 116.06, 114.65, 44.69, 39.85, 33.14, 28.57, 23.59; EI-MS (m/z): 313 (M+, (Cl37)), 311 (M+, (Cl35)); HRMS (EI) calcd for C15H18ClNO2S (M+) 311.0747, found: 311.0744.
1-Allyl-2-(3-chloropropyl)-3-(methylsulfonyl)-1H-indole (3f). Pale yellow solid (109.8 mg, yield 88%), mp 60–62 °C. 1H NMR (400 MHz, CDCl3) δ 8.05–7.94 (m, 1H), 7.40–7.23 (m, 3H), 6.09–5.90 (m, 1H), 5.26 (d, J = 10.4 Hz, 1H), 4.96 (d, J = 17.1 Hz, 1H), 4.90–4.78 (m, 2H), 3.70 (t, J = 6.0 Hz, 2H), 3.36–3.25 (m, 2H), 3.15 (s, 3H), 2.24–2.11 (m, 2H); 13C NMR (126 MHz, CDCl3) δ 144.35, 135.87, 131.86, 124.96, 123.50, 122.64, 119.62, 117.83, 110.93, 110.50, 45.89, 45.77, 44.76, 33.18, 22.40; EI-MS (m/z): 313 (M+, (Cl37)), 311 (M+, (Cl35)); HRMS (EI) calcd for C15H18ClNO2S (M+) 311.0747, found: 311.0746.
3-Allyl-2-butyl-1-(ethylsulfonyl)-1H-indole (2g). Colourless oil (113.6 mg, yield 93%). 1H NMR (400 MHz, CDCl3) δ 8.04–7.98 (m, 1H), 7.51–7.45 (m, 1H), 7.29–7.24 (m, 2H), 6.02–5.88 (m, 1H), 5.12–5.01 (m, 2H), 3.44 (d, J = 5.9 Hz, 2H), 3.17 (q, J = 7.4 Hz, 2H), 2.95–2.91 (m, 2H), 1.72–1.63 (m, 2H), 1.44–1.37 (m, 2H), 1.16 (t, J = 7.4 Hz, 3H), 0.94 (t, J = 7.4 Hz, 3H); 13C NMR (126 MHz, CDCl3) δ 139.21, 136.59, 135.79, 130.53, 124.12, 123.43, 119.06, 117.37, 115.76, 114.56, 48.20, 33.17, 28.60, 26.12, 22.85, 13.96, 7.84; EI-MS (m/z): 305 (M+); HRMS (EI) calcd for C17H23NO2S (M+) 305.1449, found: 305.1448.
1-Allyl-2-butyl-3-(ethylsulfonyl)-1H-indole (3g). Brown oil (112.4 mg, yield 92%). 1H NMR (400 MHz, CDCl3) δ 8.02–7.92 (m, 1H), 7.32–7.21 (m, 3H), 6.01–5.87 (m, 1H), 5.20 (d, J = 10.4 Hz, 1H), 4.86 (d, J = 17.1 Hz, 1H), 4.79–4.74 (m, 2H), 3.18 (q, J = 7.4 Hz, 2H), 3.12–3.03 (m, 2H), 1.69–1.56 (m, 2H), 1.52–1.45 (m, 2H), 1.27 (t, J = 7.4 Hz, 3H), 0.96 (t, J = 7.3 Hz, 3H); 13C NMR (126 MHz, CDCl3) δ 147.11, 135.88, 131.99, 125.55, 123.08, 122.34, 119.87, 117.39, 110.33, 107.91, 51.71, 45.74, 32.73, 24.59, 22.98, 13.87, 7.82; EI-MS (m/z): 305 (M+); HRMS (EI) calcd for C17H23NO2S (M+) 305.1449, found: 305.1452.
3-Allyl-2-butyl-1-(phenylsulfonyl)-1H-indole (2h). Colourless oil (124.4 mg, yield 88%). 1H NMR (400 MHz, CDCl3) δ 8.15 (d, J = 8.2 Hz, 1H), 7.62 (dd, J = 8.3, 1.0 Hz, 2H), 7.45–7.38 (m, 1H), 7.35–7.26 (m, 3H), 7.23–7.14 (m, 2H), 5.89–5.76 (m, 1H), 4.93 (dd, J = 10.1, 1.4 Hz, 1H), 4.82 (dd, J = 17.1, 1.6 Hz, 1H), 3.36–3.27 (m, 2H), 2.92 (t, J = 7.8 Hz, 2H), 1.68–1.59 (m, 2H), 1.41–1.33 (m, 2H), 0.90 (t, J = 7.3 Hz, 3H); 13C NMR (126 MHz, CDCl3) δ 138.96, 138.65, 136.94, 135.57, 133.50, 130.94, 129.12, 126.22, 124.18, 123.62, 118.89, 118.79, 115.63, 115.40, 33.04, 28.50, 26.37, 22.86, 13.96; EI-MS (m/z): 353 (M+); HRMS (EI) calcd for C21H23NO2S (M+) 353.1449, found: 353.1445.
