DOI:
10.1039/D4QI01732C
(Review Article)
Inorg. Chem. Front., 2024,
11, 6275-6306
Metal–organic framework derived micro-/nano-materials: precise synthesis and clean energy applications
Received
10th July 2024
, Accepted 13th August 2024
First published on 15th August 2024
Abstract
Metal–organic frameworks (MOFs) and their derived materials have emerged as leading contenders in the realm of advanced materials science, heralded for their versatility, high surface areas, and tunable properties. These materials distinguish themselves through their exceptional ability to be tailored during synthesis, offering precise control over their morphology and functionalities. The derivation process from MOFs not only preserves their inherent large surface areas but also enhances their electrical conductivity and stability. Herein, we summarize the different synthetic strategies of MOF-derived micro-/nano-materials to date, including but not limited to calcining, phosphating, sulfurization, the selenylation method, ion exchange, and etching strategies. Recent progress in MOF-derived micro-/nano-nanomaterials for various applications including supercapacitors, metal-ion batteries, Li–S batteries, metal–air batteries, hydrogen evolution reaction, oxygen evolution reaction and oxygen reduction reaction is reviewed. Through detailed summary of these applications and the innovative approaches for their synthesis, this work highlights the utility and potential that MOF-derived materials hold. Concluding with a discussion on the challenges and future prospects of these materials, we underscore their transformative potential in advancing materials science and technology.
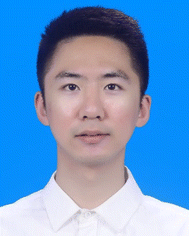 Guangxun Zhang | Guangxun Zhang is now a graduate student under Professor Pang's supervision, at the Yangzhou University of Chemistry and Chemical Engineering, China. His research mainly focuses on multifunctional materials based on metal–organic frameworks and their applications in electrochemistry. |
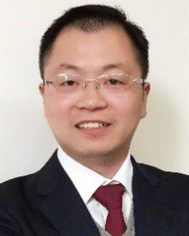 Huan Pang | Huan Pang received his Ph.D. degree from Nanjing University in 2011. He is now a distinguished professor at Yangzhou University, a Young Changjiang Scholar of the Ministry of Education in China, and a senior member of the Chinese Chemical Society. He is the managing editor of EnergyChem, an editorial board member of FlatChem and Rare Metals, and youth editorial board member of Nano Res, Nano Res Energy, and eScience among other distinguished academic journals. He was recognized as a highly cited researcher in Cross-Field by Clarivate Analytics in 2020 to 2022. His research area mainly focuses on MOF-related materials. |
1 Introduction
Currently, the world is grappling with a pronounced energy deficit, a concern that has attracted attention from many.1 At present, many countries and regions are confronting significant energy dilemmas, amplified by the relentless consumption of non-renewable resources.2 In response, there is a concerted effort among researchers to pioneer sustainable, eco-conscious energy alternatives. This pursuit of green energy sources represents a proactive approach to not only mitigating the impending energy shortage but also steering the global community towards a more sustainable and environmentally harmonious future.3,4 In the quest for rational and efficient energy acquisition and storage, nanomaterials have emerged as a focal point of scholarly interest.5,6 Among these, micro-nano materials, defined as materials possessing at least one dimension within the nanoscale (1–100 nm) or constructed from such dimensions as their fundamental units, stand out for their remarkable attributes.7 Nanomaterials, in particular, are renowned for their exceptional capabilities in the realm of new energy, including the efficient absorption of solar energy and superior energy storage performance.8
Metal–organic frameworks (MOFs) are important multifunctional materials, which are celebrated for their myriad benefits: extensive surface area, and adjustable pore sizes and structures, along with the potential for pre- and post-synthesis structural modifications.9 The modularity of MOFs, achievable through alterations in the orderly arrangement of metal clusters and organic linkers containing carbon, underscores their versatility.10 Furthermore, MOFs serve as an exemplary precursor for crafting materials pivotal in electrochemical energy storage and conversion.11,12 Their unique pore architecture lends itself advantageously to gas adsorption and separation processes.13,14 Moreover, the adjustable crystal structure of MOFs provides an ideal platform for delving into the relationship between material structure and performance, thereby holding significant promise both in fundamental research and practical applications.15 Despite their myriad strengths, MOFs exhibit certain limitations, notably in conductivity and stability.16 Nonetheless, the distinct benefits of MOFs continue to garner attention from researchers worldwide, solidifying their distinguished position within the materials science arena. It is worth noting that MOF-derived materials not only preserve their intrinsic porous architecture and morphology but also exhibit remarkable stability and electrical conductivity.17,18 By undergoing calcination under various gas atmospheres, including oxidizing, inert, and reducing gases, MOF-derived materials can be synthesized with diverse properties and structures, leading to the creation of distinct derivatives.19,20 The annealing process facilitates the transformation of unsaturated metal-based active sites from metal atoms and the carbon carrier from organic ligands, owing to the MOF's composition of metal units and specialized organic ligands.21 This transformation enhances charge transfer efficiency, thereby significantly improving the electrochemical performances. With their superior conductivity, MOF-derived materials are excellently suited for use as electrode materials.22,23 A deeper comprehension of electron transport mechanisms within MOF-derived materials has revealed that their electrical conductivity can be finely tuned through the strategic design of organic ligands and metal sites or clusters.24 This insight builds upon the significant contributions of numerous distinguished scientists in the field of MOFs and MOF-derived materials. In applications such as environmental areas, the resistance of these materials to various solvents enhances their durability against corrosive substances in wastewater, facilitating efficient decomposition or adsorption.25 For energy storage and conversion, the high metal content and cost-effectiveness of catalytic active sites position MOF-derived materials as potential substitutes for precious metals, underscoring the critical role they play in catalysis.26 The unique pore structure of MOFs allows for more active sites and the adsorption of more impurities, while the special concave design of some MOFs supports effective mass and electron transport.27,28 Their extensive surface area and porosity further broaden their application spectrum. Because of the brittleness and interlayer interaction of the two-dimensional MOF, it is not easy to prepare high-quality MOF crystal monolayers. Researchers found a single layer of single crystals grown by autocoagulation-assisted chemical vapor deposition (poly [Fe(benzimidazole)]). The realization of highly selective ammonia sensing was achieved by the ultra-thin van der Waals heterostructure obtained by the growth of this MOF single crystal on MoS2.29 Diatomic catalysts have high catalytic activity and are widely used in sustainable energy conversion and storage. They are optimized for the adsorption–desorption of oxygen-containing intermediates, accelerate the chemical reaction kinetics of ORR and OER, and show excellent performance in zinc–air batteries.30 Hydrogen overflow is a special effect in heterogeneous catalysis and hydrogen storage processes. Hydrogen spillover in MOF can be regulated by functional groups or embedded water molecules to achieve long-distance stable movement of active hydrogen. Highly selective hydrogenation of heteroaromatic hydrocarbons was realized.31
In this review, the rational design of MOF-derived micro-/nano-materials to improve performance for energy conversion and storage is summarized in terms of compositional and construction aspects. MOF-derived materials ingeniously crafted through meticulously designed processes, reaction conditions, and choice of raw materials, have been tailored to exhibit specific morphologies and functionalities. The synthetic strategies of MOF-derived materials to date, including but not limited to calcining, phosphating, sulfurization, the selenylation method, ion exchange, and etching strategies are summarized.
Phosphating can create a porous amorphous structure with interconnections, hollow and abundant catalytic sites, and large specific surface area. Such a structure is more conducive to improving the electrochemical activity and promoting the development of the catalyst.32 Polymetallic phosphide has good conductivity, so the introduction of polymetallic phosphide into the MOF can solve the material problem, improve the electron transfer and accelerate the chemical reaction kinetics.33 Sulfuration can enable MOF materials to obtain excellent electrochemical activity and stability, which are conducive to the mutual transfer of electrons and ions, showing an obvious synergistic effect. In solving the problem of the shuttle effect in lithium batteries, researchers provided a solution strategy of using poly(ethylene oxide)-based electrolyte as a filler to regulate the movement of lithium ions and polysulfide transport. This strategy had high cycling stability at 60 °C and 0.5 °C.34 The appearance of selenide provided capacity density for the MOF, gave the material better Coulomb efficiency and cycling stability, and improved the electrochemical performance of the electrocatalyst.
Subsequent sections delve into the pivotal role played by MOF-derived materials in energy storage, particularly through the lens of supercapacitors and various battery technologies. This exploration underscores the potential of these materials to mitigate the contemporary energy crisis by facilitating efficient energy storage mechanisms. Furthermore, the discourse expands into the electrocatalytic applications of these materials, with a focus on hydrogen evolution and oxidation–reduction reactions, highlighting their contribution to sustainable energy solutions. Additionally, the review examines the utility of MOF-derived materials in adsorption and photocatalysis, revealing their practical significance in environmental remediation and renewable energy production, respectively. Concluding with a forward-looking perspective, the article identifies the challenges currently facing the development and application of MOF-derived materials while optimistically forecasting the future directions of this burgeoning field. The ongoing advancements and the innovative application of these materials underscore their transformative potential, paving the way for their increased integration into various sectors of materials science and engineering.
2 Controlled synthesis of MOF-derived materials
MOF-derived micro-/nano-materials have been investigated in the field of energy conversion and storage (Fig. 1 and 2). By controlled thermal or chemical treatment, MOFs/MOF composites can be converted into MOF-derived metal compounds, carbon, and metal/N/C composites that combine the advantageous features of metal/carbon (e.g. abundant active metal sites and desirable conductivity)35–37 and MOFs (e.g. high porosity/high surface area),38 offering great opportunities as electrodes for clean energy applications, and many breakthroughs have been made in this emerging field.29,39
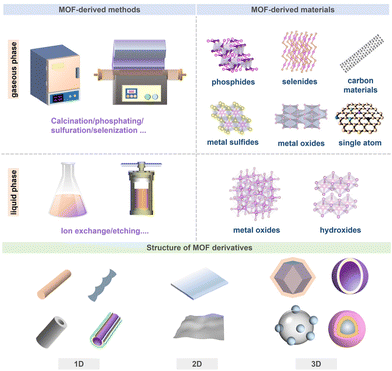 |
| Fig. 1 Schematic diagram of different derivation tactics, crystal structures, and morphologies of MOF-derived micro-/nano-materials. | |
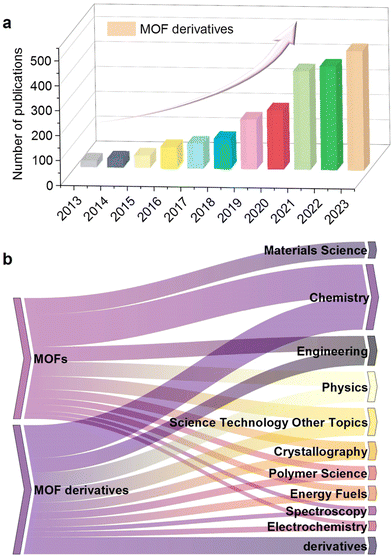 |
| Fig. 2 (a) The number of published papers on MOF-derived nanomaterials. (b) Fields of application of MOF derivatives (Data Source: Web of Science, 2013–2023). | |
2.1 Calcination of MOF materials
The format and preparation of MOFs as sacrificial precursors/templates has recently been considered a promising strategy used to prepare metal/carbon electrocatalysts with attractive architectures and outstanding properties.40 However, these electrocatalysts are hampered by aggregation due to poor electron conductivity and calcination. These issues need to be addressed soon. An in situ confined pyrolysis protocol was reported for the conversion of ZIF-67 nanocrystals on nitrogen-enriched carbon shell and hollow carbon spheres (HCSs) to cobalt, leading to the formation of hierarchical HCS@Co/NCs.41,42 Structurally, different metallic cobalt nanoparticles (NPs) were firmly anchored and dispersed in a network of nanocarbon shells, which not only enhanced conductivity but also reduced the aggregation of active sites. In addition, the unique concave design allowed for efficient mass transport and electronic transfer. The structural and elemental advantages of the HCS@Co/NC catalyst contributed to its exceptional oxygen reduction performance, exceeding the commercial Pt/C benchmark. Using novel synthesis methods, special layered Co3O4–Bi2O3 materials were prepared by the adsorption-calcination method using bismuth group as precursor. In the composite, the surface of Bi2O3 was loaded with Co3O4 particles, forming a particularly tight honeycomb-like structure.43 In experiments on dye degradation, the Co3O4–Bi2O3 composite demonstrated significantly superior activation performance of peroxymonosulfate (PMS) in RhB degradation compared with pure Co3O4 or Bi2O3. In addition, in the experiment, the Co3O4–Bi2O3/PMS system still maintained unique excellent catalytic performance over a wide pH range, so that it showed excellent performance in a series of azo dyes. In addition, its excellent chemical stability ensured perfect reusability in four unique tests. Electrochemical analysis of XPS showed that the activation capacity of Co3O4 nanoparticles was enhanced by Bi interaction.44 Moreover, the outcome of quenching experiments on radicals coupled with ESR analysis suggested that the primary functional species in the Co3O4–Bi2O3/PMS system were superoxide radicals and singlet oxygen. Additionally, a rational activation mechanism was proposed. For the removal of impurities, the application of MOFs to pollutants in high-level oxidation processes has attracted considerable attention. New MOF-derived catalysts for AOPs have not been well investigated. In one project, a stable and efficient active catalyst, peroxonosulfate (PMS), was prepared. The catalyst was prepared from vulcanized co-doped MOFs at various calcination temperatures (300–600 °C);45 this could effectively decrease the resistance of the charge transfer presence of Co(II) due to Co doping and vulcanization became more significant. This catalyst exhibited significantly enhanced Fischer–Tropsch synthesis (FTS) activity (high turnover frequency of 3.8 × 10−2 s−1 at 200 °C) and Cs+ selectivity (80.7%). Further density functional theory (DFT) calculations showed that the designed Ru/Zr dual sites effectively reduced the rate-limiting barriers during chain growth from C to Cs by weakening the C–O bond, thereby promoting chain growth and greatly improving FTS performance. The work demonstrates the effectiveness of dual-atomic-site design in enhancing FTS performance, and offers new opportunities for developing efficient industrial catalysts. Researchers designed a low-temperature thermal Ru Zr/Co catalyst with dual atomic sites of Ru and Zr on the surface of Co nanoparticles using a MOF synthesis strategy46 (Fig. 3a). The calcination method was used to adjust the electronic structure and increase the number of surface-active sites of the photocatalyst nano MIL-125(Ti)-250, while preserving its crystal morphology47 (Fig. 3b). The experimental findings revealed that this approach resulted in the presence of abundant Ti+ ions generated by oxygen vacancies and a high specific surface area of the photocatalyst. These features were found to enhance the adsorption and activation of N2 molecules during the process of photocatalytic nitrogen fixation. Furthermore, electrochemical tests indicated that a high Ti+/Ti+ atomic ratio improved carrier separation, leading to increased efficiency in photocatalytic N2 fixation. Overall, this study offered valuable insights into the development of novel photocatalysts for a variety of chemical reduction reactions. Progress in highly efficient catalysts is essential for the activation of peroxymonosulphate (PMS).
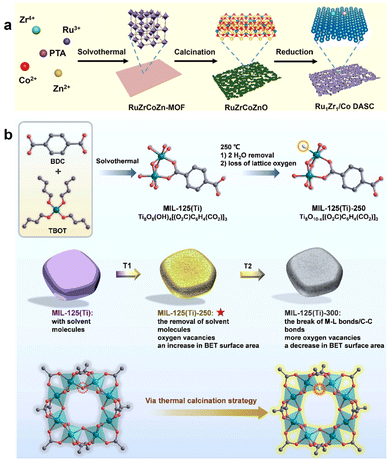 |
| Fig. 3 (a) Schematic illustration of the MOF-mediated strategy to synthesize Ru1Zr1/Co DASC. (b) The preparation processes of MIL-125(Ti) via the solvothermal method and followed by thermal calcination. For clarity, H atoms are omitted. BDC: terephthalic acid. TBOT: tetra-butyl ortho-titanate. T1 and T2 stand for the increasing calcination temperature. The O from Ti–OH–Ti in Ti8O8(OH)4 clusters is removed (red circle). Ti: green, C: gray, O: red, white O: oxygen vacancy. (a) Reproduced with permission. Copyright 2023,46 American Chemical Social. (b) Reproduced with permission.47 Copyright 2023, Wiley. | |
Fe–N–C catalysts exhibited excellent PMS activation properties due to the contribution of doped N, Fe–Nx and Fe3C sites.48 In one work, a series of high-performance Fe–N–C catalysts was obtained by pyrolysis of Fe–Zn-MOF precursors.49 The variation in the formation of active sites in the precursor and the chemical bonds during the pyrolysis process was explained by means of characterization analysis and relevant catalytic experiments.50 Fe–Nx, Fe3C and graphitic N were found to synergistically activate PMS for the degradation of ciprofloxacin (CIP). Furthermore, the catalytic effect was directly related to the calcination temperature and doped iron. In addition, the Fe–N–C-3-800/PMS system had good interference suppression capability and good recovery performance. Through a combination of quenching experiments and electron paramagnetic resonance (EPR), a reaction pathway based on non-radical O2 was proposed. Moreover, PMS had the capability to react with Fe–N–C-3-800 and form an intermediate that facilitated charge transfer. Therefore, accelerating the electron transfer between PMS and CIP was crucial for achieving the degradation of CIP. Subsequently, six primary pathways for CIP degradation were suggested. These included direct oxidation of CIP and cleavage of the N–C bond on the piperazine ring. These studies provided new ideas in developing heterogeneous carbon catalysts for advanced oxidation applications. A mixture of Ni-MOF and Cu-MOF (mass ratio 6
:
4) was calcined under the protection of N2 to obtain magnetic Ni and Cu bimetallic particles embedded in carbon sheets C@CueNi. The degradation of 2,4,6-trichlorophenol (2,4,6-TCP) by peroxonosulfate (PMS) was catalyzed by it.51 This adequately confirmed that C@CueNi had supreme catalytic performance for PMS activation.52 Electron paramagnetic resonance (EPR) analysis and reactive oxygen species (ROS) quenching showed ROS to be composed of –OH, SO4, O2 and singlet oxygen (O2), and this was the major contributor to the rapid degradation of 2,4,6-TCP. Among these, 1O2 and O2˙− were crucial. Electrochemical impedance spectroscopy (EIS) revealed that the C@CueNi material had improved electron transport and electrical conductivity, hence increasing its catalytic activity. C@CueNi, on the other hand, demonstrated exceptional stability and could be employed five times in a row without deterioration in catalytic efficacy. High-performance liquid chromatography-mass spectrometry (HPLC-MS/MS) was used to examine the major intermediates of 2,4,6-TCP degradation, and potential routes of 2,4,6-TCP degradation were postulated in a single step. The remarkable stability and superior catalytic activity of C@Cu–Ni, as well as the ease of separation from wastewater through magnetization, indicated that the newly synthesized material could provide a potential alternative route for highly efficient decomposition of organic contaminants.53,54 In a variety of portable energy storage systems, supercapacitors are chosen because of their good cycling stability, high power density, fast charging speed, and stable portable electrochemical energy storage systems.55 Researchers suggested a MOF founded on self-templating for the creation and composition of nanoporous carbon nanostructures consisting of bimetallic oxide (Ni–Fe–O/NPC) on carbon nanofibres possessing porosity56 (Fig. 4a). Ni–Fe–O/NPC helped to improve the overall electrochemical performance and accelerated the redox reaction rate. Due to their upright alignment on the PCNFs, the paths of ion/electrode disseminated at the electrode–electrolyte interface were shortened. As an independent electrode of the supercapacitor, the cycling life was good, and it has great research value.
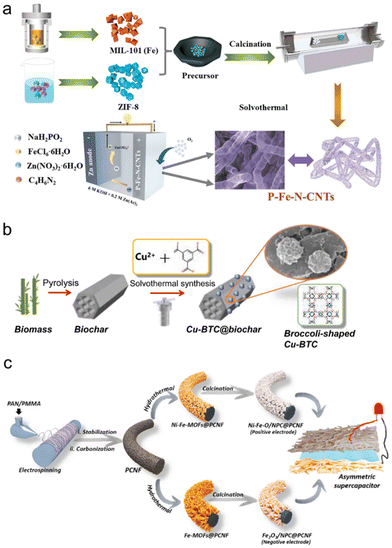 |
| Fig. 4 (a) Schematic diagram of the synthesis procedure of expanded P–Fe–N-CNTs. (b) Schematic representation of the synthesis of Cu-BTC @biochar. (c) Schematic representation of fabrication of electrode materials synthesis for ASC device. (A color version of this figure can be viewed online.) (a) Reproduced with permission.56 Copyright 2023, Elsevier. (b) Reproduced with permission.57 Copyright 2020, Elsevier. (c) Reproduced with permission.58 Copyright 2023, Elsevier. | |
Coal is easy to mine, transport and store, and it has a stable price advantage. Coal-fired power is the main form of electricity generation in many countries. But it is not a green energy source, and the volatile organic compounds and flue gases produced by coal combustion will inevitably affect the environment and human health. To solve the problem, the MOF biochar complex Cu-BTB-loaded biochar material (Cu-BTC@biochar) was selected to adsorb flue gas pollutants. CU-BTC@biochar with adsorption was synthesized by using a simple pot of water. Biochar was the precursor (Fig. 4b). It had excellent performance for adsorption of toluene as volatile organic matter, at medium and high temperatures. Compared with the original biochar, its BET surface area was significantly increased, and it had more layered pore structure characteristics, which could accommodate more adsorbed matter. The results showed that CU-BTC@biochar had better adsorption capacity at increasing temperature, but it had higher and better adsorption capacity at 60–150 °C. The experiment showed that the van der Waals interaction force played an important role in the adsorption process of toluene, and the electrostatic interaction force of CU-BTC@biochar was significantly higher than that of CU-BTC. Porous nanomaterials have many applications: they can be used as catalysts, adsorbents, electrolytic materials, supercapacitors and so on. However, it is difficult to study new vertical structures for high-performance energy storage electrode materials, but it is not impossible. In order to increase the overall electrochemical properties of the material and thus accelerate the redox reaction rate, a new material has been suggested for the creation and combination of porous carbon-supported bimetallic oxides/nanoporous carbon nanostructures (Ni–Fe–O/NPC) on porous carbonaceous nanofiber materials (PCNFs) (Fig. 4c).58 Using this particular structure, the diffusion path of ions/electrodes at the interface between the electrode and electrolyte can be reduced, leading to enhanced electrochemical performance.59 Experiments showed that it had a high specific capacitance, capacitor retention after many cycles was still good, it had a very good cycling life, and in a high-power environment, the energy density could have a very high value and a very high magnification. It has a broad application prospect. Tungsten disulfide (WS2) is well known for its remarkable electrocatalytic capacity. However, its semiconductor properties and intrinsic inertness hinder its practical application. One study demonstrated the effective inhibition of WS2 growth via a Co-based zeolite imidazole framework (ZIF-67); few-layered WS2 was limited to the surface of Co, N-doped carbon polyhedron (WS2@Co9S8), with higher conductivity and more marginal active sites (Fig. 5a).60 First, WS2@Co9S8 was fabricated by mixing in a liquid phase pot and then calcination. WS2 achieved a uniform distribution on the surface of the polyhedron through electrostatic adsorption in the liquid phase. The increase in catalytic activity could be attributed to the higher presence of sulfur edge sites due to WS2, the specific mutually beneficial effect of Co9S8 and WS2 on the MOF surface, and the effective reduction of the diffusion path through the hollow multi-channel structure (Fig. 5e and f). Therefore, the stable catalyst (WS2@Co9S8) that was created using a simple and effective synthesis method in this study will act as a promising bifunctional catalyst.
