DOI:
10.1039/D4QM00395K
(Research Article)
Mater. Chem. Front., 2024,
8, 2874-2881
Design of Cr3+-activated broadband NIR phosphors with tunable and abnormal thermal quenching behavior for NIR pc-LEDs†
Received
13th May 2024
, Accepted 27th June 2024
First published on 1st July 2024
Abstract
Cr3+-activated broadband near-infrared (NIR) phosphors usually show controllable and excellent photoluminescence (PL) properties, but their poor thermal stability remains a big challenge. Herein, a series of Lu3−xCaxGa5−xSixO12:Cr3+ garnet phosphors with tunable and abnormal thermal quenching performance have been successfully proposed. It is found that both the crystal field strength and calculated energetic difference between 4T2 and 2E states decrease obviously with increasing [Ca2+–Si4+] co-substitution, resulting in the thermal occupation of the 4T2 state and broadened PL spectra. More importantly, the Lu3−xCaxGa5−xSixO12:Cr3+ phosphors show improved PL thermal stability depending on the different thermal population between 4T2 and 2E states, and the mechanism is investigated in detail. The PL intensity of the optimal sample reaches up to 125% and 121% at 425 K and 475 K compared with that at 300 K, respectively, which is much better than those of most Cr3+-activated broadband NIR phosphors. A NIR phosphor-converted light-emitting diode (NIR pc-LED) has been fabricated using the as-prepared thermally stable phosphor and its application in bio-imaging and night vision is demonstrated.
1. Introduction
Nowadays, NIR spectroscopy is widely used in many fields of our daily life, such as food quality analysis, night vision, medical imaging, plant growth, and so on.1 Among the various NIR sources, NIR pc-LEDs have received much attention due to the merits of high efficiency, small size, energy saving, environmentally friendly nature and tunable PL properties over the traditional ones (NIR LEDs, tungsten and halogen lamps).2,3 As a key component of NIR pc-LEDs, the PL properties of phosphors have a great influence on the performance of LED devices.4 It is known that the working temperature of the LED device can reach up to above 100 °C (even 150 °C) because of accumulated heat from the chip.5 The PL intensity of NIR phosphors usually decreases obviously due to enhanced non-radiative transition with increasing temperature,6 leading to a decreased device performance. Therefore, the development of NIR phosphors with improved thermal stability is quite important for further applications.7
Cr3+-activated NIR phosphors usually show strong absorption (4A2 → 4T1) in the blue light region, which can match well with that of the commercial blue LED chip.8 In addition, the broadband NIR emission (peak position and bandwidth) of Cr3+ can be easily tuned depending on the crystal field strength, due to its unique 3d3 electron configuration.4,9 Thanks to the above characteristics, Cr3+ is considered to be an ideal NIR emitter and has been extensively explored in recent years. For example, Na3ScF6:Cr3+ (λem = 774 nm, FWHM ≈ 108 nm, and I425K ≈ 27%) shows a high Cr3+ doping concentration (35.96%) and the internal quantum efficiency (IQE) and external quantum efficiency (EQE) are as high as 91.5% and 40.82%, respectively.10 Through lithium ion compensation, Mg4Ta2O9:Cr3+ (λem = 842 nm, FWHM ≈ 167 nm, and I425K ≈ 25%) reaches a record EQE of 61.25%.11 Compounds with a garnet structure are popular hosts for Cr3+ ions due to the wide bandgap, high structural rigidity and adjustable composition, such as Gd2.4Lu0.6Ga4AlO12:Cr3+ (λem = 728 nm, FWHM ≈ 107 nm, and I425K ≈ 75%),12 CaLu2Mg2Si3O12:Cr3+ (λem = 765 nm, FWHM ≈ 120 nm, and I425K ≈ 82%),13 and Gd3Sc2Ga3O12:Cr3+ (λem = 760 nm, FWHM ≈ 120 nm, and I425K ≈ 86%).14 Although the above-mentioned Cr3+-activated broadband NIR phosphors show excellent PL properties and high luminous efficiency, the poor thermal stability still remains a big challenge before practical application, especially for high-power devices.
In this work, a series of Lu3−xCaxGa5−xSixO12:Cr3+ phosphors with tunable and abnormal thermal quenching performance are designed and presented. It is found that the temperature-dependent luminescence properties of Lu3−xCaxGa5−xSixO12:Cr3+ can be controlled by the thermal population of the 4T2 energy level, which is highly related to the energetic difference between 4T2 and 2E states.
2. Experimental section
2.1 Synthesis
A series of Lu3−xCaxGa5−xSixO12:0.010Cr3+ (x = 0–0.5) phosphors were synthesized by a traditional high-temperature solid-state reaction method. Lu2O3 (99.995%), CaCO3 (A.R.), SiO2 (99.95%), Cr2O3 (A.R.), Ga2O3 (99.99%) and NH4Cl (A. R.) were used as raw materials without further purification. The raw materials were weighed stoichiometrically (0.3 wt% of NH4Cl as flux) and finely ground in an agate mortar for 20 min. The mixture was transferred to a corundum crucible and sintered at 1300 °C for 5 h. After cooling to room temperature, the samples were reground for further characterization. Lu2.7Ca0.3Ga4.7−xSi0.3O12:xCr3+ (x = 0.005–0.040) phosphors were synthesized similarly according to the above procedures.
