DOI:
10.1039/D4QI01603C
(Research Article)
Inorg. Chem. Front., 2024,
11, 5905-5912
Multi-step cation substitution facilitating the exploration of potential infrared nonlinear optical materials†
Received
26th June 2024
, Accepted 28th July 2024
First published on 29th July 2024
Abstract
Crystal structure regulation and optical performance enhancement are huge challenges, especially for the laborious and inefficient trial-and-error method, in the research on infrared nonlinear optical (IR NLO) materials. In this work, multi-step cation substitutions were adopted to modulate the crystal structure for the effective design of well-performing IR NLO materials. Specifically, starting from the famous AgGaS2 (AGS, I
2d) crystal, Ag2CdSiS4 (Pmn21) with diamond-like crystal structure, BaAg2SiS4 (I
2m) with three-dimensional tunnel structure and LaAgSiS4 (Ama2) with two-dimensional layer structure were designed and experimentally synthesized through multi-step cation substitutions. Structural analysis reveals the fundamental reason of their tetrahedral framework transformation: the size effect of cations and the reduction of component tetrahedra caused by cationic substitution change the assembly mode of the tetrahedral units. Additionally, three non-centrosymmetric Ag-based thiosilicates exhibit wide transmittance range (0.5–17 μm), higher laser-induced damage thresholds (2 times that of AGS) and significant phase-matchable second harmonic generation (SHG) enhancement from 0.6 to 3.2 times that of AGS. This work demonstrates that multi-step cation substitution is an effective way to extend non-centrosymmetric structures, which facilitate the exploration of potential infrared nonlinear optical materials.
Introduction
With the advancement of optical fields such as optical communication, optical imaging, laser guidance, and environmental monitoring,1–4 there is a growing demand for infrared nonlinear optical (IR NLO) crystals with exceptional properties including large second harmonic generation (SHG) response, phase matching capabilities (suitable Δn), high laser-induced damage threshold (LIDT), wide light transmission range, and good physical and chemical stability.5 Prominent commercially available IR NLO materials include chalcopyrite-type ionic crystals AgGaS2 (AGS), AgGaSe2 (AGSe), and ZnGeP2 (ZGP), which are known for their large second harmonic generation (SHG) responses and broad IR transparency. However, their potential applications are significantly constrained by inherent limitations, including a low laser induced damage threshold (LIDT), poor phase matching ability and harmful two-photon absorption.6–8
Great efforts have been devoted to the exploration of new IR SHG materials.9–12 A series of tetrahedron-based compounds, such as AgGa2PS6 (1 × AGS), Hg3P2S8 (4.2 × AGS) and Li4MgGe2S7 (0.7 × AGS), were produced by combinations between different tetrahedral active groups.13–16 Choosing [AsS3]3− and [SbS3]3− anions with stereochemical lone pair electrons or π-conjugated planar triangular [BS3]3− as infrared nonlinear optical genes led to the isolation of new IR SHG crystals such as LaBS3 (1.2 × AGS) and BaHgSe2 (1.5 × AGS).17–20 Alkaline-earth or transition metal cations were introduced to adjust the band gaps as in BaGa4S7 (0.9 × AGS) and Cd4SiS6 (1.3 × AGS).21–26 Searching for new IR NLO material systems, such as pnictides, oxyhalides and adducts, led to the discovery of new materials such as RuSi4P4 (1.4 × AGS), Pb17O8Cl18 (2 × AGS), Pb18O8Cl15I5 (1 × AGS) and (CuBr)7(P4Se3)3 (3.5 × AGS).27–31
Despite great achievements, most previous explorations are based on the laborious and inefficient trial-and-error synthetic methods.32 Researchers are starting to find more efficient ways to design new IR NLO materials. One good way is based on computer-assisted structure and optical property prediction, which led to the discovery of Zn3(PS4)2 (52 × KH2PO4), Zn2BS3Br (2 × AGS), etc.33–35 Another strategy is to screen potential nonlinear optical materials, such as LiGaSe2 (2 × AGS), from the database.36 In addition, chemical substitutions were also considered an effective way to explore novel nonlinear optical crystals. For example, BiFSeO3 with strong SHG response of 13.5 times than that of KH2(PO)4 at 1064 nm was obtained using [SeO3]2− and F− to simultaneously aliovalently substitute the [IO3]− and O2− anions.37 However, in previous reports, such works have only involved single-step substitution and similar crystal structures. Related systematic studies on structure and performance regulation are scarce, prompting us to utilize multi-step cation substitutions to extend more crystal structure types.38,39
To experimentally prove the effectiveness of multi-step cation substitution, the famous AGS was selected as the template due to its dominant diamond-like structure.40–44 First, Ag2CdSiS4 with a similar diamond-like structure was designed using one Cd2+ and one Si4+ to substitute two Ga3+ in AGS. Second, to preliminarily break the tetrahedral close-packed framework, Ba2+ with larger radius was adopted to substitute Cd2+ in Ag2CdSiS4, producing the three-dimensional tunnel structural BaAg2SiS4. Finally, by substituting one Ba2+ and one Ag+ with a La3+, LaAgSiS4 was created, forming two-dimensional [AgSiS4]3− layers with interlayer space filled by La3+ cations. Although Ag2CdSiS4 and BaAg2SiS4 were reported before, related studies on systematic structure regulation and IR NLO property are still absent.45,46 Structure analysis revealed the fundamental reasons of the tetrahedral framework transformation: the size effect of cations and the reduction of the component tetrahedra caused by cationic substitution change the assembly mode of basic tetrahedral units. Furthermore, Ag2CdSiS4, BaAg2SiS4 and LaAgSiS4 crystals exhibit wide transmittance range (0.5–17 μm), relatively high laser-induced damage thresholds (2 times that of AGS) and significant phase matchable SHG response enhancement (0.6, 1.4 and 3.2 times of AGS). Our work proves that multi-step cation substitution is an effective method to explore potential IR NLO crystals.