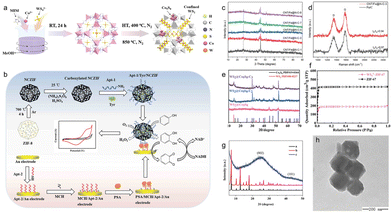 |
| Fig. 5 (a) Schematic illustration of the synthesis process of WS2@Co9S8. (b) Schematic diagram of the synthetic process and sensing application of the NCZIF conjugated with PSA aptamer and tyrosinase-triggered redox cycling system. (c) XRD spectrum of the CNT/Fe@N–C-x prepared with different mass ratio of melamine and MIL-101(Fe) (d). The Raman spectra of CNT/Fe@N–C-3 and Fe/C. (e) XRD patterns of WS2@Co9S8, WS2@Co9S8-C2, and WS2@Co9S8-C3. (f) N2 adsorption–desorption isotherms of ZIF-67 and WS42-ZIF-67. (g) PXRD patterns of simulated ZIF-8, synthesized ZIF-8, and NCZIF. (h) TEM images of DNA-1/Tyr/NCZIF (a, e and f) Reproduced with permission.60 Copyright 2022, American Chemical Society. (b, g and h) Reproduced with permission.61 Copyright 2021, Springer. (c and d) Reproduced with permission.62 Copyright 2022, Elsevier. | |
To improve the efficiency of aptasensors, a method of amplifying signals has been proposed. This approach combines the redox cycling triggered by tyrosinase (Tyr) with nanoscale porous carbon (NCZIF) synthesized by heating ZIF-8 crystals under an inert atmosphere. NCZIF was successfully obtained (Fig. 5b). The material had large surface area, excellent biocompatibility, and beneficial function, allowing the successful binding of sufficient Tyr and aptamer together. When targeting prostate specific antigen (PSA), the sandwich structure of NCZIF following Tyr functionalization is similar to an aptamer-modified gold electrode61 (Fig. 5g and h). Next, Tyr's function is to change the electroinactive phenol in the substrate to electroactive catechol, leading to redox cycling triggered by NADH, as well as a low-background signal. The use of a redox cycling system triggered by tyrosine, derived from ZIF-8, and porous carbon has provided a promising way to develop reliable, quick, easy, and low-background aptasensing methods. These methods show great potential in the fields of biomedicine and disease diagnosis.
Magnetic CNTs coated with nitrogen-doped carbon (CNT/Fe@N–C) were obtained by heating melamine and MIL-101(Fe) under an inert atmosphere at 900 °C.63 Melamine/mil-101 derivatives of CNT/Fe@N–C with different mass ratios of N–C were tested to study the effects of the adsorbent in successfully removing organic dye.64,65 The results showed that the prepared CNT/Fe@N–C had an excellent performance in the removal of organic dyes (malachite green, methyl orange and rhodamine B) at a 3
:
1 mass ratio of melamine and MIL-101(Fe).62 Experimental elements including pH, contact time, adsorbent amount and initial organic dye concentration were explored. The pseudo-second-order model and the Langmuir isotherm model were applied to the adsorption process of the three dyes on the CNT/Fe@N–C material. The findings proved that adsorption would be a single-layer chemisorption process to some extent. The p–p stacking interaction and electrostatic interactions may be responsible for the detailed adsorption mechanism of organic dyes on this sorbent material (Fig. 5c and d). Finally, CNT/Fe@N–C material that was regenerated five times still had a significantly efficient removal rate. The results showed that CNT/Fe@N–C was an excellent and promising organic dye adsorbent.66 In another study, CNT/Metal@N-doped carbon material with outstanding adsorption properties was prepared by calcination of the MOF complex and melamine using nitrogen-free MOF as precursor. A Prussian blue analogue (CuCo-PBA) was annealed under an argon environment to transform CuCo nanocube/N-doped carbon nanotube (CuCo/NCNT) derivatives, and the Prussian blue analogue (CuCo-PBA) could be synthesized by a simple co-precipitation method.67 Its special porous cubic structure, special three-dimensional conductive network built on the basis of carbon nanotubes and the non-uniform interface of Cu/Co/C enhanced interface polarization made this material particularly good for microwave absorption. Graphene-based aerogels (NiCo/C/CNT/RGO-based multidimensional graphene aerogels) decorated with metal–organic frames were considered to be a convenient and simple way to solve the electromagnetic pollution problem.68 Their absorption strength was excellent at a thickness of 1.8 mm.69,70 Due to their special hierarchical structure, they have a non-uniform interface structure, abundant defects and unique and excellent multi-dimensional gradient structure. Derived carbon materials that restrict metal ions were considered as a promising synthetic strategy to address the confinement of metal ions in MOFs. Metal ions of different valence states were confined to the cobalt benzimidazole framework in the shape of a quasi-microcube by using a spatial restriction strategy. A series of metal ion-derived carbon materials was obtained by high-temperature pyrolysis.71 They exhibited special double layer and pseudocapacitance properties due to the presence of metal ions of different valence states, which could accelerate the insertion/extraction of Nat, thus increasing their special electrochemical adsorption. Titanium-containing materials have impressively high cycling stability for desalting capabilities in capacitive deion applications. The MOF has been identified as an ideal template for the synthesis of metal heteroatom co-doped carbon materials. However, the slow process of heteroatom doping is an obstacle to the maximization of catalytic performance. Researchers created a series of highly efficient carbon-based composites co-doped with Co and N, P heteroatoms. First, a new in situ P-doped MOF was constructed with larger 2-methylimidazole and N, P-containing ligands as the mixed ligands. The MOFs were then calcined at extremely high temperatures. The gases generated by the thermal disintegration of the organic ligands were released from the interior of the P-ZIF materials during the pyrolysis process, resulting in the reduction of the Co-Co2P@NC-P catalysts.72 Researchers synthesized bimetallic organic frameworks (MOF: Ni-ZIF-8) by doping nickel metal elements of different weights, using organic substances as ligands and nanometallic oxides as precursors, and employing hydrothermal and facile uniform co-precipitation as preparation methods.73 A series of ZnO–MOF nanostructures (9% Ni–ZnO, 7% Ni–ZnO, 5% Ni–ZnO, 3% Ni–ZnO, 1% Ni–ZnO, pure MOF–ZnO) was realized after calcination. The surface electronic, structural and morphological features were characterized by EIS, XPS, UV-Vis, BET, SEM and XRD. By employing the WS-30A measurement system, the gas-sensitive performances of as-prepared materials were investigated and studied, and the influence of nickel dopant was discussed. Alternative energy sources are required to maintain sustainable standards for atmospheric greenhouse gases while meeting global energy demand. The hydrolysis of NaBH4 to H2 is widely regarded as a convenient and straightforward method of storing and releasing H2. Using large pores and free amine group (NH2) in NH2-UIO-66 MOF, a real-time anion exchange technique was used to encapsulate monometallic Co and Ru nanoclusters and ultra-small bimetallic Ru–Co alloy nanoclusters with an average particle size of 1.5 nm. To investigate the role played by NH2 groups and MOF pores in the formation of uniformly dispersed ruthenium and cobalt nanoclusters, the researchers continuously calcined NH2-UO-66 in air for 3 h at 600 °C to prepare N-doped carbonaceous ZrO2 (ZrO2N@C) nanocomposites.74 Hydrazine hydrate (HH) was prepared by microwave irradiation (MWI). These materials were used as new catalysts for the hydrolysis of sodium borohydride (NaBH4) to produce hydrogen. The discovered catalyst had excellent catalytic activity due to the small particle sizes of Co and Ru nanoclusters in the MOF pores, the co-coordination of Co and Ru nanoclusters in the alloy morphology, and the large surface area of NH2-UiO-66.75 The researchers studied the influence of the catalyst on the reaction in terms of dosage, NaBH4 concentration, reaction temperature (30–60 °C) and so on. It was found that the alloy nanoclusters had strong leaching resistance in the MOF matrix, and the catalyst had a high recovery efficiency of more than 4 times. These newly discovered catalysts could be classified as highly efficient energy catalysts.
The development of high-performance gas sensors utilizing MOF sensing materials based on MOFs has proved to be challenging due to structural damage incurred during the annealing process. In a study, researchers utilized a solvothermal reaction and cation exchange to produce mesoporous In2O3–NiO hollow spheres from nanosheets, overcoming this challenge (Fig. 6b and c).76 In addition, damage to the hierarchical structure and porous reticular skeleton of Ni-MOF during calcination could be avoided by transforming Ni-MOF into In/Ni-MOF by exchanging Ni2+ ions for In3+ ions (Fig. 6a). Thus, the large specific surface area and high porosity of the mesoporous In2O3–NiO hollow composites facilitated the creation of sufficient permeability pathways for VOC molecules to enhance VOC capture capability and maximize active site exploitation. These effects can be attributed to the p–n junction of In2O3–NiO and the hierarchical structure of the mesoporous hollow structure. The preparation method described in this study could provide a versatile platform applicable to a range of mesoporous hollow structures based on composite sensing materials. Materials derived from MOFs have gained increasing attention in the field of energy storage and conversion. The carbonization of MOFs is a commonly used method for obtaining MOF-derived materials (Fig. 6d). Nonetheless, contemporary calcination conditions frequently lead to a noticeable reduction in the specific surface area and structural breakdown. One study utilizes an argon–hydrogen gas mixture during MOF calcination to produce an N-doped porous carbon material with exceptional properties (Fig. 6e–i).77 Hydrogen reduced the evaporation of zinc in ZIF-8 at high temperatures, preventing the carbothermic reduction reaction between the carbon framework of the MOFs and the zinc content.78 This protection preserved the framework of the MOFs, leaving a significant number of micropores. As a result, after carbonization the MOFs retained an extremely high pore volume and specific surface area, exceeding that of the MOF precursors.
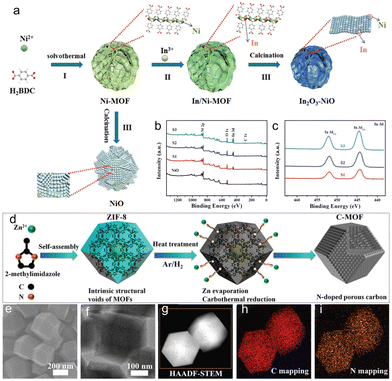 |
| Fig. 6 (a) Schematic diagram of MOF-derived NiO and In2O3–NiO. SEM images of P3-ZIF and CoeCo2P@NC-P3-700 catalyst (b and c). XPS spectra of NiO and In2O3–NiO (S1, S2, S3): (b) survey scan, (c) In 3d. (d) Illustration of processes and mechanism for the preparation of three-dimensional nitrogen-doped porous carbon. (e–i) SEM, TEM, and HAADF-STEM images of C-MOF-900 and the corresponding C- and N-elemental mappings, respectively. (a–c) Reproduced with permission.76 Copyright 2021 American Chemical Society. (d–i) Reproduced with permission.77 Copyright 2020 American Chemical Society. | |
2.2. Phosphating of MOF materials
Because of their numerous catalytic active sites, interconnected porous structures and amorphous materials have been extensively used in electrocatalysis.79 Amorphous compounds of CoPx–CoOy with porous structures that are interconnected were created through low-temperature phosphating of Co oxides (Fig. 7a).80,81 Additionally, the production of porous structures through low-temperature phosphating of Co oxide and other metal oxides with different morphologies has been demonstrated, thus proving the method's universality. The prepared catalyst, CoPx–CoOy, shows outstanding electrocatalytic activity for oxygen evolution reactions (OERs) in alkaline medium. The remarkable performance was attributed to the inclusion of porous textures, the amorphous phase and the phosphorus in the catalytic conditions. The structural features provided numerous active sites and effectively shortened the electron transfer pathway – the foundation of the investigation into the evolution of active site-rich electrocatalysts. The introduction of phosphorus enabled the synthesis of amorphous interconnected porous structures, supporting the application of highly efficient catalysts in electrocatalysis and other critical chemical reactions.
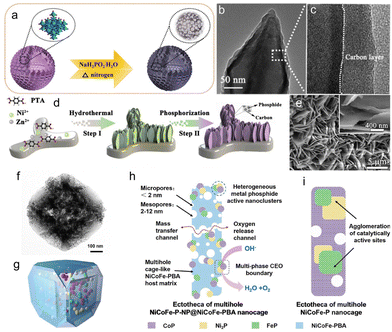 |
| Fig. 7 (a) Schematic illustration of the preparation process of hollow amorphous CoPx–CoOy with interconnected pores. (b) TEM images. (c) HRTEM images. (d) The illustration of the synthetic strategy of ZnNiP nanosheet arrays. (e) The SEM images of ZnNiP-0.25. (f) TEM image. (g) Corresponding schematic model of the porous NiCoFe-P-NP@NiCoFe-PBA nanocages. Schematic diagram for comparison of characteristics of structure and composition of (h) the porous NiCoFe-P-NP@NiCoFe-PBA nanocages and (i) the NiCoFe-P nanocages in the OER process. (a) Reproduced with permission.81 Copyright 2020, Elsevier. (b and c) Reproduced with permission.82 Copyright 2019, Elsevier. (d and e) Reproduced with permission.85 Copyright 2021, Elsevier. (f–i) Reproduced with permission.86 Copyright 2020, American Chemical Society. | |
A fundamental reaction in many renewable energy technologies is the OER. To reduce the cost and complexity of processing renewable energy systems, developing practical and cost-effective alternatives to very high activity precious metal catalysts for OER is highly desirable. Nowadays, on the basis of the abundance of transition metals on Earth, metal phosphides, particularly bimetallic phosphates, have become hopeful candidates for high-efficiency OER catalysts. The rational design of nanostructured bimetallic phosphides is important for the actual applications of electrocatalysis, requiring a good understanding of the nanocrystal growth process and content control. In one work, the researchers summarized a concise strategy for the synthesis of new iron–cobalt bimetallic phosphides (FeCoP) with hollow structures through the phosphorization of Fe (Co)-centred MOFs.82 The obtained FeCoP hollow polyhedron, which had a large specific surface area offering abundant catalytic active sites, revealed excellent electrocatalytic activity for OER with notable stability, small Tafel slope, and low overpotential83 (Fig. 7b and c). This project offers a new strategy for the exploration of highly efficient phosphide catalysts and opens up new avenues for the advancement of OER catalysts. The development of nickel-based phosphide electrodes for next-generation secondary batteries and high-power supercapacitors with stringent safety requirements has attracted much attention.84 In one work, a binder-free Zn–NiP electrode based on Ni MOF and an improved Zn doping process were developed, achieving high electrochemical performance in both Zn-battery and hybrid supercapacitor.85 Excitingly, with increased electronic conductivity, redox reactions and enriched electrochemical active cores, the involvement of the Zn source and the phosphating process created an intrinsic synergy. The ZnNiP-0.5, with its solid structure, had an excellent specific capacity and excellent cycling stability in the alkaline electrolyte (Fig. 7d and e). This project showed a feasible method and provided a useful guideline for the rational design of binder-free electrodes with sophisticated components and structures, which could motivate significant new inventions for the production of electrodes for alkaline batteries and hybrid supercapacitors with high safety.
Metal-based nanoclusters are used in heterogeneous catalytic reactions, but it is very difficult to fabricate such structures while preserving surface activity and avoiding aggregation. NiCoFe Prussian Blue Analogue (PBA) nanocages have been developed as a support for the in situ dispersion and anchoring of polymetallic phosphating nanoparticles (Fig. 7f–i).86 The porous surface and synergistic effect between PMP-NPs and PBA in NiCoFe-P-NP@NiCoFe-PBA nanocages resulted in a remarkable increase in catalytic generation of reactive oxygen species.87 This technique of regulating electron transfer from metal phosphide to PBA substrate offers novel approaches for enhancing the Gibbs free energy of the OER. Introducing Ni2P derived from MOFs with high dispersion was a strategic approach for minimizing the rate of electron–hole recombination during photocatalysis, so as to achieve a more efficient visible-light-driven water decomposition. The precursor of Ni-MOF-74/Ni2P was prepared by low-temperature phosphating.88 The special structure of Ni-MOF-74/Ni2P/MoSx ternary heterojunction was obtained.89 The special structure of Ni-MOF-74/Ni2P provided benefits to MoS loadings. UV-VIS diffuse reflectance spectra results showed that the addition of Ni2P improved the visible light utilization rate of 10%-NPMS, accelerated the production of more electrons, and enhanced the photocatalytic hydrogen production activity.90 The characterisation results, including LSV, PL, EIS and others, indicated that the introduction of Ni2P improved the dispersion of photogenerated carriers. The 10% Ni-MOF-74/Ni2P/MoSx composite showed excellent performance and stability in photocatalytic hydrogen evolution.
Low-temperature phosphating can produce interconnected porous amorphous structures, which provide good electrocatalytic activity and promote the development of catalysts. The synthesis of bimetallic phosphide results in a hollow structure with abundant catalytic sites and large specific surface area. Such a structure is more conducive to improving electrochemical activity, and has excellent specific capacity and good cycling stability in zinc batteries and supercapacitors.
2.3 Sulfurization of MOF materials
Electrocatalytic water decomposition is a new and useful method in sustainable hydrogen energy production technology.91,92 However, it still has the limitation of slow reaction kinetics, so it is necessary to find an efficient, stable and excellent electrocatalyst to break through this limitation. To lower the energy barrier, sectional sulphuration is used to prepare MOFs based on nickel (Ni) and nickel sulphide (NiS) hetero-nanoparticles embedded in semi-MOFs. Unlike the original conventional OER catalysts, semi-MOF catalysts or fully pyrolyzed MOF catalysts can effectively extend the metal–organic framework (MOF) and nanoparticles. Ni/NiS heterogeneous nanoparticles and carbon nanotube (CNT) networks in semi-MOF nanosheets could form Ni-M@C-130 electrocatalysts with excellent unique structures.93 They outperformed all other transition metal-based electrocatalysts reported to date. Because of the special excellent catalytic performance of Ni-M@C-130, MOF nanosheets have comprehensive advantages, synergistic effects, improved mechanical stability and excellent electrical conductivity (Fig. 8a). A study described the effective enhancement of the bi-functional activity of different nanoparticles obtained with excellent electrochemical activity after comprehensive vulcanization, by carbon nanotubes integrated with MOF in MOF nanosheets. Researchers successfully synthesised hollow rod-like NiCoMn ternary metal sulfide nanosheets derived from MOFs. The nanosheets were synthesised by additional sulfurization and etching/ion-exchange reaction. The resulting hollow structure exhibited a characteristic hierarchical architecture composed of NiCoMn-S nanosheets and internal porous channels, which was favourable for electron/ion transport and electrolyte penetration.94 The as-prepared NiCoMn-S nanosheets exhibited a pronounced synergy between the transition metal ions, which resulted in an enhancement of the electrochemical performance. The improved NiCoMn-S nanosheets exhibited better specific capacitance than the corresponding hydroxide precursor (NiCoMn-OH) and the other controlled sulfides with different Ni/Co/Mn ratios due to compositional and structural advantages (Fig. 8b). As a result, the as-prepared NiCoMn-S electrode materials are in the foreground for a wide range of applications in high-performance supercapacitors.
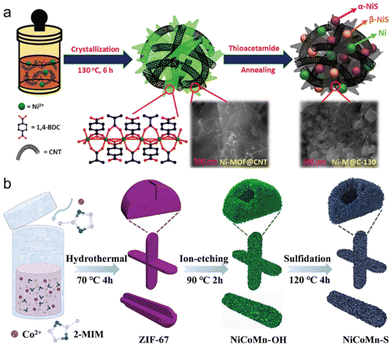 |
| Fig. 8 (a) Schematic illustration of Ni/(α, β)-NiS heteronanoparticle-implanted semi-MOF nanosheets fabrication strategy. (b) The preparation process of hollow rod-like NiCoMn-S ternary metal sulfide. (a) Reproduced with permission.93 Copyright 2021, American Chemical Society. (b) Reproduced with permission.95,96 Copyright 2022, Elsevier. | |
Researchers constructed CdS/Cd-MOF nanocomposites by in situ sulphurisation to form CdS using Cd-MOF as a precursor, based on the high specific surface area, excellent visible light response of CdS and high porosity of MOFs, and the CdS loading was controlled by the dose of thioacetamide.97 In addition, the presence of CdS in Cd-MOF led to a significant expansion of the light response range and enhanced the utilization of visible light. Furthermore, the Mott–Schottky model test revealed that the formation of a type II heterojunction between CdS and Cd-MOF effectively reduced the recombination of photogenerated electrons and holes.96 As a result, the overall efficiency of the system was improved. Due to their versatility in terms of structural and compositional modifications, MOFs are highly promising precursors for developing effective catalysts. One project aimed to improve the performance of zinc–air batteries by utilizing MOF-precursors as functional catalysts used in the oxygen reduction reaction (ORR) and OER. A promising material for the production of doped carbons is the zeolitic imidazolate framework (ZIF). Inspired by tellurium, researchers have created a novel catalyst by incorporating a compound of cobalt, iron, and zinc into a ZIF-8 “pseudo-carbon nanotube.” While iridium-based catalysts have been considered the best choice for the OER, they are not practical due to cost constraints. Previous research has focused on first-row transition metal oxides as an alternative, but metal sulfides are more conductive and represent a promising avenue for developing cost-effective, active, and stable OER catalysts. In a study, researchers developed a mixed metal sulfide catalyst (FeCoNieS@ZIF) by incorporating iron, nickel, and cobalt into a MOF structure (ZIF-67) and subjecting it to sulfurization.98 Vanadium redox flow battery (VRFB) research has been focused on high energy efficiency and cycling stability, and the use of metal–organic skeleton materials doped with other nanomaterials to make electrodes is considered to be a good approach. The composite electrode GF@Fe–N/S-CNFS was prepared using a bimetallic organic skeleton as a precursor by loading Fe, N, S co-doped carbon nanofibers on the graphite felt surface (Fig. 9a and b).