2.2 Characterization
The phase purity and crystal structure of the samples were confirmed using a Bruker D8 Advance X-ray diffractometer (XRD) operating with a Cu Kα radiation source (λ = 1.5405 Å) under 40 kV and 40 mA. Measurements were carried out at room temperature over 5–90° 2θ range. Rietveld refinement was performed using TOPAS-Academic V5 software. The PL excitation (PLE) spectra, PL spectra, and PL decay curves were measured on a FLS1000 spectrometer (Edinburgh Instruments, a 450 W Xe900 continuous xenon lamp as the excitation source) equipped with a thermo-electronic cooled (−22 °C) R928P photomultiplier (PMT) from Hamamatsu and a MercuryiTC temperature controller (Oxford).
2.3 LED fabrication
The NIR pc-LED device was fabricated by coating the prepared NIR phosphor on an InGaN blue chip (450 nm, 1 W) with a mass ratio of the phosphor to epoxy resin of 1
:
1. The current-dependent electroluminescence and output power were collected using an ATA-1000 photoelectric measuring system (EVERFINE, China).
3. Results and discussion
3.1. Crystal structure of Lu3−xCaxGa5−xSixO12
The phase purity of as-prepared Lu3−xCaxGa5−xSixO12:Cr3+ (x = 0–0.5) phosphors was examined by X-ray diffraction (XRD). All the diffraction peaks are in good agreement with the standard data of Lu3Ga5O12 (PDF no. 13-0400) and no impurity peaks are observed (Fig. 1a), indicating that the co-substitution of [Lu3+–Ga3+] by [Ca2+–Si4+] has almost no influence on the crystal structure. The Rietveld refinement (Fig. 1b) is performed using Lu3Ga5O12 as the starting model, and the small residual factors (Rp = 6.00%, Rwp = 10.23%, Table S1, ESI†) further confirm the good phase purity. Lu3−xCaxGa5−xSixO12 crystallize in the same space group Ia
d (no. 230) as the Lu3Ga5O12 garnet and there are three kinds of polyhedra in the garnet structure: (Lu/Ca)O8 dodecahedra, GaO6 octahedra and (Ga/Si)O4 tetrahedra (Fig. 1c). Cr3+ ions (r = 0.615 Å, CN = 6) prefer to replace the octahedral Ga3+ site (r = 0.62 Å, CN = 6) due to the similar radius. The (Ga/Cr)O6 octahedra might be enlarged and distorted (Fig. 1c) due to the much smaller ionic radius of Si4+ (r = 0.26 Å and CN = 4) than Ga3+ (r = 0.47 Å and CN = 4), indicating a weakening crystal field strength,15 and then a broadened emission can be anticipated with increasing x values in Lu3−xCaxGa5−xSixO12:Cr3+ phosphors.
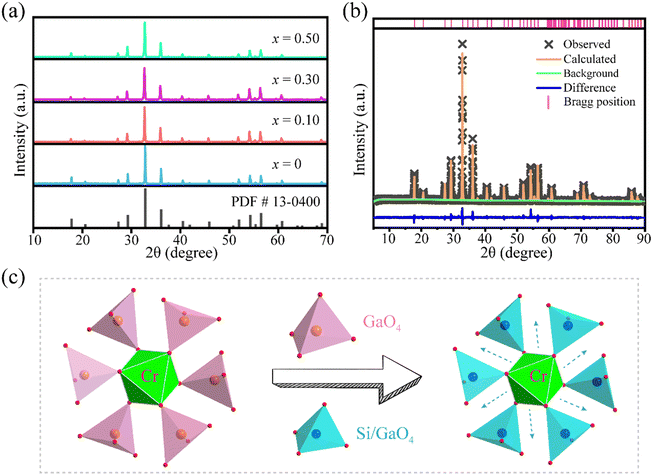 |
| Fig. 1 (a) XRD patterns of Lu3−xCaxGa5−xSixO12:Cr3+ (x = 0–0.5). (b) The Rietveld refinement of Lu2.7Ca0.3Ga4.7Si0.3O12:Cr3+ (Rp = 6.00%, Rwp = 10.23%). (c) The crystal structure. | |
Fig. S1 (ESI†) presents the SEM and elemental mapping images of Lu2.7Ca0.3Ga4.7Si0.3O12:Cr3+ phosphors, which show an irregular shape with several hundred nanometers in size. All the elements such as Lu, Ga, Ca, Si, O and Cr are found to be distributed uniformly in the matrix.
3.2. PL properties of Lu3−xCaxGa5−xSixO12:Cr3+
To investigate the influence of [Ca2+–Si4+] co-substitution on the PL properties, the emission spectra of Lu3−xCaxGa5−xSixO12:0.01Cr3+ are studied in detail. It can be seen that the room-temperature PL spectrum of Lu3Ga5O12:0.01Cr3+ is dominated by a sharp emission peaked at 705 (R1 line) and 691 nm (R2 line) corresponding to 2E → 4A2 transition of Cr3+ (Fig. 2a). Meanwhile, the broadband emission from 620 to 830 nm comes from 4T2 → 4A2 transition.16 With increasing [Ca2+–Si4+] co-substitution, the PL intensity of the broadband 4T2 → 4A2 emission increases significantly (Fig. 2b), leading to an increased value (from 43 to 150 nm, Fig. S2, ESI†) of full width at half maximum (FWHM).
