DOI:
10.1039/C4RA16151C
(Paper)
RSC Adv., 2015,
5, 15487-15493
Bis(ammonium) ionic liquids with herbicidal anions†
Received
10th December 2014
, Accepted 26th January 2015
First published on 26th January 2015
Abstract
The method of synthesis of the novel bis(ammonium) herbicidal ionic liquids with the cation containing two quaternary nitrogen atoms was described. New compounds, containing three structurally different linkers, were prepared via acid–base reaction between the corresponding bis(ammonium) hydroxides and herbicides in the form of free acids. In addition, phase transitions, thermal stability and solubility in common solvents were determined. The herbicidal efficacy was tested in greenhouse experiments, wherein test plants were lambsquarters (Chenopodium album L.) cornflower (Centaurea cyanus L.), white mustard (Sinapis alba L.) and winter rape (Brassica napus L.). The biological activity of the anion (2,4-D, MCPA, Mecoprop, Dicamba) in all new HILs was preserved. Moreover, the efficacy of some salts was several times higher when compared to commercial formulations.
Introduction
Gemini surfactants are a specific group of amphiphilic compounds composed of two cationic surfactant moieties connected together by a linker group. Structurally, they comprise two aliphatic chains (called “tails”) and two hydrophilic groups (called “heads”) linked together by a linker, which may be either rigid or more susceptible to deformation.1–3 The most commonly used linkers are disulfide bridges, amides or straight alkyl chains. Furthermore, recent studies revealed that their structure affects both surface activity as well as biodegradability of the molecule. Gemini surfactants reduce the surface tension with remarkable efficiency and their effectiveness may be even 1000 times higher in comparison to conventional surfactants.4–7 In addition, they have the ability to form stable micelles at much lower concentrations and are also more soluble than most of the traditional amphiphiles, whilst preserving a high degree of biodegradation.4,8 Due to the specific composition of gemini surfactants, the insertion of various functional groups into their structure is possible and allows the preparation of compounds with various desired properties. Many of the gemini surfactants have exceptional antibacterial activity against both Gram-positive and Gram-negative bacteria, and they are often effective fungicides as well.9,10 The change of groups which form the surfactant “head” may cause the appearance of different properties in a surfactant molecule. For instance, amine derivatives containing sugar residues in the ‘head’ may be used as excellent carriers in gene delivery systems, which do not exhibit a bactericidal activity.11,12
One of the main problems in the cultivation of plants is their destruction by harmful organisms such as weeds, pathogenic fungi and pests. The negative effect of weeds is associated with the fact that they are in competition with crop plants for light, water and nutrients in the soil. Moreover, weeds cause severe difficulties during harvest and also prolong this operation. Currently, the most common method of controlling weeds is the use of herbicides.13
Extensive research has already led to the emergence of third generation of ionic liquids, which possess an expected, targeted biological activity, from pharmaceutically active compounds or new disinfectants to feeding deterrents.14–17 However, the insertion of the herbicidal anion into the structure of ionic liquids opened up a new possibility for their application. Thus, the definition of herbicidal ionic liquids (HILs) was introduced to the world literature in 2011: herbicidal ionic liquids are chemical compounds which are liquids below the temperature of 100 °C, composed of the organic cation and the anion exhibiting a herbicidal activity.18
HILs as practically non-volatile compounds are much safer in use in comparison to the compounds offered commercially. Their application will minimize the risk of poisoning by pesticide vapours and significantly improve the comfort of people performing treatment on the field.19,20 Simultaneously, as hydrophobic substances, HILs do not transfer easily into the groundwater, which gives them the status of environmentally friendly compounds.21 The most recent studies confirmed the hypothesis that the acute toxicity of HILs may be controlled by a suitable selection of the cation in the molecule. Therefore, it is possible to obtain new effective herbicides with a lower toxicity compared to caffeine or aspirin.19 As a result of intensive research, ILs with single herbicidal function (an anion as the herbicide),17–19,21–25 growth retardant function26 as well as with dual pesticidal function (an anion as the herbicide and a cation as a growth regulator20,27 or a fungicide28) have been already described. Moreover, a group of bis(ammonium) herbicidal ionic liquids with herbicidal anions (MCPA and Dicamba) was synthesized and described. Performed preliminary experiments revealed that long alkyl substituents attached to nitrogen atoms may enhance a herbicidal activity, however, the role of linker has not been fully understood.29
Promising results derived from the combination of cationic surfactants and herbicidal anions contributed to the development of the concept of combining gemini surfactants with phenoxy acid anions. This work shows the influence of the linker structure on physicochemical properties and biological activity of new gemini HILs different, and also presents their potential of application.
Results and discussion
The bis(ammonium) bromide as well as both chlorides were prepared by conducting the Menschutkin reaction of dimethyldecylamine with a quaternizing agent, which was 1,12-dibromododecane in the case of salt 1, while chlorides 2 and 3 were obtained from bis(2-chloroethyl) ether and 1,2-bis(2-chloroethoxy)ethane. Bis(ammonium) precursors of HILs were solids (Table 1) with a purity higher than 96% (surfactant content), determined by a direct two-phase titration technique (EN ISO 2871-2:2010).
Table 1 Prepared bis(ammonium) halides
Salt |
X |
R |
Yield (%) |
Purity (%) |
Tm (°C) |
1 |
Br |
(CH2)12 |
95 |
99 |
109–111 |
2 |
Cl |
(CH2)2O(CH2)2 |
92 |
96 |
207–209 |
3 |
Cl |
(CH2)2O(CH2)2O(CH2)2 |
94 |
98 |
108–110 |
The salts presented in Table 2 were synthesized via the neutralization reaction between intermediates – bis(ammonium) hydroxides and different herbicidal acids, such as (4-chloro-2-methylphenoxy)acetic acid (MCPA), 2-(4-chloro-2-methyl-phenoxy)propionic acid (Mecoprop), (2,4-dichlorophenoxy)-acetic acid (2,4-D) and 3,6-dichloro-2-methoxybenzoic (Dicamba). The acid–base reaction (Scheme 1) occurred immediately at room temperature (25 °C) and allowed to prepare compounds with the yields between 89 and 97%.