1-Allyl-2-butyl-3-(phenylsulfonyl)-1H-indole (3h). Brown solid (124.4 mg, yield 88%), mp 63–64 °C. 1H NMR (400 MHz, CDCl3) δ 8.04–7.86 (m, 3H), 7.61–7.52 (m, 2H), 7.52–7.35 (m, 3H), 6.34 (d, J = 0.8 Hz, 1H), 5.99–5.86 (m, 1H), 5.14 (dd, J = 10.3, 0.9 Hz, 1H), 4.83–4.73 (m, 3H), 2.71 (t, J = 7.8 Hz, 2H), 1.75–1.67 (m, 2H), 1.48–1.40 (m, 2H), 0.96 (t, J = 7.4 Hz, 3H); 13C NMR (126 MHz, CDCl3) δ 146.27, 143.17, 135.78, 132.76, 132.63, 132.57, 131.83, 129.15, 127.38, 120.45, 118.69, 116.83, 109.87, 100.09, 45.58, 30.45, 26.52, 22.60, 13.99; EI-MS (m/z): 353 (M+); HRMS (EI) calcd for C21H23NO2S (M+) 353.1449, found: 353.1447.
3-Allyl-2-butyl-1-tosyl-1H-indole (2i). Colourless oil (130.8 mg, yield 89%). 1H NMR (400 MHz, CDCl3) δ 8.28–8.15 (m, 1H), 7.59 (dd, J = 8.4, 1.6 Hz, 2H), 7.43–7.37 (m, 1H), 7.30–7.20 (m, 2H), 7.14 (d, J = 8.5 Hz, 2H), 5.97–5.84 (m, 1H), 5.09–4.84 (m, 2H), 3.48–3.33 (m, 2H), 3.05–2.96 (m, 2H), 2.31 (s, 3H), 1.75–1.67 (m, 2H), 1.49–1.40 (m, 2H), 0.97 (t, J = 7.3 Hz, 3H); 13C NMR (101 MHz, CDCl3) δ 144.47, 138.63, 136.87, 136.05, 135.62, 130.89, 129.69, 126.23, 124.05, 123.47, 118.81, 118.55, 115.57, 115.35, 33.06, 28.50, 26.36, 22.85, 21.54, 13.95; EI-MS (m/z): 367 (M+); HRMS (EI) calcd for C22H25NO2S (M+) 367.1606, found: 367.1608.
1-Allyl-2-butyl-3-tosyl-1H-indole (3i). Brown solid (95.6 mg, yield 65%), mp 105–106 °C. 1H NMR (400 MHz, CDCl3) δ 8.15–8.09 (m, 1H), 7.86 (d, J = 8.2 Hz, 2H), 7.26–7.19 (m, 5H), 6.02–5.81 (m, 1H), 5.18 (d, J = 10.4 Hz, 1H), 4.86 (d, J = 17.2 Hz, 1H), 4.72 (d, J = 4.5 Hz, 2H), 3.11–3.15 (m, 2H), 2.35 (s, 3H), 1.59–1.44 (m, 4H), 0.95 (t, J = 7.1 Hz, 3H); 13C NMR (126 MHz, CDCl3) δ 146.23, 143.06, 141.79, 135.83, 131.99, 129.59, 126.30, 125.31, 122.99, 122.37, 120.14, 117.51, 111.20, 110.24, 45.80, 32.17, 24.81, 23.10, 21.57, 13.87; EI-MS (m/z): 367 (M+); HRMS (EI) calcd for C22H25NO2S (M+) 367.1606, found: 367.1601.
3-Allyl-2-butyl-5-fluoro-1-(methylsulfonyl)-1H-indole (2j). Colourless oil (113.9 mg, yield 92%). 1H NMR (400 MHz, CDCl3) δ 7.95 (dd, J = 9.1, 4.5 Hz, 1H), 7.16–7.10 (m, 1H), 7.02–6.96 (m, 1H), 5.96–5.85 (m, 1H), 5.11–5.03 (m, 2H), 3.42–3.37 (m, 2H), 2.95–2.89 (m, 5H), 1.67–1.61 (m, 2H), 1.44–1.37 (m, 2H), 0.94 (t, J = 7.3 Hz, 3H); 13C NMR (126 MHz, CDCl3) δ 160.03 (d, JC–F = 240.6 Hz), 140.35, 135.26, 132.64, 132.06 (d, JC–F = 9.6 Hz), 118.16 (d, JC–F = 3.8 Hz), 116.19, 115.74 (d, JC–F = 9.2 Hz), 111.90 (d, JC–F = 25.0 Hz), 104.90 (d, JC–F = 23.9 Hz), 39.99, 32.87, 28.67, 26.12, 22.83, 13.92; EI-MS (m/z): 309 (M+); HRMS (EI) calcd for C16H20FNO2S (M+) 309.1199, found: 309.1197.