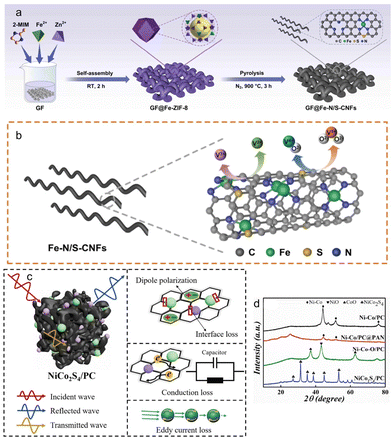 |
| Fig. 9 (a) Schematic representation of the procedure for preparing graphite felt electrode decorated with Fe–N/S-CNFs. (b) Schematic illustrations of structure advantage of GF@Fe–N/S-CNFs for vanadium redox couples. (c) Absorption mechanism diagram of NiCo2S4/PC composite. (d) XRD of the composite materials. (a and b) Reproduced with permission.99 Copyright 2023, Elsevier. (c and d) Reproduced with permission.100 Copyright 2022, Springer. | |
GF@Fe–N/S-CNFs had excellent electrocatalytic activity.99 It is highly conductive. In the redox reaction to V2+/V3+, it was found to show excellent electrocatalytic activity, with excellent energy efficiency and voltage efficiency, because it had high conductivity, rich active center and large specific surface area. In addition, GF@Fe–N/S-CNFs could run smoothly under high current density, and its energy efficiency maintained good stability under the condition of multiple charge and discharge cycles.
With the rapid development of modern science and technology, in order to realize the transmission and reception of information, electronic equipment based on electromagnetic waves has been used in many fields. However, there is a threat from electromagnetic radiation. It was found that the best way to improve the electromagnetic wave absorption performance of products is through the vulcanization reaction. A NiCo2S4/PC composite material was prepared by introducing metal Co through a series of reactions such as polyaniline coating, oxidation and vulcanization reaction, and Ni-MOF/PC was used as the precursor100 (Fig. 9c and d). The Ni–Co bimetal/PC composite material was an ideal absorber of electromagnetic waves due to its excellent electrical conductivity, large surface area and high attenuation due to eddy current losses, due to its carbon skeleton and bimetallic sulfide composition. It has a wide application prospect in electromagnetic wave-absorbing materials.
2.4 Selenization of MOF materials
Hollow porous three-dimensional Co(Ni)Se2/N, prepared by using a metal organic skeleton (MOF) precursor as template by normal doping of carbon compound particles, was an efficient negative electrode material for sodium ion batteries.101 The hollow porous three-dimensional Co(Ni)Se2/N material was synthesized by employing a metal organic skeleton (MOF) precursor as a template. This involved the normal doping of carbon compound particles. This material proved to be highly effective as a negative electrode material in sodium ion batteries. In addition, the metal selenide offered a higher capacity density, while the N-doped multi-hole carbon structure enhanced the electrical conductivity and structural stability of the material, effectively mitigating volume expansion. The synergistic combination of these features resulted in exceptional electrochemical performance. Moreover, the material demonstrated remarkable coulombic efficiency, cycling stability, and kinetic performance, further highlighting its potential as a sodium-ion battery anode material.
Although MOFs containing metal centers with low coordination numbers have demonstrated potential as electrocatalysts in the OER, there has been relatively little investigation into MOFs containing metal centers with high coordination numbers. In one study, a new and good preparation method of surface coating was introduced, which was to coat the surface of Co-MOF (Co(OH)2/Co-MOF) with a layer of Co(OH)2 by in situ cathodic electroconversion technology (Fig. 10a).102 Both experimental analyses and DFT calculations demonstrated that electrons were transferred from Co(OH)2 to Co-MOF, resulting in a synergistic modification of the adsorption free energy of oxygenic intermediates during the OER. This, in conjunction with the distinctive structure of Co-MOF, accounted for its remarkable OER activity and durability over extended periods. Sodium-ion batteries (SIBs) are considered a promising power source because of the abundance and low cost of battery-grade sodium. However, in practice, SIBs have some problems, such as slow intrinsic diffusion kinetics and serious changes in electrode material volume. Metal–organic skeleton (MOF)-derived carbonaceous metal mixtures offer rich and promising applications in electrode materials because of their customizable composition, nanostructure, and physical and chemical properties. From this, researchers manufactured layered MOF-derived carbon nickel selenide with a biphasic content to improve sodium storage capacity103 (Fig. 10b). With the extension of MOF formation time, the pyrolysis and selenide products gradually changed from monophasic Ni3Se4 to biphasic NiSex and NiSe2, and the morphology developed from solid sphere to a layered sea urchur-like yolk-shell structure.
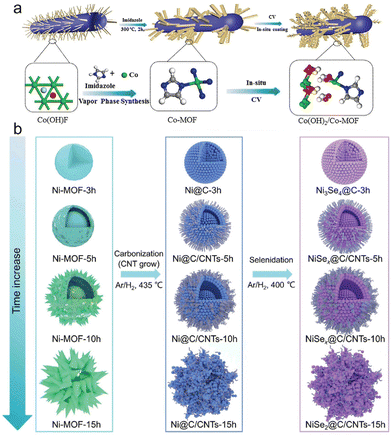 |
| Fig. 10 (a) Schematic illustration of the synthetic methods of Co(OH)2/Co-MOF. (b) Schematic illustrations for the evolution of Ni-MOF at different hours and strategy for fabricating nickel selenides/carbon composites used as anode materials for SIBs. (a) Reproduced with permission.102 Copyright 2020, Elsevier. (b) Reproduced with permission.103 Copyright 2020, Springer. | |
The computational simulation further proved that the lattice mismatch at the phase boundary improved the extra gap in sodium ion storage. Understanding of the phase boundary engineering of switching MOFs and their morphological development will facilitate the manufacture of new composite/hybrid materials for catalysis, sensors, batteries and environmental remediation. Catalysts are used in many aspects and processes of chemical reactions, so it is necessary to improve the catalytic performance of catalysts. Changing their structure to expose the active site and increasing the specific surface area are both practical and feasible methods. During selenization, by controlling the volume of the ionic liquid, a CoSe2-NiS2 heterostructure coated with N, P, F triple-doped carbon (NPFC) was generated, thus regulating the atomic doping and special structure of the CoNi metal–organic skeleton. The specific surface area of CoSe2–NiSe2/NPFC was five times larger than that of flaky air structures without ionic liquids because of its unique cubic porous structure.104 In addition, CoSe2–NiS2/NPFC, as a positive electrode material, showed good HER activity in both acidic and alkaline liquid surroundings, and showed excellent performance (Fig. 11a–c). In flexible solid-state supercapacitors, it could still have a considerable and good energy density at extremely high power. Because of the very high specific capacities, a variety of electronic properties, and very high electrochemical activity, transition metal selenides are thought of as potential anodes for sodium-ion and lithium-ion storage. However, their electrochemical performance is still limited by large volume expansion and weak electronic conductivity during cycling. One project involved the anchoring of CoSe hollow polyhedrons to graphene (CoSe/G)106 (Fig. 11d). CoSe nanoparticles were formed and dispersed on graphene sheets by subjecting a MOF to an in situ high-temperature selenium treatment.105 The unique design ensured structural cohesion and detected metal ion reaction rates, validated by in situ and off-site test methods and kinetic analysis. New insights into the structure of MOF derivatives for high-performance storage devices were provided by the improved electrochemical performance for alkaline ion storage. Catalysts play an important role in sustainable energy conversion technology, and research on new high-performance friction catalysts for the hydrogen evolution reaction (HER), OER, and ORR is of great significance in the development of catalysts. In one project, a template supporting MOF was prepared using an iron-doped copper-cobalt selenized hollow carbon nanofiber mat (iron-doped MOF CuCoSe@HCNFs) as a three-functional-point catalyst. In this process, the hydroxide metal was often sacrificed as a precursor to develop a porous carbon network. In addition, the absolute characterization of the structure of the synthetic material showed that templated MOFs had significant effects on porous carbon networks, surface defects, surface area, and interfacial charge transfer.107 Due to its unique structural characteristics, the iron-doped CuCoSe@HCNF nanostructure promoted efficient electron transfer between the electrode and the electrolyte interface, realized the effective three-function electrocatalysis of water decomposition, and had excellent electrocatalytic activity. For the convenience of comparison, we have summarized the different synthesis methods of MOF derivatives in Table 1.
 |
| Fig. 11 Schematic of preparation processes of (a) CoSe2-NiS2/C, (b) CoSe2-NiS2/NPFC-0.1, and (c) CoSe2-NiS2/NPFC. (d) Schematic illustration of the synthesis process for CoSe/G. (a–c) Reproduced with permission.104 Copyright 2023, Wiley. (d) Reproduced with permission.105 Copyright 2021, Wiley. | |
Table 1 Synthesis methods and applications of MOF derivatives
Materials |
Precursors |
Methods |
Environments |
Applications |
Ref. |
HCS@Co/NC |
HCS |
Pyrolysis |
— |
Catalyst |
42
|
Co3O4–Bi2O3 |
MOF(Bi-BTC) |
Calcination |
— |
Catalyst |
43
|
PMS |
SCo-MOF |
Calcination |
300–600 °C |
Catalyst |
45
|
C@CueNi |
Ni-MOFs and Cu-MOFs |
Calcination |
N2 |
Catalyst |
52
|
Ni–Fe–O/NPC |
PCNFs |
Build |
— |
Supercapacitors |
52
|
P–Fe–N-CNTs |
Fe–N–C |
Pyrolysis |
— |
Catalyst |
58
|
CU-BTC@biochar |
Biochar |
Facile one pot method |
— |
Adsorption |
57
|
WS2@Co9S8 |
ZIF-67 |
Calcination |
Liquid |
Catalyst |
60
|
NCZIF |
ZIF-8 |
Calcination |
Inert gas |
Biomedicine |
61
|
CNT/Fe@N–C |
MIL-101(Fe) |
Calcination |
900 °C |
Adsorption |
62
|
CuCo/NCNT |
CuCo-PBA |
Anneal |
Argon |
Adsorption |
76
|
NiCo/C/CNT/RGO |
ZIF-67 |
Pyrolysis |
Argon, 600 °C |
Adsorption |
68
|
Co-Co2P@NC-P3-700 |
P-ZIF |
Pyrolysis |
— |
Catalyst |
72
|
ZrO2 (ZrO2N @ C) |
NH2-UiO-66 |
Calcination |
600 °C |
Catalyst sensing |
74
|
In2O3–NiO |
Ni-MOF |
Anneal |
— |
Catalyst |
76
|
Ni-MOF-74/Ni2P/MoSx |
Ni-MOF-74/Ni2P |
Phosphate |
Low temperature |
Catalyst |
89
|
CdS/Cd-MOF |
Cd-MOF |
Phosphate |
— |
Catalyst |
96
|
FeCoNieS@ZIF |
ZIF-67 |
Phosphate |
— |
Catalyst |
98
|
GF@Fe–N/S-CNFs |
— |
Phosphate |
— |
Catalyst |
99
|
NiCo2S4/PC |
Ni-MOF/PC |
Phosphate |
— |
Adsorption |
100
|
Fe-doped MOF CuCoSe@HCNFs |
HCNF |
Build |
— |
Catalyst |
107
|
2.5 Other derivative methods
MOF derivatives can also be prepared by many other methods, four of which are introduced here: etching, one-pot hydrothermal method or one-pot self-templating method, templating strategy and crystalline transforming.108 MOF is a viable option for metal protection. However, MOFs have limited active sites and poor stability in acid–base solutions, which greatly limits their wide application. The acidic properties of tannic acid (TA) were exploited to etch surface-active sites.
Subsequently, with the protonated TA the exposed metal active site was chelated, that very efficiently protected the metal ions.111 At the same time, a large abundance of phenolic hydroxyl groups was provided to improve the stability of imidazole-coordinated MOFs by TA. The electrochemical test results revealed that the MOF composites synthesized by this method had high effective capacitance and excellent cycling stability. Another study involved a cooperative etching–coordination–reorganization method starting from ZIF-67 NPs.112 Due to their advantages of structure and composition, the materials exhibited superior electrocatalytic performance towards the OER, and excellent stability. A study reported that in situ etching of PBA particles induced nucleation and growth of TiO2 nanoflakes onto the concave surface of PBA particles, and thus enhanced the interlayer interaction.113 The materials (Prussian blue analog (PBA)-TiO2) provided support for water reduction and oxidation reactions. These results revealed the importance of interface design at the micro level. In a study a spontaneously etched mesoscopic crystal, cyanide-bridged cobalt–iron (CN-CoFe) organometrically hybridized to an atomically fabricated open framework, was filled with corrosion-resistant high surface energy defects.114 In a study, monodisperse hollow multi-shell mesoporous MOF (UiO-66 NH2) particles were fabricated that had a structure of continuously growing multilayer MOFs alternating with two types of mesoscopic layers, using one-step selective etching (Fig. 12a–h).109 The catalytic activity of the synthesized hollow multi-shell mesoporous UiO-66-NH2 particles for the CO2 cycloaddition reaction was improved due to the accessibility of the Lewis acidic site and the accumulation of reactant within the multi-compartment structure. The two-template oriented continuous assembly strategy paves the way for the rational construction of multi-layer MOF nanostructures with different physical and chemical characteristics. The properties of the etched MOF materials can be improved to varying degrees, thus ensuring the electrochemical stability of the MOF. It is important to develop a class of MOFs that can be used as electrochemical energy storage devices, because it is difficult to maintain the original physical and chemical properties of MOFs in alkaline aqueous solutions surroundings. A highly alkaline-stable metal oxide @ MOF composite Co3O4 nanocube @ Co-MOF (Co3O4@Co-MOF) was synthesized by a controlled and simple one-pot hydrothermal method in higher alkaline surroundings (Fig. 12i).110 The obtained composite materials exhibited excellent alkaline stability and also maintained their original structure in 3.0 M KOH for at least 15 days. Due to the excellent alkaline stability, special structure, and a large surface area, the Co3O4@Co-MOF composite exhibited unique capacitance and high cycling stability. In another study, Ni–Fe layered double hydroxide (LDH) nanocages with adjustable shells were synthesized by a one-pot self-templating method. By adjusting the degree of template etching at the interface, the number of shells could be precisely controlled.115 Due to their large electroactive surface area and excellent chemical composition, these materials exhibited very attractive and excellent electrocatalytic activity in the OER and excellent stability in alkaline electrolytes. Based on a MOF templating strategy, investigators have synthesized integrated nanostructures consisting of copper-substituted CoS2@CuxS double-shell nanoboxes (Cu CoS2@CuxSDSNBs).116 Due to their unique shell structure and complex composition, they had stronger electrochemical performance, excellent rate ability and excellent cycling stability. It is important to study the crystallization transformation process in MOFs. A theoretically impossible transformation direction from a 3D ZIF to a 2D ZIF was achieved by the Marangoni effect in the droplet.117 This study not only demonstrated another feasible pathway and direction for the transformation of MOF crystals, but also provides a completely new perspective for the construction of MOF nano frameworks and improves our understanding of the growth mechanism and design of innovative materials.
 |
| Fig. 12 (a) Schematic illustration of the synthesis process. (b–d) SEM and (e and f) TEM images of 3S-mesoUiO-66-NH2 HoMSs. (g) Magnified TEM image showing the mesoporous shells in an individual 3S-mesoUiO-66-NH2 HoMS. (h) Scanning transmission electron microscopy (STEM) and elemental mapping images for Zr, C, and N elements. (i) Schematic illustration of one-pot hydrothermal synthesis of Co3O4@Co-MOF composite. (a–h) Reproduced with permission.109 Copyright 2023, Nature. (i) Reproduced with permission,110 Copyright 2019, China Science Publishing & Media Ltd. | |
3 Energy storage applications of MOF derivatives
Because of their excellent electrical conductivity and good stability in various solvents such as water and chloroform, MOF materials are ideal for electrochemical applications such as capacitors and batteries.118,119 For batteries, these can be lithium, sodium, potassium batteries, including lithium sulfur batteries and air batteries in a new direction (Fig. 13).120,121 In terms of capacitors, they have good cycling stability, power density, fast charging speed, and are a relatively stable portable electrochemical energy storage system. Because of their good cycling life, they have great research value in academia.
 |
| Fig. 13 Electrochemical energy storage applications of MOF derivative materials. | |
3.1 Supercapacitors
The supercapacitor is a kind of component between capacitor and rechargeable battery. It is a new type of chemical reversible energy storage device with a large capacity of farad level.122,123 The working power of a supercapacitor is much higher than that of an ordinary battery, because of its short charging time and long service life, which has attracted attention from energy battery scientists.124 But supercapacitors are not without their drawbacks. Compared with ordinary batteries, the energy density of supercapacitors is much lower, which limits their development. In order to solve this weakness, scientists are constantly studying to improve the energy density of supercapacitors.125 MOF-derived materials have strong conductivity, good stability and high adjustability, and their application in supercapacitors is also a direction worthy of development.126,127 Researchers have proposed a common way of synthesizing even 3D metal carbides, nitrides, and carbonitrides (MXene)/MOF compounds (Co-PBA, Ti3C2TX/ZIF-8, Ti3C2TX/Cu-BTC, Ti3C2TX/ZIF-67, and Ti3C2TX/Fe) that unite the advantages of MXenes and MOFs to improve stability and enhance conductivity.128,129 Afterwards, 3D hollow Ti3C2TX/ZIF-67/CoV2O6 compounds with excellent electron and ion-transport properties separate from Ti3C2TX/ZIF-67 were compounded (Fig. 14a–e). The findings of this study pave the way for a novel approach for designing and synthesizing MXene/MOF compounds and complex hollow structures with customizable structures and compositions for diverse applications.130
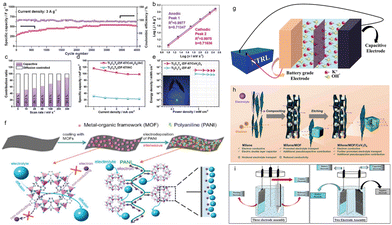 |
| Fig. 14 (a) Long-term cycling stability of Ti3C2TX/ZIF-67/CoV2O6 over 4000 GCD cycles at a current density of 3 Ag.1 (b) Logarithm peak current versus logarithm scan rate plots. (c) Capacitive contributions of Ti3C2TX/ZIF-67/CoV2O6. (d) Specific capacitance of Ti3C2TX/ZIF-67//AC and Ti3C2TX/ZIF-67/CoV2O6//AC at current densities from 1 to 5 mA cm−2. (e) Energy density versus power density plots of Ti3C2TX/ZIF-67//AC and Ti3C2TX/ZIF-67/CoV2O6//AC. (f) Schematic illustration of the two-step fabrication process of PANI-ZIF-67-CC electrode. After interweaving the MOF crystal with PANI, both electrons and electrolyte can enter the MOF surface and EDLC is formed on the surface of the PANI-ZIF-67-CC electrode. (g) Schematic of supercapattery device. (h) Schematic of electrolyte/electron transport in Ti3C2TX, Ti3C2TX/ZIF-67 and hollow Ti3C2TX/ZIF-67/CoV2O6 electrodes. (i) Three-electrode assembly used for testing different electrode materials. (a–e) Reproduced with permission.128 Copyright 2022, Wiley. (f) Reproduced with permission.131 Copyright 2022, Elsevier. (g–i) Reproduced with permission.132 Copyright 2021, Elsevier. | |
In the last few years, the speedy development of MOF supercapacitors has reached a level that meets the needs of portable and wearable electronic devices.131 Here, the researchers focused on the latest advances in flexible solid-state supercapacitors (FSSCS) based on MOFs. Some original MOFs were reported for FSSCs (Fig. 14f). However, they were often limited by their low conductivity. In recent times, researchers have pursued three main strategies to increase the conductivity of MOF materials: utilizing newly synthesized highly conductive MOFs, developing multifunctional composite materials based on MOFs, and producing MOF-derived materials. The classification of FSSC electrode materials based on MOFs and the representative manufacturing technology of flexible devices based on MOFs were discussed. The general electrochemical performance and recent progress of MOF-based material assembly devices were summarized, which increasingly show the potential to achieve high-performance MOFs-based FSSCs in the future. Supercapacitors are currently receiving much attention because they have excellent specific energy (Es) without affecting their specific power (Ps), which is better than batteries. Compared with the common pseudo capacitors (PCs) and electric double-layer capacitors (EDLCs), supercapattery devices have exhibited bigger specific capacitance (Fig. 14g).132 Material-based metal oxides, phosphates, sulfides and MOFs have been widely used in the development of hybrid energy storage devices (HESDs) (Fig. 14h). Currently, the challenge for this technology is to increase the energy density without reducing the power density. The advantages of the two technologies (battery and supercapacitor (SC)) combined into a single system provided tremendous power from the capacitive content and high specific energy from battery-grade materials (BGM) (Fig. 14i). In addition, the study aimed to elucidate the electrochemical properties of metal oxides, phosphates, sulfides and MOFs for energy storage applications.
3.2 Metal-ion batteries
The Li-ion battery has a long service life. Compared with other batteries, the Li-ion battery can be used for seven to eight years under the same conditions.133 Moreover, the Li-ion battery has the advantages of fast charging, high temperature resistance, large capacity and good safety, which provides a lot of convenience for people using them in daily life.134 But the Li-ion battery also has its shortcomings: Li-ion battery positive vibration density is small, it has large volume, poor conductivity, and poor performance at low temperature; these are problems of the Li-ion battery that have to be solved. The significant advantage of the Na-ion battery is that there are abundant sodium ion resources, and it has low cost and high safety.135,136 However, compared with Li-ion batteries, the Na-ion battery has low energy density and slow development progress, and is still in the early stage of development.137 The K-ion battery also shows great potential due to its low cost and rapid ion transport dynamics in electrolytes.127 However, the strong activity of potassium also has safety risks, and it is difficult to deal with due to its violent reaction in water. MOF derivatives have good stability, strong conductivity and can be used in batteries, which is a new direction for the development of Li, Na and K-ion batteries in the future.