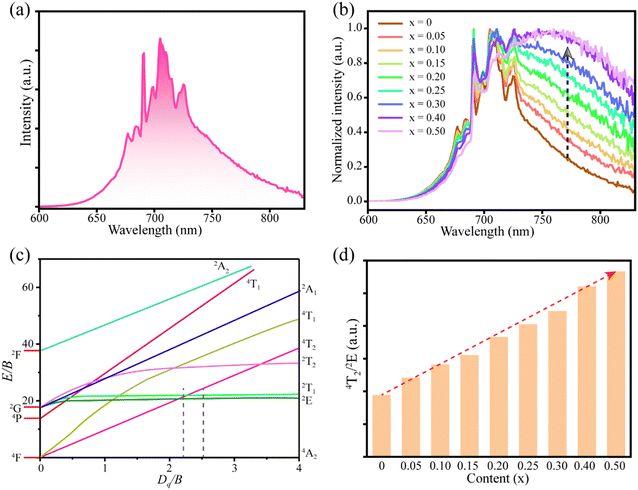 |
| Fig. 2 (a) Emission spectrum of Lu3Ga5O12:0.01Cr3+ (λex = 430 nm). (b) Emission spectra (λex = 430 nm) of Lu3−xCaxGa5−xSixO12:0.01Cr3+ (x = 0–0.5). (c) Tanabe–Sugano diagram of Cr3+ ions in an octahedral coordination. (d) Fluorescence intensity ratio (FIR) of 4T2 → 4A2 to 2E → 4A2 [I(4T2)/I(2E)] transitions of Lu3−xCaxGa5−xSixO12:0.01Cr3+ (x = 0–0.5). | |
The spectral broadening of Lu3−xCaxGa5−xSixO12:0.01Cr3+ is probably related to the decrease of crystal field strength with x value. As is well known, the energy of the 4T2 level of Cr3+ is lower than that of the 2E level when exposed to a weak crystal field, according to the Tanabe–Sugano diagram for the 3d3 electron configuration (Fig. 2c), and the PL spectra consist of an intense broadband emission band. The energy of the 2E level is lower as a result of a strong crystal field, and the PL spectra consist of a sharp emission line. When Cr3+ ions are exposed to intermediate crystal fields, both emission bands are visible in the PL spectra. Therefore, to further explore the influence of [Ca2+–Si4+] co-substitution on the PL properties, the crystal field splitting parameters (Dq and B) of Lu3−xCaxGa5−xSixO12:0.01Cr3+ (x = 0–0.5) are calculated from the PL excitation spectra (PLE, Fig. S3, ESI†) with the following equations:17,18
| 10Dq = E(4T2) = E(4A2→4T2) | (1) |
| 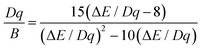 | (2) |
| ΔE = E(4T1) − E(4T2) = E(4A2→4T1) − E(4A2→4T2) | (3) |
Here,
Dq and
B represent the crystal field strength and repulsive force between 3d electrons, respectively.
16 The calculated results are shown in
Table 1. It can be seen that the
Dq/
B values of Lu
3−xCa
xGa
5−xSi
xO
12:Cr
3+ decrease from 2.52 to 2.23 with increasing
x, indicating that a decreased crystal field strength is obtained with increasing [Ca
2+–Si
4+] co-substitution. The weakened crystal field strength downshifts the
4T
2 energy level (
Fig. 2c), due to which the possibility of thermal population at the
4T
2 state will increase compared with that at the
2E state,
15 leading to an increased fluorescence intensity ratio (FIR) of
4T
2 →
4A
2 to
2E →
4A
2 [
I(
4T
2)/
I(
2E)] transitions (
Fig. 2d). The decreased lifetime of Lu
3−xCa
xGa
5−xSi
xO
12:0.01Cr
3+ (Fig. S4, ESI
†) is another evidence for the thermally populated
4T
2 energy level.
19
Table 1 Crystal field splitting parameter Dq, Racah parameter B and values of energy levels of Lu3−xCaxGa4.99−xSixO12:0.01Cr3+ (x = 0–0.5)
x
|
Dq (cm−1) |
B (cm−1) |
Dq/B |
Δ
1 (cm−1) |
Sℏω (cm−1) |
Δ
2 (cm−1) |
0 |
1652.89 |
655.07 |
2.52 |
2344.53 |
1551.25 |
793.28 |
0.05 |
1639.34 |
667.91 |
2.45 |
2209.05 |
1592.36 |
616.69 |
0.1 |
1631.32 |
680.03 |
2.40 |
2148.91 |
1648.02 |
500.89 |
0.15 |
1626.02 |
680.84 |
2.39 |
2115.89 |
1674.10 |
441.79 |
0.2 |
1623.38 |
684.91 |
2.37 |
2109.47 |
1709.93 |
399.54 |
0.25 |
1618.12 |
685.71 |
2.36 |
2116.53 |
1742.04 |
374.49 |
0.3 |
1612.90 |
693.89 |
2.32 |
2064.33 |
1749.85 |
314.48 |
0.4 |
1602.56 |
702.92 |
2.28 |
1960.94 |
1733.48 |
227.46 |
0.5 |
1594.90 |
715.45 |
2.23 |
1923.72 |
1706.64 |
217.08 |
To further analyze the PL properties of Lu3−xCaxGa5−xSixO12:0.01Cr3+ in detail, a configurational coordinate diagram (Fig. 3a) is constructed based on the obtained spectral characteristics. Δ1 is the energetic difference between the R1 line and the maximum of the 4A2 → 4T2 PLE band.20 The energetic difference Δ2 between 4T2 and 2E electronic manifolds, which determines the thermal occupation of the 4T2 energy level, can be obtained from the following relationship:21,22
where
S and ℏ
ω are the Huang–Rhys factor and effective phonon energy, respectively. The values of
S and ℏ
ω can be calculated using the following equations:
23 | 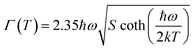 | (6) |
where Δ
E is the Stokes shift (energy difference between the first absorption and corresponding emission bands) and
Γ(
T) is the FWHM of the emission band at temperature
T. The
Δ2 values of Lu
3−xCa
xGa
5−xSi
xO
12:0.01Cr
3+ are calculated according to the above equations and the results are shown in
Fig. 3b. It can be seen that the values of
Δ2 decrease with increasing
x, indicating a higher probability of
2E →
4T
2 transition (
i.e. thermally populated
4T
2 state) which is consistent with the enhanced
4T
2 →
4A
2 broadband emission and increased FIR of
I(
4T
2)/
I(
2E) of Lu
3−xCa
xGa
5−xSi
xO
12:0.01Cr
3+ (as shown in
Fig. 2b and d).