1-Allyl-2-butyl-5-fluoro-3-(methylsulfonyl)-1H-indole (3j). Pale yellow solid (100.2 mg, yield 81%), mp 81–82 °C. 1H NMR (400 MHz, CDCl3) δ 7.69–7.62 (m, 1H), 7.21 (dd, J = 9.0, 4.3 Hz, 1H), 7.02–6.95 (m, 1H), 5.98–5.87 (m, 1H), 5.22 (dd, J = 10.4, 0.7 Hz, 1H), 4.89 (dd, J = 17.1, 0.7 Hz, 1H), 4.77–4.73 (m, 2H), 3.11–3.06 (m, 5H), 1.67–1.60 (m, 2H), 1.50–1.43 (m, 2H), 0.96 (t, J = 7.3 Hz, 3H); 13C NMR (126 MHz, CDCl3) δ 159.34 (d, JC–F = 238.4 Hz), 147.57, 132.26, 131.77, 125.79 (d, JC–F = 11.0 Hz), 117.76, 111.54 (d, JC–F = 26.2 Hz), 111.35 (d, JC–F = 9.6 Hz), 110.48 (d, JC–F = 4.1 Hz), 105.26 (d, JC–F = 25.9 Hz), 46.02, 45.91, 32.39, 24.64, 22.94, 13.84; EI-MS (m/z): 309 (M+); HRMS (EI) calcd for C16H20FNO2S (M+) 309.1199, found: 309.1203.
3-Allyl-2-butyl-5-chloro-1-(methylsulfonyl)-1H-indole (2k). Yellow oil (117.3 mg, yield 90%). 1H NMR (400 MHz, CDCl3) δ 7.95–7.90 (m, 1H), 7.46–7.43 (m, 1H), 7.23 (dd, J = 8.9, 2.1 Hz, 1H), 5.97–5.83 (m, 1H), 5.16–4.94 (m, 2H), 3.48–3.30 (m, 2H), 2.96–2.87 (m, 5H), 1.67–1.60 (m, 2H), 1.45–1.36 (m, 2H), 0.94 (t, J = 7.4 Hz, 3H); 13C NMR (101 MHz, CDCl3) δ 140.05, 135.22, 134.73, 132.12, 129.54, 124.36, 118.81, 117.63, 116.24, 115.66, 40.21, 32.84, 28.53, 26.03, 22.82, 13.92; EI-MS (m/z): 327 (M+, (Cl37)), 325 (M+, (Cl35)); HRMS (EI) calcd for C16H20ClNO2S (M+) 325.0903, found: 325.0905.
1-Allyl-2-butyl-5-chloro-3-(methylsulfonyl)-1H-indole (3k). Pale yellow solid (112.1 mg, yield 86%), mp 93–94 °C. 1H NMR (400 MHz, CDCl3) δ 7.99 (dd, J = 1.6, 0.9 Hz, 1H), 7.24–7.19 (m, 2H), 5.97–5.88 (m, 1H), 5.23 (d, J = 10.9 Hz, 1H), 4.89 (d, J = 16.7 Hz, 1H), 4.76–4.73 (m, 2H), 3.11–3.08 (m, 5H), 1.68–1.63 (m, 2H), 1.52–1.44 (m, 2H), 0.96 (t, J = 7.3 Hz, 3H); 13C NMR (101 MHz, CDCl3) δ 147.44, 134.25, 131.65, 128.49, 126.18, 123.66, 119.33, 117.86, 111.48, 110.26, 46.04, 45.98, 32.43, 24.60, 22.98, 13.88; EI-MS (m/z): 327 (M+, (Cl37)), 325 (M+, (Cl35)); HRMS (EI) calcd for C16H20ClNO2S (M+) 325.0903, found: 325.0907.
3-Allyl-6-bromo-2-butyl-1-(methylsulfonyl)-1H-indole (2l). Gray solid (131.8 mg, yield 89%), mp 62–63 °C. 1H NMR (400 MHz, CDCl3) δ 7.89 (d, J = 8.9 Hz, 1H), 7.59 (d, J = 2.0 Hz, 1H), 7.37 (dd, J = 8.8, 2.0 Hz, 1H), 5.97–5.85 (m, 1H), 5.11–5.01 (m, 2H), 3.41–3.37 (m, 2H), 2.95–2.89 (m, 5H), 1.66–1.61 (m, 2H), 1.43–1.37 (m, 2H), 0.94 (t, J = 7.3 Hz, 3H); 13C NMR (126 MHz, CDCl3) δ 139.95, 135.21, 135.15, 132.62, 127.09, 121.88, 117.54, 117.27, 116.28, 116.08, 40.25, 32.87, 28.52, 26.03, 22.84, 13.94; EI-MS (m/z): 371 (M+, (Br81)), 369 (M+, (Br79)); HRMS (EI) calcd for C16H20BrNO2S (M+) 369.0398, found: 369.0403.