With abundant and cost-effective sodium resources, energy storage systems that rely on sodium chemistry hold great promise as a viable alternative to conventional lithium-ion rechargeable batteries (LIBs).138 Aqueous sodium-ion rechargeable batteries (ASIBs) have emerged as a safer, more cost-effective, and more ionically conductive alternative to batteries that rely on traditional organic electrolytes. This is due to the fact that ASIBs operate using aqueous electrolytes (Fig. 15c). ASIBs are particularly well suited for large-scale energy storage applications. In this regard, multifunctional organometallic frameworks have emerged as a highly promising option for electrode materials for Na+ storage in ASIBs. MOFs possess a range of desirable characteristics, including 3D multihole crystallinity, compatibility with aqueous electrolytes, long cycling life, and ease of synthesis (Fig. 15a and b). The review article outlines the methods that have been developed using MOFs to enable their successful implementation in ASIBs. It also identifies the key challenges that need to be addressed in this field of research based on the latest knowledge and insights. The safety of high-energy storage systems is a crucial requirement for their potential large-scale applications. In the case of lithium–sulfur (Li–S) batteries, the conventional polyolefin separators are prone to fire and explosion in extreme conditions due to their poor mechanical strength and low flash point. To address this issue, one project proposed the development of a hierarchical functional separator (Z-PMIA separator) using in situ formed Co-containing zeolitic imidazolate framework ZIF-L(Co) secondary nanostructures and a poly (m-phenylene isophthalamide) (PMIA) membrane (Fig. 15f). The Z-PMIA separator was designed to be thermotolerant and non-flammable (Fig. 15d and e).139 The Z-PMIA separator offered a range of desirable properties, including high mechanical strength and outstanding thermal stability, thanks to the properties of the aramid fibers and unique secondary ZIF-L(Co) nanostructures. Additionally, the Z-PMIA separator effectively restricted the progression of the polysulfide shuttle and lithium dendrites. The Z-PMIA separator demonstrated significant potential for use in future high-performance and high-security energy storage systems, indicating a promising commercial outlook.
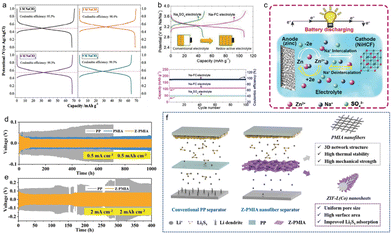 |
| Fig. 15 (a) Charge/discharge profiles of NiHCF in 1, 3, 6, and 9 M NaClO4 electrolytes at a current density of 0.5 C. (b) Charge/discharge profiles and cycling performance of NiHCF in 0.1 mL of 1 M Na2SO4 or 0.1 M redox-active sodium hexacyanoferrate (Na-FC, Na4Fe(CN)6) electrolytes. (c) Schematic illustration of the redox process for the NiHCF|Zn full cell during the charge–discharge processes. (d) Li plating/stripping performance in symmetric cells with different separators. (e) The Li plating/stripping stability for PP, PMIA and Z-PMIA separators at a current density of 0.5 mA cm−2 with an aerial capacity of 0.5 mA h cm−2. (f) Schematic illustration of the Z-PMIA separator for regulating Li deposition and blocking polysulfide shuttle in Li–S batteries. (a–c) Reproduced with permission.138 Copyright 2020, Elsevier. (d–f) Reproduced with permission.139 Copyright 2021, Elsevier. | |
3.3 Li–S battery
The Li–S battery is a kind of Li-ion battery, with sulfur as the positive electrode of the battery, lithium as the negative electrode.140,141 The battery is cheap, does not pollute the environment too much, is less toxic after use and requires less energy to recycle. The drawbacks of the Li–S battery cannot be ignored.142 The electronic and ionic conductivity of elemental sulfur is poor, and the conductivity of sulfur materials at room temperature is extremely low.143,144 The intermediate discharge product of the Li–S battery will increase the viscosity of the electrolyte and reduce the ionic conductivity.145 In the process of charge and discharge, the Li–S battery will produce expansion which results in change of the positive electrode morphology and structure. MOF-derived materials have strong tunability and stability.146 The addition of MOF-derived materials can enhance the stability of the Li–S battery and make up for its shortcomings.
Multi-hole crystalline materials, including COFs and MOFs, have attracted considerable interest in energy storage applications, particularly batteries, because the ordered multi-hole structures can provide rapid transport and storage pathways without large volume fluctuations.147 In recent years, the original COFs and MOFs used in batteries have attracted attention because of their good characteristics. In one work, the researchers reviewed advances in three of the most important applications of batteries, including electrode or host materials, solid electrolytes, and separators.148 The article summarized the latest challenges and benefits of the emerging field, offered a comprehensive understanding of the underlying mechanism, and put forth design criteria for practical battery applications. Lithium–sulfur batteries have been identified as one of the most promising next-generation rechargeable batteries because of their cost effectiveness and excellent energy density. However, due to the slow flow reduction process in the reaction, their practical application has been hindered to a certain extent, and they have not been fully and reliably studied. However, the interaction and catalytic activity of lithium sulfide on metal nodes are easily affected by the existence of MOF due to its organic ligands, so MOF has been widely studied as a sulfur fixative.149 In one project, the researchers combined transition states between metal oxides and MOFs to act as electrocatalysts for sulfur by controlling ligand exchange strategies. The newly prepared MOFs not only had the original porous structure, but also exposed a large number of metal nodes as catalytic active sites, making robust LiPs with good absorption. The reversible ligation/deligation of the quasi-MOF and its influence on the durability of the catalyst during the whole electrochemical process was identified, which conferred a high level of catalytic activity. Thanks to its unique structural features, the quasi-MOF exhibited excellent discharge performance and minimal capacity fading over extended cycling periods. The project aimed not only to offer insights into the rational design of quasi-MOF-based materials, but also to provide guidance for their utilization in Li–S batteries. A simple one-pot synthesis strategy was adopted to target a manganese-based polymetallic MOF (bimetallic and trimetallic MIL-100) nanooctahedral battery.146 The types and proportions of these combined elements could be adjusted while retaining the native topology. Through a combination of theoretical calculations and X-ray absorption fine structure tests, the explanation for other metal ions was proved at the atomic level. In addition, these polymetallic manganese MIL-100 nanometer octahedrons were used as sulfur host system to prepare the negative electrode of lithium sulfur batteries. The above synthesis strategy introduced metal ions into the MOF structure, which inhibited the preparation of monometallic MOF nanooctahedra, and thus rationally generated a new polymetallic MOF. Importantly, this strategy plays an important role in the synthesis and research of various micro/nano MOFs in the field of energy storage. The shuttle effect of Li–S batteries and their slow electrochemical dynamics hinder their utility. In the process of lithium-ion deposition, insoluble Li2S/Li2S2 will be deposited on the anode surface, resulting in uncontrolled growth of lithium dendrites.150 This phenomenon can lead to safety hazards such as short circuits. Therefore, inhibiting the shuttle effect and improving the chemical reaction kinetics are the most important steps to solve these problems. This problem can be solved to some extent by means of physical blocking or chemisorption.151 According to the structural characteristics of MOF, physical adsorption can be increased by adding other chemical groups through modification, to achieve the purpose of reducing lithium ion deposition, thereby speeding up the chemical reaction kinetics. In addition, dimethyl sulfoxide can promote the mesoscale phase separation of the two polymers, which can significantly improve the material's electrical conductivity through synergistic secondary doping.152
3.4 Metal–air battery
The biggest difference between an air battery and dry battery is that the oxidizer of the air battery is taken from the oxygen in the air.153,154 The air battery can also be differentiated as zinc air battery, lithium air battery, aluminum air battery and so on. Any kind of air battery has the very significant advantage of being environmentally friendly. The power of air battery has even reached ten times that of the Li-ion battery, with a high energy density.155 However, the development of the air battery has also been slow; for example, the zinc air battery suffers from electrolyte leakage, high self-discharge rate and other problems, the air battery has serious problems, and the current scientific research needs to be committed to solving these problems.156 However, the addition of MOF-derived materials could also help solve the existing air battery problems. Better development of the air battery is the eagerly awaited outcome. Progress in air batteries could help solve the existing energy crisis and the problem of damage to the environment caused by batteries.
The key to large-scale applications of rechargeable Zn–air batteries is the careful design and structure of high-grade bifunctional oxygen electrocatalysts with high activity, low price and high stability.157,158 Research has proposed an effective approach for enhancing the catalytic activity of the OER/ORR by employing nitrogen-doped 1D bead-like carbon nanofibers decorated with nitrogen-doped CuCo2O4 nanoparticles (N-CuCo2O4@CNFs). The N-CuCo2O4@CNFs catalysts exhibited improved reaction kinetics and superior charge transfer capability due to the synergistic effect between the interconnected 1D hierarchical multi-hole carbon nanofiber structure and the highly active N-doped CuCo2O4 nanoparticles derived from bimetallic MOFs. Notably, the resulting catalysts demonstrated remarkable electrocatalytic performance and superior stability for OER/ORR, surpassing even commercial Pt/C and RuO2 catalysts. In recent years, Co-MOFs have received a lot of attention because of their ease of preparation, adjustable structure and high porosity, making them promising candidates for energy storage and conversion.48 However, due to the poor electrical conductivity of the original Co-MOF, its practical application on a large scale has been hindered. To overcome this limitation and improve electrical conductivity and stability during cycling, researchers have explored various Co-MOF-derived materials as electrodes for rechargeable batteries.159 In addition, due to the high consumption but limited sources of lithium metal, finding rechargeable batteries to replace lithium-ion batteries is a very urgent task. The paper reviewed the applications of MOF-derived materials in various rechargeable batteries, including Li-ion batteries, sodium-ion batteries, Li–sulfur batteries, zinc–air batteries and other rechargeable batteries, and their uses in anode, cathode, electrocatalyst and separator. The morphology of different Co-MOF derivatives, such as cobalt oxide, cobalt chalcogenide, porous carbon, and cobalt phosphide, drives the electrochemical properties, and the corresponding compounds were classified and compared. Finally, the challenges to mass-producing and commercializing Co-MOF-derived materials and practical solutions were discussed. The commercialization of rechargeable zinc–air batteries could be accelerated through materials innovation to address the slow oxygen electrocatalytic kinetics. It is challenging to integrate two sites to achieve bifunctional catalysis. The inclusion of F dopant established a multi-component synergistic active site that showed excellent bifunctional catalysis. This project offered a very promising approach for development.160 Li–CO2 batteries have a lower operating voltage because of the high kinetic barrier between gaseous CO2 and Li2CO3 products, which reduces energy efficiency. Researchers have demonstrated an excellent new Li–CO2 battery system that selectively converted carbon dioxide to lithium oxalate through the synergistic action of carbon dioxide, a soluble REDOX medium, and a REDOX graphene electrode. This research could greatly improve the equilibrium potential and improve the round-trip efficiency. It provided a thoughtful and feasible design for the conversion reaction of dicarbonized carbon.161 Since most MOF materials have good electrical conductivity in terms of electrons and ions, electron transfer or conduction discontinuities caused by two types of electrons or ions in a cell can be effectively avoided.162 MOF solid electrolyte materials have also been widely used in metal ion batteries, showing excellent very good battery performance.
4 Electrocatalytic applications
Electrocatalysis is a kind of catalysis that accelerates the charge transfer at the interface of the electrode and electrolyte.163,164 In the electrocatalytic process, appropriate electrode materials are selected to accelerate the electrode reaction, and the selected materials play the role of catalysts in the electrification process.165,166 The main applications of electrocatalysis are the electrocatalytic treatment of organic sewage, electrocatalytic degradation of chrome-containing wastewater, electrolytic desulfurization of flue gas and raw coal, and so on.167 MOF-derived materials have advantages for electrocatalysis, for instance high specific surface area, porous structure, high proportion of metal sites, catalytic active atoms, etc., and their cost is not too high, so they are expected to replace precious metals as electrocatalysts.
4.1 Hydrogen evolution reaction
Green, sustainable and clean energy is important. Hydrogen is the cleanest fuel and is produced by an electrochemical HER. But it has some problems, including slow electrochemical kinetics. To solve this problem, researchers need to develop catalysts that speed up the kinetics of chemical reactions and ensure their stability.168 The increasing demand for sustainable energy transition and use has led to the exploration of various reactions such as ORR, OER, HER, and CO2RR. These reactions are crucial in areas such as energy, environmental science, and electro- and photoelectron-catalysis.169 To address the need for more efficient and cost-effective catalysts, researchers are focusing on finding alternatives to noble metal-based catalysts. MOFs, which combine the benefits of both inorganic and organic materials, are gaining attention as highly versatile electro- and photoelectron-catalytic platforms (Fig. 16a).170 The exceptional characteristics of MOFs, such as their high surface area, unique porosity, adjustable pore size, remarkable structural and chemical versatility, and the ability to alter their structure pre- and post-synthesis, make them ideal candidates for electro- and photoelectron-catalysis (Fig. 16b and c).207 Bimetallic MOFs (BMOFs) and their derivatives have several advantages over monometallic compositions.171 They exhibit enhanced electrical conductivity, increased active sites, higher charging capacity, and adjustable electrochemical activity (Fig. 16d–i).208 Furthermore, BMOFs can be amalgamated with other electroactive materials to produce advanced compounds with greater specific surface areas, improved electrical conductivity, and enhanced dispersion. Some BMOFs have also been found to exhibit superior electrocatalytic activity when exposed to light, making them ideal candidates for use as photocatalysts.172 The study provides an overview of the latest advancements in BMOFs and their derivatives for various electro- and photoelectrocatalytic applications. It also focuses on the future prospects of such metal-infiltrated compounds and materials, with particular emphasis on energy and electrocatalysis, and highlights the challenges and opportunities in the field.
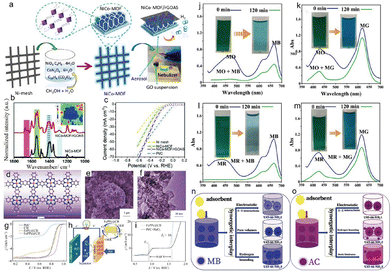 |
| Fig. 16 (a) Schematic illustration of the synthetic process of nano-flocks of NiCo-MOF and graphene oxide aerosol skeleton covered nano-flocks of NiCo-MOF. (b) FTIR spectrum and 2D chemical image (in the inset). (c) LSV curves at 5 mV s−1 for HER. (d) Chemical structure of FePPc; (e and f) TEM images of FePPc@CB; (g) LSV curves of the catalysts; (h) metal–air battery assembly indicating ORR/OER with prepared catalyst; and (i) LSV plots for both ORR and OER. Adapted with permission from ref. The UV-Vis spectra and adsorption images of different mixtures, (j) MO/MB, (k) MO/MG, (l) MR/MB, and (m) MR/MG before and after adsorption onto UiO-66. (n and o) Schematic illustration of the proposed mechanisms for adsorption of organic dyes onto UiO-66-NH2 nanoparticles. (a–c) Reproduced with permission.207 Copyright 2018, The Royal Society of Chemistry. (d–i) Reproduced with permission.208 Copyright 2020, Springer. (j–m) Reproduced with permission.209 Copyright 2020, Elsevier. (n and o) Reproduced with permission.210 Copyright 2020, Elsevier. | |
Platinum-based catalysts still have some shortcomings for the HER in alkaline medium, such as slow chemical kinetics and high cost. Researchers wanted to accelerate the chemical kinetics of hydrogen precipitation and found a way to optimize the metal oxides. By changing the heterojunction between Pt cluster and MO2−, the ability to promote the adsorption and activation of water can be achieved, the reaction energy barrier can be reduced, and better hydrogen generation can be achieved.174 Researchers constructed a bifunctional catalyst, which is dominated by Pt–Er alloy clusters. It can work stably in high-current acidic environments, which is surprising. It showed excellent HER activity, tolerance and stability. The introduction of Er atom greatly improved its stability and tolerance to the acidic environment.175 Metal catalysts have excellent hydrogenation capability, which is suitable for use in the HER, and they are an ideal model and direction for designing catalysts.176 It is very important to improve the chemical reaction kinetics, reduce the reaction energy barrier and strengthen their stability in different acid–base environments.
4.2 Oxygen evolution reaction
The OER is essential for a variety of energy storage and conversion systems, such as water decomposition, various rechargeable batteries, etc. However, the OER also has the problem of slow chemical reaction kinetics, so developing an efficient, fast and stable OER catalyst is still a point of concern for researchers. Removal of harmful pollutants has become an important environmental issue, and MOFs show great potential in this field because of their unique properties, including their ultra-high porosity, large specific surface area, a large number of active adsorption sites, tunable surface chemistry, precise and easily discoverable pore size distribution, and strong host–guest interactions.177,178 Among the various types of MOF, Zr-based MOFs, specifically the UiO-66 family, are highly regarded for their potential applications in wastewater treatment173 (Fig. 16d–o). UiO-66 MOFs are highly desirable for wastewater treatment due to their exceptional thermal stability, and chemical resistance to various solvents (such as acetone, benzene, isoalcohols, dimethylformamide, acid and alkaline solutions). They have good stability under various conditions, including water, air and chloroform, and good resistance to high pressure.179 The strong Zr-bond properties and high coordination number between organic ligands and Zr clusters make UiO-66 MOFs ideal for various wastewater treatment methods.209 One study focuses on the use of UIO-66 MOFs and their improved derivatives as adsorbents for the removal (adsorption/separation and degradation/reduction) of a wide range of water contaminants, including fluorides and phosphates, personal care products, heavy metal ions, pharmaceuticals and organic dyes, due to their excellent high efficiency and water stability. Additionally, the work thoroughly examines the adsorption/separation and degradation/reduction mechanisms of UiO-66 and its derivatives for the elimination of water contaminants. The paper serves as a valuable resource and source of inspiration for researchers interested in exploring the practical applications of UiO-66 MOFs in water and wastewater treatment processes.180 It offers guidance and motivation for researchers to conduct further investigations and experiments to fully realize the potential of UiO-66 MOFs as efficient and effective tools for removing various water pollutants. The unique properties of MOFs, such as their adjustable structures, high porosity, and crystallinity, have garnered significant attention in recent years.210 However, the insufficient electrical conductivity and chemical stability of certain MOFs have limited their use as electrode materials.181 Fortunately, MOF derivatives, particularly phosphide materials, have emerged as promising alternatives due to their exceptional conductivity, large specific surface area, and excellent charge mobility.182 The study provided an overview of the recent advancements in MOF-derived phosphides for energy-related electrocatalysis, including their remarkable performance in electrochemical energy storage and the diverse synthetic strategies and approaches used to develop them.183 In addition, the obstacles and the viable proposals for phosphides derived from MOFs were also discussed. MOF materials are increasingly used in the OER because of their excellent electrocatalytic properties. Researchers found a defect-engineered material, the defective MOF material, had very rich oxygen active sites, which was conducive to oxygen adsorption, thus conducive to the improvement of OER activity.184 In the alkaline electrolyte environment, MOF-derived amorphous porous materials had high performance in the OER, because this material is simple to synthesize, can effectively control and accelerate the chemical reaction kinetics, and has high corrosion resistance and durability in the alkaline environment.185
The OER plays an important role in environmental protection and energy conversion. In the traditional OER process, a large amount of energy is lost. Therefore, we need to study an efficient and durable MOF with good electrocatalytic activity, accelerate its chemical reaction kinetics, and improve the activity of the OER, not only in neutral and acidic environments, but also in alkaline environments.
4.3 Oxygen reduction reaction
The ORR mainly exists in fuel cells, metal air batteries and other batteries, and materials with high surface area and high conductivity have great advantages in this regard. Research has shown that the advanced oxidation process (AOP) is an effective method for wastewater treatment. However, the use of nanomaterials as catalysts in AOPs is hampered by a number of challenges, including limited mass transfer, surface scaling, poor stability, and inconsistent recovery.186 In recent years, MOFs have received a lot of attention because of their highly adjustable and ultra-high porosity; MOF catalysts with exquisite structural and compositional properties are promising precursors.187 Many MOF-derived catalysts have very different physicochemical properties and can show the best performance in various AOPs.188 To address the challenges associated with using nanomaterials as catalysts in AOPs, a study proposed an investigation into the performance optimization of MOF-derived catalysts through multi-component design and micro/macro-structure to elucidate the structure–composition–performance relationship.154 It is important to highlight that this study presented, for the first time, a design strategy for MOF-derived catalyst materials based on microstructure, macrostructure, and multi-component (such as polymetal, heteroatom doping, M/C hybrid, etc.). Furthermore, this paper provided an overview of the significant progress made in MOF-derived catalysts applied in various AOPs, including Fenton, persulfate AOPs, electrochemical processes, photocatalysis, and hybrid AOPs. The main obstacles to the practical application of lithium–sulfur batteries (LSBs) are the sluggish conversion kinetics and the shuttling behavior of the intermediate lithium polysulphides (LiPS). One study presented a highly efficient sulfur host to overcome these limitations, a 1D π-d conjugated MOF, Ni-MOF-1D.189 Ni-MOF-1D was shown to have exceptional binding strength for trapping soluble LiPS species, based on laboratory results and DFT calculations (Fig. 17b and d). Ni-MOF-1D also played the role of a business-like catalyst for the reduction of S during the discharging process and for the oxidation of Li2S during the charging process. Moreover, the π-d system of Ni-MOF-1D allowed for enhanced electron transfer and delocalization of electrons, resulting in improved electrical conductivity. As a result, cathodes based on Ni-MOF-1D exhibited outstanding performance in lithium–sulfur batteries (LSBs) (Fig. 17e–h). The demonstrated strategies and advantages can be successfully generalized to a larger range of PI-D-conjugated MOF materials, which the authors suggest have a high capacity as a sulfur host in LSBs.
 |
| Fig. 17 (a) Possible reaction mechanism of SMT degradation in the FeCu@C/H2O2 system. (b) Galvanostatic charge/discharge profiles of various electrodes with a 0.1 C current rate. (c) The reaction mechanism of p-nitrophenol degradation with the CFP@PANI@MIL-101 (400) cathode. (d) Potentiostatic discharge profile at 2.05 V on S@Ni-MOF-1D and S@Super P electrodes with Li2S8 catholyte to evaluate the nucleation kinetics of Li2S. (e) Nyquist plot of EIS data from S@Ni-MOF-1D electrodes before and after cycling at 1 C. (f) Cycling stability of S@Ni-MOF-1D electrodes at 3 C over 700 cycles. (g) High-loading cycling performances with sulfur loadings of 4.3, 5.5, and 6.7 mg cm−2 at 0.1 C of S@Ni-MOF-1D electrodes. (h) Capacity retention of S@Ni-MOF-1D electrodes with a high sulfur loading of 4.3 mg cm−2 at 1 C. Inset shows the digital photograph of LED lamps powered by one S@Ni-MOF-1D Li–S coin cell. (a and c) Reproduced with permission.190 Copyright 2022, Elsevier. (b and d–h) Reproduced with permission.189 Copyright 2022, Wiley. | |
Fenton-like channels are widely accepted because of their high oxidation potential and good environmental compatibility190 (Fig. 17a and c). MOF is very suitable as a new heterogeneous Fenton catalyst due to its advantages of large surface area, diverse structure and abundant active sites. One project introduced the research progress of MOF derivative materials in Fenton-like processes, including metal compounds and metal nodule carbon.191 Firstly, the strategies used to prepare, construct and assemble the MOFs were presented. Then, the elimination of organic pollutants in Fenton, photo-Fenton and electro-Fenton processes catalyzed by MOFs derivatives was reviewed in turn. The content focused particularly on establishing links between preparing, building, building components and performing. The mechanisms of performance enhancement were also discussed in detail. Finally, the prospects of MOF derivatives for Fenton-like applications were presented.