Table 2 New HILs with a bis(ammonium) cation
Salt |
A |
R |
Yield (%) |
State at 25 °C |
Mp. = 102–105 °C (BÜCHI melting point B-540). |
1a |
Mecoprop |
(CH2)12 |
95 |
Solida |
1b |
Dicamba |
(CH2)12 |
91 |
Grease |
2a |
Mecoprop |
(CH2)2O(CH2)2 |
94 |
Grease |
2b |
Dicamba |
(CH2)2O(CH2)2 |
90 |
Grease |
2c |
MCPA |
(CH2)2O(CH2)2 |
95 |
Grease |
2d |
2,4-D |
(CH2)2O(CH2)2 |
96 |
Grease |
3a |
Mecoprop |
(CH2)2O(CH2)2O(CH2)2 |
92 |
Grease |
3b |
Dicamba |
(CH2)2O(CH2)2O(CH2)2 |
96 |
Grease |
3c |
MCPA |
(CH2)2O(CH2)2O(CH2)2 |
97 |
Grease |
3d |
2,4-D |
(CH2)2O(CH2)2O(CH2)2 |
89 |
Grease |
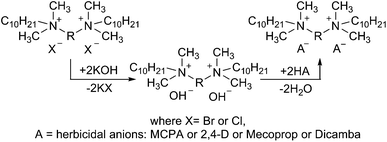 |
| Scheme 1 General synthesis of bis(ammonium) salts derived from different herbicides. | |
After the syntheses the ionic liquids were dried at 65 °C for 24 hours under vacuum. The results of elemental analysis confirmed the high purity the obtained compounds. The water content was determined to be less than 0.5% by volumetric Karl-Fischer titration.
In general, most of the synthesized salts with the C12 alkyl linker were hygroscopic greases, except compound 1a, which had a melting point above 100 °C and therefore cannot be classified as ionic liquid.
The analysis of 1H NMR spectra has shown noticeable changes in chemical shifts of protons located in the vicinity of the nitrogen atom. The significant difference in the signal position of protons derived from the methyl groups was observed between salt 2a comprising Mecoprop and salts 2b, 2d with Dicamba and 2,4-D anions, respectively (Table 3). Moreover, the presence of MCPA or 2,4-D anions in the structure also resulted in a change of chemical shifts of protons in the linker group. The lowest values were noted for the salt 2a with Mecoprop as an anions – 3.12 and 3.90 ppm. Similar tendency was noted for methyl groups of salts 3a–3d (Table 4). However, the protons from linker revealed no significant changes in chemical shifts. Only the 2,4-D anions had a visible influence on this value in comparison to other herbicidal anions.
Table 3 The chemical shiftsa (1H NMR) of salts 2a–2d
Protons |
Salts |
2a |
2b |
2c |
2d |
Shifts in ppm. |
N+CH3 |
2.99 |
3.25 |
3.11 |
3.24 |
N+CH2CH2O |
3.12 |
3.39 |
4.63 |
4.52 |
N+CH2CH2O |
3.90 |
4.11 |
3.99 |
4.09 |
Table 4 The chemical shiftsa (1H NMR) of salts 3a–3d
Protons |
Salts |
3a |
3b |
3c |
3d |
Shifts in ppm. |
N+CH3 |
3.04 |
3.26 |
3.07 |
3.23 |
N+CH2CH2O |
3.35 |
3.34 |
3.31 |
3.64 |
OCH2CH2O |
3.55 |
3.63 |
3.56 |
3.73 |
N+CH2CH2O |
3.77 |
3.74 |
3.81 |
3.92 |
The thermal stability was assessed by the thermal gravimetric analysis (TGA) according to two characteristic temperatures: Tonset5 – the temperature of 5% of sample decomposition and Tonset50 – the temperature of 50% of sample decomposition. The results were presented in Table 5.
Table 5 Thermal analysis (DSC and TGA) of the synthesized HILs
Salt |
Tma (°C) |
Tcb (°C) |
Tgc (°C) |
Tonset5%d (°C) |
Tonset50e (°C) |
Tm – melting point. Tc – temperature of crystallization. Tg – glass transition temperature. Tonset5% – decomposition temperature of 5% sample. Tonset – decomposition temperature. |
1a |
103.7 |
53.0 |
— |
210 |
332 |
1b |
— |
— |
−11.8 |
195 |
335 |
2a |
— |
— |
−19.7 |
205 |
265 |
2b |
— |
— |
−15.0 |
186 |
220 |
2c |
— |
— |
−18.1 |
210 |
265 |
2d |
— |
— |
−15.7 |
219 |
270 |
3a |
— |
— |
−19.4 |
203 |
282 |
3b |
— |
— |
−18.6 |
184 |
220 |
3c |
— |
— |
−25.1 |
205 |
270 |
3d |
— |
— |
−15.3 |
210 |
294 |
Almost all prepared ionic liquids exhibited glass transition below −11 °C. In the case of salts with one atom of oxygen in the linker 2a–2d, the glass transition temperature was in the range from −19.7 for the Mecoprop anions (2a) to −15.0 °C for the Dicamba anions (2b). In this group, the glass transition temperature increased in the following order: Mecoprop < MCPA < 2,4-D < Dicamba. On the other hand, for HILs with two oxygen atoms in the linker 3a–3d the glass transition temperatures ranged from −25.1 for MCPA anions (3a) up to −15.3 °C for 2,4-D anions, 3d. Moreover, in this group glass transition temperature rised in a different order compared to the salts 2a–2d: MCPA < Mecoprop < Dicamba < 2,4-D.