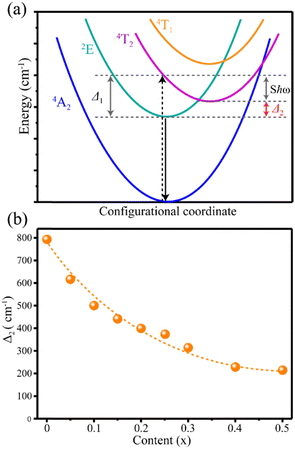 |
| Fig. 3 (a) Schematic configurational coordinate diagram and (b) energy difference between 4T2 and 2E states of Lu3−xCaxGa5−xSixO12:0.01Cr3+ (x = 0–0.5). | |
3.3. Thermal stability of Lu3−xCaxGa5−xSixO12:Cr3+
The PL intensity of phosphors usually shows a decreasing trend due to enhanced non-radiative transition with increasing temperature, which is a major obstacle for their application. Therefore, it is of great importance to develop NIR phosphors with improved thermal stability. In previous work,24 it is verified that the PL thermal stability of Cr3+ can be optimized by the electron occupation between 4T2 and 2E states. As discussed above, the energetic difference Δ2 and crystal field strength (Table 1) between 4T2 and 2E states (thermal population of the 4T2 state) of Lu3−xCaxGa5−xSixO12:0.01Cr3+ can be easily tuned by co-substitution of [Lu3+–Ga3+] by [Ca2+–Si4+], which may provide an alternative way for improving the thermal stability.
In order to evaluate the influence of [Ca2+–Si4+] co-substitution on thermal stability, the temperature-dependent PL spectra of Lu3−xCaxGa5−xSixO12:0.01Cr3+ (x = 0, 0.15 and 0.30) are studied in detail (Fig. 4 and Fig. S5, ESI†). On the one hand, it is noted that the integrated PL intensity of all Lu3−xCaxGa5−xSixO12:0.01Cr3+ (x = 0, 0.15 and 0.30) samples increases first and then decreases with increasing temperature (Fig. 4a–c). On the other hand, the thermal stability of Lu3−xCaxGa5−xSixO12:0.01Cr3+ becomes better and better with increasing x and the PL intensity at x = 0.30 reaches up to 125% and 121% at 425 K and 475 K compared with that at 300 K (Fig. 4c), respectively, which is much better than those of most Cr3+-activated broadband NIR phosphors (Table 2).
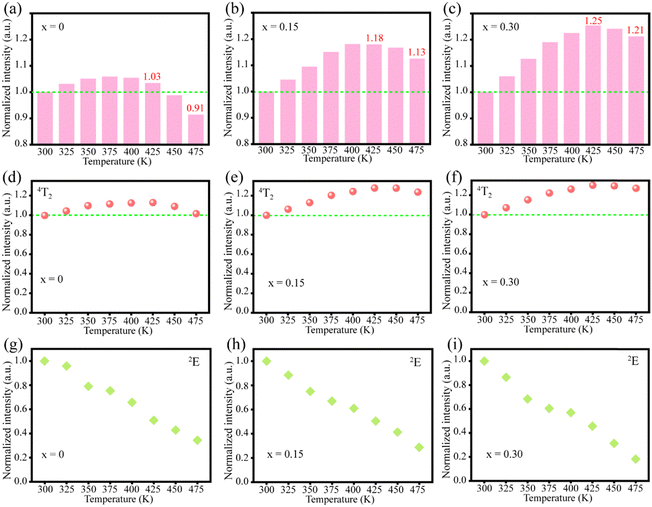 |
| Fig. 4 Normalized PL intensity of (a)–(c) 4T2 + 2E →4A2, (d)–(f) 4T2 → 4A2 and (g)–(i) 2E → 4A2 transitions of Lu3−xCaxGa5−xSixO12:0.01Cr3+ as a function of temperature (300–475 K) with x = 0, 0.15 and 0.30, respectively. | |
Table 2 Thermal stability of Cr3+-activated broadband NIR phosphors
Phosphors |
λ
em (nm) |
I
423K (%) |
Ref. |
Lu2.7Ca0.3Ga4.7Si0.3O12:Cr3+ |
748 |
125 |
This work |
Y3In2Ga3O12:Cr3+ |
760 |
100 |
25
|
Ca3Sc2Si3O12:Cr3+ |
770 |
97.4 |
26
|
GaTaO4:Cr3+ |
840 |
60 |
27
|
ScBO3:Cr3+ |
800 |
51 |
28
|
Na3Al2Li3F12:Cr3+ |
750 |
99 |
29
|
K2NaInF6:Cr3+ |
774 |
78.3 |
30
|
K2NaIScF6:Cr3+ |
765 |
89.6 |
31
|
ScF3:Cr3+ |
853 |
85.5 |
32
|
To understand the mechanism behind the abnormal thermal stability, the PL spectra are deconvoluted into separate 4T2 → 4A2 broadband and 2E → 4A2 sharp-line emissions for all temperatures.22 The PL intensity of the 4T2 → 4A2 broadband emission (Fig. 4d–f) exhibits a similar trend to the corresponding total PL intensity (Fig. 4a–c), while the 2E → 4A2 sharp-line emission (Fig. 4g–i) decreases monotonically with x. In the Lu3−xCaxGa5−xSixO12 system, the 2E state can serve as a reservoir for the higher lying 4T2 state.19 The electrons will migrate from the 2E to 4T2 energy level with increasing temperature and reach a new thermal equilibrium, leading to a thermally populated 4T2 state (which can be further verified by the decay curves in Fig. S6, ESI†). This explains why Lu3−xCaxGa5−xSixO12:0.01Cr3+ phosphors exhibit an abnormal thermal stability before 425 K. At higher temperatures, the 4T2→4A2 broadband emission dominates the PL spectra and then a traditional thermal quenching phenomenon is observed due to the increased non-radiative rate of the 4T2 level.