1-Allyl-6-bromo-2-butyl-3-(methylsulfonyl)-1H-indole (3l). Gray solid (122.9 mg, yield 83%), mp 113–114 °C. 1H NMR (400 MHz, CDCl3) δ 8.14 (d, J = 1.9 Hz, 1H), 7.34 (dd, J = 8.7, 1.9 Hz, 1H), 7.16 (d, J = 8.7 Hz, 1H), 5.97–5.86 (m, 1H), 5.22 (d, J = 10.4 Hz, 1H), 4.87 (d, J = 17.1 Hz, 1H), 4.75–4.72 (m, 2H), 3.11–3.07 (m, 5H), 1.67–1.60 (m, 2H), 1.51–1.44 (m, 2H), 0.96 (t, J = 7.3 Hz, 3H); 13C NMR (126 MHz, CDCl3) δ 147.31, 134.55, 131.60, 126.69, 126.24, 122.26, 117.82, 116.03, 111.88, 110.13, 46.05, 45.94, 32.38, 24.54, 22.95, 13.86; EI-MS (m/z): 371 (M+, (Br81)), 369 (M+, (Br79)); HRMS (EI) calcd for C16H20BrNO2S (M+) 369.0398, found: 369.0395.
3-Allyl-2-butyl-6-methyl-1-(methylsulfonyl)-1H-indole (2m). Brown solid (110.0 mg, yield 90%), mp 43–44 °C. 1H NMR (400 MHz, CDCl3) δ 7.84 (s, 1H), 7.36 (d, J = 7.9 Hz, 1H), 7.10 (d, J = 7.9 Hz, 1H), 5.98–5.86 (m, 1H), 5.13–4.98 (m, 2H), 3.46–3.37 (m, 2H), 2.95–2.88 (m, 5H), 2.48 (s, 3H), 1.67–1.60 (m, 2H), 1.44–1.36 (m, 2H), 0.94 (t, J = 7.3 Hz, 3H); 13C NMR (101 MHz, CDCl3) δ 137.82, 136.84, 135.80, 134.43, 128.60, 125.06, 118.74, 118.23, 115.79, 114.90, 39.77, 32.93, 28.74, 26.02, 22.81, 22.02, 13.97; EI-MS (m/z): 305 (M+); HRMS (EI) calcd for C17H23NO2S (M+) 305.1449, found: 305.1446.
1-Allyl-2-butyl-6-methyl-3-(methylsulfonyl)-1H-indole (3m). Yellow oil (106.3 mg, yield 87%). 1H NMR (400 MHz, CDCl3) δ 7.85 (d, J = 8.9 Hz, 1H), 7.12–7.07 (m, 2H), 5.99–5.90 (m, 1H), 5.21 (d, J = 11.0 Hz, 1H), 4.90 (d, J = 17.2 Hz, 1H), 4.74–4.71 (m, 2H), 3.12–3.08 (m, 5H), 2.47 (s, 3H), 1.67–1.60 (m, 2H), 1.50–1.45 (m, 2H), 0.96 (t, J = 7.3 Hz, 3H); 13C NMR (101 MHz, CDCl3) δ 145.63, 136.19, 133.20, 132.07, 124.05, 122.91, 119.25, 117.41, 110.30, 110.08, 45.90, 45.62, 32.49, 24.37, 22.90, 21.86, 13.85; EI-MS (m/z): 305 (M+); HRMS (EI) calcd for C17H23NO2S (M+) 305.1449, found: 305.1448.
3-Allyl-2-butyl-1-(methylsulfonyl)-1H-indole-5-carbonitrile (2n). Brown solid (108.8 mg, yield 86%), mp 66–68 °C. 1H NMR (400 MHz, CDCl3) δ 8.11–8.09 (m, 1H), 7.80 (d, J = 1.1 Hz, 1H), 7.51 (dd, J = 8.7, 1.6 Hz, 1H), 5.99–5.83 (m, 1H), 5.16–4.98 (m, 2H), 3.46–3.41 (m, 2H), 3.04 (s, 3H), 2.99–2.86 (m, 2H), 1.68–1.60 (m, 2H), 1.45–1.36 (m, 2H), 0.94 (t, J = 7.3 Hz, 3H); 13C NMR (101 MHz, CDCl3) δ 140.87, 138.11, 134.91, 130.74, 127.28, 123.90, 119.58, 117.39, 116.60, 115.20, 107.05, 41.14, 32.80, 28.41, 25.92, 22.81, 13.85; EI-MS (m/z): 316 (M+); HRMS (EI) calcd for C17H20N2O2S (M+) 316.1245, found: 316.1243.