4.4 Hydrogen oxidation reaction
The hydrogen oxidation reaction (HOR) is an effective method for hydrogen energy conversion. Its chemical reaction kinetics is slow, so the preparation of HOR with high efficiency, economy and stability has been of wide concern for researchers. Organic pollutants are generally stable chemicals in the water environment, but their long-term presence will cause irreversible damage to the entire aquatic ecosystem on a global scale. Therefore, we need to use advanced technology to improve the water environment and protect the environment in which we live (Fig. 18b, g and h). However, the photoFenton process can effectively remove organic pollutants from water. Due to their abundant porosity, high catalytic performance, non-toxicity, sufficient dosage and low price, Fe-MOFs have excellent prospects for practical applications.192 Compared with other MOFs, Fe-MOFs are ideal photocatalysts and have a high coupling strength with Fenton reagents. One paper gave an overview of the progress that has been made in the study of Fe MOFs using the Fenton method and showed how the limitations of the Fenton method can be overcome.193 It discussed the various factors which have an influence on these processes, the reusability and stability of the materials after application, and how the Fenton reactions occur when different materials have been modified. The study provided a comprehensive overview of the use of Fe-MOFs in photoFenton systems for wastewater treatment containing organic pollutants. The conclusions drawn from this research, as well as future prospects for the use of Fe-MOFs in this field, were also presented. The paper serves as a valuable resource for researchers interested in utilizing Fe-MOFs to remove organic contaminants from water.
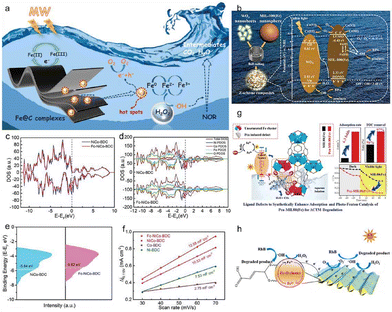 |
| Fig. 18 (a) Possible NOR degradation mechanism over Fe@C700 under MW irradiation. (b) Z-scheme WO3/MIL-100(Fe) degraded BPA in H2O2 system; (c) total density of states, (d) partial density of states. (e) Valence band for the Fc-NiCo-BDC and NiCo-BDC. (f) Current density plots (at 1.15 V vs. RHE) vs different scan rate. (g) Defect engineering of NH2-MIL-88B(Fe) degraded acetamiprid in H2O2 system; (h) GO encapsulated in MIL-88A(Fe)degraded RhB in H2O2 system. (a) Reproduced with permission.193 Copyright 2022, Elsevier. (c–f) Reproduced with permission.194 Copyright 2022, Elsevier. (b, g and h) Reproduced with permission.192 Copyright 2022, Elsevier. | |
A 2D MOF was successfully prepared using monocoordinated ferrocenecarboxylic acid (Fc).194 Theoretical calculations and laboratory results showed that the import of highly electronegative Fc not only altered the electronic structure of the MOF, but also opened up a larger number of under-saturated active sites, thus modifying the adsorptive properties of the intermediate species during the catalytic process (Fig. 18c–f). The project represents a novel approach to the exploration of highly efficient MOFs on the basis of electrocatalysts. A novel ferromagnetic nanoparticle containing mesoporous carbon (Fe@C700) was prepared by pyrolysis of the MIL-101Fe precursor and was used for microwave degradation of norfloxacin (NOR) in an H2O2 environment.193 The FeO nanoparticles were successfully anchored in the carbon matrix as demonstrated by the characterisation results. The catalyst exhibited outstanding degradation performance across a broad pH range. The incorporation of mesoporous carbon promoted electron transfer, enhanced the cycle of Fe(III)/Fe(II) reaction, increased the contact area of Fe2+ and FeO with H2O2, and accelerated the formation of ˙OH.195 In order to find the most active sites in NOR and analyze the possible degradation pathways of NOR and its intermediates, DFT calculations were performed (Fig. 18a). The test results indicated that the intermediates exhibited lower toxicity than NOR. This study has introduced a novel approach for the rational design of FeO carbon compounds as microwave-assisted Fenton-like catalysts, with potential applications in wastewater treatment.
5 Photocatalysis applications
Photocatalysis is the acceleration of a light reaction in the presence of a catalyst. In catalytic photolysis, light is absorbed by an adsorbed substrate. Photocatalysis mainly includes organic conversion, water decomposition, carbon dioxide reduction and so on.196,197 The main challenges of photocatalysis are characterized by poor use of sunlight, recombination of charge carriers and limited exposure of the active site.198 There are many applications of MOF-derived materials in photocatalysis. The porosity, stability and adsorptivity of MOF-derived materials suggest that they will play a great role in photocatalysis. Based on their confidence in MOF-derived materials, scientists have begun promising research in this direction. MOFs have emerged as promising materials and have attracted attention from researchers because of their special chemical and physical properties, such as design flexibility, tunable pore channels and high S/V ratios. This allows them to be used in a variety of research areas – gas separation, gas storage, adsorption, catalysis, drug delivery, sensing, ion exchange, and so on. The rising levels of CO2 in the atmosphere have become a significant global issue, primarily due to the excessive use of fossil fuels in recent years. To mitigate the adverse effects of CO2, it is crucial to either capture it through adsorption or convert it into value-added products.199 This is essential for the betterment of both the environment and humanity, as CO2 is the primary contributor to global warming.
In recent times, there has been growing interest in utilizing MOFs as photocatalysts for CO2 reduction purposes.200 MOFs are considered to be an optimal choice owing to the limitations of semiconductors in utilizing visible light for photocatalytic CO2 reduction. The study highlights the latest advancements in utilizing MOFs as hosts, compounds, and their derivatives in the photocatalytic reduction of CO2 to CO and diverse organic chemicals such as CH4, CH3OH, and HCOOH. To achieve effective charge separation and utilization of visible light, the integration of efficient photocatalytic active sites is a crucial aspect that is discussed in the study. The utilization of materials with high absorption rates and the potential approaches for the logical design and development of superior catalysts are also examined. The study concludes by addressing the major challenges and outlining future prospects in this field.
Global warming and energy scarcity are driving demand for sustainable energy. Thus, it is necessary to convert carbon emissions into sustainable energy as they contribute significantly to the problem of global warming. This opportunity can be exploited through green chemistry routes and through optoelectronic technologies combined with specific compounds such as organometallic frameworks, used in a wide range of fields such as heterogeneous catalysis, energy storage, gas storage and gas sensing, where they hold great promise. MOFs can be used both as a carbon dioxide capture material and as an efficient photocatalyst for the production of value-added products.201 For photocatalyzed CO2 conversion, pure MOFs can be used as catalysts, or they can be modified by linking agents and metal knot structures, or they can be used to form a mixed system. Photocatalytic CO2 reduction may generate several value-added products, including ethane, formaldehyde, methane and carbon monoxide. The paper delves into the synthesis and performance aspects of MOFs, as well as the latest techniques and exceptional advancements in MOF-based photocatalytic conversion of CO2 to methanol. The study aims to assess the progress made, the potential applications, and the opportunities for utilizing MOF-based photocatalysts with modified shapes for CO2 to methanol conversion. The research concludes by summarizing the findings and exploring the prospects of utilizing MOF-based photocatalytic systems for CO2 reduction.
The development of high-performance porous nanomaterials for environmental clean-up is a growing area of interest. MOFs are a class of inorganic–organic hybrid porous materials. They contain organic molecules and metal–oxygen clusters. MOFs are becoming increasingly popular in environmental remediation applications due to their exceptional properties, and are gradually replacing conventional inorganic porous materials like silicas and zeolites. For example, researchers synthesized core–shell MOF-derived 2D hierarchical nanocatalysts for enhanced Fenton-like activities (Fig. 19a).203 Recent research has demonstrated that MOFs are among the most effective materials for gas separation, photocatalysis, and solar energy conversion (Fig. 19c and g). This article provides an overview of the advancements made in the past few years in the study of adsorption and degradation of gaseous contaminants using MOFs (Fig. 19h). The paper discusses the chemical and physical adsorption of gaseous pollutants from the air by MOFs and systematically summarizes strategies for optimizing adsorption capacity through precise control of the chemical and physical properties of MOFs at the atomic level. Specifically, a promising strategy based on the synergistic effect of adsorption-concentration photo-oxidation of gaseous contaminants with this new type of MOF is recommended, which offers the advantages of high efficiency, low cost and prevention of secondary pollution, and is ideally suited for the treatment of gaseous pollutants.202 Finally, the challenges, the perspectives and the personal views of the researchers on the future direction of research are described and assessed.
 |
| Fig. 19 (a) Simplified representation of ZIF-67@ZIF-8@GO catalyst. (b) Possible reaction mechanism for the photocatalytic oxidation of VOCs on the TiO2-UiO-66-NH2 composite. (c) Band energies of common semiconductor photocatalysts and associated redox potentials at pH 7. Emission spectrum of the UV lamp (d), schematic illustration of the used set-up (e) and (f). (g) Maximum efficiency for a solar cell (single layer) given by the Shockley–Queisser limits with the independence of the bandgap energy; (h) minimum CO2 which shows one-pass conversion with the selectivity of CH3OH and specific CO2 capture costs. (i) Scheme indicating the main mechanisms during the two transitions of ferroin perchlorate when annealing at 350 °C and at 500 °C. (a) Reproduced with permission.203 Copyright 2021, Elsevier. (c, g and h) Reproduced with permission.202 Copyright 2020, Elsevier. (b, d, e and f) Reproduced with permission.204 Copyright 2019, Elsevier. (i) Reproduced with permission.205 Copyright 2017, Elsevier. | |
There has been significant progress in the preparation of multi-hole coordination complex crystals. Using a straightforward solvent evaporation technique, TiO2-UiO-66-NH2 nanocomposites of all kinds of mass ratios were effectively synthesized for the purpose of photocatalytic oxidation of acetaldehyde and toluene in UV light conditions (Fig. 19b). Experimental results showed that the nanocomposites displayed better removal efficiencies for acetaldehyde and toluene than TiO2 alone. Long-term evaluation of UiO-66-NH2 and other TiO2 -based porous materials was carried out for up to 720 min under flow conditions. The highest CO2 selectivity and photocatalytic activity were observed for the as-prepared 75TiO2-25UN nanocomposites.204 The N2 adsorption–desorption and SEM analyses showed that the increased surface area facilitated the dispersion of TiO2 and promoted effective connection between the nanocomposites and the VOCs, resulting in improved photocatalytic oxidation performance (Fig. 19d–f). The photoelectrochemical properties showed that the photoinduced charge carriers separated and transferred in the interface of the two elements with a high degree of efficiency. The 75TiO2-25UN sample exhibited excellent deactivation resistance thanks to the oxygen-rich and efficient charge transfer conditions. The radical scavenger experiments indicated that the primary active species were O2− and H+, and based on these findings, a possible mechanism for VOC oxidation was proposed. This study conclusively demonstrated that MOF-based compounds can function as highly efficient and excellent photocatalysts for VOC oxidation applications.
Coordination complexes, a major class of catalytic materials, provide a large surface area for many applications.206 However, the preparation of multi-hole coordination crystals remains a significant challenge, in contrast to MOFs.205 Researchers utilized ferroin perchlorate, a model system with a common ionic complex and a highly reactive counterion, to demonstrate a straightforward approach for creating and regulating the porosity of coordination complex crystals. Using thermal chemical vapor deposition (CVD), they showed that pores can be created in the crystal by annealing the crystals at 350 °C under a flow of argon, oxygen, ethylene and hydrogen. They showed that the key to pore formation was small amounts of oxygen, which could combine with hydrogen to form water. They also showed that by changing the ethylene flow, the density and size of the pores could be easily controlled. Upon increasing the annealing temperature to 500 °C, a secondary transition was observed wherein carbon nanofibers (CNFs) emerged from the multi-hole crystal (Fig. 19i). This route provided a simple and efficient means of synthesizing multi-hole materials with great control over pore density and size. In addition, by briefly changing process parameters such as gas flow and temperature, these findings suggest that it is feasible to produce intricate networks of nanostructures, such as CNFs, using this method.
We have summarized the abbreviations of this review in Table 2.
Table 2 Abbreviations
Abbreviations |
Full name |
ASIBs |
Aqueous sodium-ion batteries |
BMOFs |
Bimetallic MOFs |
BTC |
Benzene-1,3,5-tricaboxylic |
CIP |
Ciprofloxacin |
CNF |
Carbon nanofiber |
CNT |
Carbon nanotube |
COF |
Carbon organic framework |
CVD |
Chemical vapor deposition |
DFT |
Density functional theory |
EDLCs |
Electric double layer capacitors |
EIS |
Electrochemical impedance spectroscopy |
EPR |
Electron paramagnetic resonance |
Fc |
Ferrocenecarboxylic acid |
FSSCS |
Flexible solid-state supercapacitors |
FTS |
Fischer Tropsch synthesis |
HCS |
Hollow carbon spheres |
HER |
Hydrogen evolution reaction |
HESD |
Hybrid energy storage devices |
LDH |
Layered double hydroxide |
LIBs |
Lithium-ion batteries |
LiPS |
Lithium polysulphides |
LSBs |
Lithium–sulfur batteries |
LSV |
Linear sweep voltammetry |
MIL |
Material of Institute Lavoisier |
MMMs |
Mixed matrix membranes |
MOFs |
Metal–organic frameworks |
MWI |
Microwave irradiation |
NADH |
Nicotinamide adenine dinucleotide |
NCNT |
N-doped carbon nanotube |
OER |
Oxygen evolution reaction |
ORR |
Oxygen reduction reactions |
PBA |
Prussian blue analogue |
PCNFs |
Porous carbonaceous nanofiber materials |
PCs |
Pseudo capacitors |
PL |
Photoluminescence spectroscopy |
PMIA |
Poly m-phenylene isophthalamide |
PSA |
Prostate specific antigen |
RGO |
Reduced graphene oxide |
ROS |
Reactive oxygen species |
SC |
Supercapacitor |
SEM |
Scanning electron microscope |
SIB |
Sodium-ion batteries |
TA |
Tannic acid |
TEM |
Transmission electron microscope |
Tyr |
Tyrosinase |
VOC |
Volatile organic compounds |
VRFB |
Vanadium redox flow battery |
XPS |
X-ray photoelectron spectroscopy |
XRD |
X-ray diffraction |
ZIF |
Zeolitic imidazolate framework |
6 Conclusions and perspectives
Over the last two decades, MOFs and their derivatives have found widespread use in multiple energy applications. The rapid development of MOF-derived materials in recent years can be attributed to their exceptional versatility, outstanding electrical conductivity, and robust stability. This review outlined the key processes involved in the synthesis of MOF-derived materials, including calcining, phosphating, sulfurization, the selenylation method, ion exchange, and etching strategies. A particular emphasis was placed on the roles played by these materials in energy storage, notably in the development of supercapacitors and batteries based on lithium, sodium, and potassium. Furthermore, the electrocatalytic applications of MOF-derived materials, such as in hydrogen evolution and redox reactions, were explored, demonstrating the versatility of these materials in facilitating diverse chemical processes. The practicality of MOF-derived materials extends into the realms of adsorption and photocatalysis, offering tangible benefits for everyday life. As research and development in MOFs and their derivatives continue to advance, there is a promising outlook for alleviating the energy crisis through these innovative materials. The exploration of MOFs and their derivatives has seen a remarkable surge in both theoretical and experimental studies, highlighting their potential as cornerstones for future technological advancements. The ability to tailor these materials for a myriad of applications sets a promising pathway for innovation. Nevertheless, precision in the proportioning of raw materials, alongside stringent control over reaction conditions such as temperature, duration, and atmosphere, is crucial. The transformation of MOFs into their desired derivatives necessitates meticulous adjustment of variables, with each step requiring exacting accuracy to achieve the intended outcomes. The diversity in properties and efficiency of MOF-derived materials, influenced by variations in reaction atmospheres and temperatures, underscores the need for scrupulous experimentation and optimization. MOF materials have achieved great results. There has been good and rapid development in the fields of point catalysis, supercapacitors, batteries, and so on. However, the structure of MOF materials still needs some improvement to adapt to current development, and it is important to improve their conductivity and electrochemical stability. We can adopt simple one-pot synthesis methods, complex defect engineering, atomic doping and other strategies. Over years of dedicated research, scientists have incrementally refined the preparation techniques for several widely utilized MOF-derived materials. Advancements in technology and methodology promise avenues for more cost-effective production routes, improving efficiency and minimizing losses in the synthesis of MOF-derived materials. In the face of an acute global energy crisis, the imperative for developing MOF-derived materials optimized for efficient energy storage has never been more critical. Through persistent exploration and innovation, the future of MOF-derived materials shines brightly, promising to play a pivotal role in addressing energy storage needs and beyond.
Author contributions
This manuscript was written through contributions of all authors.
Data availability
No primary research results, software or code have been included and no new data were generated or analysed as part of this review.
Conflicts of interest
There are no conflicts to declare.
Acknowledgements
This work was supported by the National Natural Science Foundation of China (52371240). This work was also supported by the Postgraduate Research & Practice Innovation Program of Jiangsu Province (Yangzhou University, KYCX23_3510).
References
- R. He, W. Wang, Z. Yi, F. Lang, C. Chen, J. Luo, J. Zhu, J. Thiesbrummel, S. Shah, K. Wei, Y. Luo, C. Wang, H. Lai, H. Huang, J. Zhou, B. Zou, X. Yin, S. Ren, X. Hao, L. Wu, J. Zhang, J. Zhang, M. Stolterfoht, F. Fu, W. Tang and D. Zhao, Improving interface quality for 1-cm2 all-perovskite tandem solar cells, Nature, 2023, 618, 80–86 CrossRef CAS PubMed.
- F. Xu, M. Zhang, Z. Li, X. Yang and R. Zhu, Challenges and Perspectives toward Future Wide-Bandgap Mixed-Halide Perovskite Photovoltaics, Adv. Energy Mater., 2023, 13, 2203911 CrossRef CAS.
- Z. Shi, X. Zhang, X. Lin, G. Liu, C. Ling, S. Xi, B. Chen, Y. Ge, C. Tan, Z. Lai, Z. Huang, X. Ruan, L. Zhai, L. Li, Z. Li, X. Wang, G. H. Nam, J. Liu, Q. He, Z. Guan, J. Wang, C. S. Lee, A. R. J. Kucernak and H. Zhang, Phase-dependent growth of Pt on MoS2 for highly efficient H2 evolution, Nature, 2023, 621, 300–305 CrossRef CAS PubMed.
- X. Li, X. I. Pereira-Hernández, Y. Chen, J. Xu, J. Zhao, C. W. Pao, C. Y. Fang, J. Zeng, Y. Wang, B. C. Gates and J. Liu, Functional CeOx nanoglues for robust atomically dispersed catalysts, Nature, 2022, 611, 284–288 CrossRef CAS PubMed.
- H. Chen, Y. Zheng, J. Li, L. Li and X. Wang, AI for Nanomaterials Development in Clean Energy and Carbon Capture, Utilization and Storage (CCUS), ACS Nano, 2023, 17, 9763–9792 CrossRef CAS PubMed.
- A. Sikdar, F. Héraly, H. Zhang, S. Hall, K. Pang, M. Zhang and J. Yuan, Hierarchically Porous 3D Freestanding Holey-MXene Framework via Mild Oxidation of Self-Assembled MXene Hydrogel for Ultrafast Pseudocapacitive Energy Storage, ACS Nano, 2024, 18, 3707–3719 CrossRef CAS PubMed.
- X. Wu, Y. Ru, Y. Bai, G. Zhang, Y. Shi and H. Pang, PBA composites and their derivatives in energy and environmental applications, Coord. Chem. Rev., 2022, 451, 214260 CrossRef CAS.
- E. Vargo, L. Ma, H. Li, Q. Zhang, J. Kwon, K. M. Evans, X. Tang, V. L. Tovmasyan, J. Jan, A. C. Arias, H. Destaillats, I. Kuzmenko, J. Ilavsky, W. R. Chen, W. Heller, R. O. Ritchie, Y. Liu and T. Xu, Functional composites by programming entropy-driven nanosheet growth, Nature, 2023, 623, 724–731 CrossRef CAS PubMed.
- Z. Jiang, X. Xu, Y. Ma, H. S. Cho, D. Ding, C. Wang, J. Wu, P. Oleynikov, M. Jia, J. Cheng, Y. Zhou, O. Terasaki, T. Peng, L. Zan and H. Deng, Filling metal–organic framework mesopores with TiO2 for CO2 photoreduction, Nature, 2020, 586, 549–554 CrossRef CAS PubMed.
- A. M. Eagleton, E. K. Ambrogi, S. A. Miller, N. Vereshchuk and K. A. Mirica, Fiber Integrated Metal–Organic Frameworks as Functional Components in Smart Textiles, Angew. Chem., Int. Ed., 2023, 62, e202309078 CrossRef CAS PubMed.
- X.-J. Hong, C.-L. Song, Y. Yang, H.-C. Tan, G.-H. Li, Y.-P. Cai and H. Wang, Cerium Based Metal–Organic Frameworks as an Efficient Separator Coating Catalyzing the Conversion of Polysulfides for High Performance Lithium–Sulfur Batteries, ACS Nano, 2019, 13, 1923–1931 CAS.