The improved thermal stability of Lu3−xCaxGa5−xSixO12:0.01Cr3+ (x = 0, 0.15 and 0.30) with increasing x is due to the different energetic difference Δ2 between 4T2 and 2E states which has a great influence on the initial thermal equilibrium between 4T2 and 2E energy levels (i.e. FIR of I(4T2)/I(2E)) and determines the thermal quenching performance at elevated temperatures. The PL intensity of 2E → 4A2 sharp-line emission is strong at large Δ2 values (small x), whose decrease has a great influence on the whole PL intensity with increasing temperature. Then the 2E → 4T2 transition strengthens with decreasing Δ2 (increasing x). Finally, the 2E → 4T2 transition has little influence on the thermal quenching performance due to the insufficient 2E electrons for smaller Δ2 values. That is why the thermal stability of Lu3−xCaxGa5−xSixO12:0.01Cr3+ decreases when x > 0.30 (Fig. S7, ESI†). In this work, it is obvious that Lu3−xCaxGa5−xSixO12:0.01Cr3+ phosphors have optimal Δ2 values and the best thermal stability when x = 0.30.
Considering the higher thermal stability (Fig. 3c) and PL intensity (Fig. S8, ESI†) of Lu3−xCaxGa5−xSixO12:0.01Cr3+ at x = 0.30, a series of Lu2.7Ca0.3Ga4.7Si0.3O12:Cr3+ with various Cr3+ concentrations were prepared (Fig. S9, ESI†) to further optimize the PL properties (Fig. 5a). The PL intensity of Lu2.7Ca0.3Ga4.7−xSi0.3O12:xCr3+ reaches its maximum value at x = 0.02 (Fig. S10, ESI†) and then decreases owing to the concentration quenching effect. Moreover, it is found that both the FWHM (from to 140 to 182 nm, Fig. 5b) and FIR (Fig. 5c) of I(4T2)/I(2E) show an increasing trend as the Cr3+ concentration increases, just like that of Lu3−xCaxGa5−xSixO12:0.01Cr3+ (Fig. 2b, d). But the reason for the increased 4T2 → 4A2 broadband emission with increasing Cr3+ content is different from that of Lu3−xCaxGa5−xSixO12:0.01Cr3+ (which is due to a decreased crystal field strength). In this case, the normalized PLE spectra of Lu2.7Ca0.3Ga4.7−xSi0.3O12:xCr3+ almost overlap with each other (Fig. S11 (ESI†), implying an unchanged crystal field strength). It is considered that the distance between Cr3+ and Cr3+ ions in Lu2.7Ca0.3Ga4.7−xSi0.3O12:xCr3+ decreases with increasing Cr3+ concentration, which is conducive to the electron migration from the 2E to 4T2 state, thus resulting in a thermally populated 4T2 state (i.e. enhanced 4T2 → 4A2 broadband emission) at higher Cr3+ content.24
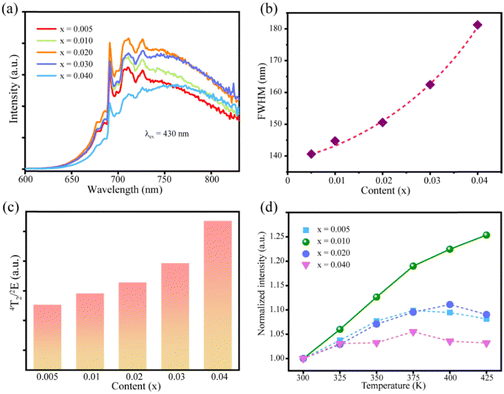 |
| Fig. 5 (a) Emission spectra, (b) FWHM and (c) FIR of 4T24A2 to 2E → 4A2 transitions of Lu2.7Ca0.3Ga4.7−xSi0.3O12:xCr3+ (x = 0.005–0.040). (d) Normalized PL intensity of Lu2.7Ca0.3Ga4.7−xSi0.3O12:xCr3+ as a function of temperature (300–425 K). | |
Due to the varying FIR values of I(4T2)/I(2E) of Lu2.7Ca0.3Ga4.7−xSi0.3O12:xCr3+, it is necessary to investigate the thermal stability. The temperature-dependent PL spectra of Lu2.7Ca0.3Ga4.7−xSi0.3O12:xCr3+ are measured and shown in Fig. 5d and Fig. S12 (ESI†). As expected, the thermal stability of Lu2.7Ca0.3Ga4.7−xSi0.3O12:xCr3+ becomes better before x = 0.010 and then decreases due to the gradually exhausting 2E electrons at higher Cr3+ concentration. From the obtained results, it can be inferred that both the Δ2 value and Cr3+ concentration have a significant influence on the thermal stability of Lu3−xCaxGa5−xSixO12:Cr3+.