1-Allyl-2-butyl-3-(methylsulfonyl)-1H-indole-5-carbonitrile (3n). Yellow oil (97.5 mg, yield 77%). 1H NMR (400 MHz, CDCl3) δ 8.35–8.30 (m, 1H), 7.47 (dd, J = 8.6, 1.5 Hz, 1H), 7.37 (d, J = 8.4 Hz, 1H), 6.00–5.88 (m, 1H), 5.25 (d, J = 10.4 Hz, 1H), 4.87 (d, J = 17.1 Hz, 1H), 4.82–4.78 (m, 2H), 3.14–3.07 (m, 5H), 1.69–1.60 (m, 2H), 1.52–1.44 (m, 2H), 0.95 (t, J = 7.3 Hz, 3H); 13C NMR (101 MHz, CDCl3) δ 148.85, 137.47, 131.20, 126.23, 125.01, 124.98, 119.78, 118.15, 111.50, 111.47, 105.82, 46.17, 46.11, 32.26, 24.57, 22.94, 13.79; EI-MS (m/z): 316 (M+); HRMS (EI) calcd for C17H20N2O2S (M+) 316.1245, found: 316.1248.
3-Allyl-2-butyl-1-(methylsulfonyl)-5-(trifluoromethyl)-1H-indole (2o). Yellow solid (126.5 mg, yield 88%), mp 72–73 °C. 1H NMR (300 MHz, CDCl3) δ 8.12 (d, J = 8.8 Hz, 1H), 7.74 (s, 1H), 7.52 (d, J = 8.7 Hz, 1H), 6.01–5.86 (m, 1H), 5.18–4.98 (m, 2H), 3.46 (d, J = 5.9 Hz, 2H), 3.06–2.90 (m, 5H), 1.71–1.59 (m, 2H), 1.48–1.35 (m, 2H), 0.95 (t, J = 7.3 Hz, 3H); 13C NMR (126 MHz, CDCl3) δ 140.45, 137.94, 135.18, 130.56, 126.10 (q, JC–F = 32.2 Hz), 124.72 (q, JC–F = 271.9 Hz), 121.03 (q, JC–F = 3.3 Hz), 118.02, 116.48 (q, JC–F = 3.9 Hz), 116.35, 114.81, 40.70, 32.87, 28.47, 26.02, 22.86, 13.91; EI-MS (m/z): 359 (M+); HRMS (EI) calcd for C17H20F3NO2S (M+) 359.1167, found: 359.1169.
1-Allyl-2-butyl-3-(methylsulfonyl)-5-(trifluoromethyl)-1H-indole (3o). White solid (119.3 mg, yield 83%), mp 89–90 °C. 1H NMR (300 MHz, CDCl3) δ 8.29 (s, 1H), 7.49 (d, J = 8.6 Hz, 1H), 7.38 (d, J = 8.7 Hz, 1H), 6.03–5.86 (m, 1H), 5.24 (d, J = 10.4 Hz, 1H), 4.88 (d, J = 17.2 Hz, 1H), 4.80 (d, J = 2.9 Hz, 2H), 3.22–3.06 (m, 5H), 1.69–1.62 (m, 2H), 1.54–1.43 (m, 2H), 0.96 (t, J = 7.2 Hz, 3H); 13C NMR (126 MHz, CDCl3) δ 148.25, 137.25, 131.45, 124.88 (q, JC–F = 272.1 Hz), 124.87 (q, JC–F = 32.2 Hz), 124.65, 120.05 (q, JC–F = 3.4 Hz), 117.92, 117.45 (q, JC–F = 4.0 Hz), 111.48, 110.88, 46.14, 46.02, 32.35, 24.57, 22.94, 13.81; EI-MS (m/z): 359 (M+); HRMS (EI) calcd for C17H20F3NO2S (M+) 359.1167, found: 359.1162.
Acknowledgements
We gratefully acknowledge financial support from the National Natural Science Foundation of China (Grants 21021063, 91229204, and 81025017), National S&T Major Projects (2012ZX09103101-072, 2012ZX09301001-005, 2013ZX09507-001, and 2014ZX09507002-001) and Chengdu University New Faculty Start-up Funding (No. 2081915037).