Results and discussion
Phase analysis
Single crystals of Ag2CdSiS4, BaAg2SiS4 and LaAgSiS4 were obtained through high-temperature solid state reactions under vacuum (ESI, Fig. S1†). Due to the deviations in the exposure of the sample's crystal planes from the ideal ones, even though the experimental and theoretical powder X-ray diffraction patterns may not look very similar, their purities are still confirmed by the same characteristic peak positions (Fig. S2†). The molar ratios of Ag
:
M
:
Si
:
S obtained by energy-dispersive X-ray spectroscopy are 2.1
:
1
:
1
:
4, 1
:
2.1
:
1.1
:
3.9 and 1
:
1.1
:
1.1
:
4.1 for Ag2CdSiS4, BaAg2SiS4 and LaAgSiS4, respectively, which are in good agreement with those determined from crystal structure analyses (Fig. S3†).
Crystal structures
Similar to AGS, AgCdSiS4 features a three-dimensional diamond-like crystal structure that is close-packed by the vertex-shared AgS4, CdS4 and SiS4 tetrahedra (Fig. 1a and b). Ag2CdSiS4 crystalizes in the orthorhombic polar space group Pmn21 (no. 31) (Table S1†). Its asymmetric unit consists of one Ag (4b), one Cd (2a), one Si (2a) and 3 S atoms occupying two 2a and one 4b sites (Table S2†). However, when two Ga3+ cations were replaced by one Cd2+ and one Si4+, the AgS4, CdS4 and SiS4 tetrahedra in Ag2CdSiS4 exhibits obvious distortion due to the mismatch of geometric sizes, which is manifested in the bond length elongation of the Ag(1)–S(1)#2 (2.973(5) Å), Cd(1)–S(2)#6 (2.929(8) Å) and Si(1)–S(3)#7 (2.456(8) Å) (Table S3†). This case indicates that substitution between different component tetrahedra only change their arrangements and distortions but the diamond-like tetrahedral framework is retained.
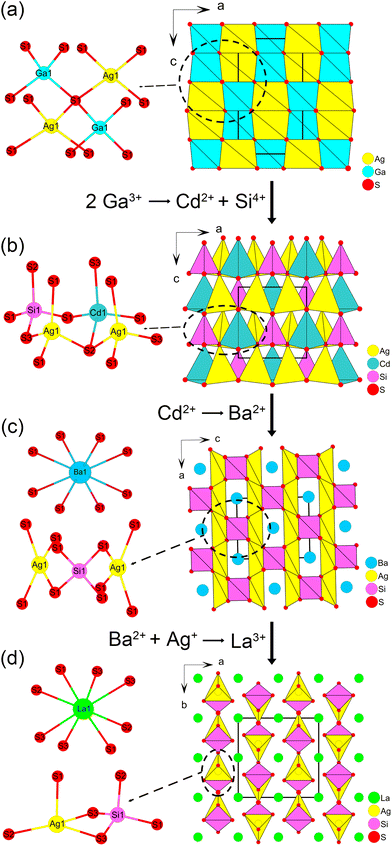 |
| Fig. 1 Vertex-shared AgS4, GaS4 tetrahedra; three-dimensional diamond-like AgGaS2 crystal structures (a); vertex-shared AgS4, CdS4 and SiS4 tetrahedra; three-dimensional tetrahedra closed-packed AgCdSiS4 crystal structures (b); coordination environment of Ba2+; vertex-shared AgS4 and SiS4 tetrahedra; three-dimensional tunnel structure of BaAg2SiS4 (c); coordination environment of La3+; edge-shared AgS4 and SiS4 tetrahedra; crystal structure of LaAgSiS4 composed of [AgSiS4]3− layers (d). | |
To induce structural transformation, the Cd2+ ions in Ag2CdSiS4 were replaced by larger Ba2+ cations. BaAg2SiS4 crystallize in the tetragonal space group I
2m (Table S1†), and its asymmetric unit contains one Ba (2a), one Ag (4d), one Si(2b), and one S (8i) atom. As shown in Fig. 1b, the Ba2+ ion is eight-coordinated by surrounding atoms in a distorted bicapped trigonal prismatic geometry, with Ba–S bond lengths ranging from 3.236(12) to 3.301(13) Å. Both Ag and Si are tetrahedrally coordinated by S atoms with Ag–S distance of 2.5997(11) Å and Si–S distance of 2.1245(3) Å (Table S3†). The vertex-sharing interconnection of AgS4 and SiS4 tetrahedra result in a three-dimensional open anionic framework with 1D tunnels of 4-member rings, which are filled by charge-balance Ba2+ cations (Fig. 1c).