- Y. Jia, Y. Zhang, H. Xu, J. Li, M. Gao and X. Yang, Recent Advances in Doping Strategies to Improve Electrocatalytic Hydrogen Evolution Performance of Molybdenum Disulfide, ACS Catal., 2024, 14, 4601–4637 CrossRef CAS.
- Y. Jiang, Y. Hu, B. Luan, L. Wang, R. Krishna, H. Ni, X. Hu and Y. Zhang, Benchmark single-step ethylene purification from ternary mixtures by a customized fluorinated anion-embedded MOF, Nat. Commun., 2023, 14, 401 CrossRef PubMed.
- X. Liu, X. Xu, X. Xuan, W. Xia, G. Feng, S. Zhang, Z.-G. Wu, B. Zhong, X. Guo, K. Xie and Y. Yamauchi, Unlocking Enhanced Capacitive Deionization of NaTi2(PO4)3/Carbon Materials by the Yolk–Shell Design, J. Am. Chem. Soc., 2023, 145, 9242–9253 CrossRef CAS PubMed.
- R. Sahoo, S. Mondal, S. Chand, A. K. Manna and M. C. Das, A Water-Stable Cationic SIFSIX MOF for Luminescent Probing of Cr2O72− via Single-Crystal to Single-Crystal Transformation, Small, 2023, 19, 2304581 CrossRef CAS PubMed.
- X. Zhang, X.-L. Tian, Y. Qin, J. Qiao, F. Pan, N. Wu, C. Wang, S. Zhao, W. Liu, J. Cui, Z. Qian, M. Zhao, J. Liu and Z. Zeng, Conductive Metal–Organic Frameworks with Tunable Dielectric Properties for Boosting Electromagnetic Wave Absorption, ACS Nano, 2023, 17, 12510–12518 CrossRef CAS PubMed.
- O. M. Yaghi and Z. Rong, Decoding complex order in reticular frameworks, Science, 2023, 379, 330–331 CrossRef CAS PubMed.
- F. Li, M. Du, X. Xiao and Q. Xu, Self-Supporting Metal-Organic Framework-Based Nanoarrays for Electrocatalysis, ACS Nano, 2022, 16, 19913–19939 CrossRef CAS PubMed.
- H. Zhang, A. Chen, Z. Bi, X. Wang, X. Liu, Q. Kong, W. Zhang, L. Mai and G. Hu, MOF-on-MOF-Derived Ultrafine Fe2P-Co2P Heterostructures for High-Efficiency and Durable Anion Exchange Membrane Water Electrolyzers, ACS Nano, 2023, 17, 24070–24079 CrossRef CAS PubMed.
- J. Li, C. Hou, C. Chen, W. Ma, Q. Li, L. Hu, X. Lv and J. Dang, Collaborative Interface Optimization Strategy Guided Ultrafine RuCo and MXene Heterostructure Electrocatalysts for Efficient Overall Water Splitting, ACS Nano, 2023, 17, 10947–10957 CrossRef CAS PubMed.
- G. Zhang, L. Jin, R. Zhang, Y. Bai, R. Zhu and H. Pang, Recent advances in the development of electronically and ionically conductive metal-organic frameworks, Coord. Chem. Rev., 2021, 439, 213915 CrossRef CAS.
- W. Yin, G. Zhang, X. Wang and H. Pang, One – dimensional metal – organic frameworks for electrochemical applications, Adv. Colloid Interface Sci., 2021, 298, 102562 CrossRef CAS PubMed.
- K. Hou, J. Börgel, H. Z. H. Jiang, D. J. SantaLucia, H. Kwon, H. Zhuang, K. Chakarawet, R. C. Rohde, J. W. Taylor, C. Dun, M. V. Paley, A. B. Turkiewicz, J. G. Park, H. Mao, Z. Zhu, E. E. Alp, J. Zhao, M. Y. Hu, B. Lavina, S. Peredkov, X. Lv, J. Oktawiec, K. R. Meihaus, D. A. Pantazis, M. Vandone, V. Colombo, E. Bill, J. J. Urban, R. D. Britt, F. Grandjean, G. J. Long, S. DeBeer, F. Neese, J. A. Reimer and J. R. Long, Reactive high-spin iron(IV)-oxo sites through dioxygen activation in a metal-organic framework, Science, 2023, 382, 547–553 CrossRef CAS.
- J. Y. Choi, J. Flood, M. Stodolka, H. T. B. Pham and J. Park, From 2D to 3D: Postsynthetic Pillar Insertion in Electrically Conductive MOF, ACS Nano, 2022, 16, 3145–3151 CrossRef CAS.
- K. Xu, S. Zhang, X. Zhuang, G. Zhang, Y. Tang and H. Pang, Recent progress of MOF-functionalized nanocomposites : From structure to properties, Adv. Colloid Interface Sci., 2024, 323, 103050 CrossRef CAS.
- S. Yao, S. Zhang, G. Zhang, Y. Tang, R. Zhu, Y. Peng, Y. Chen and H. Pang, Mesoporous Iron Family Element (Fe, Co, Ni) Molybdenum Disulfide/Carbon Nanohybrids for High-Performance Supercapacitors, Inorg. Chem., 2023, 62, 16038–16046 CrossRef CAS.
- R. Beucher, R. D. Andrei, C. Cammarano, A. Galarneau, F. Fajula and V. Hulea, Selective Production of Propylene and 1-Butene from Ethylene by Catalytic Cascade Reactions, ACS Catal., 2018, 8, 3636–3640 CrossRef CAS.
- J. Jang, Y. Jo and C. B. Park, Metal–Organic Framework-Derived Carbon as a Photoacoustic Modulator of Alzheimer's β-Amyloid Aggregate Structure, ACS Nano, 2022, 16, 18515–18525 CrossRef CAS PubMed.
- L. Luo, L. Hou, X. Cui, P. Zhan, P. He, C. Dai, R. Li, J. Dong, Y. Zou, G. Liu, Y. Liu and J. Zheng, Self-condensation-assisted chemical vapour deposition growth of atomically two-dimensional MOF single-crystals, Nat. Commun., 2024, 15, 3618 CrossRef CAS.
- B. Tang, Y. Zhou, Q. Ji, Z. Zhuang, L. Zhang, C. Wang, H. Hu, H. Wang, B. Mei, F. Song, S. Yang, B. M. Weckhuysen, H. Tan, D. Wang and W. Yan, A Janus dual-atom catalyst for electrocatalytic oxygen reduction and evolution, Nat. Synth., 2024, 3, 878–890 CrossRef.
- X. Bai, C. Yang and Z. Tang, Enabling long-distance hydrogen spillover in nonreducible metal-organic frameworks for catalytic reaction, Nat. Commun., 2024, 15, 6263 CrossRef CAS PubMed.
- L. A. Brandner, B. Marmiroli, M. Linares-Moreau, M. Barella, B. Abbasgholi-Na, M. De, J. Velásquez-Hernández, K. L. Flint, S. Dal Zilio, G. P. Acuna, H. Wolinski, C. J. Doonan and P. Falcaro, Ordered Transfer from 3D-oriented MOF Superstructures to Polymeric Films: Microfabrication, Enhanced Chemical Stability, and Anisotropic Fluorescent Patterns, Adv. Mater., 2024, 2404384 CrossRef PubMed.
- H. Zhou, S. Gu, Y. Lu, G. Zhang, B. Li, F. Dou, S. Cao, Q. Li, Y. Sun, M. Shakouri and H. Pang, Stabilizing Ni2 + in Hollow Nano MOF/Polymetallic Phosphides Composites for Enhanced Electrochemical Performance in 3D-Printed Micro-Supercapacitors, Adv. Mater., 2024, 36, 2401856 CrossRef CAS PubMed.
- J. Li, F. Xie, W. Pang, Q. Liang, X. Yang and L. Zhang, Regulate transportation of ions and polysulfides in all–solid-state Li-S batteries using ordered-MOF composite solid electrolyte, Sci. Adv., 2024, 10, 27–34 Search PubMed.
- L. Hu, W. Wu, M. Hu, L. Jiang, D. Lin, J. Wu and K. Yang, Double-walled Al-based MOF with large microporous specific surface area for trace benzene adsorption, Nat. Commun., 2024, 15, 3204 CrossRef CAS.
- J. Wang, J. Liu, H. Wang, M. Zhou, G. Ke, L. Zhang, J. Wu, Z. Gao and D. Lu, A comprehensive transformer-based approach for high-accuracy gas adsorption predictions in metal-organic frameworks, Nat. Commun., 2024, 15, 1904 CrossRef CAS PubMed.
- J. Guo, Q. Xia, W. Y. Tang, Z. Li, X. Wu, L. J. Liu, W. P. To, H. X. Shu, K. H. Low, P. C. Y. Chow, T. W. B. Lo and J. He, Visible light-mediated intermolecular crossed [2 + 2] cycloadditions using a MOF-supported copper triplet photosensitizer, Nat. Catal., 2024, 7, 307–320 CrossRef CAS.
- Y. Liang, X. Yang, X. Wang, Z. J. Guan, H. Xing and Y. Fang, A cage-on-MOF strategy to coordinatively functionalize mesoporous MOFs for manipulating selectivity in adsorption and catalysis, Nat. Commun., 2023, 14, 5223 CrossRef CAS PubMed.
- A. Salehi, N. Shariatifar, G. Jahed-Khaniki, P. Sadighara and M. Hozoori, Simple and rapid determination of tartrazine in fake saffron using the metal organic framework (Fe SA MOF@CNF) by HPLC/PDA, Sci. Rep., 2024, 14, 8217 CrossRef CAS PubMed.
- C. Liu, Y. Bai, W. Li, F. Yang, G. Zhang and H. Pang, In Situ Growth of Three-Dimensional MXene/Metal – Organic Framework Composites for High-Performance Supercapacitors, Angew. Chem., Int. Ed., 2022, 61, e202116282 CrossRef CAS PubMed.
- Y. Li, Z. Jin and T. Zhao, Performance of ZIF-67 – Derived fold polyhedrons for enhanced photocatalytic hydrogen evolution, Chem. Eng. J., 2020, 382, 123051 CrossRef CAS.
- L. Zhong, H. Zhou, R. Li, T. Bian, S. Wang and A. Yuan, In situ confinement pyrolysis of ZIF-67 nanocrystals on hollow carbon spheres towards efficient electrocatalysts for oxygen reduction, J. Colloid Interface Sci., 2021, 584, 439–448 CrossRef CAS PubMed.
- Z. Li, X. Tang, G. Huang, X. Luo, D. He, Q. Peng, J. Huang, M. Ao and K. Liu, Bismuth MOFs based hierarchical Co3O4-Bi2O3 composite: An efficient heterogeneous peroxymonosulfate activator for azo dyes degradation, Sep. Purif. Technol., 2020, 242, 116825 CrossRef CAS.
- S. Liu, L. Kang, J. Zhang, E. Jung, S. Lee and S. C. Jun, Structural engineering and surface modification of MOF-derived cobalt-based hybrid nanosheets for flexible solid-state supercapacitors, Energy Storage Mater., 2020, 32, 167–177 CrossRef.
- J. Li, W. Zhu, Y. Gao, P. Lin, J. Liu, J. Zhang and T. Huang, The catalyst derived from the sulfurized Co-doped metal–organic framework (MOF) for peroxymonosulfate (PMS) activation and its application to pollutant removal, Sep. Purif. Technol., 2022, 285, 120362 CrossRef CAS.
- J. Sun, L. Tao, C. Ye, Y. Wang, G. Meng, H. Lei and S. Zheng, MOF-Derived Ru 1 Zr 1 /Co Dual-Atomic-Site Catalyst with Promoted Performance for Fischer − Tropsch Synthesis, J. Am. Chem. Soc., 2023, 145, 7113–7122 CrossRef CAS PubMed.
- Y. Sun, H. Ji, Y. Sun, G. Zhang, H. Zhou and S. Cao, Synergistic Effect of Oxygen Vacancy and High Porosity of Nano MIL-125 (Ti) for Enhanced Photocatalytic Nitrogen Fixation, Angew. Chem., Int. Ed., 2024, 63, e202316973 CrossRef CAS PubMed.
- X. Lu, H. Xu, P. Yang, L. Xiao, Y. Li, J. Ma, R. Li, L. Liu, A. Liu, V. Kondratiev, O. Levin, J. Zhang and M. An, Zinc-assisted MgO template synthesis of porous carbon-supported Fe-Nx sites for efficient oxygen reduction reaction catalysis in Zn-air batteries, Appl. Catal., B, 2022, 313, 121454 CrossRef CAS.
- X. Xu, X. Zhang, Z. Xia, R. Sun, H. Li, J. Wang, S. Yu, S. Wang and G. Sun, Solid phase microwave-assisted fabrication of Fe-doped ZIF-8 for single-atom Fe-N-C electrocatalysts on oxygen reduction, J. Energy Chem., 2021, 54, 579–586 CrossRef CAS.
- T. Xiao, Y. Wang, J. Wan, Y. Ma, Z. Yan, S. Huang and C. Zeng, Fe-N-C catalyst with Fe-NX sites anchored nano carboncubes derived from Fe-Zn-MOFs activate peroxymonosulfate for high-effective degradation of ciprofloxacin: Thermal activation and catalytic mechanism, J. Hazard. Mater., 2022, 424, 127380 CrossRef CAS PubMed.
- M. B. Zakaria, Y. Guo, J. Na, R. Tahawy, T. Chikyow, W. A. El-Said, D. A. El-Hady, W. Alshitari, Y. Yamauchi and J. Lin, Layer-by-Layer Motif Heteroarchitecturing of N,S-Codoped Reduced Graphene Oxide-Wrapped Ni/NiS Nanoparticles for the Electrochemical Oxidation of Water, ChemSusChem, 2020, 13, 3269–3276 CrossRef CAS PubMed.
- D. Zhang, Y. Li, J. Guo, L. Zhou, Y. Lan and C. Chen, MOFs-derived magnetic C@Cu–Ni bimetal particles: An efficient peroxymonosulfate activator for 2,4,6-trichlorophenol degradation, Chemosphere, 2021, 269, 129394 CrossRef CAS PubMed.
- V. Hegde, U. T. Uthappa, M. Suneetha, T. Altalhi, S. S. Han and M. D. Kurkuri, Functional porous Ce-UiO-66 MOF@Keratin composites for the efficient adsorption of trypan blue dye from wastewater: A step towards practical implementations, Chem. Eng. J., 2023, 461, 142103 CrossRef CAS.
- J. Hei, G. Xu, B. Wei, L. Zhang, H. Ding and D. Liu, NiFeP nanosheets on N-doped carbon sponge as a hierarchically structured bifunctional electrocatalyst for efficient overall water splitting, Appl. Surf. Sci., 2021, 549, 149297 CrossRef CAS.
- F. Diao, W. Huang, G. Ctistis, H. Wackerbarth, Y. Yang, P. Si, J. Zhang, X. Xiao and C. Engelbrekt, Bifunctional and Self-Supported NiFeP-Layer-Coated NiP Rods for Electrochemical Water Splitting in Alkaline Solution, ACS Appl. Mater. Interfaces, 2021, 13, 23702–23713 CrossRef CAS PubMed.
- H. Chang, Y. Guo, X. Liu, P. Wang, Y. Xie and T. Yi, Applied Catalysis B : Environmental Dual MOF-derived Fe/N/P-tridoped carbon nanotube as high-performance oxygen reduction catalysts for zinc-air batteries, Appl. Catal., B, 2023, 327, 122469 CrossRef CAS.
- J. Zhang, J. Shao, X. Zhang, G. Rao, G. Li, H. Yang, S. Zhang and H. Chen, Facile synthesis of Cu-BTC@biochar with controlled morphology for effective toluene adsorption at medium–high temperature, Chem. Eng. J., 2023, 452, 139003 CrossRef CAS.
- D. Acharya, I. Pathak, B. Dahal, P. Chandra, R. Mangal, A. Muthurasu, T. Kim, T. Hoon, K. Chhetri and H. Yong, Immoderate nanoarchitectures of bimetallic MOF derived Ni – Fe – O/NPC on porous carbon nanofibers as freestanding electrode for asymmetric supercapacitors, Carbon, 2023, 201, 12–23 CrossRef CAS.
- W. Chen, S. Chang, H. Yu, W. Li, H. Zhang and Z. Zhang, FeNiP nanoparticle/N,P dual-doped carbon composite as a trifunctional catalyst towards high-performance zinc-air batteries and overall water electrolysis, Nanoscale, 2021, 13, 17136–17146 RSC.
- L. Xia, K. Pan, H. Wu, F. Wang, Y. Liu, Y. Xu, Z. Dong, B. Wei and S. Wei, Few-Layered WS2 Anchored on Co, N-Doped Carbon Hollow Polyhedron for Oxygen Evolution and Hydrogen Evolution, ACS Appl. Mater. Interfaces, 2022, 14, 22030–22040 CrossRef CAS PubMed.
- H. Wan, X. Cao, M. Liu, F. Zhang, C. Sun, J. Xia and Z. Wang, Aptamer and bifunctional enzyme co-functionalized MOF-derived porous carbon for low-background electrochemical aptasensing, Anal. Bioanal. Chem., 2021, 413, 6303–6312 CrossRef CAS PubMed.
- P. Qin, D. Chen, M. Li, D. Li, Y. Gao, S. Zhu, M. Mu and M. Lu, Melamine/MIL-101(Fe)-derived magnetic carbon nanotube-decorated nitrogen-doped carbon materials as sorbent for rapid removal of organic dyes from environmental water sample, J. Mol. Liq., 2022, 359, 119231 CrossRef CAS.
- P. Geng, Y. Lin, M. Du, C. Wu, T. Luo, Y. Peng, L. Wang, X. Jiang, S. Wang, X. Zhang, L. Ni and S. Chen, Confined Synthesis of Amorphous Al 2 O 3 Framework Nanocomposites Based on the Oxygen-Potential Diagram as Sulfur Hosts for Catalytic Conversion, Adv. Sci., 2023, 10, 2302215 CrossRef CAS PubMed.
- K. Srinivas, Y. Chen, B. Wang, B. Yu, X. Wang, Y. Hu, Y. Lu, W. Li, W. Zhang and D. Yang, Metal-Organic Framework-Derived NiS/Fe3O4Heterostructure-Decorated Carbon Nanotubes as Highly Efficient and Durable Electrocatalysts for Oxygen Evolution Reaction, ACS Appl. Mater. Interfaces, 2020, 12, 31552–31563 CrossRef CAS PubMed.
- L. Chai, Z. Hu, X. Wang, L. Zhang, T. T. Li, Y. Hu, J. Pan, J. Qian and S. Huang, Fe7C3 nanoparticles with in situ grown CNT on nitrogen doped hollow carbon cube with greatly enhanced conductivity and ORR performance for alkaline fuel cell, Carbon, 2021, 174, 531–539 CrossRef CAS.
- Y. Sun, H. Ji, Y. Sun, G. Zhang, H. Zhou and S. Cao, Synergistic Effect of Oxygen Vacancy and High Porosity of Nano MIL-125 (Ti) for Enhanced Photocatalytic Nitrogen Fixation, Angew. Chem., Int. Ed., 2024, 63, e202316973 CrossRef CAS PubMed.
- Z. Yao, S. Xu, X. Zhang, J. Yuan, C. Rong, Z. Xiong, X. Zhu, Y. Yu, H. Yu, S. Kang and F. Kuang, CuCo Nanocube/N-Doped Carbon Nanotube Composites for Microwave Absorption, ACS Appl. Nano Mater., 2023, 6, 1325–1338 CrossRef CAS.
- L. Yang, Y. Wang, Z. Lu, R. Cheng, N. Wang and Y. Li, Construction of multi-dimensional NiCo/C/CNT/rGO aerogel by MOF derivative for efficient microwave absorption, Carbon, 2023, 205, 411–421 CrossRef CAS.
- L. Wan, C. He, D. Chen, J. Liu, Y. Zhang, C. Du, M. Xie and J. Chen, In situ grown NiFeP@NiCo2S4 nanosheet arrays on carbon cloth for asymmetric supercapacitors, Chem. Eng. J., 2020, 399, 125778 CrossRef CAS.
- I. Hussain, M. Z. Ansari, C. Lamiel, T. Hussain, M. S. Javed, T. Kaewmaraya, M. Ahmad, N. Qin, K. Zhang, I. Hussain, M. Z. Ansari, C. Lamiel, T. Hussain, M. S. Javed, T. Kaewmaraya, M. Ahmad, N. Qin and K. Zhang, In Situ Grown Heterostructure Based on MOF-Derived Carbon Containing n-Type Zn–In–S and Dry-Oxidative p-Type CuO as Pseudocapacitive Electrode Materials, ACS Energy Lett., 2023, 8, 1887–1895 CrossRef CAS.
- S. Cao, Y. Li, Y. Tang, Y. Sun, W. Li, X. Guo and F. Yang, Space-Confined Metal Ion Strategy for Carbon Materials Derived from Cobalt Benzimidazole Frameworks with High Desalination Performance in Simulated Seawater, Adv. Mater., 2023, 35, 2301011 CrossRef CAS PubMed.
- B. Tang, S. Wang and J. Long, Novel in situ P-doped metal-organic frameworks derived cobalt and heteroatoms co-doped carbon matrix as high-efficient electrocatalysts, Int. J. Hydrogen Energy, 2020, 45, 32972–32983 CrossRef CAS.
- S. Pan, Y. Guo, G. Chen, L. Sun, J. Huang, L. Wang, L. Cheng and Z. Jiao, MOFs-derived synthesis of Ni-doped ZnO nanostructutred material towards excellent N-butanol sensing performance and long-term stability, J. Mater. Sci.: Mater. Electron., 2022, 33, 7501–7514 CrossRef CAS.
- M. Farrag, Ultrasmall bimetallic Ru-Co alloy nanoclusters immobilized in amino-functionalized UiO-66 and N-doped carbonaceous zirconium oxide nanocomposite for hydrogen generation, J. Alloys Compd., 2022, 920, 165893 CrossRef CAS.
- S. Liu, Z. Teng, H. Liu, T. Wang, G. Wang and Q. Xu, A Ce-UiO-66 Metal – Organic Framework-Based Graphene- Embedded Photocatalyst with Controllable Activation for Solar Ammonia Fertilizer Production, Angew. Chem., Int. Ed., 2022, 61, e202207026 CrossRef CAS PubMed.
- Q. Yu, R. Jin, L. Zhao, T. Wang, F. Liu, X. Yan, C. Wang, P. Sun and G. Lu, MOF-Derived Mesoporous and Hierarchical Hollow-Structured In2O3-NiO Composites for Enhanced Triethylamine Sensing, ACS Sens., 2021, 6, 3451–3461 CrossRef CAS PubMed.
