DOI:
10.1039/D0QO00609B
(Review Article)
Org. Chem. Front., 2020,
7, 2325-2348
Recent advances in metal catalyzed or mediated cyclization/functionalization of alkynes to construct isoxazoles
Received
19th May 2020
, Accepted 19th June 2020
First published on 22nd June 2020
Abstract
Isoxazoles and their derivatives represent privileged heteroaromatic motifs, which exhibit remarkable biological and pharmaceutical activities. Notably, metal catalyzed or mediated cyclization/functionalization of alkynes such as alkynone oxime ethers, acetylinic oximes, propargylic amino ethers, alkynyl nitrile oxides, and propargylic alcohols has recently attracted much attention from synthetic organic chemists because of their high efficiency in the assembly of structurally diverse isoxazole architectures under mild conditions with exceptional chemo-, stereo- and regioselectivities. In this review, a wide array of metal catalysts, such as palladium, copper, iron, gold, silver, and other metals, have been summarized and discussed in detail. Moreover, we also highlight some representative synthetic strategies along with reaction mechanisms involved during the reaction. And, the literature has been surveyed from 2010 until the beginning of 2020.
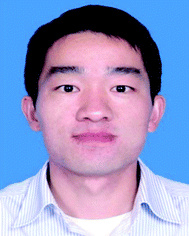 Jianxiao Li | Jianxiao Li received his B.Sc. degree (2008) from Fuyang Normal University. He earned his M.Sc. under the supervision of Prof. Zhao-Yang Wang at South China Normal University in 2011. He completed his Ph.D. in 2014 under the direction of Prof. Huanfeng Jiang at the South China University of Technology. From 2014 to 2017, he worked as a postdoctoral fellow with Prof. Huanfeng Jiang. He has now joined the School of Chemistry and Chemical Engineering at the South China University of Technology as an associate professor. His current research interests include transition metal-catalyzed coupling reactions and green chemistry. |
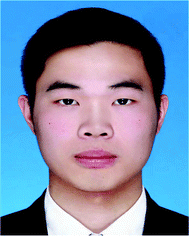 Zidong Lin | Zidong Lin was born (1996) in Guangdong Province, China. He obtained his B.Sc. degree from Jiangnan University in 2019. And then, he pursued his M.Sc. degree under the supervision of Associate Prof. Jianxiao Li at the School of Chemistry and Chemical Engineering, South China University of Technology. His research focuses on transition metal-catalyzed coupling reactions. |
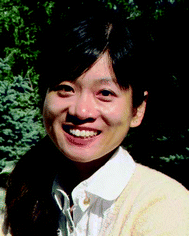 Wanqing Wu | Prof. Wanqing Wu received her PhD from Peking University with Professors Zhen Yang and Chi-Sing Lee in 2010. And then she joined Professor Huanfeng Jiang's group as a postdoctoral researcher at the South China University of Technology (SCUT). In 2014, she was promoted to Professor and her research interests include the development and applications of new synthetic methods. |
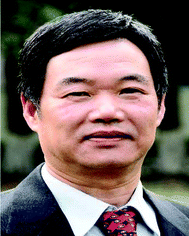 Huanfeng Jiang | Prof. Huanfeng Jiang received his PhD from the Shanghai Institute of Organic Chemistry (SIOC) with Professor Xiyan Lu in 1993. And then he joined the Guangzhou Institute of Chemistry as a research fellow. In 2003, he moved to the South China University of Technology (SCUT) as the Leading Professor of Chemistry. He received a Chinese Chemical Society-BASF Young Investigator's Award in 2002 and National Natural Science Funds for Distinguished Young Scholar in 2006. His research interests focus on synthetic methodologies and green and sustainable chemistry. |
1 Introduction
Among the vibrant classes of aromatic heterocyclic compounds, isoxazoles, discovered by Claisen in 1888, have always been considered as a privileged class of molecules in organic chemistry.1 As one of the important five-membered heteroaromatic ring scaffolds, isoxazoles exhibit significant biological and pharmaceutical activities in natural products, pharmaceutical agents and preclinical candidates, including anticancer, antimicrobial, antibiotic, and anti-inflammatory activities (Fig. 1).2 For example, valdecoxib has been established as a COX-2 inhibitor.3 Oxacillin is used as a β-lactam antibiotic.4 NVP-AUY922 is an experimental drug candidate developed by Vernalis for treating cancer.5 Moreover, the drug ring system analysis shows that the isoxazole ring ranks 33rd among the 351 ring systems found in marketed drugs.6 Besides, isoxazoles can serve as synthetically versatile intermediates for the highly efficient assembly of polyfunctionalized organic small molecules and functional materials.7 Therefore, the development of expeditious and practical synthetic methodologies for the rapid and direct assembly of structurally diverse polyfunctionalized isoxazoles is more appealing.