In order to further reduce the dimensionality of the tetrahedral anionic framework, the La3+ with higher valence was chosen to simultaneously substitute one Ba2+ and one Ag+ in BaAg2SiS4. LaAgSiS4 crystallizes in the orthorhombic polar space group Ama2 (no. 40). The asymmetric unit of LaAgSiS4 contains one La (4a), one Ag (4b) and three S (4b and 8c) atoms. The La3+ cation is eight-coordinated by surrounding S atoms in a LaS8 bicapped trigonal prism geometry, with the La–S distances varying from 2.924(3) to 3.089(4) Å. Both Ag and Si are tetrahedrally coordinated by S atoms with Ag–S and Si–S distances in the range of 2.495(5)–2.727(4) and 2.117(5)–2.147(7) Å, respectively (Table S3†). When Ba2+ was substituted by La3+ with higher positive charge, one half of Ag+ in the anionic framework was eliminated. This led to a change in the connection mode between neighboring tetrahedral groups to sharing both the edge and corner, producing two-dimensional [AgSiS4]3− layers. These anionic [AgSiS4]3− layers are packed in an ABAB… pattern along the a-axis and separated by the La3+ cations (Fig. 1d).
Cation substitution-induced tetrahedral framework transformation
The crystal structure evolution of three compounds reveals that stepwise cationic substitution from a diamond-like structure is an effective way to extend new structures. To explore the underlying mechanisms, the minimal repeating unit of tetrahedral frameworks was analyzed. As shown in Fig. 1, from Ag2CdSiS4 to BaAg2SiS4 then to LaAgSiS4, accompanied with stepwise substitutions of higher valence cations, the component CdS4 and AgS4 tetrahedra in the minimal repeating unit of the tetrahedral frameworks are correspondingly reduced. This reduction of the component tetrahedra not only changes the connection mode of the tetrahedra but also the packing fashion to achieve the corresponding overall frameworks. On the other hand, the larger sizes of Ba2+ and La3+ cations also aid the formation of tunnels and layers in the tetrahedral frameworks, according to the theory of dimensional reduction.47 Therefore, the size effect of cations and the reduction of component tetrahedra caused by cation substitutions are the fundamental reasons why the tetrahedral frameworks of three Ag-based quaternary thiosilicates transform from a three-dimensional close-packed structure to a tunneling structure and finally to a two-dimensional layer. In fact, the above analysis also coincides Mei et al.'s review on the tetrahedron-based infrared nonlinear materials that the tetrahedral arrangement pattern will evolve with the ratio of metal to tetrahedra.41
Optical properties
As shown in the infrared spectrum, the powder samples of all the three compounds exhibit good light transmission in the 2.5–17 μm band, which indicate that the asymmetric Ag2CdSiS4, BaAg2SiS4 and LaAgSiS4 could be potential infrared nonlinear optical materials. The absorption band near 500 cm−1 is attributed to the Si–S bond vibration, and the splitting vibration modes may be caused by the SiS4 tetrahedral distortion (Fig. 2b and S4†).
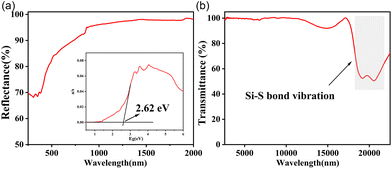 |
| Fig. 2 UV-vis-IR diffuse reflectance spectrum (a) and Fourier transform infrared spectrum (b) of LaAgSiS4. | |
According to the UV–vis–NIR diffuse reflectance spectrum (Fig. 2a and S5†), the experimental band gap values of Ag2CdSiS4, BaAg2SiS4 and LaAgSiS4 were measured to be 2.56, 2.32, and 2.62 eV, respectively, which are similar to that of AGS (2.72 eV) and match their yellow crystal colors. The unexpected smaller band gap value of BaAg2SiS4 agree with the reported one, which can be attributed to the outer 5d orbital of Ba2+.46 Combining the IR spectrum, the title compounds exhibit wide transmittance range from 0.5 to 17 μm.
Particle size-dependent SHG response measurements revealed that three asymmetric Ag2CdSiS4, BaAg2SiS4 and LaAgSiS4 crystals exhibit phase-matchable SHG response intensity of 0.6, 1.4 and 3.2 times that of AGS under 2.05 μm laser irradiation (Fig. 3a and b). By comparing the SHG effect of new and reported thiosilicates, it can be found that LaAgSiS4 achieves the largest SHG effect among known thiosilicates (Fig. 3c).48–57 Moreover, the SHG effect of title compounds are also comparable to similar thiogeranates and thiogstannates, such as Li2CdGeS4 (1 × AGSe), BaAg2GeS4 (1.7 × AGS), BaAg2SnS4 (0.4 × AGS), and BaCdSnS4 (0.7 × AGS).58–65
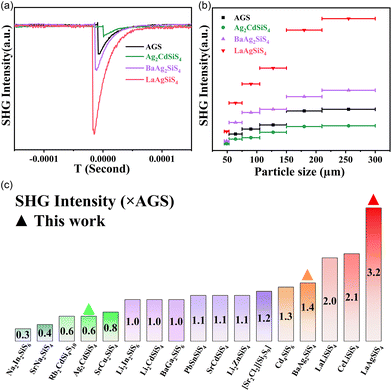 |
| Fig. 3 Particle size-dependent SHG intensity curve for Ag2CdSiS4, BaAg2SiS4 and LaAgSiS4 (a and b); SHG intensity near 2 μm for the title and typical reported thiosilicates (c). | |
Interestingly, from Ag2CdSiS4 to LaAgSiS4, their SHG responses increase with the reducing dimensionality of the tetrahedral frameworks. In order to understand the origin of the large SHG difference between polar structural Ag2CdSiS4 and LaAgSiS4, their local dipole moments of each fundamental group and unit cell were calculated based on the atomic coordinates. As listed in Table S4,† although the local dipole moments of SiS4 and AgS4 tetrahedral groups in both compounds are very close, the net dipole moments of per unit cell in LaAgSiS4 (54.060 D) are much larger than that of Ag2CdSiS4 (18.028 D). This indicates that after the introduction of cations, the crystal structures achieve a more ordered arrangement of active groups in LaAgSiS4, which is conducive to the superposition of strong microscopic SHG responses.