- C. Wang, X. Wang, F. Lai, Z. Liu, R. Dong, W. Li, H. Sun and B. Geng, Pt Nanoparticles Supported on N-Doped Porous Carbon Derived from Metal-Organic Frameworks for Oxygen Reduction, ACS Appl. Nano Mater., 2020, 3, 5698–5705 CrossRef CAS.
- Y. Li, P. Zhang, L. Wan, Y. Zheng, X. Qu, H. Zhang, Y. Wang, K. Zaghib, J. Yuan, S. Sun, Y. Wang, Z. Zhou and S. Sun, A General Carboxylate-Assisted Approach to Boost the ORR Performance of ZIF-Derived Fe/N/C Catalysts for Proton Exchange Membrane Fuel Cells, Adv. Funct. Mater., 2021, 31, 2009645 CrossRef CAS.
- Q. Mu, R. Liu, H. Kimura, J. Li, H. Jiang, X. Zhang, Z. Yu and X. Sun, Supramolecular self-assembly synthesis of hemoglobin-like amorphous CoP @ N, P-doped carbon composites enable ultralong stable cycling under high-current density for lithium-ion battery anodes, Adv. Compos. Hybrid Mater., 2023, 6, 1–11 CrossRef.
- X. Yan, G. Wang, Y. Zhang, Q. Guo and Z. Jin, 3D layered nano-flower MoSx anchored with CoP nanoparticles form double proton adsorption site for enhanced photocatalytic hydrogen evolution under visible light driven, Int. J. Hydrogen Energy, 2020, 45, 2578–2592 CrossRef CAS.
- H. Zhou, M. Zheng and H. Pang, Synthesis of hollow amorphous cobalt phosphide-cobalt oxide composite with interconnected pores for oxygen evolution reaction, Chem. Eng. J., 2021, 416, 127884 CrossRef CAS.
- J. Wang, J. Wang, M. Zhang, S. Li, R. Liu and Z. Li, Metal-organic frameworks-derived hollow-structured iron-cobalt bimetallic phosphide electrocatalysts for efficient oxygen evolution reaction, J. Alloys Compd., 2020, 821, 153463 CrossRef CAS.
- T. Feng, F. Wang, Y. Xu, M. Chang, X. Jin, Y. Zhou, J. Piao and J. Lei, CoP/Ni2P heteronanoparticles integrated with atomic Co/Ni dual sites for enhanced electrocatalytic performance toward hydrogen evolution, Int. J. Hydrogen Energy, 2021, 46, 8431–8443 CrossRef CAS.
- F. Sun, Q. Li, Y. Bai, G. Zhang, S. Zheng, M. Peng, X. Chen, N. Lin and H. Pang, A controllable preparation of two-dimensional cobalt oxalate-based nanostructured sheets for electrochemical energy storage, Chin. Chem. Lett., 2022, 33, 3249–3254 CrossRef CAS.
- Y. Yang, S. Li, W. Huang, S. Duan, P. Si and L. Ci, Rational construction of ternary ZnNiP arrayed structures derived from 2D MOFs for advanced hybrid supercapacitors and Zn batteries, Electrochim. Acta, 2021, 387, 138548 CrossRef CAS.
- G. Zhang, Y. Li, X. Xiao, Y. Shan, Y. Bai, H. Xue, H. Pang, Z. Tian and Q. Xu, In Situ Anchoring Polymetallic Phosphide Nanoparticles within Porous Prussian Blue Analogue Nanocages for Boosting Oxygen Evolution Catalysis, Nano Lett., 2021, 21, 3016–3025 CrossRef CAS PubMed.
- Y. Zhu, Q. Zong, Q. Zhang, H. Yang, Q. Wang and H. Wang, Three-dimensional core-shell NiCoP@NiCoP array on carbon cloth for high performance flexible asymmetric supercapacitor, Electrochim. Acta, 2019, 299, 441–450 CrossRef CAS.
- X. Cao, P. Wen, R. Ma, Y. Liu, S. Sun, Q. Ma, P. Zhang and Y. Qiu, Ni2P nanocrystals modification on Ta:α-Fe2O3 photoanode for efficient photoelectrochemical water splitting: In situ formation and synergistic catalysis of Ni2P@NiOOH cocatalyst, Chem. Eng. J., 2022, 449, 137792 CrossRef CAS.
- T. Li and Z. Jin, Unique ternary Ni-MOF-74/Ni2P/MoSx composite for efficient photocatalytic hydrogen production: Role of Ni2P for accelerating separation of photogenerated carriers, J. Colloid Interface Sci., 2022, 605, 385–397 CrossRef CAS PubMed.
- X. Ren, D. Philo, Y. Li, L. Shi, K. Chang and J. Ye, Recent advances of low-dimensional phosphorus-based nanomaterials for solar-driven photocatalytic reactions, Coord. Chem. Rev., 2020, 424, 213516 CrossRef CAS.
- Z. Yu, Y. Duan, X. Feng, X. Yu, M. Gao and S. Yu, Clean and Affordable Hydrogen Fuel from Alkaline Water Splitting : Past, Recent Progress, and Future Prospects, Adv. Mater., 2021, 33, 2007100 CrossRef CAS PubMed.
- W. Yuan, T. Jiang, X. Fang, Y. Fan, S. Qian, Y. Gao, N. Cheng, H. Xue and J. Tian, Interface engineering of S-doped Co 2 P @ Ni 2 P core – shell heterostructures for efficient and energy-saving water splitting, Chem. Eng. J., 2022, 439, 135743 CrossRef CAS.
- K. Srinivas, Y. Chen, X. Wang, B. Wang, M. Karpuraranjith, W. Wang, Z. Su, W. Zhang and D. Yang, Constructing Ni/NiS Heteronanoparticle-Embedded Metal-Organic Framework-Derived Nanosheets for Enhanced Water-Splitting Catalysis, ACS Sustainable Chem. Eng., 2021, 9, 1920–1931 CrossRef CAS.
- C. Kang, L. Ma, Y. Chen, L. Fu, Q. Hu, C. Zhou and Q. Liu, Metal-organic framework derived hollow rod-like NiCoMn ternary metal sulfide for high-performance asymmetric supercapacitors, Chem. Eng. J., 2022, 427, 131003 CrossRef CAS.
- C. Kang, L. Ma, Y. Chen, L. Fu, Q. Hu, C. Zhou and Q. Liu, Metal-organic framework derived hollow rod-like NiCoMn ternary metal sulfide for high-performance asymmetric supercapacitors, Chem. Eng. J., 2022, 427, 131003 CrossRef CAS.
- C. Jing, Y. Zhang, J. Zheng, S. Ge, J. Lin, D. Pan, N. Naik and Z. Guo,
In situ constructing visible light CdS/Cd-MOF photocatalyst with enhanced photodegradation of methylene blue, Particuology, 2022, 69, 111–122 CrossRef CAS.
- M. Zhang, Q. Hu, K. Ma, Y. Ding and C. Li, Pyroelectric effect in CdS nanorods decorated with a molecular Co-catalyst for hydrogen evolution, Nano Energy, 2020, 73, 104810 CrossRef CAS.
- S. Agarwal, X. Yu and A. Manthiram, A pair of metal organic framework (MOF)-derived oxygen reduction reaction (ORR) and oxygen evolution reaction (OER) catalysts for zinc-air batteries, Mater. Today Energy, 2020, 16, 100405 CrossRef.
- Q. Jiang, Y. Ren, Y. Yang, H. Liu, L. Wang, J. Li and L. Dai, High-activity and stability graphite felt supported by Fe, N, S co-doped carbon nanofibers derived from bimetal-organic framework for vanadium redox flow battery, Chem. Eng. J., 2023, 460, 141751 CrossRef CAS.
- Z. Jia, X. Zhang, Z. Gu and G. Wu, MOF-derived Ni-Co bimetal/porous carbon composites as electromagnetic wave absorber, Adv. Compos. Hybrid Mater., 2023, 6, 28 CrossRef CAS.
- Z. Xu, Y. Huang, C. Chen, L. Ding, Y. Zhu, Z. Zhang and Z. Guang, MOF-derived hollow Co(Ni)Se2/N-doped carbon composite material for preparation of sodium ion battery anode, Ceram. Interfaces, 2020, 46, 4532–4542 CrossRef CAS.
- N. Yao, Z. Fan, R. Meng, H. Jia and W. Luo, A cobalt hydroxide coated metal-organic framework for enhanced water oxidation electrocatalysis, Chem. Eng. J., 2021, 408, 127319 CrossRef CAS.
- S. Lu, H. Wu, J. Hou, L. Liu, J. Li, C. J. Harris, C. Y. Lao, Y. Guo, K. Xi, S. Ding, G. Gao, A. K. Cheetham and R. V. Kumar, Phase boundary engineering of metal-organic-framework-derived carbonaceous nickel selenides for sodium-ion batteries, Nano Res., 2020, 13, 2289–2298 CrossRef CAS.
- M. Yi, J. Ma, Y. Ren, H. Wang, L. Xie, Z. Zhu and J. Zhang, Ionic Liquid Meets MOF : A Facile Method to Optimize the Structure of CoSe2-NiSe2 Heterojunctions with N, P, and F Triple-Doped Carbon Using Ionic Liquid for Efficient Hydrogen Evolution and Flexible Supercapacitors, Adv. Sci., 2023, 10, 2206029 CrossRef CAS PubMed.
- Y. Jiang, M. Xie, F. Wu, Z. Ye, Y. Zhang, Z. Wang, Y. Zhou, L. Li and R. Chen, Cobalt Selenide Hollow Polyhedron Encapsulated in Graphene for High-Performance Lithium/Sodium Storage, Small, 2021, 17, 2102893 CrossRef CAS PubMed.
- X. Wang, J. He, B. Yu, B. Sun, D. Yang, X. Zhang, Q. Zhang, W. Zhang, L. Gu and Y. Chen, CoSe2 nanoparticles embedded MOF-derived Co-N-C nanoflake arrays as efficient and stable electrocatalyst for hydrogen evolution reaction, Appl. Catal., B, 2019, 258, 117996 CrossRef CAS.
- S. H. Chae, A. Muthurasu, T. Kim, J. S. Kim, M. S. Khil, M. Lee, H. Kim, J. Y. Lee and H. Y. Kim, Templated fabrication of perfectly aligned metal-organic framework-supported iron-doped copper-cobalt selenide nanostructure on hollow carbon nanofibers for an efficient trifunctional electrode material, Appl. Catal., B, 2021, 293, 120209 CrossRef CAS.
- G. Zhang, Y. Lu, Y. Yang, H. Yang, Z. Yang, S. Wang, W. Li, Y. Sun, J. Huang, Y. Luo, H. Y. Chen, Y. F. Liao, H. Ishii, S. Gull, M. Shakouri, H. G. Xue, Y. Hu and H. Pang, Dynamic Phase Transformations of Prussian Blue Analogue Crystals in Hydrotherms, J. Am. Chem. Soc., 2024, 16659–16669 CrossRef CAS PubMed.
- H. Xu, J. Han, B. Zhao, R. Sun, G. Zhong, G. Chen, Y. Yamauchi and B. Guan, A facile dual-template-directed successive assembly approach to hollow multi-shell mesoporous metal–organic framework particles, Nat. Commun., 2023, 14, 8062 CrossRef CAS PubMed.
- S. Zheng, Q. Li, H. Xue, H. Pang and Q. Xu, A highly alkaline-stable metal oxide@metal-organic framework composite for high-performance electrochemical energy storage, Natl. Sci. Rev., 2020, 7, 305–314 CrossRef CAS PubMed.
- Y. Lu, G. Zhang, H. Zhou, S. Cao, Y. Zhang, S. Wang and H. Pang, Enhanced Active Sites and Stability in Nano-MOFs for Electrochemical Energy Storage through Dual Regulation by Tannic Acid, Angew. Chem., Int. Ed., 2023, 62, e202311075 CrossRef CAS PubMed.
- S. L. Zhang, B. Y. Guan, X. F. Lu, S. Xi, Y. Du and X. W. Lou, Metal Atom-Doped Co3O4 Hierarchical Nanoplates for Electrocatalytic Oxygen Evolution, Adv. Mater., 2020, 32, 2002235 CrossRef CAS PubMed.
- C. Shi, S. Ye, X. Wang, F. Meng, J. Liu, T. Yang, W. Zhang, J. Wei, N. Ta, G. Q. Lu, M. Hu and J. Liu, Modular Construction of Prussian Blue Analog and TiO2 Dual-Compartment Janus Nanoreactor for Efficient Photocatalytic Water Splitting, Adv. Sci., 2021, 8, 2001987 CrossRef CAS PubMed.
- G. Yilmaz, C. F. Tan, M. Hong and G. W. Ho, Functional Defective Metal-Organic Coordinated Network of Mesostructured Nanoframes for Enhanced Electrocatalysis, Adv. Funct. Mater., 2018, 28, 1704177 CrossRef.
- J. Zhang, L. Yu, Y. Chen, X. F. Lu, S. Gao and X. W. Lou, Designed Formation of Double-Shelled Ni–Fe Layered-Double-Hydroxide Nanocages for Efficient Oxygen Evolution Reaction, Adv. Mater., 2020, 32, 1906432 CrossRef CAS PubMed.
- Y. Fang, D. Luan, Y. Chen, S. Gao and X. W. Lou, Synthesis of Copper-Substituted CoS2@CuxS Double-Shelled Nanoboxes by Sequential Ion Exchange for Efficient Sodium Storage, Angew. Chem., Int. Ed., 2020, 59, 2644–2648 CrossRef CAS PubMed.
- W. Bai, J. Chen, X. Wang, J. Zhu and Y. Fu, Transformation of ZIF-67 Nanocubes to ZIF-L Nanoframes, J. Am. Chem. Soc., 2024, 146, 79–83 CrossRef CAS PubMed.
- S. Cao, Y. Lu, Y. Tang, Y. Sun, H. Zhou, G. Zhang and X. Lin, Journal of Colloid And Interface Science Constructing ion-transport blockchain by polypyrrole to link CoTi-ZIF-9 derived carbon materials for high-performance seawater desalination, J. Colloid Interface Sci., 2024, 654, 466–475 CrossRef CAS PubMed.
- M. Du, P. Geng, C. Pei, X. Jiang, Y. Shan, W. Hu and L. Ni, Li – S Batteries High-Entropy Prussian Blue Analogues and Their Oxide Family as Sulfur Hosts for Lithium-Sulfur Batteries, Angew. Chem., Int. Ed., 2022, 61, e202209350 CrossRef CAS PubMed.
- T. Lv, G. Zhu, S. Dong, Q. Kong, Y. Peng, S. Jiang, G. Zhang, Z. Yang, S. Yang, X. Dong and H. Pang, Co-Intercalation of Dual Charge Carriers in Metal-Ion-Confining Layered Vanadium Oxide Nanobelts for Aqueous Zinc-Ion Batteries Angewandte, Angew. Chem., Int. Ed., 2023, 62, e202216089 CrossRef CAS PubMed.
- Q. Li, Y. Zhang, X. Guo, G. Zhang, Y. Yang, M. Du, T. Lv, H. Zhou, Y. Fan, Y. Chen, Y. Wang and H. Pang, Journal of Colloid And Interface Science Layered (AlO) 2 OH·VO 3 composite superstructures for ultralong lifespan aqueous zinc-ion batteries, J. Colloid Interface Sci., 2024, 663, 697–706 CrossRef CAS PubMed.
- T. Lv, Y. Peng, G. Zhang, S. Jiang, Z. Yang and S. Yang, How About Vanadium-Based Compounds as Cathode Materials for Aqueous Zinc Ion Batteries ?, Adv. Sci., 2023, 10, 2206907 CrossRef CAS PubMed.
- Y. Su, J. Hu, G. Yuan, G. Zhang, W. Wei and Y. Sun, Regulating Intramolecular Electron Transfer of Nickel-Based Coordinations through Ligand Engineering for Aqueous Batteries, Adv. Mater., 2023, 35, 2307003 CrossRef CAS PubMed.
- J. Zhao, C. Ge, Z. Zhao, Q. Wu, M. Liu, M. Yan, L. Yang, X. Wang and Z. Hu, Sub-nanometer-scale fine regulation of interlayer distance in Ni–Co layered double hydroxides leading to high-rate supercapacitors, Nano Energy, 2020, 76, 105026 CrossRef CAS.
- C. Xiong, B. Li, H. Liu, W. Zhao, C. Duan, H. Wu and Y. Ni, A smart porous wood-supported flower-like NiS/Ni conjunction with vitrimer co-effect as a multifunctional material with reshaping, shape-memory, and self-healing properties for applications in high-performance supercapacitors, catalysts, and sensors, J. Mater. Chem. A, 2020, 8, 10898–10908 RSC.
- B. Li, G. Zhang, K. Huang, L. Qiao and H. Pang, One-step synthesis of CoSn (OH) 6 nanocubes for high- performance all solid-state flexible supercapacitors, Rare Met., 2017, 36, 457–464 CrossRef CAS.
- Y. Wang, W. Kang, X. Pu, Y. Liang, B. Xu, X. Lu, D. Sun and Y. Cao, Template-directed synthesis of Co2P/MoSe2 in a N-doped carbon hollow structure for efficient and stable sodium/potassium ion storage, Nano Energy, 2022, 93, 106897 CrossRef CAS.
- C. Liu, Y. Bai, W. Li, F. Yang, G. Zhang and H. Pang, In Situ Growth of Three-Dimensional MXene/Metal–Organic Framework Composites for High-Performance Supercapacitors, Angew. Chem., Int. Ed., 2022, 61, e202116282 CrossRef CAS PubMed.
- Y. Bai, C. Liu, T. Chen, W. Li, S. Zheng, Y. Pi and Y. Luo, MXene-Copper/Cobalt Hybrids via Lewis Acidic Molten Salts Etching for High Performance Symmetric Supercapacitors, Angew. Chem., Int. Ed., 2021, 60, 25318–25322 CrossRef CAS PubMed.
- G. Zhang, H. Yang, H. Zhou, T. Huang, Y. Yang and G. Zhu, MXene-mediated Interfacial Growth of 2D-2D Heterostructured Nanomaterials as Cathodes for Zn-based Aqueous Batteries, Angew. Chem., Int. Ed., 2024, 63, e202402903 Search PubMed.
- J. Yan, T. Liu, X. Liu, Y. Yan and Y. Huang, Metal-organic framework-based materials for flexible supercapacitor application, Coord. Chem. Rev., 2022, 452, 214300 CrossRef CAS.
- M. Z. Iqbal and U. Aziz, Supercapattery: Merging of battery-supercapacitor electrodes for hybrid energy storage devices, J. Energy Storage, 2022, 46, 103823 CrossRef.
- W. Wang, X. Zhu and L. Fu, Touch ablation of lithium dendrites via liquid metal for high-rate and long-lived batteries, CCS Chem., 2021, 3, 686–695 CrossRef CAS.
- Y. Li, L. Ma, Z. Yi, Y. Zhao, J. Mao, S. Yang, W. Ruan, D. Xiao, N. Mubarak, J. Wu, T. S. Zhao, Q. Chen and J. K. Kim, Metal-organic framework-derived carbon as a positive electrode for high-performance vanadium redox flow batteries, J. Mater. Chem. A, 2021, 9, 5648–5656 RSC.
- Y. Zhang, L. Liu, L. Zhao, C. Hou, M. Huang, H. Algadi and D. Li, Sandwich-like-CoMoP 2/MoP heterostructures coupling N, P co-doped carbon nanosheets as advanced anodes for high-performance lithium-ion batteries, Adv. Compos. Hybrid Mater., 2022, 5, 2601–2610 CrossRef CAS.
- Y. Shen, Y. Jiang, Z. Yang, J. Dong, W. Yang, Q. An and L. Mai, Electronic Structure Modulation in MoO2/MoP Heterostructure to Induce Fast Electronic/Ionic Diffusion Kinetics for Lithium Storage, Adv. Sci., 2022, 9, 2104504 CrossRef CAS PubMed.
- K. Ngoc, Q. Liang, C. Du, J. Zhao, A. Iing, Y. Tok, H. Mao and Q. Yan, Nano Today Nanostructured metallic transition metal carbides, nitrides, phosphides, and borides for energy storage and conversion, Nano Today, 2019, 25, 99–121 CrossRef.
- D. Choi, S. Lim and D. Han, Advanced metal–organic frameworks for aqueous sodium-ion rechargeable batteries, J. Energy Chem., 2021, 53, 396–406 CrossRef CAS.
- J. Liu, J. Wang, L. Zhu, X. Chen, Q. Ma, L. Wang, X. Wang and W. Yan, A high-safety and multifunctional MOFs modified aramid nanofiber separator for lithium-sulfur batteries., Chem. Eng. J., 2021, 411, 128540 CrossRef CAS.
- X. Liu, P. Geng, G. Zhang, Y. Zhang, F. Dou and H. Pang, Synthesis of 2D Co-MOF Nanosheets and Low-Temperature Calcination Activation for Lithium-Ion Batteries, Inorg. Chem., 2023, 62, 6527–6536 CrossRef CAS PubMed.
- Z. Qiu, Y. Li, Y. Gao, Z. Meng, Y. Sun and Y. Bai, 2D MOF-assisted Pyrolysis-displacement-alloying Synthesis of High- entropy Alloy Nanoparticles Library for Efficient Electrocatalytic Angewandte, Angew. Chem., Int. Ed., 2023, 62, e202306881 CrossRef CAS PubMed.
- M. Du, Q. Li, G. Zhang, F. Wang and H. Pang, Metal – Organic Framework-Based Sulfur-Loaded Materials, Energy Environ. Mater., 2022, 5, 215–230 CrossRef CAS.
- H. Zhang, M. Zhang, R. Liu, T. He, L. Xiang, X. Wu, Z. Piao, Y. Jia, C. Zhang, H. Li, F. Xu, G. Zhou and Y. Mai, Fe3O4-doped mesoporous carbon cathode with a plumber's nightmare structure for high-performance Li-S batteries, Nat. Commun., 2024, 15, 5451 CrossRef CAS PubMed.
- S. Tian, Q. Zeng, G. Liu, J. Huang, X. Sun, D. Wang, H. Yang, Z. Liu, X. Mo, Z. Wang, K. Tao and S. Peng, Multi-Dimensional Composite Frame as Bifunctional Catalytic Medium for Ultra-Fast Charging Lithium–Sulfur Battery, Nano-Micro Lett., 2022, 14, 196 CrossRef CAS PubMed.