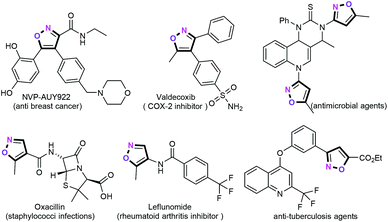 |
| Fig. 1 Representative examples of isoxazoles with pharmacological activities. | |
Owing to their unique chemical and biological characteristics, the development of efficient and practical synthetic methodologies for the construction of functionalized isoxazole building blocks has continued to capture the interest of synthetic organic chemists in the fields of heterocyclic chemistry and medicinal chemistry over the past few decades.8 Traditionally, the classical [3 + 2] cycloaddition reactions of alkynes with nitrile oxides displayed the direct and efficient synthetic strategies for the preparation of highly substituted isoxazole frameworks.9 However, most of these synthetic approaches required functionalized precursors, harsh conditions, and/or limited substrate scope. Undoubtedly, transition metal-catalyzed C–H functionalization of isoxazoles represents the most straightforward and rapid synthetic approaches for the construction of these structural motifs with high regioselectivities.10 Nevertheless, the substrate scope of these protocols is more limited. Alternatively, condensation reactions from β-dicarbonyls with hydroxylamine also represented one of the most important synthetic methods for the assembly of these functionalized heterocycles.11 Despite these significant advances, these developments suffer from relatively harsh reaction conditions and lower regioselectivities. In recent years, metal-catalyzed cycloisomerizations have emerged as fascinating and flourishing synthetic methodologies for the construction of various high value-added isoxazoles in a step- and atom-economical manner. There are two reviews on the synthesis of isoxazoles that have been published in recent years.12 Szostak and co-workers reviewed the recent developments in the synthesis and reactivity of isoxazoles from 2005 to 2014.13 Reactions of [3 + 2] cycloadditions, metal-free cycloisomerization reactions, condensation reactions, and functionalization of isoxazoles were discussed. However, the plausible reaction mechanisms were not addressed in detail. In 2018, Nakamura's group reviewed the synthetic approaches to functionalized isoxazoles, with particular focus on 1,3-dipolar cycloaddition, condensation, cycloisomerization, and direct functionalization.12b However, this review considers the limited aspects of the chemistry of isoxazoles and does not address the advances that have taken place in the past years. Nevertheless, the majority of such reviews reported thus far have been restricted to selected metals or selected strategies. To the best of our knowledge, there are no reports wherein metal-catalyzed cyclization/functionalization of alkynes was reviewed in particular along with recent achievements (mainly from 2018 to 2020) for the construction of highly functionalized isoxazole heteroaromatic motifs. Combining this with our interest in palladium-catalyzed chemical transformations of alkynes,14 we summarized the application of the ionic liquids as the green solvent for palladium-catalyzed coupling reactions of alkynes and/or alkenes for the preparation of diverse heterocyclic frameworks in 2016.15 Recently, in 2019, we also elucidated the palladium-catalyzed cascade cyclization/alkynylation reactions for the synthesis of structurally diverse monocyclic, bicyclic, fused polycyclic, and spirocyclic skeletons with excellent chemoselectivities.16 In this review, we will present a comprehensive summary of the most recent advancements (from 2010 until the beginning of 2020) in this rapidly developing area of synthesis of more elaborated isoxazole derivatives by metal catalyzed or metal mediated cross-coupling from alkynes, including our recent contributions. It is worth noting that this review will cover some synthetic methodologies which have been recently reviewed elsewhere and these will not be discussed in detail. In addition, we have divided this review into seven sections according to the use of different metal catalysts such as palladium, copper, iron, gold, silver, and other metals.