The LIDT tests were performed on the single crystals in the 150–210 μm particle size range with AGS as the reference. The results show that the LIDTs of Ag2CdSiS4, BaAg2SiS4 and LaAgSiS4 are about twice of that of AGS (Table S5†), which may be attributed to the stronger Si–S covalent bonds compared with Ga–S bonds and the introduction of strong Ba–S or La–S ionic bonds. In conclusion, Ag2CdSiS4, BaAg2SiS4 and LaAgSiS4 achieve excellent IR NLO properties including large phase matchable SHG effect, wide IR transparent range and high LIDT.
Theoretical calculations
To further understand the relationship between the structures and properties of the title compounds, first-principles calculations based on DFT methods were performed.66,67 The theoretical band gap values of Ag2CdSiS4, BaAg2SiS4, and LaAgSiS4 were calculated to be 1.13, 0.90, 1.57, respectively, which are much smaller compared to the experimental values due to the limitations of the GGA method (Fig. 4a and S6†).
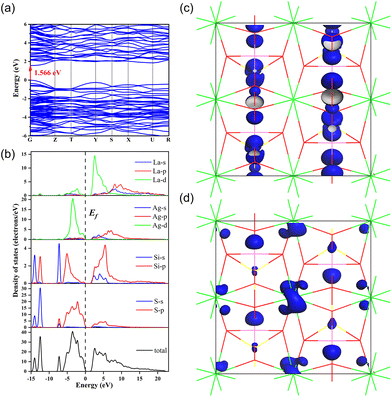 |
| Fig. 4 Calculated band structures (a) and partial density of states (b) and SHG density plots [VB (c) and CB (d)] for LaAgSiS4. | |
The partial density of states (DOS) analysis revealed the band gap contributions of title compounds (Fig. 4b and S7†). For all the Ag2CdSiS4, BaAg2SiS4 and LaAgSiS4 compounds, the Ag 4d and S 3p orbitals dominated the topmost valence bands (VB). The bottom of the conduction bands (CB), however, are not only contributed by the empty Ag-5s, 5p and unoccupied S-3p orbitals but also dominated by the outer 5d empty orbitals of the heavy cations. Overall, the band gaps of the three compounds are mainly determined by the AgS4 group, which explains why they exhibit similar direct band gap values.
The theoretical birefringence values (Δn) of Ag2CdSiS4, BaAg2SiS4 and LaAgSiS4 were calculated to be 0.070, 0.169 and 0.080, respectively (Fig. S8†). The suitable Δn of three asymmetric compounds coincide their phase-matching abilities. Given that all three crystal structural frameworks are composed of AgS4 and SiS4 tetrahedra, the apparent difference in Δn can be attributed to different tetrahedral arrangements. Remarkably, the large theoretical birefringence value (Δn) of BaAg2SiS4 breaks the bias that highly symmetric tetrahedral groups cannot generate large optical anisotropy. Proper crystal structure design is also essential to obtain large optical anisotropy.
The effective NLO coefficients (deff) of Ag2CdSiS4, BaAg2SiS4 and LaAgSiS4 were calculated to be 7.05, 6.43 and 13.16 pm V−1 under the restriction of space group and Kleinman's symmetry, respectively, which agree with the experimental results that LaAgSiS4 achieves a much larger SHG effect. It is worth mentioning that small deviations between experimental and theoretical values may be due to the fact that the experimental samples are powders but the theoretical samples are simulated grown single crystals. The SHG-weighed electron density (SHG density) plots intuitively display the distribution of the source of SHG effects in their crystal structure. As shown in Fig. 4c, d and S9,† in the valence band, the SHG effects originate from the Ag 4d electronic states and S 3p non-bonding states for all three compounds, while in the conduction band, the SHG process is contributed by the unoccupied Ag 5s, Si 3p, and S 3p electronic states for Ag2CdSiS4 and BaAg2SiS4 and by the unoccupied La 5d, Ag 5s, and Si 3p electronic states for LaAgSiS4. The different origins of the SHG effect also explain why they exhibit a large difference in the SHG performance. Furthermore, the contribution percentages of each structure building group were obtained by integrating the SHG density over VB and CB, which turns out to be 34.33%, 44.72% and 20.95% for SiS4, AgS4 and CdS4 groups in Ag2CdSiS4; 46.04%, 44.96% and 9.00% for SiS4, AgS4 and Ba2+ in BaAg2SiS4; 32.88%, 26.25% and 40.87% for SiS4, AgS4 and LaS8 groups in LaAgSiS4, respectively. For Ag2CdSiS4 and BaAg2SiS4, the tetrahedral group dominates the SHG contribution, while for LaAgSiS4, the LaS8 group contributes more, which emphasizes the benefits of adopting multi-step cation substitution to introduce well-performing functional crystal material genes.
Conclusions
In summary, three quaternary thiosilicates, namely, Ag2CdSiS4, BaAg2SiS4 and LaAgSiS4, were designed and synthesized successfully through multi-step cation substitutions from AGS. Interestingly, their tetrahedral frameworks transform from a three-dimensional close-packed structure to a tunneling structure and finally to a two-dimensional layered structure, which was essentially caused by the size effect of cations and the cation substitution-induced component tetrahedra reduction. In addition, as IR NLO materials, Ag2CdSiS4, BaAg2SiS4 and LaAgSiS4 crystals exhibit wide transmittance range (0.5–17 μm), relatively high laser-induced damage thresholds (2 times that of AGS), phase-matching ablility, and significant SHG response enhancement (0.6, 1.4 and 3.2 times of AGS, respectively), which results from the more ordered arrangement of active groups induced by cationic substitution. Theoretical calculations suggest that the large SHG effects of LaAgSiS4 originated from not only SiS4 and AgS4 tetrahedral groups but also from the active LaS8 cationic genes introduced through multi-step cation substitution. This work demonstrates the multi-step substitution-induced crystal structure transformation and nonlinear optical performance enhancement, indicating that multi-step cation substitution is an effective method to explore potential functional IR NLO crystal materials.