3.4. NIR pc-LED applications
Considering the excellent thermal quenching behavior of the as-prepared samples, a NIR pc-LED was fabricated by combining a Lu2.7Ca0.3Ga4.7Si0.3O12:Cr3+ phosphor with a commercial blue InGaN chip and the electroluminescence spectrum is shown in Fig. S13 (ESI†). The NIR output power (Fig. 6a) increases monotonously from 50 to 350 mA, while the photoelectric conversion efficiency decreases slightly (Fig. 6b). The NIR light from the LED device can penetrate human fingers easily and the blood vessels can be observed clearly (Fig. 6c). In addition, with the aid of a NIR camera, the words on the bottle surface are clearly distinguished by using the packaged NIR pc-LED as the light source (Fig. 6d). The above results indicate that the as-prepared sample can be potentially used in the fields of bio-imaging and night vision.
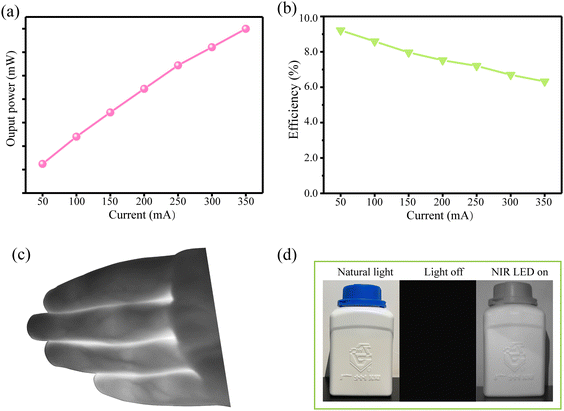 |
| Fig. 6 (a) Output power and (b) photoelectric conversion efficiency of the fabricated NIR-LED device as a function of driven current. (c) Photograph of fingers taken with the NIR camera under irradiation of the LED device. (d) Photographs of a bottle under natural light and NIR LED light captured with a visible camera (left and middle) and the NIR camera (right). | |
4. Conclusions
In summary, Lu3−xCaxGa5−xSixO12:Cr3+ phosphors with tunable PL properties and abnormal thermal stability were synthesized by a high-temperature solid-state method. The FWHM of the PL spectra of Lu3−xCaxGa5−xSixO12:Cr3+ broadens from 43 to 150 nm due to the enhanced 4T2 → 4A2 emission, resulting from a decreased crystal field strength. The calculated energetic difference (Δ2) between 4T2 and 2E states also shows a decreasing trend with increasing [Ca2+–Si4+] co-substitution, leading to a thermally populated 4T2 state. Based on the different thermal population of the 4T2 state, the thermal stability of Lu3−xCaxGa5−xSixO12:Cr3+ can be easily tuned. With the optimal [Ca2+–Si4+] co-substitution, Lu3−xCaxGa5−xSixO12:0.01Cr3+ (x = 0.30) shows an abnormal thermal quenching performance and the PL intensity can reach up to 125% and 121% at 425 K and 475 K compared with that at 300 K, respectively. In addition, it is found that the 4T2/2E thermal population and PL thermal stability of Cr3+ can be further optimized by the Cr3+ concentration. Finally, a NIR pc-LED based on the Lu3−xCaxGa5−xSixO12:Cr3+ phosphor shows excellent performance in bio-imaging and night vision.
Data availability
The data supporting this article have been included as part of the ESI.†
Conflicts of interest
There are no conflicts to declare.
Acknowledgements
This work has been financially supported by the National Natural Science Foundation of China (No. 52272143), the Major Science and Technology Project of Zhongshan City (No. 2022A1007), the Guangdong Basic and Applied Basic Research Foundation (No. 2023A1515010866 and 2021A1515110404), the Science and Technology Project of Guangzhou City (No. 2023A04J0299), the GDAS’ Project of Science and Technology Development (No. 2022GDASZH-2022010104, 2023GDASZH-2023010104 and 2024GDASZH-2024010102), and Young Talent Project of GDAS (No. 2023GDASQNRC-0302).
Notes and references
- R.-J. Xie, Light-emitting diodes: brighter NIR-emitting phosphor making light sources smarter, Light: Sci. Appl., 2020, 9, 155 CrossRef PubMed.
- M. H. Fang, Z. Bao, W. T. Huang and R. S. Liu, Evolutionary generation of phosphor materials and their progress in future applications for light-emitting diodes, Chem. Rev., 2022, 122, 11474–11513 CrossRef CAS.