In the case of benzoates (Dicamba), an incorporation of one atom of oxygen to the linker resulted in a better flexibility of the compound, and this caused the decrease of its melting point. For the prepared HILs, specifically in case of Dicamba anions, there was a reduction of glass transition from −11.8 °C for a linker without oxygen (1b), to −15.0 °C (2b) for the cation with one oxygen atom in linker, even to −18.6 °C for the cation with two oxygen atom in linker (3b). For salts with Mecoprop anions and the linker without an oxygen atom, the glass transition temperatures was no observed, 1a – this compound possessed only a melting point (103.7 °C) and temperature of crystallization (53.0 °C). However, the incorporation of an oxygen atom into the linker causes the appearance of a glass transition temperature at around −20 °C in both cases.
Many times before, the influence of both cation and anions on thermal stability of ionic liquid has been described in the literature. In our studies, this property was determined by the TGA technique, which revealed that the obtained herbicidal ionic liquids are stable up to 200 °C. For the prepared ionic liquids, the thermal stability increased in the following order: Dicamba < Mecoprop < MCPA < 2,4-D. In this case, with the increase of nucleophilicity of the anions, the thermal stability (Tonset5) decreased from 219 °C for 2d to 205 °C for 2a. However, for HILs with Mecoprop anions, the influence of the oxygen atom in the linker was marginal. In the case of absence of heteroatom in the linker Tonset5 was equal to 210 °C (1a), but for salts with one or two oxygen atoms this value was slightly lower: 205 °C for 2a and 203 °C for 3a. The same trend was noticeable for HILs with Dicamba anions.
During the thermal decomposition of all the prepared ionic liquids the multistep degradation has been observed. In the case of alkyl linker and Dicamba anions (1b), there were four steps of degradation in the range 148–235, 238–330, 332–450 and 430–500 °C. Moreover, the three steps of degradation were observed for the salt containing the Dicamba anions and two heteroatoms in the linker (3b). The first one was from 185 to 240 °C, then the second was between 240–292 °C and the last step was from 300 to 390 °C. However, for the Mecoprop HILs 1a the first step appeared earlier (at 140–230 °C), then the second ranged from 232 to 418 °C and the last one from 420 to around 500 °C.
The other prepared HILs demonstrated two steps of thermal decomposition. The thermal decomposition of salts comprising the cation with one oxygen atom in the linker 2a–2d was in ranges: 170–300 °C and from around 300 to 370 °C for Mecoprop anions (2a); 176–305 °C and 307–420 °C for 2,4-D anions (2b); 180–310 °C and 310–380 °C for MCPA anions (2c); and 160–275 °C, 275–400 °C for Dicamba anions (2d). For this group it was observed that the first step caused around 70% of mass loss of the sample.
The two steps of thermal degradation for three HILs with two heteroatoms in linker 3a, 3c, 3d were noted in ranges: 162–275 °C and 280–400 °C for Mecoprop anions (3a); 180–280 °C and from around 280 up to 400 °C for MCPA anions (3c); 170–280 °C and 285–400 °C for 2,4-D anions (3d). However, in this group the first step of thermal decomposition step amounted to only around 50% of mass loss.
The increase in number of oxygen atoms in the linker did not cause a significant change in the thermal stability of prepared compounds. For example, HILs 2a and 3a started to decompose at 205 and 203 °C, but the first degradation step decreased with an increase of oxygen atom amount in linker form 70% to 44% for one and two heteroatoms in the linker, respectively. This was also observed in the case of imidazolium gemini ionic liquids described by Huang.30
The solubility of the obtained salts was determined in ten selected solvents, such as water, methanol, DMSO, acetonitrile, acetone, 2-isopropanol, ethyl acetate, chloroform, toluene and hexane (Table 6), according to Vogel's Textbook of Practical Organic Chemistry.31
Table 6 Determined solubility of salts at 20 °Ca
Solvent |
Salt |
1a |
1b |
2a |
2b |
2c |
2d |
3a |
3b |
3c |
3d |
“+”, complete solubility; “±”, limited solubility; “−”, insoluble. |
Water |
+ |
+ |
± |
± |
+ |
± |
± |
± |
± |
± |
Methanol |
+ |
+ |
+ |
± |
+ |
+ |
+ |
+ |
+ |
+ |
DMSO |
+ |
+ |
− |
± |
+ |
− |
− |
+ |
+ |
− |
Acetonitrile |
+ |
− |
+ |
− |
+ |
− |
+ |
+ |
+ |
+ |
Acetone |
+ |
+ |
+ |
− |
+ |
− |
+ |
+ |
+ |
+ |
2-Propanol |
+ |
+ |
+ |
± |
+ |
+ |
+ |
+ |
+ |
+ |
Ethyl acetate |
+ |
± |
+ |
± |
+ |
± |
+ |
+ |
− |
+ |
Chloroform |
+ |
+ |
+ |
+ |
+ |
+ |
+ |
+ |
+ |
+ |
Toluene |
+ |
− |
+ |
− |
+ |
− |
+ |
+ |
− |
+ |
Hexane |
− |
− |
− |
− |
− |
− |
− |
− |
− |
− |
The synthesized salts were characterized by good solubility in chloroform due to the presence of long alkyl substituents in the cation, which contributed to hydrophobic properties of the molecule. Moreover, all ionic liquids were soluble in methanol, 2-isopropanol and were insoluble in hexane. For salts derived from the precursor 1, the type of anion affected the solubility in acetonitrile, ethyl acetate and toluene. In general, the presence of Dicamba anions (1b) caused lower solubility in comparison to salt 1a comprising the Mecoprop anions. In the case of salts with cation 2 the influence of the anions was noticeable for solubility in DMSO, acetonitrile, acetone and toluene. Only the salt with MCPA anions (2c) demonstrated good solubility in all selected solvents except hexane.