2 Palladium catalysts
In recent years, palladium-catalyzed cross-coupling reactions have been proven to be the most powerful and flexible synthetic methodologies for the assembly of structurally diverse complicated molecules with maximum atom and step economy in both academic and industrial applications.17 In this research field, Chen and co-workers demonstrated a palladium-catalyzed cascade cyclization–alkenylation for the synthesis of trisubstituted isoxazoles from 2-alkyn-1-one O-methyl oximes (1) with olefins (2) (Scheme 1).18 The unexpected direct cyclization by-product, 3,5-disubstituted isoxazoles, can be effectively inhibited by the addition of n-Bu4NBr as the additive. Employing Pd(TFA)2 (10 mol%) as the catalyst, CuCl2 (2 equiv.) as the metal oxidant, n-Bu4NBr (1 equiv.) as the additive, and Li2CO3 (2 equiv.) as the base, both 2-alkyn-1-one O-methyl oximes and olefins were perfectly accommodated for this reaction, giving the corresponding trisubstituted isoxazoles in moderate to good yields. Moreover, under the optimized conditions, a wide variety of functional groups such as ether, nitrile, ketone, aldehyde, ester, and amide groups are compatible with the reaction conditions. However, the electron-rich alkene such as vinyl acetate was not amenable to this protocol. In addition, this protocol also provides a straightforward synthetic pathway for the preparation of polycyclic naphtho[1,2-c] isoxazole (4) through palladium-catalyzed cyclization–alkenylation-Heck cascade process.19
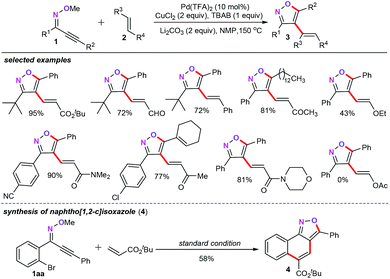 |
| Scheme 1 Palladium-catalyzed cascade cyclization/alkenylation for the synthesis of trisubstituted isoxazoles. | |
After this, the same group reported two regioselective synthetic routes towards 3,5-disubstituted isoxazoles (7) from ynones (5) (Scheme 2).20 This synthetic approach shows good complementariness with the method reported previously for the synthesis of trisubstituted isoxazoles.18 In their report, one pathway takes place by the subsequent dehydration condensation and palladium-catalyzed intramolecular cyclization process (path a). The other pathway involves a cyclocondensation and subsequent acid mediated dehydration process (path b). Moreover, the authors also emphasized that the palladium-catalyzed cyclization approach is more advantageous in the synthesis of sophisticated 3,5-disubstituted isoxazoles. More importantly, the newly obtained 3,5-disubstituted isoxazoles can be easily iodinated, which may show their further extension and potential application with the current protocol.
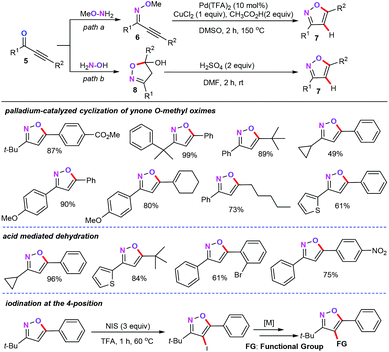 |
| Scheme 2 Complementary regioselective synthesis of 3,5-disubstituted isoxazoles from ynones. | |
Based on the experimental results and previous reports,21 the authors also proposed the plausible mechanism. As outlined in Scheme 3, the coordination of oxime ethers (6) with Pd(TFA)2 gives intermediate Int-1. Then, the Pd-catalyzed 5-endo-dig cyclization process forms the heteroaryl palladium intermediate Int-2. Subsequently, on nucleophilic attack by Cl−, intermediate Int-3 would be obtained through the loss of a methyl group. After this, depalladation–protonation of intermediate Int-3 affords the target products.