Notes and references
-
(a) A. Grossmann, S. Bartlett, M. Janecek, J. T. Hodgkinson and D. R. Spring, Angew. Chem., Int. Ed., 2014, 53, 13093 CrossRef CAS PubMed;
(b) R. A. Stavenger and S. L. Schreiber, Angew. Chem., Int. Ed., 2001, 40, 3417 CrossRef CAS;
(c) W. Liu, V. Khedkar, B. Baskar, M. Schürmann and K. Kumar, Angew. Chem., Int. Ed., 2011, 50, 6900 CrossRef CAS PubMed;
(d) B. Jiang, W.-J. Hao, X. Wang, F. Shi and S.-J. Tu, J. Comb. Chem., 2009, 11, 846 CrossRef CAS PubMed;
(e) N. Gigant, E. Claveau, P. Bouyssou and I. Gillaizeau, Org. Lett., 2012, 14, 844 CrossRef CAS PubMed;
(f) E. Lenci, G. Menchi, A. Guarna and A. Trabocchi, J. Org. Chem., 2015, 80, 2182 CrossRef CAS PubMed;
(g) H. Kim, T. T. Tung and S. B. Park, Org. Lett., 2013, 15, 5814 CrossRef CAS PubMed;
(h) A. Kumar, G. Gupta and S. Srivastava, J. Comb. Chem., 2010, 12, 458 CrossRef CAS PubMed.
-
(a) Z.-C. Geng, S.-Y. Zhang, N.-K. Li, N. Li, J. Chen, H.-Y. Li and X.-W. Wang, J. Org. Chem., 2014, 79, 10772 CrossRef CAS PubMed;
(b) O. Sangmi, J. J. Hwan, K. K. Sung, K. Yeonjin and B. P. Seung, J. Comb. Chem., 2010, 12, 548 CrossRef PubMed;
(c) O. Kwon, S. B. Park and S. L. Schreiber, J. Am. Chem. Soc., 2002, 124, 13402 CrossRef CAS PubMed;
(d) H. Oguri and S. L. Schreiber, Org. Lett., 2005, 7, 47 CrossRef CAS PubMed;
(e) J. K. Sello, P. R. Andreana, D. Lee and S. L. Schreiber, Org. Lett., 2003, 5, 4125 CrossRef CAS PubMed;
(f) D. Lee, J. K. Sello and S. L. Schreiber, Org. Lett., 2000, 2, 709 CrossRef CAS PubMed;
(g) W. Tan, X.-T. Zhu, S. Zhang, G.-J. Xing, R.-Y. Zhu and F. Shi, RSC Adv., 2013, 3, 10875 RSC;
(h) J. Mahatthananchai, A. M. Dumas and J. W. Bode, Angew. Chem., Int. Ed., 2012, 51, 10954 CrossRef CAS PubMed.
-
(a) A. Kumar, S. Srivastava, G. Gupta, V. Chaturvedi, S. Sinha and R. Srivastava, ACS Comb. Sci., 2011, 13, 65 CrossRef CAS PubMed;
(b) S. Dandapani, A. R. Germain, I. Jewett, S. L. Quement, J. C. Marie, G. Muncipinto, J. R. Duvall, L. C. Carmody, J. R. Perez, J. C. Engel, J. Gut, D. Kellar, J. L. Siqueira-Neto, J. H. McKerrow, M. Kaiser, A. Rodriguez, M. A. Palmer, M. Foley, S. L. Schreiber and B. Munoz, ACS Med. Chem. Lett., 2014, 5, 149 CrossRef CAS PubMed;
(c) G. L. Thomas, R. J. Spandl, F. G. Glansdorp, M. Welch, A. Bender, J. Cockfield, J. A. Lindsay, C. Bryant, D. F. Brown, O. Loiseleur, H. Rudyk, M. Ladlow and D. R. Spring, Angew. Chem., Int. Ed., 2008, 47, 2808 CrossRef CAS PubMed;
(d) W. R. Galloway, A. Bender, M. Welch and D. R. Spring, Chem. Commun., 2009, 18, 2446 RSC.
- S. Marcaccini and T. Torroba, in Multicomponent Reactions, ed. J. Zhu and H. Bienaymé, Wiley-VCH, Weinheim, 2005, pp. 33–75 Search PubMed.
- N. A. Afagh and A. K. Yudin, Angew. Chem., Int. Ed., 2010, 49, 262 CrossRef CAS PubMed.
-
(a) G. Cuny, M. Bois-Choussy and J. Zhu, J. Am. Chem. Soc., 2004, 126, 14475 CrossRef CAS PubMed;
(b) W. Erb, L. Neuville and J. Zhu, J. Org. Chem., 2009, 74, 3109 CrossRef CAS PubMed.