Slight differences were observed for the salts derived from precursor 3. Compounds with Dicamba and MCPA anions were soluble in DMSO (3b, 3c), in contrast to salts with Mecoprop and 2,4-D (3a, 3d). However, only the IL with MCPA anions (3c) was insoluble in ethyl acetate.
The greenhouse tests were evaluated to establish the biological activity of the synthesized HILs. Data showed in Table 7 indicated that the efficacy of ionic liquids containing the bis(ammonium) cation with the long alkyl linker depended both on the anion used in the synthesis and plant species. It was noted that the ionic liquid with MCPA was more active compared to those with Dicamba. Generally, herbicidal activity of salts 1a and 1b was similar to the commercial formulation, excluding efficacy of salt 1b against cornflower. In this case the ionic liquid was less active in comparison to the commercial formulation of Dicamba. Likewise, compounds 2a and 3a (both with Mecoprop anions) were by far more effective than the MCPA standard (Fig. 1). The commercial formulation containing 2,4-D in the form of dimethylammonium salt affected the fresh weight reduction of test plants slightly in contrast to HILs 2d and 3d, which contained the same herbicidal anion (47 and 27% of the fresh weight reduction of lambsquarter, respectively). In general, the experiments performed on white mustard confirmed the high herbicidal activity of the prepared HILs, except salts 2a and 2b, comprising the cation with a short linker. Efficacy of both these compounds did not exceeded 9%.
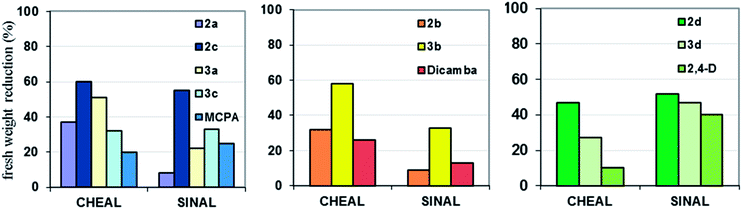 |
| Fig. 1 Fresh weight reduction (%) of HILs in the greenhouse experiment. CHEAL – Lambsquarters (Chenopodium album L.); SINAL – white mustard (Sinapis alba L.), MCPA, Dicamba, 2,4-D – the reference commercial herbicides. | |
Table 7 The influence of salts 1a and 1b on a weed control
Salt |
Fresh weight reduction (%) |
Winter rape (Brassica napus L.) |
Lambsquarters (Chenopodium album L.) |
Cornflower (Centaurea cyanus L.) |
The commercial herbicides. The commercial herbicides. |
1a |
76 |
81 |
96 |
1b |
54 |
95 |
54 |
MCPAa |
75 |
95 |
79 |
Dicambab |
55 |
88 |
80 |
Conclusions
A new family of bis(ammonium) ionic liquids demonstrating a high herbicidal efficacy was described. Nine out of ten synthesized and studied compounds may be classified as the third generation of ionic liquids, due to their physicochemical properties and biological activity. Moreover, the possibility of insertion of the active substance (Mecoprop, Dicamba, MCPA or 2,4-D) into the ionic liquid moiety, allowed to control its solubility and mobility in soil. The additional advantage of bis(ammonium) ionic liquids is the high thermal stability in a wide range of temperatures. The efficacy of the HILs in greenhouse tests depended on the species of weed. All but one of the HILs exhibited significantly higher activity than the commercial formulation against lambsquarters. Moreover, only three new HILs, comprising Mecoprop or Dicamba as the anions, were slightly less effective against white mustard than the commercial formulation.
Experimental section
General
All chemicals such as dimethyldecylamine, dibromoalkanes, ethers, solvents and other reagents were purchased from Aldrich and POCh (reagent grade ≥98%) and used without further purification. Herbicidal acids were supplied by Organika-Sarzyna S.A. Poland (MCPA and Mecoprop) and PCC Rokita SA (2,4-D). The structures of the obtained salts were confirmed by the analysis of nuclear magnetic resonance spectra (1H and 13C). The NMR spectra were recorded by Varian Mercury spectrophotometer working at 300 MHz for the 1H spectrum and at 75 MHz for the 13C spectrum. Tetramethylsilane (TMS) was used as an internal standard. CHN elemental analyses were performed at A. Mickiewicz University, Poznan (Poland). The water content was determined by using an Aquastar volumetric Karl-Fischer titration with Composite 5 solution as the titrant and anhydrous methanol as a solvent.
Preparation of bis(ammonium)halides
The appropriate dibromoalkane or ether (0.1 mol) was poured into a round-bottom flask. Next, decyldimethylamine with an 10% excess and 100 mL of acetonitrile were added. The synthesis was conducted at the boiling point temperature of the solvent for 24 hours. Then, the solvent was removed by a vacuum evaporator and 100 mL of ethyl acetate was added. The product, which precipitated in the form of a white solid, was isolated by filtration, washed with small portions of ethyl acetate (5 × 10 mL) and dried under a reduced pressure at 65 °C for 24 hours.
Synthesis of bis(ammonium) HILs
The appropriate bis(ammonium) halide (0.1 mol) was dissolved in 50 mL of anhydrous methanol, followed by the addition of 0.2 mol of potassium hydroxide dissolved in 25 mL of methanol. The reaction was conducted for 10 minutes and the precipitated inorganic by-product (potassium halide) was removed by filtration. Next, the filtrate containing bis(ammonium) hydroxide was neutralized with the selected herbicidal acid (1a–3d). The solvent was removed under reduced pressure and the crude product was obtained. Afterwards, the residue was dissolved in 50 mL of acetone and inorganic salt and other impurities were filtered off. After the evaporation of acetone, the compound was dried under vacuum for 24 hours at the temperature 65 °C.