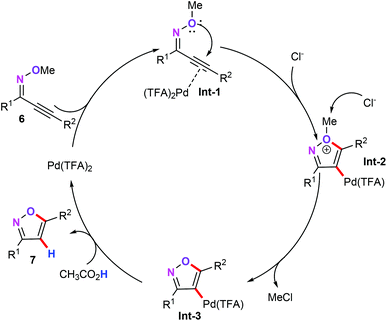 |
| Scheme 3 Plausible mechanism for palladium-catalyzed intramolecular cyclization. | |
Building on our recent developments in palladium-catalyzed coupling of alkynes22 and metal-catalyzed cross-coupling reaction for the synthesis of heterocycle frameworks,23 our group also successfully accomplished palladium-catalyzed cascade cyclization/alkynylation and alkenylation of alkynone O-methyloximes with terminal alkynes.24 Interestingly, acidic ionic liquid [C2O2mim]Cl (1-carboxymethyl-3-methylimidazolium chloride) as the additive played an important role in this chemical transformation. Other well-known halide additives such as LiCl, LiBr, nBu4NCl, and nBu4NI were totally ineffective for this approach. For the palladium-catalyzed cascade cyclization/alkynylation, we optimized the cascade cyclization/alkynylation reaction as follows: oximes (8, 0.2 mmol), alkynes (9, 1.2 equiv.), and Pd(TFA)2 (5 mol%) as the catalyst, 50 mg 4 Å MS as the water absorbent, [C2O2mim]Cl (2 equiv.) as the additive, and DMSO (2 mL) at 80 °C for 8 h. Both electron-donating (OMe, N(Me)2) and electron-withdrawing (CF3, CO2Me) substituents on the phenyl ring of alkynes were well accommodated, affording the desired alkynylation products (10) in moderate to good yields (Scheme 4). In addition, a wide range of diverse aliphatic alkynes were also compatible with the optimized reaction conditions, giving the corresponding products in moderate yields.
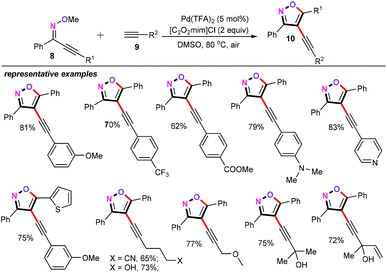 |
| Scheme 4 Palladium-catalyzed cascade cyclization/alkynylation of oximes with terminal alkynes. | |
It is particularly noteworthy that when using HOAc (1.5 mL) and DMF (0.5 mL) as the co-solvents at 100 °C for 12 h, the cascade cyclization/alkenylation of oximes (11) could also couple with terminal alkynes (12) to produce the 4-vinyl substituted isoxazole derivatives (13) in moderate to good yields with excellent stereoselectivity (Scheme 5).24 We further comprehensively investigated the substrate scope and limitations of both terminal alkynes and the oximes. As illustrated in Scheme 5, a library of aryl/heteroaryl alkynes (12) with various substituent groups such as alkyl, alkoxyl, halogen, trifluoromethyl, ester, and even aldehyde groups were all well tolerated under the current catalytic system. Unfortunately, aliphatic alkynes were not compatible with the optimized conditions. As for the substrate scope of oximes (11), various different functionalities such as alkyl, cycloalkyl, styrenyl, and heteroaryl were well suitable for this transformation. The gram-scale synthesis of this protocol also demonstrated the efficiency and practicability of this synthetic strategy.