- G. N. A. De Guzman, M.-H. Fang, C.-H. Liang, Z. Bao, S.-F. Hu and R.-S. Liu, [INVITED] Near-infrared phosphors and their full potential: a review on practical applications and future perspectives, J. Lumin., 2020, 219, 116944 CrossRef CAS.
- M. H. Fang, K. C. Chen, N. Majewska, T. Lesniewski, S. Mahlik, G. Leniec, S. M. Kaczmarek, C. W. Yang, K. M. Lu, H. S. Sheu and R. S. Liu, Hidden structural evolution and bond valence control in near-infrared phosphors for light-emitting diodes, ACS Energy Lett., 2021, 6, 109–114 CrossRef CAS.
- J. Feng, H. Liu, Z. Ma, J. Feng, L. Chen, J. Li, Y. Cai, Q. Zeng, D. Wen and Y. Guo, A super stable near-infrared garnet phosphor resistant to thermal quenching, thermal degradation and hydrolysis, Chem. Eng. J., 2022, 449, 137892 CrossRef CAS.
- G. Liu and Z. Xia, Modulation of thermally stable photoluminescence in Cr3+-based near-infrared phosphors, J. Phys. Chem. Lett., 2022, 13, 5001–5008 CrossRef CAS.
- J. M. Xiang, J. M. Zheng, X. Q. Zhao, X. Zhou, C. H. Chen, M. K. Jin and C. F. Guo, Synthesis of broadband NIR garnet phosphor Ca4ZrGe3O12:Cr3+,Yb3+ for NIR pc-LED applications, Mater. Chem. Front., 2022, 6, 440–449 RSC.
- H. Xiao, J. Zhang, L. Zhang, H. Wu, H. Wu, G. Pan, F. Liu and J. Zhang, Cr3+ activated garnet phosphor with efficient blue to far-red conversion for pc-LED, Adv. Opt. Mater., 2021, 9, 2101134 CrossRef CAS.
- J. Zhong, Y. Zhuo, F. Du, H. Zhang, W. Zhao and J. Brgoch, Efficient and tunable luminescence in Ga2-xInxO3:Cr3+ for near-infrared imaging, ACS Appl. Mater. Interfaces, 2021, 13, 31835–31842 CrossRef CAS.
- F. He, E. Song, Y. Zhou, H. Ming, Z. Chen, J. Wu, P. Shao, X. Yang, Z. Xia and Q. Zhang, A general ammonium salt assisted synthesis strategy for Cr3+-doped hexafluorides with highly efficient near infrared emissions, Adv. Funct. Mater., 2021, 31, 2103743 CrossRef CAS.
- S. Wang, R. Pang, T. Tan, H. Wu, Q. Wang, C. Li, S. Zhang, T. Tan, H. You and H. Zhang, Achieving high quantum efficiency broadband NIR Mg4Ta2O9:Cr3+ phosphor through lithium-Ion compensation, Adv. Mater., 2023, 35, 2300124 CrossRef CAS.
- X. Zou, X. Wang, H. Zhang, Y. Kang, X. Yang, X. Zhang, M. S. Molokeev and B. Lei, A highly efficient and suitable spectral profile Cr3+-doped garnet near-infrared emitting phosphor for regulating photomorphogenesis of plants, Chem. Eng. J., 2022, 428, 132003 CrossRef CAS.
- R. Li, Y. Liu, C. Yuan, G. Leniec, L. Miao, P. Sun, Z. Liu, Z. Luo, R. Dong and J. Jiang, Thermally stable CaLu2Mg2Si3O12:Cr3+ phosphors for NIR LEDs, Adv. Opt. Mater., 2021, 9, 2100388 CrossRef CAS.
- E. T. Basore, W. Xiao, X. Liu, J. Wu and J. Qiu, Broadband near-infrared garnet phosphors with near-unity internal quantum efficiency, Adv. Opt. Mater., 2020, 8, 2000296 CrossRef CAS.
- M. Mao, T. Zhou, H. Zeng, L. Wang, F. Huang, X. Tang and R.-J. Xie, Broadband near-infrared (NIR) emission realized by the crystal-field engineering of Y3−xCaxAl5−xSixO12:Cr3+ (x = 0-2.0) garnet phosphors, J. Mater. Chem. C, 2020, 8, 1981–1988 RSC.
- F. Chi, W. Dai, L. Qiu, S. Liu, X. Wei, Y. Chen and M. Yin, Photoluminescence properties of far-red emitting Lu2CaAl4GeO12:Cr3+ garnet phosphor, J. Lumin., 2022, 251, 119198 CrossRef CAS.
- H. J. Yu, J. Chen, R. Y. Mi, J. Y. Yang and Y. G. Liu, Broadband near-infrared emission of K3ScF6:Cr3+ phosphors for night vision imaging system sources, Chem. Eng. J., 2021, 417, 129271 CrossRef CAS.
- J. M. Xiang, X. Zhou, X. Q. Zhao, Z. Y. Wu, C. H. Chen, X. J. Zhou and C. F. Guo, Ab Initio site-selective occupancy and luminescence enhancement in broadband nir emitting phosphor Mg7Ga2GeO12:Cr3+, Laser Photonics Rev., 2023, 17, 2200965 CrossRef CAS.
- B. Malysa, A. Meijerink and T. Jüstel, Temperature dependent luminescence Cr3+-doped GdAl3(BO3)4 and YAl3(BO3)4, J. Lumin., 2016, 171, 246–253 CrossRef CAS.