Dodecamethylene-1,12-bis(dimethyldecylammonium) di[2-(4-chloro-2-methylphenoxy)propioniate] (1a). δH (DMSO-d6, 298 K, 300 MHz): 0.86 (t, J = 6.7 Hz, 6H, N+CH2(CH2)8CH3); 1.25 (s, 44H, N+CH2CH2(CH2)7CH3, N+CH2-CH2(CH2)8CH2CH2N+); 1.37 (d, J = 6.80 Hz, 6H, OCH(CH3)COO); 1.60 (s, 8H, N+CH2CH2(CH2)7CH3, N+CH2CH2(CH2)8CH2CH2N+); 2.14 (t, J = 8.50 Hz, 6H, CH
CHCH3); 3.03 (t, J = 19.5 Hz, 12H, N+CH3); 3.27 (t, J = 7.33 Hz, 8H, N+CH2CH2); 4.16 (kw, J = 6.80 Hz, 2H, OCH(CH3)COO); 6.70 (d, J = 8.80 Hz, 2H, CH
CH); 7.05 (m, 4H, CH
CH); δC (DMSO-d6, 298 K, 75 MHz): 13.9, 16.0, 19.3, 21.7, 21.8, 22.1, 25.8, 28.5, 28.6, 28.7, 28.8, 28.9, 29.0, 31.3, 49.8, 62.6, 76.1, 113.4, 122.1, 125.1, 127.6, 129.1, 156.1, 173.2; elemental analysis calcd (%) for C56H98Cl2N2O6 (M = 966.29): C 69.61, H 10.22, N 2.90, found: C 69.53, H 10.39, N 2.96.
Dodecamethylene-1,12-bis(dimethyldecylammonium) di[3,6-dichloro-2-methoxybenzoate] (1b). δH (DMSO-d6, 298 K, 300 MHz): 0.86 (t, J = 7.1 Hz, 6H, N+CH2(CH2)8CH3); 1.25 (s, 44H, N+CH2CH2(CH2)7CH3, N+CH2CH2(CH2)8CH2CH2N+); 1.61 (s, 8H, N+CH2CH2(CH2)7CH3, N+CH2CH2(CH2)8CH2CH2N+); 3.04 (s, 12H, N+CH3); 3.27 (t, J = 8.30 Hz, 8H, N+CH2CH2); 3.80 (t, J = 8.1 Hz, 6H, OCH3); 7.06 (d, J = 8.5 Hz, 2H, CH
CH); 7.21 (d, J = 8.5 Hz, 2H, CH
CH); δC (DMSO-d6, 298 K, 75 MHz): 13.9, 21.7, 21.7, 22.1, 25.7, 25.8, 28.5, 28.5, 28.7, 28.8, 28.9, 28.9, 31.3, 49.7, 60.9, 62.7, 125.1, 125.2, 127.0, 127.5, 139.8, 151.3, 165.2; elemental analysis calcd (%) for C52H88Cl4N2O6 (M = 979.08): C 63.79, H 9.06, N 2.86; found: C 63.88, H 9.19, N 2.73.
Oxybis(ethylene)bis(dimethyldecylammonium) di[2-(4-chloro-2-methylphenoxy)propioniate] (2a). δH (CDCl3, 298 K, 300 MHz): 0.89 (t, J = 6.7 Hz, 6H, N+CH2(CH2)8CH3); 1.26 (s, 28H, N+CH2CH2(CH2)7CH3); 1.51 (s, 4H, N+CH2CH2(CH2)7CH3); 1.53 (s, 6H, OCH(CH3)COO); 2.22 (s, 6H, CH
CCH3); 2.99 (s, 12H, N+CH3); 3.12 (m, 4H, N+CH2CH2O); 3.51 (s, 4H, N+CH2CH2); 3.90 (s, 4H, N+CH2CH2O); 4.44 (s, 2H, OCH(CH3)COO); 6.72 (d, J = 8.7 Hz, 2H, CH
CH); 7.00 (d, J = 8.5 Hz, 2H, CH
CH); 7.05 (s, 2H, CH
CH); δC (CDCl3, 298 K, 75 MHz): 13.9, 16.3, 19.2, 22.6, 26.2, 29.2, 29.4, 31.7, 51.0, 63.4, 64.3, 65.5, 75.2, 113.1, 123.9, 125.9, 128.7, 129.9, 155.7, 176.7; elemental analysis calcd (%) for C48H82Cl2N2O7 (M = 870.08): C 66.26, H 9.50, N 3.22; found: 66.41, H 9.69, N 3.30.
Oxybis(ethylene)bis(dimethyldecylammonium) di[3,6-dichloro-2-methoxybenzoate] (2b). δH (CDCl3, 298 K, 300 MHz): 0.89 (t, J = 6.8 Hz, 6H, N+CH2(CH2)8CH3), 1.25 (s, 28H, N+CH2CH2(CH2)7CH3), 1.58 (s, 4H, N+CH2CH2(CH2)7CH3), 3.25 (s, 12H, N+CH3), 3.39 (s, 4H N+CH2CH2O), 3.82 (s, 4H, N+CH2CH2), 3.90 (s, 6H, OCH3), 4.11 (s, 4H, N+CH2CH2O), 6.99 (d, J = 8.5 Hz, 2H, CH
CH), 7.09 (d, J = 8.4 Hz, 2H, CH
CH); δC (CDCl3, 298 K, 75 MHz): 13.8, 22.3, 25.9, 28.9, 29.1, 31.5, 50.8, 61.4, 63.3, 64.3, 65.2, 125.2, 125.8, 125.9, 127.5, 139.3, 151.4, 167.5; elemental analysis calcd (%) for C44H72Cl4N2O7 (M = 882.86): C 59.86, H 8.22, N 3.17; found: C 59.70, H 8.19, N 3.29.