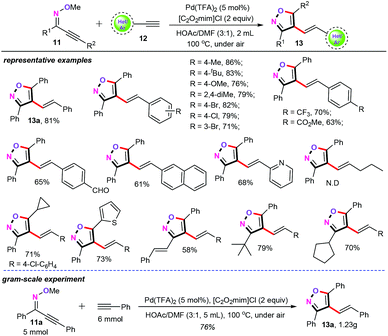 |
| Scheme 5 Palladium-catalyzed cascade cyclization/alkenylation of oximes with terminal alkynes. | |
The plausible mechanism for the palladium-catalyzed cascade cyclization/alkynylation and alkenylation reaction is described in Scheme 6. The cascade cyclization/alkynylation (left pathway) is initiated through trans-oxypalladation of oximes (8) to give an oxonium intermediate Int-4. Then, intermediate Int-4 undergoes sequential removal of methyl chloride and alkyne coordination in a weak acidic reaction medium, generating vinylpalladium species Int-5. Subsequently, a reductive elimination produces the desired products 10. The other pathway, vinylpalladium catalytic species Int-6, undergoes alkyne insertion giving vinylpalladium intermediate Int-7. Next, protonolysis of vinylpalladium species Int-7 with HOAc as the proton sources affords the corresponding alkenylation products 13.
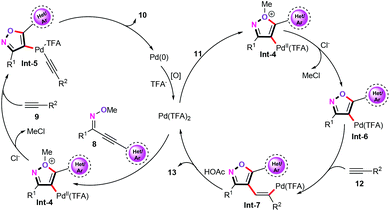 |
| Scheme 6 Plausible mechanism for palladium-catalyzed cyclization/alkynylation and alkenylation. | |
Based on the above-mentioned formation of 4-alkynylation and 4-alkenylation isoxazole derivatives, this cascade cyclization/functionalization strategy was extended to the synthesis of functionalized 4-alkynylisoxazoles. Satisfyingly, a highly regioselective palladium-catalyzed three-component cascade cyclization/alkynylation of alkynone O-methyloximes (14), propargyl p-toluenesulfonate (15), and secondary amines (16) was efficiently developed in ionic liquids (Scheme 7).25 A whole variety of conventional solvents such as DMSO, DMF, toluene, and DCE were observed; however, all of them were found to be less effective. Delightfully, the ionic liquid [Bmim]Cl (1-butyl-3-methylimidazolium chloride) was found to be the best solvent for this transformation. In the presence of 0.5 mol% of NHC-Pd (17) as the catalyst, [Bmim]Cl (2 mL) as the green solvent, ligands and additive free conditions, this protocol provided an efficient and eco-friendly way for the assembly of structurally diverse 4-alkynylisoxazoles (18) in moderate to good yields. Gratifyingly, a variety of functional groups such as alkyl, cycloalkyl, halogen, trifluoromethyl, ester, naphthyl, styryl, and trifluoromethylsulfanyl groups were nicely compatible with the current catalytic system. However, as for the secondary amines, diphenylamine and cyclohexylamine were not amenable to this approach, and failed to afford the desired products.
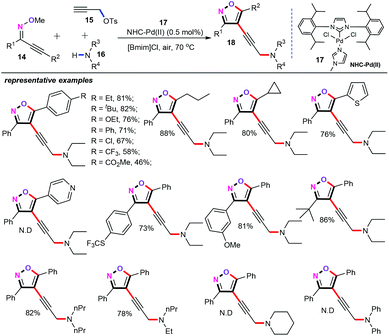 |
| Scheme 7 Palladium-catalyzed three-component cascade cyclization/alkynylation. | |
Mechanistic investigations showed that a Pd(0)/Pd(II) catalytic pathway is involved in this transformation. Therefore, we also proposed a plausible mechanism in Scheme 8. Initially, trans-oxypalladation of alkynone O-methyloximes (14) produces the vinyl palladium species Int-8. In the meantime, the alkynylamine intermediate Int-9 was generated in situ from propargyl p-toluenesulfonate (15) and secondary amines (16). Subsequently, alkyne intermediate Int-9 coordinates with the vinyl palladium species Int-8 and forms palladium intermediate Int-10.26 After this, a reductive elimination gives the target products 18. Finally, the palladium(0) is additionally oxidized by air to generate the palladium(II) active catalyst species to complete this catalytic cycle.