- M. Du, X. Wang, P. Geng, Q. Li, Y. Gu, Y. An and H. Pang, Polypyrrole-enveloped Prussian blue nanocubes with multi-metal synergistic adsorption toward lithium polysulfides : high-performance lithium-sulfur batteries, Chem. Eng. J., 2021, 420, 130518 CrossRef CAS.
- W. Li, X. Guo, P. Geng, M. Du, Q. Jing, X. Chen, G. Zhang, H. Li, Q. Xu, P. Braunstein and H. Pang, Rational Design and General Synthesis of Multimetallic Metal–Organic Framework Nano-Octahedra for Enhanced Li–S Battery, Adv. Mater., 2021, 33, 2105163 CrossRef CAS.
- C. Li, L. Liu, J. Kang, Y. Xiao, Y. Feng, F. F. Cao and H. Zhang, Pristine MOF and COF materials for advanced batteries, Energy Storage Mater., 2020, 31, 115–134 CrossRef.
- Z. Wu, S. Chen, L. Wang, Q. Deng, Z. Zeng, J. Wang and S. Deng, Implanting nickel and cobalt phosphide into well-defined carbon nanocages : A synergistic adsorption-electrocatalysis separator mediator for durable high-power Li-S batteries, Energy Storage Mater., 2021, 38, 381–388 CrossRef.
- D. Luo, C. Li, Y. Zhang, Q. Ma, C. Ma, Y. Nie, M. Li, X. Weng, R. Huang, Y. Zhao, L. Shui, X. Wang and Z. Chen, Design of Quasi-MOF Nanospheres as a Dynamic Electrocatalyst toward Accelerated Sulfur Reduction Reaction for High-Performance Lithium–Sulfur Batteries, Adv. Mater., 2022, 34, 2105541 CrossRef CAS PubMed.
- Z. Li, J. Wang, H. Yuan, Y. Yu and Y. Tan, Recent Progress and Challenge in Metal–Organic Frameworks for Lithium–Sulfur Battery Separators, Adv. Funct. Mater., 2024, 2405890 CrossRef.
- B. E. R. Snyder, A. B. Turkiewicz, H. Furukawa, M. V. Paley, E. O. Velasquez, M. N. Dods and J. R. Long, A ligand insertion mechanism for cooperative NH3 capture in metal–organic frameworks, Nature, 2023, 613, 287–291 CrossRef CAS PubMed.
- C. L. Wenqiang Wang, L. Hua, Y. Zhang and G. Wang, A Conductive Binder Based on Mesoscopic Interpenetration with Polysulfides Capturing Skeleton and Redox Intermediates Network for Lithium Sulfur Batteries, Angew. Chem., Int. Ed., 2024, e202405920 Search PubMed.
- L. Zhong, C. Jiang, M. Zheng, X. Peng, T. Liu, S. Xi, X. Chi, Q. Zhang, L. Gu, S. Zhang, G. Shi, L. Zhang, K. Wu, Z. Chen, T. Li, M. Dahbi, J. Alami, K. Amine and J. Lu, Wood Carbon Based Single-Atom Catalyst for Rechargeable Zn-Air Batteries, ACS Energy Lett., 2021, 6, 3624–3633 CrossRef CAS.
- C. Zhe-qin, Z. Xiao-cong, X. Yong-min, L. Jia-ming, X. Zhi-feng and W. Rui-xiang, A high-performance nitrogen-rich ZIF-8-derived Fe-NC electrocatalyst for the oxygen reduction reaction, J. Alloys Compd., 2021, 884, 160980 CrossRef.
- Y. Zhang, R. Jiang, Z. Wang, Y. Xue, J. Sun and Y. Guo, (Fe,N-codoped carbon nanotube)/(Fe-based nanoparticle) nanohybrid derived from Fe-doped g-C3N4: A superior catalyst for oxygen reduction reaction, J. Colloid Interface Sci., 2020, 579, 391–400 CrossRef CAS PubMed.
- L. Zong, X. Chen, S. Dou, K. Fan, Z. Wang, W. Zhang, Y. Du, J. Xu, X. Jia, Q. Zhang, X. Li, Y. Deng, Y. Chen and L. Wang, Stable confinement of Fe/Fe3C in Fe, N-codoped carbon nanotube towards robust zinc-air batteries, Chin. Chem. Lett., 2021, 32, 1121–1126 CrossRef CAS.
- X. Lu, L. Ge, P. Yang, O. Levin, V. Kondratiev, Z. Qu, L. Liu, J. Zhang and M. An, N-doped carbon nanosheets with ultra-high specific surface area for boosting oxygen reduction reaction in Zn-air batteries, Appl. Surf. Sci., 2021, 562, 150114 CrossRef CAS.
- Y. Zhang, Z. Chen, J. Tian, M. Sun, D. Yuan and L. Zhang, Nitrogen doped CuCo2O4 nanoparticles anchored on beaded-like carbon nanofibers as an efficient bifunctional oxygen catalyst toward zinc-air battery, J. Colloid Interface Sci., 2022, 608, 1105–1115 CrossRef CAS PubMed.
- J. Zhou, Q. Yang, Q. Xie, H. Ou, X. Lin, A. Zeb, L. Hu, Y. Wu and G. Ma, Recent progress in Co–based metal–organic framework derivatives for advanced batteries, J. Mater. Sci. Technol., 2022, 96, 262–284 CrossRef CAS.
- X. Wang, K. Li, D. Yang, X. Yang, M. Xiao, L. Zheng, W. Xing, C. Liu and J. Zhu, F Doping-Induced Multicomponent Synergistic Active Site Construction toward High-Efficiency Bifunctional Oxygen Electrocatalysis for Rechargeable Zn–Air Batteries, Small, 2024, 20, 2310250 CrossRef CAS PubMed.
- Y. Wang, L. Song, L. Zheng, Y. Wang, J. Wu and J. Xu, Reversible Carbon Dioxide/Lithium Oxalate Regulation toward Advanced Aprotic Lithium Carbon Dioxide Battery, Angew. Chem., Int. Ed., 2024, 63, e202400132 CrossRef CAS PubMed.
- X. Wang, D. Guan, C. Miao, D. Kong, L. Zheng and J. Xu, Metal − Organic Framework-Based Mixed Conductors Achieve Highly Stable Photo-assisted Solid-State Lithium − Oxygen Batteries, J. Am. Chem. Soc., 2023, 145, 5718–5729 CrossRef CAS PubMed.
- K. Srinivas, Y. Lu, Y. Chen, W. Zhang and D. Yang, FeNi3-Fe3O4 Heterogeneous Nanoparticles Anchored on 2D MOF Nanosheets/1D CNT Matrix as Highly Efficient Bifunctional Electrocatalysts for Water Splitting, ACS Sustainable Chem. Eng., 2020, 8, 3820–3831 CrossRef CAS.
- E. Hu, Y. Yao, Y. Cui and G. Qian, Strategies for the enhanced water splitting activity over metal–organic frameworks-based electrocatalysts and photocatalysts, Mater. Today Nano, 2021, 15, 100124 CrossRef CAS.
- K. Srinivas, Y. Chen, B. Wang, B. Yu, Y. Lu, Z. Su, W. Zhang and D. Yang, Metal-Organic Framework-Derived Fe-Doped Ni3Fe/NiFe2O4Heteronanoparticle-Decorated Carbon Nanotube Network as a Highly Efficient and Durable Bifunctional Electrocatalyst, ACS Appl. Mater. Interfaces, 2020, 12, 55782–55794 CrossRef CAS PubMed.
- D. Yang, Z. Su, Y. Chen, Y. Lu, B. Yu, K. Srinivas, B. Wang and W. Zhang, Double-shelled hollow bimetallic phosphide nanospheres anchored on nitrogen-doped graphene for boosting water electrolysis, J. Mater. Chem. A, 2020, 8, 22222–22229 RSC.
- M. R. Gao, J. X. Liang, Y. R. Zheng, Y. F. Xu, J. Jiang, Q. Gao, J. Li and S. H. Yu, An efficient molybdenum disulfide/cobalt diselenide hybrid catalyst for electrochemical hydrogen generation, Nat. Commun., 2015, 6, 5982 CrossRef CAS PubMed.
- X. Sun, R. B. Araujo, E. C. dos Santos, Y. Sang, H. Liu and X. Yu, Advancing electrocatalytic reactions through mapping key intermediates to active sites via descriptors, Chem. Soc. Rev., 2024, 53, 7301–7634 RSC.
- H. Wu, C. Feng, L. Zhang, J. Zhang and D. P. Wilkinson, Non-noble Metal Electrocatalysts for the Hydrogen Evolution Reaction in Water Electrolysis, Electrochem. Energy Rev., 2021, 4, 473–507 CrossRef CAS.
- Y. Park, H. Kim, T. Lee, Y. Hong, W. Jeong, S. Kim and D. Ha, Design of nanocatalyst for electrode structure : Electrophoretic deposition of iron phosphide nanoparticles to produce a highly active hydrogen evolution reaction catalyst, Chem. Eng. J., 2022, 431, 133217 CrossRef CAS.
- Y. Zhou, R. Abazari, J. Chen, M. Tahir, A. Kumar, R. R. Ikreedeegh, E. Rani, H. Singh and A. M. Kirillov, Bimetallic metal–organic frameworks and MOF-derived composites: Recent progress on electro- and photoelectrocatalytic applications, Coord. Chem. Rev., 2022, 451, 214264 CrossRef CAS.
- W. Feng, C. Liu, G. Zhang, H. Yang, Y. Su and Y. Sun, EnergyChem Tuning the local coordination environment of single-atom catalysts for enhanced electrocatalytic activity, EnergyChem, 2024, 6, 100119 CrossRef CAS.
- F. Ahmadijokani, H. Molavi, M. Rezakazemi, S. Tajahmadi, A. Bahi, F. Ko, T. M. Aminabhavi, J. R. Li and M. Arjmand, UiO-66 metal–organic frameworks in water treatment: A critical review, Prog. Mater. Sci., 2022, 125, 100904 CrossRef CAS.
- C. Zhou, K. Ma, Z. Zhuang, M. Ran, G. Shu, C. Wang and L. Song, Tuning the Local Environment of Pt Species at CNT@MO2−x(M = Sn and Ce) Heterointerfaces for Boosted Alkaline Hydrogen Evolution, J. Am. Chem. Soc., 2024, 146(31), 21453 CrossRef PubMed.
- G. Chen, W. Chen, R. Lu, C. Ma, Z. Zhang, Z. Huang, J. Weng, Z. Wang, Y. Han and W. Huang, Near-Atomic-Scale Superfine Alloy Clusters for Ultrastable Acidic Hydrogen Electrocatalysis, J. Am. Chem. Soc., 2023, 145, 22069–22078 CrossRef CAS PubMed.
- Y. Zhong, P. Liao, J. Kang, Q. Liu, S. Wang, S. Li, X. Liu and G. Li, Locking Effect in Metal@MOF with Superior Stability for Highly Chemoselective Catalysis, J. Am. Chem. Soc., 2023, 145, 4659–4666 CrossRef CAS PubMed.
- B. Wang, Y. Chen, X. Wang, J. Ramkumar, X. Zhang, B. Yu, D. Yang, M. Karpuraranjith and W. Zhang, RGO wrapped trimetallic sulfide nanowires as an efficient bifunctional catalyst for electrocatalytic oxygen evolution and photocatalytic organic degradation, J. Mater. Chem. A, 2020, 8, 13558–13571 RSC.
- D. Yang, Y. Chen, Z. Su, X. Zhang, W. Zhang and K. Srinivas, Organic carboxylate-based MOFs and derivatives for electrocatalytic water oxidation, Coord. Chem. Rev., 2021, 428, 213619 CrossRef CAS.
- L. Zhang, Y. T. Ma, J. J. Duan, Y. Q. Yao, J. J. Feng and A. J. Wang,
In situ construction of 3D hetero-structured sulfur-doped nanoflower-like FeNi LDH decorated with NiCo Prussian blue analogue cubes as efficient electrocatalysts for boosting oxygen evolution reaction, J. Colloid Interface Sci., 2022, 611, 205–214 CrossRef CAS PubMed.
- J. Chang, S. Zang, F. Song, W. Wang, D. Wu, F. Xu, K. Jiang and Z. Gao, Heterostructured nickel, iron sulfide@nitrogen, sulfur co-doped carbon hybrid with efficient interfacial charge redistribution as bifunctional catalyst for water electrolysis, Appl. Catal., A, 2022, 630, 118459 CrossRef CAS.
- C. Yan, J. Huang, C. Wu, Y. Li, Y. Tan, L. Zhang, Y. Sun, X. Huang and J. Xiong,
In situ formed NiS/Ni coupled interface for efficient oxygen evolution and hydrogen evolution, J. Mater. Sci. Technol., 2020, 42, 10–16 CrossRef CAS.
- D. Chen, J. Zhu, X. Mu, R. Cheng, W. Li, S. Liu, Z. Pu, C. Lin and S. Mu, Nitrogen-Doped carbon coupled FeNi3 intermetallic compound as advanced bifunctional electrocatalyst for OER, ORR and zn-air batteries, Appl. Catal., B, 2020, 268, 118729 CrossRef CAS.
- X. Wang, G. Zhang, W. Yin, S. Zheng, Q. Kong, J. Tian and H. Pang, Metal–organic framework-derived phosphide nanomaterials for electrochemical applications, Carbon Energy, 2022, 4, 246–281 CrossRef CAS.
- J. Ding, D. Guo, N. Wang, H. F. Wang, X. Yang, K. Shen, L. Chen and Y. Li, Defect Engineered Metal–Organic Framework with Accelerated Structural Transformation for Efficient Oxygen Evolution Reaction, Angew. Chem., Int. Ed., 2023, 62, e202311909 CrossRef CAS PubMed.
- Y. Li, Y. Wu, T. Li, Y. Yao, H. Cai, J. Gao and G. Qian, Amorphous Engineering of Scalable Metal-Organic Framework-Derived Electrocatalyst for Highly Efficient Oxygen Evolution Reaction, Small, 2024, 20, 2311356 CrossRef CAS PubMed.
- E. Vijayakumar, S. Ramakrishnan, C. Sathiskumar, D. J. Yoo, J. Balamurugan, H. S. Noh, D. Kwon, Y. H. Kim and H. Lee, MOF-derived CoP-nitrogen-doped carbon@NiFeP nanoflakes as an efficient and durable electrocatalyst with multiple catalytically active sites for OER, HER, ORR and rechargeable zinc-air batteries, Chem. Eng. J., 2022, 428, 131115 CrossRef CAS.
- A. Parra-Puerto, K. L. Ng, K. Fahy, A. E. Goode, M. P. Ryan and A. Kucernak, Supported Transition Metal Phosphides: Activity Survey for HER, ORR, OER, and Corrosion Resistance in Acid and Alkaline Electrolytes, ACS Catal., 2019, 9, 11515–11529 CrossRef CAS.
- X. Li, D. Wu, T. Hua, X. Lan, S. Han, J. Cheng, K. S. Du, Y. Hu and Y. Chen, Micro/macrostructure and multicomponent design of catalysts by MOF-derived strategy: Opportunities for the application of nanomaterials-based advanced oxidation processes in wastewater treatment, Sci. Total Environ., 2022, 804, 150096 CrossRef CAS PubMed.
- D. Yang, Z. Liang, P. Tang, C. Zhang, M. Tang, Q. Li, J. J. Biendicho, J. Li, M. Heggen, R. E. Dunin-Borkowski, M. Xu, J. Llorca, J. Arbiol, J. R. Morante, S. L. Chou and A. Cabot, A High Conductivity 1D π–d Conjugated Metal–Organic Framework with Efficient Polysulfide Trapping-Diffusion-Catalysis in Lithium–Sulfur Batteries, Adv. Mater., 2022, 34, 2108835 CrossRef CAS PubMed.
- X. Du, S. Wang, F. Ye and Z. Qingrui, Derivatives of metal-organic frameworks for heterogeneous Fenton-like processes: From preparation to performance and mechanisms in wastewater purification – A mini review, Environ. Res., 2022, 206, 112414 CrossRef CAS PubMed.
- A. Kondori, Z. Jiang, M. Esmaeilirad, M. Tamadoni Saray, A. Kakekhani, K. Kucuk, P. Navarro Munoz Delgado, S. Maghsoudipour, J. Hayes, C. S. Johnson, C. U. Segre, R. Shahbazian-Yassar, A. M. Rappe and M. Asadi, Kinetically Stable Oxide Overlayers on Mo3P Nanoparticles Enabling Lithium–Air Batteries with Low Overpotentials and Long Cycle Life, Adv. Mater., 2020, 32, 2004028 CrossRef CAS PubMed.
- C. Du, Y. Zhang, Z. Zhang, L. Zhou, G. Yu, X. Wen, T. Chi, G. Wang, Y. Su, F. Deng, Y. Lv and H. Zhu, Fe-based metal organic frameworks (Fe-MOFs) for organic pollutants removal via photo-Fenton: A review, Chem. Eng. J., 2022, 431, 133932 CrossRef CAS.
- Y. Wang, R. Wang, N. Lin, J. Xu, X. Liu, N. Liu and X. Zhang, Degradation of norfloxacin by MOF-derived lamellar carbon nanocomposites based on microwave-driven Fenton reaction: Improved Fe(III)/Fe(II) cycle, Chemosphere, 2022, 293, 133614 CrossRef CAS PubMed.
- M. Li, H. Sun, J. Yang, M. Humayun, L. Li, X. Xu, X. Xue, A. Habibi-Yangjeh, K. Temst and C. Wang, Mono-coordinated metallocene ligands endow metal-organic frameworks with highly efficient oxygen evolution and urea electrolysis, Chem. Eng. J., 2022, 430, 132733 CrossRef CAS.
- R. Hao, J. T. Ren, X. W. Lv, W. Li, Y. P. Liu and Z. Y. Yuan, N-doped porous carbon hollow microspheres encapsulated with iron-based nanocomposites as advanced bifunctional catalysts for rechargeable Zn-air battery, J. Energy Chem., 2020, 49, 14–21 CrossRef.
- W. Zhang, C. Deng, W. Wang, H. Sheng and J. Zhao, Achieving Almost 100% Selectivity in Photocatalytic CO 2 Reduction to Methane via In situ Atmosphere Regulation Strategy, Adv. Mater., 2024, 36, 2405825 CrossRef CAS PubMed.
- Z. Li, C. Liu, Q. Deng and W. Deng, Rational Design of Covalent Organic Frameworks as Photocatalysts for Water Splitting, Adv. Funct. Mater., 2024, 34, 2402676 CrossRef.
- A. Shu, C. Qin, M. Li, L. Zhao, Z. Shangguan, Z. Shu, X. Yuan, M. Zhu, Y. Wu and H. Wang, Electric Effects Reinforce Charge Carriers Behaviour for Photocatalysis, Energy Environ. Sci., 2024, 17, 4907–4928 RSC.
- K. Kinashi, Y. Kambe, M. Misaki, Y. Koshiba, K. Ishida and Y. Ueda, Synthesis, characterization, photo-induced alignment, and surface orientation of poly(9,9-dioctylfluorene-alt-azobenzene)s, J. Polym. Sci., Part A: Polym. Chem., 2012, 50, 5107–5114 CrossRef CAS.
- M. Nemiwal, V. Subbaramaiah, T. C. Zhang and D. Kumar, Recent advances in visible-light-driven carbon dioxide reduction by metal-organic frameworks, Sci. Total Environ., 2021, 762, 144101 CrossRef CAS PubMed.
- M. Wen, G. Li, H. Liu, J. Chen, T. An and H. Yamashita, Metal-organic framework-based nanomaterials for adsorption and photocatalytic degradation of gaseous pollutants: Recent progress and challenges, Environ. Sci. Nano, 2019, 6, 1006–1025 RSC.
- M. S. Ramyashree, S. Shanmuga Priya, N. C. Freudenberg, K. Sudhakar and M. Tahir, Metal-organic framework-based photocatalysts for carbon dioxide reduction to methanol: A review on progress and application, J. CO2 Util., 2021, 43, 101374 CrossRef.
- W. Shao, C. He, M. Zhou, C. Yang, Y. Gao, S. Li, L. Ma, L. Qiu, C. Cheng and C. Zhao, Core-shell-structured mof-derived 2d hierarchical nanocatalysts with enhanced fenton-like activities, J. Mater. Chem. A, 2020, 8, 3168–e3179 RSC.
- J. Zhang, Y. Hu, J. Qin, Z. Yang and M. Fu, TiO2-UiO-66-NH2 nanocomposites as efficient photocatalysts for the oxidation of VOCs, Chem. Eng. J., 2020, 385, 123814 CrossRef CAS.
- E. S. Avraham, O. Fleker, L. Benisvy, L. Oakes, C. L. Pint and G. D. Nessim, Inducing porosity and growing carbon nanofibers in ferroin perchlorate: An example of morphological transitions in coordination complexes, J. Solid State Chem., 2017, 253, 21–28 CrossRef CAS.
- X. Chen, W. Li, G. Zhang, F. Sun, Q. Jing and H. Pang, Highly stable and activated Cerium-based MOFs superstructures for ultrahigh selective uranium(VI) capture from simulated seawater, Mater. Today Chem., 2022, 23, 100705 CrossRef CAS.
- M. Khalid, A. Hassan, A. M. B. Honorato, F. N. Crespilho and H. Varela, Nano-flocks of a bimetallic organic framework for efficient hydrogen evolution electrocatalysis, Chem. Commun., 2018, 54, 11048 RSC.
- W. Z. Cheng, J. L. Liang, H. B. Yin, Y. J. Wang, W. F. Yan and J. N. Zhang, Bifunctional iron-phtalocyanine metal–organic framework catalyst for ORR, OER and rechargeable zinc–air battery, Rare Met., 2020, 39, 815 CrossRef CAS.
- F. Ahmadijokani, R. Mohammadkhani, S. Ahmadipouya, A. Shokrgozar, M. Rezakazemi, H. Molavi, T. M. Aminabhavi and M. Arjmand, Superior chemical stability of UiO-66 metal-organic frameworks (MOFs) for selective dye adsorption, Chem. Eng. J., 2020, 399, 125346 CrossRef CAS.
- Y. Z. Zhang, X. S. Song and J. M. Yang, Modulation of driving forces fo UiO-66 analog adsorbents by decoration with amino functional groups: Superior adsorption of hazardous dyes, J. Mol. Struct., 2020, 1220, 128716 CrossRef CAS.
Footnote |
† These authors contributed equally to this work. |
|
This journal is © the Partner Organisations 2024 |
Click here to see how this site uses Cookies. View our privacy policy here.