Oxybis(ethylene)bis(dimethyldecylammonium) di[(4-chloro-2-methylphenoxy)acetate] (2c). δH (CDCl3, 298 K, 300 MHz): 0.88 (t, J = 6.7 Hz, 6H, N+CH2(CH2)8CH3); 1.28 (s, 28H, N+CH2CH2(CH2)7CH3); 1.56 (s, 4H, N+CH2CH2(CH2)7CH3); 2.24 (s, 6H, CH
CHCH3); 3.11 (s, 12H, N+CH3); 3.28 (s, 4H, N+CH2CH2); 3.99 (s, 4H, CH2CH2O); 4.30 (s, 4H, OCH2COO); 4.63 (s, 4H, N+CH2CH2O); 6.72 (d, J = 8.6 Hz, 2H, CH
CH); 7.00 (d, J = 2.7 Hz, 2H, CH
CH); 7.05 (s, 2H, CH
CH); δC (CDCl3, 298 K, 75 MHz): 13.8, 16.2, 22.4, 22.5, 26.1, 29.1, 29.2, 31.6, 51.2, 63.3, 64.4, 65.4, 68.4, 112.6, 123.9, 125.9, 128.2, 130.5, 155.9, 172.6; elemental analysis calcd (%) for C46H78Cl2N2O7 (M = 842.03): C 65.61, H 9.34, N 3.33; found: C 65.61, H 9.40, N 3.22.
Oxybis(ethylene)bis(dimethyldecylammonium) di[(2,4-dichlorophenoxy)acetate] (2d). δH (CDCl3, 298 K, 300 MHz): 0.88 (t, J = 6.7 Hz, 6H, N+CH2(CH2)8CH3); 1.24 (s, 28H, N+CH2CH2(CH2)7CH3); 1.62 (s, 4H, N+CH2CH2(CH2)7CH3); 3.24 (s, 12H, N+CH3); 3.38 (m, 4H, N+CH2CH2); 3.79 (s, 4H, CH2CH2O); 4.09 (s, 4H, CH2CH2O); 4.52 (s, 4H, N+CH2CH2O); 6.87 (d, J = 8.8 Hz, 2H, CH
CH); 7.13 (d, J = 2.7 Hz, 2H, CH
CH); 7.32 (s, 2H, CH
CH); δC (CDCl3, 298 K, 75 MHz): 13.9, 22.5, 22.6, 26.1, 29.1, 29.3, 31.6, 51.1, 63.6, 64.3, 65.5, 68.1, 112.6, 114.5, 125.1, 127.4, 129.4, 153.2, 171.5; elemental analysis calcd (%) for C44H72Cl4N2O7 (M = 882.86): C 59.86, H 8.22, N 3.17; found: C 59.97, H 8.35, N 3.06.
Ethylenebis(oxyethylene)bis(dimethyldecylammonium) di[2-(4-chloro-2-methylphenoxy)propioniate] (3a). δH (CDCl3, 298 K, 300 MHz): 0.89 (t, J = 6.7 Hz, 6H, N+CH2(CH2)8CH3) 1.26 (s, 28H, N+CH2CH2(CH2)7CH3); 1.54 (s, 4H, N+CH2CH2(CH2)7CH3); 1.56 (s, 6H, OCH(CH3)COO); 2.22 (s, 6H, CH
CCH3); 3.04 (s, 12H, N+CH3); 3.18 (m, 4H, N+CH2CH2); 3.35 (s, 4H, N+CH2CH2O); 3.55 (s, 4H, OCH2CH2O); 3.77 (s, 4H, N+CH2CH2O); 4.47 (s, 2H, OCHCOO); 6.74 (d, J = 8.7 Hz, 2H, CH
CH); 6.98 (d, J = 2.5 Hz, 2H, CH
CH); 7.04 (s, 2H, CH
CH); δC (CDCl3, 298 K, 75 MHz): 13.9, 16.2, 19.1, 22.4, 26.1, 29.0, 29.2, 31.6, 51.1, 63.0, 64.5, 65.5, 69.9, 75.4, 113.1, 123.8, 125.8, 128.6, 129.8, 155.7, 176.3; elemental analysis calcd (%) for C50H86Cl2N2O8 (M = 914.13): C 65.69, H 9.48, N 3.06; found: C 65.80, H 9.55, N 2.99.
Ethylenebis(oxyethylene)bis(dimethyldecylammonium) di[3,6-dichloro-2-methoxybenzoate] (3b). δH (CDCl3, 298 K, 300 MHz): 0.88 (t, J = 6.8 Hz, 6H, N+CH2(CH2)8CH3); 1.22 (s, 28H, N+CH2CH2(CH2)7CH3); 1.61 (s, 4H, N+CH2CH2(CH2)7CH3); 3.26 (s, 12H, N+CH3); 3.28 (s, 4H, N+CH2CH2); 3.34 (s, 4H, N+CH2CH2O); 3.63 (s, 4H, OCH2CH2O); 3.74 (s, 4H, N+CH2CH2O); 3.91 (s, 6H, OCH3); 6.99 (d, J = 8.6 Hz, 2H, CH
CH); 7.09 (d, J = 8.6 Hz, 2H, CH
CH); δC (CDCl3, 298 K, 75 MHz): 13.9, 22.4, 24.7, 26.1, 29.1, 29.3, 31.6, 51.3, 61.5, 62.9, 64.6, 65.5, 69.9, 125.3, 125.9, 127.7, 139.5, 151.6, 167.9; elemental analysis calcd (%) for C46H76Cl4N2O8 (M = 926.92): C 59.61, H 8.26, N 3.02; found: C 59.77, H 8.11, N 3.25.