-
(a) Q. Cheng, J. Zhao, Y. Ishikawa, N. Asao, Y. Yamamoto and T. Jin, Org. Lett., 2013, 15, 5766 CrossRef PubMed;
(b) T. Fujihara, Y. Katafuchi, T. Iwai, J. Terao and Y. Tsuji, J. Am. Chem. Soc., 2010, 132, 2094 CrossRef CAS PubMed;
(c) M. Suginome, M. Shirakura and A. Yamamoto, J. Am. Chem. Soc., 2006, 128, 14438 CrossRef CAS PubMed;
(d) T. Ishiyama, N. Matsuda, N. Miyaura and A. Suzuki, J. Am. Chem. Soc., 1993, 115, 11018 CrossRef CAS;
(e) N. Iwadate and M. Suginome, J. Am. Chem. Soc., 2010, 132, 2548 CrossRef CAS PubMed;
(f) P. Schreyer, P. Paetzold and R. Boese, Chem. Ber., 1988, 121, 195 CrossRef CAS;
(g) H. Braunschweig, A. Damme, J. O. Jimenez-Halla, B. Pfaffinger, K. Radacki and J. Wolf, Angew. Chem., Int. Ed., 2012, 51, 10034 CrossRef CAS PubMed;
(h) M. Suginome, H. Nakamura and Y. Ito, Chem. Commun., 1996, 2777 RSC;
(i) M. Suginome, H. Nakamura and Y. Ito, Angew. Chem., Int. Ed., 1997, 36, 2516 CrossRef CAS;
(j) T. Ohmura, K. Oshima and M. Suginome, Chem. Commun., 2008, 1416 RSC;
(k) T. Ohmura, K. Oshima, H. Taniguchi and M. Suginome, J. Am. Chem. Soc., 2010, 132, 12194 CrossRef CAS PubMed;
(l) T. Ishiyama, K. Nishijima, N. Miyaura and A. Suzuki, J. Am. Chem. Soc., 1993, 115, 7219 CrossRef CAS;
(m) M. F. Lappert and B. Prokai, J. Organomet. Chem., 1964, 1, 384 CrossRef CAS;
(n) S. Hara, H. Dojo, S. Takinami and A. Suzuki, Tetrahedron Lett., 1983, 24, 731 CrossRef CAS;
(o) S. Onozawa, Y. Hatanaka, T. Sakakura, S. Shimada and M. Tanaka, Organometallics, 1996, 15, 5450 CrossRef CAS.
-
(a) T. Shimada, I. Nakamura and Y. Yamamoto, J. Am. Chem. Soc., 2004, 126, 10546 CrossRef CAS PubMed;
(b) C.-Y. Wu, M. Hu, Y. Liu, R.-J. Song, Y. Lei, B.-X. Tang, R.-J. Li and J.-H. Li, Chem. Commun., 2012, 48, 3197 RSC;
(c) F. Zhao, D.-Y. Zhang, Y. Nian, L. Zhang, W. Yang and H. Liu, Org. Lett., 2014, 16, 5124 CrossRef CAS PubMed;
(d) C.-L. Wu, F. Zhao, S.-J. Shu, J. Wang and H. Liu, RSC Adv., 2015, 5, 90396 RSC;
(e) X.-M. Zeng, R. Kinjo, B. Donnadieu and G. Bertrand, Angew. Chem., Int. Ed., 2010, 49, 942 CrossRef CAS PubMed;
(f) I. Nakamura, U. Yamagishi, D. Song, S. Konta and Y. Yamamoto, Angew. Chem., Int. Ed., 2007, 46, 2284 CrossRef CAS PubMed;
(g) I. Nakamura, Y. Sato, S. Konta and M. Terada, Tetrahedron Lett., 2009, 50, 2075 CrossRef CAS;
(h) I. Nakamura, U. Yamagishi, D. Song, S. Konta and Y. Yamamoto, Chem.–Asian J., 2008, 3, 285 CrossRef CAS PubMed;
(i) S. Cacchi, G. Fabrizi and P. Pace, J. Org. Chem., 1998, 63, 1001 CrossRef CAS;
(j) Y. D. Dhage, T. Shirai, M. Arima, A. Nakazima, H. Hikawa, I. A. T. Kusakabe, K. Takahashi and K. Kato, RSC Adv., 2015, 5, 42623 RSC;
(k) S. Kamijo and Y. Yamamoto, J. Am. Chem. Soc., 2002, 124, 11940 CrossRef CAS PubMed;
(l) P.-Y. Chiang, Y.-C. Lin, Y. Wang and Y.-H. Liu, Organometallics, 2010, 29, 5776 CrossRef CAS;
(m) K. C. Majumdar, S. Hazra and B. Roy, Tetrahedron Lett., 2001, 52, 6697 CrossRef;
(n) E. Chong and S. A. Blum, J. Am. Chem. Soc., 2015, 137, 10144 CrossRef CAS PubMed.