- M.-H. Fang, G. N. A. De Guzman, Z. Bao, N. Majewska, S. Mahlik, M. Grinberg, G. Leniec, S. M. Kaczmarek, C.-W. Yang, K.-M. Lu, H.-S. Sheu, S.-F. Hu and R.-S. Liu, Ultra-high-efficiency near-infrared Ga2O3:Cr3+ phosphor and controlling of phytochrome, J. Mater. Chem. C, 2020, 8, 11013–11017 RSC.
- M. Back, J. Ueda, M. G. Brik, T. Lesniewski, M. Grinberg and S. Tanabe, Revisiting Cr3+-doped Bi2Ga4O9 spectroscopy: crystal field effect and optical thermometric behavior of near-infrared-emitting singly-activated phosphors, ACS Appl. Mater. Interfaces, 2018, 10, 41512–41524 CrossRef CAS PubMed.
- M.-H. Fang, T.-Y. Li, W.-T. Huang, C.-L. Cheng, Z. Bao, N. Majewska, S. Mahlik, C.-W. Yang, K.-M. Lu, G. Leniec, S. M. Kaczmarek, H.-S. Sheu and R.-S. Liu, Surface-protected high-efficiency nanophosphors via space-limited ship-in-a-bottle synthesis for broadband near-infrared mini-light-emitting diodes, ACS Energy Lett., 2021, 6, 659–664 CrossRef CAS.
- M. G. Brik and N. M. Avram, Crystal Field Analysis and electron-phonon coupling in Sc2O3:Cr3+, Z. Naturforsch., A: Phys. Sci., 2014, 56, 799–803 Search PubMed.
- Q. Zhang, X. Wei, J. Zhou, B. Milićević, L. Lin, J. Huo, J. Li, H. Ni and Z. Xia, Thermal stability improvement of Cr3+-activated broadband near-infrared phosphors via state population optimization, Adv. Opt. Mater., 2023, 11, 2300310 CrossRef CAS.
- C. Li and J. Zhong, Highly efficient broadband near-infrared luminescence with zero-thermal-quenching in garnet Y3In2Ga3O12:Cr3+ phosphors, Chem. Mater., 2022, 34, 8418–8426 CrossRef CAS.
- Z. Jia, C. Yuan, Y. Liu, X.-J. Wang, P. Sun, L. Wang, H. Jiang and J. Jiang, Strategies to approach high performance in Cr3+-doped phosphors for high-power NIR-LED light sources, Light: Sci. Appl., 2020, 9, 86 CrossRef CAS.
- J. Y. Zhong, Y. Zhuo, F. Du, H. S. Zhang, W. R. Zhao, S. H. You and J. Brgoch, Efficient broadband near-infrared emission in the GaTaO4:Cr3+ phosphor, Adv. Opt. Mater., 2022, 10, 2101800 CrossRef CAS.
- M.-H. Fang, P.-Y. Huang, Z. Bao, N. Majewska, T. Leśniewski, S. Mahlik, M. Grinberg, G. Leniec, S. M. Kaczmarek, C.-W. Yang, K.-M. Lu, H.-S. Sheu and R.-S. Liu, Penetrating biological tissue using light-emitting diodes with a highly efficient near-infrared ScBO3:Cr3+ phosphor, Chem. Mater., 2020, 32, 2166–2171 CrossRef CAS.
- D. Huang, S. Liang, D. Chen, J. Hu, K. Xu and H. Zhu, An efficient garnet-structured Na3Al2Li3F12:Cr3+ phosphor with excellent photoluminescence thermal stability for near-infrared LEDs, Chem. Eng. J., 2021, 426, 131332 CrossRef CAS.
- Z. Wu, X. Han, Y. Zhou, K. Xing, S. Cao, L. Chen, R. Zeng, J. Zhao and B. Zou, Efficient broadband near-infrared luminescence of Cr3+ doped fluoride K2NaInF6 and its NIR-LED application toward veins imaging, Chem. Eng. J., 2022, 427, 131740 CrossRef CAS.
- E. Song, H. Ming, Y. Zhou, F. He, J. Wu, Z. Xia and Q. Zhang, Cr3+-Doped Sc-Based fluoride enabling highly efficient near infrared luminescence: a case study of K2NaScF6:Cr3+, Laser Photonics Rev., 2020, 15, 2000410 CrossRef.
- Q. Lin, Q. Wang, M. Liao, M. Xiong, X. Feng, X. Zhang, H. Dong, D. Zhu, F. Wu and Z. Mu, Trivalent chromium ions doped fluorides with both broad emission bandwidth and excellent luminescence thermal stability, ACS Appl. Mater. Interfaces, 2021, 13, 18274–18282 CrossRef CAS.
Footnotes |
† Electronic supplementary information (ESI) available: The excitation spectra, FWHM, decay curves and temperature-dependent spectra of Lu3−xCaxGa5−xSixO12:Cr3+; XRD patterns, PL intensity, PLE and temperature-dependent spectra of Lu2.7Ca0.3Ga4.7−xSi0.3O12:xCr3+ (x = 0.005–0.040); electroluminescence spectrum of the LED device. See DOI: https://doi.org/10.1039/d4qm00395k |
‡ Q. J. Zhu and J. S. Huo contributed equally to this work. |
|
This journal is © the Partner Organisations 2024 |
Click here to see how this site uses Cookies. View our privacy policy here.