Data availability
Supporting data for this article is presented in the ESI.† The raw data of this article can be obtained by contacting the corresponding author.
Conflicts of interest
There are no conflicts to declare.
Acknowledgements
The authors thank Dr Bing-Xuan Li (Fujian Institute of Research on Structure of Matter, Chinese Academy of Sciences) for his help with the LIDT tests. This work was supported by the National Natural Science Foundation of China (No. 22031009, 22375201 and 21921001).
References
- Y. Tang, K. Li, X. Zhang, J. Deng, G. Li and E. Brasselet, Harmonic spin-orbit angular momentum cascade in nonlinear optical crystals, Nat. Photonics, 2020, 14, 658–662 CrossRef CAS.
- T. Verbiest, S. V. Elshocht, M. Kauranen, L. Hellemans, J. Snauwaert, C. Nuckolls, T. J. Katz and A. Persoons, Strong enhancement of nonlinear optical properties through supramolecular chirality, Science, 1998, 282, 913–915 CrossRef CAS PubMed.
- F. Capasso, R. Paiella, R. Martini, R. Colombelli, C. Gmachl, T. L. Myers, M. S. Taubman, R. M. Williams, C. G. Bethea, K. Unterrainer, H. Y. Hwang, D. L. Sivco, A. Y. Cho, A. M. Sergent, H. C. Liu and E. A. Whittaker, Quantum cascade lasers: ultrahigh-speed operation, optical wireless communication, narrow linewidth, and far-infrared emission, IEEE J. Quantum Electron., 2002, 38, 511–532 CrossRef CAS.
- D. F. Eaton, Nonlinear optical materials, Science, 1991, 253, 281–287 CrossRef CAS PubMed.
- S. Guo, Y. Chi and G. Guo, Recent achievements on middle and far-infrared second-order nonlinear optical materials, Coord. Chem. Rev., 2017, 335, 44–57 CrossRef CAS.
- G. C. Catella, L. R. Shiozawa, J. R. Hietanen, R. C. Eckardt, R. K. Route, R. S. Feigelson, D. G. Cooper and C. L. Marquardt, Mid-IR absorption in aggase2 optical parametric oscillator crystals, Appl. Opt., 1993, 32, 3948–3951 CrossRef CAS PubMed.
- A. Harasaki and K. Kato, New Data on the nonlinear optical constant, phase-matching, and optical damage of AgGaS2, Jpn. J. Appl. Phys., 1997, 36, 700–703 CrossRef CAS.
- P. A. Budni, L. A. Pomeranz, M. L. Lemons, C. A. Miller, J. R. Mosto and E. P. Chicklis, Efficient mid-infrared laser using 1.9 μm-pumped Ho:YAG and ZnGeP2 optical parametric oscillators, J. Opt. Soc. Am. B, 2000, 17, 723–728 CrossRef CAS.
- K. Wu and S. Pan, A review on structure-performance relationship toward the optimal design of infrared nonlinear optical materials with balanced performances, Coord. Chem. Rev., 2018, 377, 191–208 CrossRef CAS.
- F. Liang, L. Kang, Z. Lin and Y. Wu, Mid-infrared nonlinear optical materials based on metal chalcogenides: structure–property relationship, Cryst. Growth Des., 2017, 17, 2254–2289 CrossRef CAS.
- F. Hou, D. Mei, M. Xia and Y. Wu, Structure-performance relationship in tri-coordinated nonlinear optical materials toward optimal second harmonic generation and phase matching, Coord. Chem. Rev., 2021, 444, 214038 CrossRef CAS.
- Y. Li, J. Luo and S. Zhao, Local polarity-induced assembly of second-order nonlinear optical materials, Acc. Chem. Res., 2022, 55, 3460–3469 CrossRef CAS PubMed.
- J. H. Feng, C. L. Hu, X. Xu, B. X. Li, M. J. Zhang and J. G. Mao, AgGa2PS6: A New Mid–Infrared Nonlinear Optical Material with a High Laser Damage Threshold and a Large Second Harmonic Generation Response, Chem. – Eur. J., 2017, 23, 10978–10982 CrossRef CAS PubMed.
- W. Xing, F. Liang, C. Tang, E. Uykur, Z. Lin, J. Yao, W. Yin and B. Kang, Highly Distorted [HgS4] Motif-Driven Structural Symmetry Degradation and Strengthened Second-Harmonic Generation Response in the Defect Diamond-Like Chalcogenide Hg3P2S8, ACS Appl. Mater. Interfaces, 2021, 13, 37331–37338 CrossRef CAS PubMed.
- A. Abudurusuli, J. Huang, P. Wang, Z. Yang, S. Pan and J. Li, Li4MgGe2S7: The First Alkali and Alkaline-Earth Diamond-Like Infrared Nonlinear Optical Material with Exceptional Large Band Gap, Angew. Chem., Int. Ed., 2021, 60, 24131–24136 CrossRef CAS PubMed.