-
(a) I. Nakamura, Y. Mizushima, I. D. Gridnev and Y. Yamamoto, J. Am. Chem. Soc., 2005, 127, 9844 CrossRef CAS PubMed;
(b) I. Nakamura, G. B. Bajracharya, H. Y. Wu, K. Oishi, Y. Mizushima, I. D. Gridnev and Y. Yamamoto, J. Am. Chem. Soc., 2004, 126, 15423 CrossRef CAS PubMed.
- C.-D. Wang, Y. F. Hsieh and R. S. Liu, Adv. Synth. Catal., 2014, 356, 144 CrossRef CAS.
-
(a) I. Nakamura, Y. Mizushima and Y. Yamamoto, J. Am. Chem. Soc., 2005, 127, 15022 CrossRef CAS PubMed;
(b) J. J. Hirner, D. J. Faizi and S. A. Blum, J. Am. Chem. Soc., 2014, 136, 4740 CrossRef CAS PubMed;
(c) A. Fürstner and P. W. Davies, J. Am. Chem. Soc., 2005, 127, 15024 CrossRef PubMed;
(d) Z.-Q. Liang, S.-M. Ma, J.-H. Yu and R.-R. Xu, J. Org. Chem., 2007, 72, 9219 CrossRef CAS PubMed;
(e) S. Cacchi, G. Fabrizi and L. Moro, Tetrahedron Lett., 1998, 39, 5101 CrossRef CAS.
-
(a) Q.-W. Zhang, K. An and W. He, Angew. Chem., Int. Ed., 2014, 53, 5667 CrossRef CAS PubMed;
(b) I. Nakamura, T. Sato, M. Terada and Y. Yamamoto, Org. Lett., 2008, 10, 2649 CrossRef CAS PubMed;
(c) I. Nakamura, T. Sato, M. Terada and Y. Yamamoto, Org. Lett., 2007, 9, 4081 CrossRef CAS PubMed.
- T. Matsuda and Y. Ichioka, Org. Biomol. Chem., 2012, 10, 3175 CAS.
-
(a) Y. Kobayashi, H. Kamisaki, K. Yanada, R. Yanada and Y. Takemoto, Tetrahedron Lett., 2005, 46, 7549 CrossRef CAS;
(b) Y. Kobayashi, H. Kamisaki, R. Yanada and Y. Takemoto, Org. Lett., 2006, 8, 2711 CrossRef CAS PubMed;
(c) M. R. Fielding, R. Grigg and C. J. Urch, Chem. Commun., 2000, 22, 2239 RSC.
- K. N. Tu, J. J. Hirner and S. A. Blum, Org. Lett., 2016, 18, 480 CrossRef CAS PubMed.
- T. Iwai, T. Fujihara, J. Terao and Y. Tsuji, J. Am. Chem. Soc., 2009, 131, 6668 CrossRef CAS PubMed.
- W. T. Teo, W.-D. Rao, M. J. Koh and P. W. H. Chan, J. Org. Chem., 2013, 78, 7508 CrossRef CAS PubMed.
- M. Toyofuku, S. Fujiwara, T. Shinike, H. Kuniyasu and N. Kambe, J. Am. Chem. Soc., 2005, 127, 9706 CrossRef CAS PubMed.
-
(a) M. Suginome, A. Yamamoto and M. Murakami, J. Am. Chem. Soc., 2003, 125, 6358 CrossRef CAS PubMed;
(b) A. Yamamoto and M. Suginome, J. Am. Chem. Soc., 2005, 127, 15706 CrossRef PubMed;
(c) M. Daini, A. Yamamoto and M. Suginome, J. Am. Chem. Soc., 2008, 130, 2918 CrossRef CAS PubMed.
- For representative literatures on the migration of sulfonyl group, see:
(a) X.-Y. Xin, D.-P. Wang, X.-C. Li and B.-S. Wan, Angew. Chem., Int. Ed., 2012, 51, 1693 CrossRef CAS PubMed;
(b) B. Prasad, R. Adepu, S. Sandra, D. Rambabu, G. R. Krishna, C. M. Reddy, G. S. Deora, P. Misraa and M. Pal, Chem. Commun., 2012, 48, 10434 RSC;
(c) X.-C. Hong, J. M. Mejía-Oneto, S. France and A. Padwa, Tetrahedron Lett., 2006, 47, 2409 CrossRef CAS.
Footnotes |
† Electronic supplementary information (ESI) available: Experimental details and additional spectra. See DOI: 10.1039/c6ra15945a |
‡ These authors contributed equally. |
|
This journal is © The Royal Society of Chemistry 2016 |
Click here to see how this site uses Cookies. View our privacy policy here.