Ethylenebis(oxyethylene)bis(dimethyldecylammonium) di[(4-chloro-2-methylphenoxy)acetate] (3c). δH (CDCl3, 298 K, 300 MHz): 0.86 (t, J = 6.7 Hz, 6H, N+CH2(CH2)8CH3); 1.24 (s, 28H, N+CH2CH2(CH2)7CH3); 1.64 (m, 4H, N+CH2CH2(CH2)7CH3); 2.15 (s, 6H, CH
CCH3); 3.07 (s, 12H, N+CH3); 3.31 (m, 4H, N+CH2CH2O); 3.53 (t, J = 4.3 Hz, 4H, N+CH2CH2); 3.56 (s, 4H, OCH2CH2O); 3.81 (s, 4H, N+CH2CH2O); 4.14 (s, 4H, OCH2COO); 6.69 (d, J = 8.6 Hz, 2H, CH
CH); 7.00 (d, J = 2.0 Hz, 2H, CH
CH); 7.11 (s, 2H, CH
CH); δC (CDCl3, 298 K, 75 MHz): 13.9, 16.0, 21.9, 22.1, 25.9, 28.6, 28.9, 31.3, 50.5, 62.1, 63.9, 64.1, 68.5, 69.2, 112.9, 122.5, 125.9, 127.8, 129.3, 156.3, 170.1; elemental analysis calcd (%) for C48H82Cl2N2O8 (M = 886.08): C 65.06, H 9.33, N 3.16; found: C 65.17, H 9.47, N 3.04.
Ethylenebis(oxyethylene)bis(dimethyldecylammonium) di[(2,4-dichlorophenoxy)acetate] (3d). δH (CDCl3, 298 K, 300 MHz): 0.88 (t, J = 6.8 Hz, 6H, N+CH2(CH2)8CH3); 1.25 (s, 28H, N+CH2CH2(CH2)7CH3); 1.65 (s, 4H, N+CH2CH2(CH2)7CH3); 3.23 (s, 12H, N+CH3); 3.53 (m, 4H, N+CH2CH2); 3.64 (s, 4H, N+CH2CH2O); 3.73 (s, 4H, OCH2CH2O); 3.92 (s, 4H, N+CH2CH2O); 4.45 (s, 4H, OCH2COO); 6.89 (d, J = 8.8 Hz, 2H, CH
CH); 7.13 (d, J = 8.9 Hz, 2H, CH
CH); 7.31 (s, 2H, CH
CH); δC (CDCl3, 298 K, 75 MHz): 13.8, 22.3, 26.1, 28.9, 29.1, 31.5, 51.2, 62.9, 64.5, 65.5, 68.6, 69.9, 114.5, 122.3, 124.5, 127.3, 129.1, 153.5, 171.5; elemental analysis calcd (%) for C46H76Cl4N2O8 (M = 926.92): C 59.61, H 8.26, N 3.02; found: C 59.83, H 8.11, N 3.19.
Thermal analysis
Thermal transition temperatures of the obtained salts were determined by DSC, using a Mettler Toledo Stare DSC1 apparatus (Leicester, UK). Samples between 5 and 15 mg were placed in aluminum pans and heated from 25 to 120 °C at a heating rate of 10 °C min−1 and cooled using an intracooler at a cooling rate of 10 °C min−1 to −100 °C. Thermogravimetric analysis was performed using a Mettler Toledo Stare TGA/DSC1 unit (Leicester, UK) under nitrogen. Samples between 2 and 10 mg were placed in aluminum pans and heated from 30 to 450 °C at a heating rate of 10 °C min−1. Nitrogen was used as a carrier gas.
Herbicidal activity
The obtained bis(ammonium) HILs containing the C12 alkyl linker were tested on three weed species: lambsquarters (Chenopodium album L.), cornflower (Centaurea cyanus L.) and winter rape (Brassica napus L.). The activity of the salts (8–15) was determined towards lambsquarters and white mustard (Sinapis alba L.). Plants seeds were sown into pots (0.5 L) and kept in a greenhouse at the temperature of 20 °C and humidity of 60%. The plants were treated by the herbicide after formation of 6 leaves. A spray solution was prepared by dissolving the synthesized salts in a water–ethanol mixture (1
:
1). The treatments were applied using sprayer (APORO, Poznan, Poland) with TeeJet 110/02 flat-fan nozzles (TeeJet Technologies, Wheaton, IL, USA) delivering 200 L of spray solution per 1 ha at 0.2 MPa pressure. The distance from the nozzles to the tips of the plant was about 40 cm and the sprayer was moving above the plants, at constant speed of 3.1 m s−1. The study was carried out in 4 replications in a randomized setup. After the treatment the plants were transported back into the greenhouse for 2 weeks. Later, the plants were cut to soil level and weighed with an accuracy of 0.01 g. The efficacy of the herbicides was calculated on the basis of the reduction of plant fresh weight compared to the control (no herbicides).
The synthesized ionic liquids with MCPA, Mecoprop and 2,4-D anions were applied at the dose of 400 g of an active substance per 1 ha. For salts containing Dicamba the dose was equal to 200 g of the active substance per 1 ha. Each HIL was compared with a corresponding commercial product: Chwastox Extra 300 SL (300 g of sodium and potassium salts of MCPA in 1 L), Aminopielik Standard 600 SL (600 g dimethylammonium salts of 2,4-D in 1 L) and Dikamba 480 SL (480 g Dicamba in 1 L).
Acknowledgements
This work was supported by grant PBS2/A1/9/2013, The National Centre for Research and Development, Warszawa, Poland.
Notes and references
- J. A. Kirby, P. Camilleri, J. B. Engberts, M. C. Feiters, R. J. Nolte, O. Söderman, M. Bergsma, P. C. Bell, M. L. Fielden, C. L. García Rodríguez, P. Guédat, A. Kremer, C. McGregor, C. Perrin, G. Ronsin and M. C. van Eijk, Angew. Chem., Int. Ed., 2003, 42, 1448 CrossRef PubMed.
- F. M. Menger and J. S. Keiper, Angew. Chem., Int. Ed., 2000, 39, 1906 CrossRef.