- B. Tell and H. M. Kasper, Optical and Electrical Properties
of AgGaS2 and AgGaSe2, Phys. Rev. B, 1971, 4, 4455–4459 CrossRef.
- T. K. Bera, J. H. Song, A. J. Freeman, J. I. Jang, J. B. Ketterson and M. G. Kanatzidis, Soluble direct-band-gap semiconductors LiAsS2 and NaAsS2: large electronic structure effects from weak AS...S interactions and strong nonlinear optical response, Angew. Chem., Int. Ed., 2008, 47, 7828–7832 CrossRef CAS PubMed.
- M. C. Chen, L. M. Wu, H. Lin, L. J. Zhou and L. Chen, sconnection enhances the second harmonic generation response: synthesis and characterization of Ba23Ga8Sb2S38, J. Am. Chem. Soc., 2012, 134, 6058–6060 CrossRef CAS PubMed.
- Y. X. Han, C. L. Hu, Z. Fang, Q. Q. Chen, B. X. Li, Y. Lin and J. G. Mao, a promising mid-infrared nonlinear optical material, J. Mater. Chem. C, 2022, 10, 12556–12559 RSC.
- C. Li, W. Yin, P. Gong, X. Li, M. Zhou, A. Mar, Z. Lin, J. Yao, Y. Wu and C. Chen, Trigonal planar [HgSe3]4− unit: a new kind of basic functionalgroup in ir nonlinear optical materials with large susceptibility and physicochemical stability, J. Am. Chem. Soc., 2016, 138, 6135–6138 CrossRef CAS PubMed.
- B. W. Liu, X. M. Jiang, H. Y. Zeng and G. C. Guo, Li[LiCs2Cl][Ga3S6]: Unprecedented Tetrahedra-built Nanoporous Framework with Excellent Nonlinear Optical Performance, J. Am. Chem. Soc., 2020, 142, 10641–10645 CrossRef CAS PubMed.
- Y. Zhang, H. Wu, Z. Hu, J. Wang, Y. Wu and H. Yu, Achieving a strong second harmonic generation response and a wide band gap in a Hg-based material, Inorg. Chem. Front., 2022, 9, 4075–4080 RSC.
- H. Chen, W. B. Wei, H. Lin and X. T. Wu, Transition-metal-based chalcogenides: A rich source of infrared nonlinear optical materials, Coord. Chem. Rev., 2021, 448, 214154 CrossRef CAS.
- X. Lin, G. Zhang and N. Ye, Growth and Characterization of BaGa4S7: A New Crystal for Mid-IR Nonlinear Optics, Cryst. Growth Des., 2009, 9, 1186–1189 CrossRef CAS.
- J. Yao, D. Mei, L. Bai, Z. Lin, W. Yin, P. Fu and Y. Wu, BaGa4Se7: A New Congruent-Melting IR Nonlinear Optical Material, Inorg. Chem., 2010, 49, 9212–9216 CrossRef CAS PubMed.
- J. Chen, C. Lin, S. Yang, X. Jiang, S. Shi, Y. Sun, B. Li, S. Fang and N. Ye, Cd4SiQ6 (Q = S, Se): Ternary Infrared Nonlinear Optical Materials with Mixed Functional Building Motifs, Cryst. Growth Des., 2020, 20, 2489–2496 CrossRef CAS.
- S. Lee, S. L. Carnahan, G. Akopov, P. Yox, L. L. Wang, A. J. Rossini, K. Wu and K. Kovnir, Noncentrosymmetric Tetrel Pnictides RuSi4P4 and IrSi3P3: Nonlinear Optical Materials with Outstanding Laser Damage Threshold, Adv. Funct. Mater., 2021, 31, 2010293 CrossRef CAS.
- H. Zhang, M. Zhang, S. Pan, X. Dong, Z. Yang, X. Hou, Z. Wang, K. B. Chang and K. R. Poeppelmeier, Pb17O8Cl18: A Promising IR Nonlinear Optical Material with Large Laser Damage Threshold Synthesized in an Open System, J. Am. Chem. Soc., 2015, 137, 8360–8363 CrossRef CAS PubMed.
- X. Chen, H. Jo and K. M. Ok, Lead Mixed Oxyhalides Satisfying All Fundamental Requirements for High-Performance Mid-Infrared Nonlinear Optical Materials, Angew. Chem., Int. Ed., 2020, 59, 7514–7520 CrossRef CAS PubMed.
- S. Yang, C. Lin, H. Fan, K. Chen, G. Zhang, N. Ye and M. Luo, Polar Phosphorus Chalcogenide Cage Molecules: Enhancement of Nonlinear Optical Properties in Adducts, Angew. Chem., Int. Ed., 2023, 62, e202218272 CrossRef CAS PubMed.
- J. Chen, C. Lin, D. Zhao, M. Luo, G. Peng, B. Li, S. Yang, Y. Sun and N. Ye, Anionic Aliovalent Substitution from Structure Models of ZnS: Novel Defect Diamond-like Halopnictide Infrared Nonlinear Optical Materials with Wide Band Gaps and Large SHG Effects, Angew. Chem., Int. Ed., 2020, 59, 23549–23553 CrossRef CAS PubMed.
- W. Zhou, J. Wu, W. Liu and S.-P. Guo, Ag-based chalcogenides and derivatives as promising infrared nonlinear optical materials, Coord. Chem. Rev., 2023, 477, 214950 CrossRef CAS.
- L. Kang, M. Zhou, J. Yao, Z. Lin, Y. Wu and C. Chen, Metal Thiophosphates with Good Mid-infrared Nonlinear Optical Performances: A First-Principles Prediction and Analysis, J. Am. Chem. Soc., 2015, 137, 13049–13059 CrossRef CAS PubMed.