- T. Yoshimura, A. Sakato and K. J. Esumi, J. Oleo Sci., 2013, 62, 579 CrossRef CAS PubMed.
- F. M. Menger and C. A. Littau, J. Am. Chem. Soc., 1993, 115, 10083 CrossRef CAS.
- A. Sokołowski, K. A. Wilk, U. Komorek, B. Rutkowski and L. Syper, Physicochem. Probl. Miner. Process., 2002, 36, 51 Search PubMed.
- X. Wang, J. Wang, Y. Wang, H. Yan, P. Li and R. K. Thomas, Langmuir, 2004, 20, 53 CrossRef CAS PubMed.
- U. Komorek and K. A. Wilk, J. Colloid Interface Sci., 2004, 271, 206 CrossRef CAS PubMed.
- M. J. Rosen and D. J. Tracy, J. Surfactants Deterg., 1998, 1, 547 CrossRef CAS.
- F. Zhou, J. Zhang, X. Sun, Q. Wang and Y. Sun, J. Fiber Bioeng. Informat., 2008, 11, 165 CrossRef.
- A. Shirai, T. Sumitomo, M. Yoshida, T. Kaimura, H. Nagamune, T. Maeda and H. Kourai, Chem. Pharm. Bull., 2006, 54, 639 CrossRef CAS PubMed.
- L. Karlsson, M. C. P. van Eijk and O. Söderman, J. Colloid Interface Sci., 2002, 252, 290 CrossRef CAS PubMed.
- S. J. Ryhänen, M. J. Säily, T. Paukku, S. Borocci, G. Mancini, J. M. Holopainen and P. K. Kinnunen, Biophys. J., 2003, 84, 578 CrossRef.
- R. Kordala-Markiewicz, H. Rodak, B. Markiewicz, F. Walkiewicz, A. Sznajdrowska, K. Materna, K. Marcinkowska, T. Praczyk and J. Pernak, Tetrahedron, 2014, 70, 4784 CrossRef CAS.
- K. Bica, H. Rodriguez, G. Gurau, O. A. Cojocaru, A. Riisager, R. Fehrmannd and R. D. Rogers, Chem. Commun., 2012, 48, 5422 RSC.
- W. L. Hough, M. Smiglak, H. Rodríguez, R. P. Swatloski, S. K. Spear, D. T. Daly, J. Pernak, J. E. Grisel, R. D. Carliss, M. D. Soutullo, J. H. Davis Jr and R. D. Rogers, New J. Chem., 2007, 31, 1429 RSC.
- J. Pernak, J. Nawrot, M. Kot, B. Markiewicz and M. Niemczak, RSC Adv., 2013, 3, 25019 RSC.
- B. Markiewicz, A. Sznajdrowska, Ł. Chrzanowski, Ł. Ławniczak, A. Zgoła-Grześkowiak, K. Kubiak, J. Nawrot and J. Pernak, New J. Chem., 2014, 38, 3146 RSC.
- J. Pernak, A. Syguda, D. Janiszewska, K. Materna and T. Praczyk, Tetrahedron, 2011, 26, 4838 CrossRef.
- O. A. Cojocaru, J. L. Shamshina, G. Gurau, A. Syguda, T. Praczyk, J. Pernak and R. D. Rogers, Green Chem., 2013, 15, 2110 RSC.
- J. Pernak, M. Niemczak, K. Materna, K. Marcinkowska and T. Praczyk, Tetrahedron, 2013, 69, 4665 CrossRef CAS.
- J. Pernak, A. Syguda, K. Materna, E. Janus, P. Kardasz and T. Praczyk, Tetrahedron, 2012, 68, 4267 CrossRef CAS.
- T. Praczyk, P. Kardasz, E. Jakubiak, A. Syguda, K. Materna and J. Pernak, Weed Sci., 2012, 60, 189 CrossRef CAS.
- J. T. Polit, T. Praczyk, J. Pernak, Ł. Sobiech and G. Skrzypczak, Acta Physiol. Plant., 2014, 36, 699 CrossRef CAS.
- J. Pernak, M. Niemczak, R. Giszter, J. L. Shamshina, G. Gurau, O. A. Cojocaru, T. Praczyk, K. Marcinkowska and R. D. Rogers, ACS Sustainable Chem. Eng., 2014, 2, 2845 CrossRef CAS.
- G. Ding, Y. Liu, B. Wang, D. Punyapitak, M. Guo, Y. Duan, J. Li and Y. Cao, New J. Chem., 2014, 38, 5590 RSC.
- T. Praczyk, K. Zakrocka, D. Wyrzykowska, M. Niemczak and J. Pernak, Cent. Eur. J. Chem., 2013, 11, 1816 CrossRef CAS.
- J. Pernak, M. Niemczak, K. Zakrocka and T. Praczyk, Tetrahedron, 2013, 69, 8132 CrossRef CAS.
- J. Pernak, B. Markiewicz, A. Zgoła-Grzeskowiak, Ł. Chrzanowski, R. Gwiazdowski, K. Marcinkowska and T. Praczyk, RSC Adv., 2014, 4, 39751 RSC.
- K. Marcinkowska, K. Czerniak, R. Giszter and M. Niemczak, Przem. Chem., 2013, 92, 1633 CAS.
- K. Huang, X. Han, X. Zhang and D. W. Armstrong, Anal. Bioanal. Chem., 2007, 389, 2265 CrossRef CAS PubMed.
- A. I. Vogel and B. S. Furniss, Vogel's Textbook of Practical Organic Chemistry, Longman, 4th edn, 1984 Search PubMed.
Footnote |
† Electronic supplementary information (ESI) available. See DOI: 10.1039/c4ra16151c |
|
This journal is © The Royal Society of Chemistry 2015 |
Click here to see how this site uses Cookies. View our privacy policy here.