- Z. Yang and S. Pan, Computationally assisted multistage design and prediction driving the discovery of deep-ultraviolet nonlinear optical materials, Mater. Chem. Front., 2021, 5, 3507–3523 RSC.
- C. L. Hu, Y. X. Han, Z. Fang and J. G. Mao, Zn2BS3Br: An Infrared Nonlinear Optical Material with Significant Dual-Property Enhancements Designed through a Template Grafting Strategy, Chem. Mater., 2023, 35, 2647–2654 CrossRef CAS.
- W. Cai, A. Abudurusuli, C. Xie, E. Tikhonov, J. Li, S. Pan and Z. Yang, Toward the Rational Design of Mid-Infrared Nonlinear Optical Materials with Targeted Properties via a Multi-Level Data-Driven Approach, Adv. Funct. Mater., 2022, 32, 2200231 CrossRef CAS.
- M. L. Liang, C. L. Hu, F. Kong and J. G. Mao, BiFSeO3: An Excellent SHG Material Designed by Aliovalent Substitution, J. Am. Chem. Soc., 2016, 138, 9433–9436 CrossRef CAS PubMed.
- H. Lin, W.-B. Wei, H. Chen, X. T. Wu and Q. L. Zhu, Rational design of infrared nonlinear optical chalcogenides by chemical substitution, Coord. Chem. Rev., 2020, 406, 213150 CrossRef CAS.
- J. Chen, C. L. Hu, F. Kong and J. G. Mao, High-Performance Second-Harmonic-Generation (SHG) Materials: New Developments and New Strategies, Acc. Chem. Res., 2021, 54, 2775–2783 CrossRef CAS PubMed.
- Q. Q. Liu, X. Liu, L. M. Wu and L. Chen, SrZnGeS4 : A Dual-Waveband Nonlinear Optical Material with a Transparency Spanning UV/Vis and Far-IR Spectral Regions, Angew. Chem., Int. Ed., 2022, 61, e202205587 CrossRef CAS PubMed.
- W. Wang, D. Mei, F. Liang, J. Zhao, Y. Wu and Z. Lin, Inherent laws between tetrahedral arrangement pattern and optical performance in tetrahedron-based mid-infrared nonlinear optical materials, Coord. Chem. Rev., 2020, 421, 213444 CrossRef CAS.
- J. Chen, H. Chen, F. Xu, L. Cao, X. Jiang, S. Yang, Y. Sun, X. Zhao, C. Lin and N. Ye, Mg2In3Si2P7: A Quaternary Diamond-like Phosphide Infrared Nonlinear Optical Material Derived from ZnGeP2, J. Am. Chem. Soc., 2021, 143, 10309–10316 CrossRef CAS PubMed.
- B. W. Liu, X. M. Jiang, B. X. Li, H. Y. Zeng and G. C. Guo, Li[LiCs2Cl][Ga3S6]: A Nanoporous Framework of GaS4 Tetrahedra with Excellent Nonlinear Optical Performance, Angew. Chem., Int. Ed., 2020, 59, 4856–4859 CrossRef CAS PubMed.
- F. Liang, L. Kang, Z. Lin, Y. Wu and C. Chen, Mid-Infrared Nonlinear Optical Materials Based on Metal Chalcogenides: Structure−Property Relationship, Coord. Chem. Rev., 2017, 333, 57–70 CrossRef CAS.
- O. V. Parasyuk and L. V. Piskach, Phase Equilibria in the Ag2SiS3-CdS System, Russ. J. Inorg. Chem., 1999, 44, 970–971 Search PubMed.
- J.-P. Sun, G. C. McKeown Wessler, T. Wang, T. Zhu, V. Blum and D. B. Mitzi, Structural Tolerance Factor Approach to Defect-Resistant I2-II-IV-X4 Semiconductor Design, Chem. Mater., 2020, 32, 1636–1649 CrossRef CAS.
- E. G. Tulsky and J. R. Long, Dimensional Reduction: A Practical Formalism for Manipulating Solid Structures, Chem. Mater., 2001, 13, 1149–1166 CrossRef CAS.
- Y. Yang, K. Wu, X. Wu, B. Zhang and L. Gao, A new family of quaternary thiosilicates SrA2SiS4 (A = Li, Na, Cu) as promising infrared nonlinear optical crystals, J. Mater. Chem. C, 2020, 8, 1762–1767 RSC.
- J. Zhou, Z. Fan, K. Zhang, Z. Yang, S. Pan and J. Li, Rb2CdSi4S10: novel [Si4S10] T2-supertetrahedra-contained infrared nonlinear optical material with large band gap, Mater. Horiz., 2023, 10, 619–624 RSC.
- W. Yin, K. Feng, W. Hao, J. Yao and Y. Wu, Synthesis, Structure, and Properties of Li2In2MQ6 (M = Si, Ge. Q = S, Se): A New Series of IR Nonlinear Optical Materials, Inorg. Chem., 2012, 51, 5839–5843 CrossRef CAS PubMed.
- G. M. Li, Y. Chu, J. Li and Z. X. Zhou, Li2CdSiS4, a promising IR NLO material with a balanced Eg and SHG response originating from the effect of Cd with d10 configuration, Dalton Trans., 2020, 49, 1975–1980 RSC.
- G. Li, Y. Chu and Z. Zhou, From AgGaS2 to Li2ZnSiS4: Realizing Impressive High Laser Damage Threshold Together with Large Second-Harmonic Generation Response, Chem. Mater., 2018, 30, 602–606 CrossRef CAS.
- B. W. Liu, H. Y. Zeng, M. J. Zhang, Y. H. Fan, G. C. Guo, J. S. Huang and Z. C. Dong, Syntheses, Structures, and Nonlinear-Optical Properties of Metal Sulfides Ba2Ga8MS16 (M = Si, Ge), Inorg. Chem., 2015, 54, 976–981 CrossRef CAS PubMed.
- J. Zhou, L. Luo, Y. Chu, P. Wang, Z. Guo, X. Su and J. Li, Partial congener substitution induced centrosymmetric to noncentrosymmetric structural transformation and nonlinear optical properties of PbSnSiS4, J. Alloys Compd., 2022, 899, 163366 CrossRef CAS.
- H. D. Yang, M. Y. Ran, S. H. Zhou, X. T. Wu, H. Lin and Q. L. Zhu, Rational design via dual-site aliovalent substitution leads to an outstanding IR nonlinear optical material with well-balanced comprehensive properties, Chem. Sci., 2022, 13, 10725–10733 RSC.
- C. Zhao, K. Wu, Y. Xiao, B. Zhang, H. Yu and H. Zhang, [Sr4Cl2][Si3S9]: Ultrawide-bandgap salt-inclusion thiosilicate nonlinear optical material with unprecedented tri-polymerized [Si3S9] clusters, J. Mater. Chem. C, 2023, 11, 4439–4443 RSC.
- Y. X. Han, C. L. Hu, B. X. Li and J. G. Mao, LnLiSiS4 (Ln = La and Ce): Promising infrared nonlinear optical materials designed by aliovalent substitution from SrCdSiS4, Mater. Today Phys., 2023, 31, 100987 CrossRef CAS.
- L. Nian, K. Wu, G. He, Z. Yang and S. Pan, Effect of Element Substitution on Structural Transformation and Optical Performances in I2BaMIVQ4 (I = Li, Na, Cu, and Ag. MIV = Si, Ge, and Sn. Q = S and Se), Inorg. Chem., 2018, 57, 3434–3442 CrossRef CAS PubMed.
- J. A. Brant, D. J. Clark, Y. S. Kim, J. I. Jang, J. Zhang and J. A. Aitken, Li2CdGeS4, A Diamond-Like Semiconductor with Strong Second-Order Optical Nonlinearity in the Infrared and Exceptional Laser Damage Threshold, Chem. Mater., 2014, 26, 3045–3048 CrossRef CAS.
- H. Chen, P. Liu, B. Li, H. Lin, L. Wu and X. Wu, Experimental and theoretical studies on the NLO properties of two quaternary non-centrosymmetric chalcogenides: BaAg2GeS4 and BaAg2SnS4, Dalton Trans., 2018, 47, 429–437 RSC.
- Y. Guo, F. Liang, W. Yin, Z. Li, X. Luo, Z. Lin, J. Yao, A. Mar and Y. Wu, BaHgGeSe4 and SrHgGeSe4: Two New Hg-Based Infrared Nonlinear Optical Materials, Chem. Mater., 2019, 31, 3034–3040 CrossRef CAS.
- N. Zhen, K. Wu, Y. Wang, Q. Li, W. Gao, D. Hou, Z. Yang, H. Jiang, Y. Dong and S. Pan, BaCdSnS4 and Ba3CdSn2S8: syntheses, structures, and non-linear optical and photoluminescence properties, Dalton Trans., 2016, 45, 10681–10688 RSC.
- W. Zhou, Q. Zhang, W. Yao, H. Xue and S. Guo, Stepwise Li Substitution Induced Structure Evolution and Improved Nonlinear Optical Performance for Diamond-like Sulfides, Inorg. Chem., 2021, 60, 12536–12544 CrossRef CAS PubMed.
- W. Zhou, W. Liu and S. Guo, (Na0.74Ag1.26)BaSnS4: A New AgGaS2-Type Nonlinear Optical Sulfide with a Wide Band Gap and High Laser Induced Damage Threshold, Chem. – Eur. J., 2022, 28, e202202063 CrossRef CAS PubMed.
- M. Yan, Z. Sun, W. Yao, W. Zhou, W. Liu and S. Guo, A highly distorted HgS4 tetrahedron-induced moderate second-harmonic generation response of EuHgGeS4, Inorg. Chem. Front., 2020, 7, 2451–2458 RSC.
- V. Milman, B. Winkler, J. A. White, C. J. Pickard, M. C. Payne, E. V. Akhmatskaya and R. H. Nobes, Electronic structure, properties, and phase stability of inorganic crystals: A pseudopotential plane-wave study, J. Quantum Chem., 2000, 77, 895–910 CrossRef CAS.
- M. D. Segall, P. J. D. Lindan, M. J. Probert, C. J. Pickard, P. J. Hasnip, S. J. Clark and M. C. Payne, First-principles simulation: ideas, illustrations and the CASTEP code, J. Phys.: Condens. Matter, 2002, 14, 2717–2744 CrossRef CAS.
Footnote |
† Electronic supplementary information (ESI) available: Syntheses, methods, instrumentations, computational details, crystallographic data, dipole moments, LIDT test, powder XRD, EDS, IR and UV–vis–NIR spectra, and computational results of title compounds. CCDC 2343723, 2343733 and 2343740. For ESI and crystallographic data in CIF or other electronic format see DOI: https://doi.org/10.1039/d4qi01603c |
|
This journal is © the Partner Organisations 2024 |
Click here to see how this site uses Cookies. View our privacy policy here.