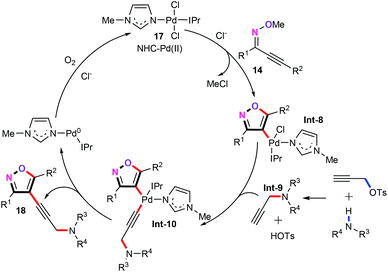 |
| Scheme 8 Plausible mechanism for palladium-catalyzed cascade cyclization/alkynylation. | |
Furthermore, by using a similar cascade cyclization/functionalization synthetic tactics, our group developed a palladium-catalyzed cascade annulation/allylation of alkynone O-methyloximes (19) with allyl halides (20) for the rapid construction of fully substituted isoxazole derivatives (21) (Scheme 9).27 Using 3 mol% of Pd(OAc)2 as the catalyst and 1 equiv. nBuN4Br as the additive in DMF at 80 °C for 5–40 min, both aromatic and aliphatic alkynone O-methyloximes (19) with different functional groups were smoothly transformed into the desired products in good to excellent yields with high regioselectivities. In addition, in most cases, the yields of the fully substituted isoxazoles ranged between 93% and 97%. However, disubstituted allyl bromide or trisubstituted allyl bromide such as 3-bromobut-1-ene and 3-bromo-3-methylbut-1-ene was not compatible with the current catalytic system. Specifically, long linear chain alkenes were perfectly tolerated under the optimized conditions, delivering the desired products in good yields. Remarkably, a 10 g scale synthesis was also achieved, which further demonstrated the potential capabilities to assemble high value-added products in synthetic and pharmaceutical chemistry.
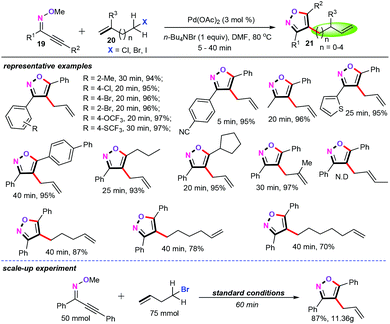 |
| Scheme 9 Palladium-catalyzed cascade annulation/allylation reaction. | |
A plausible reaction mechanism for the palladium-catalyzed cascade annulation/allylation reaction was then proposed. Mechanistic studies revealed that this protocol may involve a well-known “chain-walking” process, in which the alkylpalladium intermediates undergo rapid β-hydride elimination and reinsertion to change the position of the palladium on the alkyl chain, allowing the assembly of molecular complexity.28 As depicted in Scheme 10, heteroaryl palladium intermediate Int-11 is formed by sequential 5-endo-dig cyclization and nucleophilic attack with Br− as the nucleophile. And then, alkene migration insertion occurs to afford intermediate Int-12. Subsequently, intermediate Int-12 undergoes the rapid β-hydride elimination and reinsertion (namely “chain-walking”) to afford intermediate Int-13. Finally, β-X elimination produces the target products and gives Pd(II) active species.
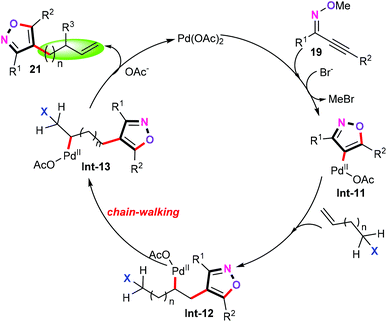 |
| Scheme 10 Plausible mechanism for palladium-catalyzed annulation/allylation. | |
During these studies, trans-oxypalladation of alkynone O-methyloximes gives the vinyl palladium species, which can be captured by a wide range of activated alkenes, such as acrylaldehyde, olefin ketone, acrylate, and styrene derivatives as well as allyl halides. However, some unactivated alkenes such as long-chain enols are still scarcely studied owing in part to the electron-rich properties of the alkenyl motifs.29 In 2019, in a further investigation of chain-walking synthetic strategies, our group discovered a palladium-catalyzed cascade 5-endo cyclization and alkylation from alkynone O-methyloximes (22) and long-chain enols (23) for the synthesis of functionalized β-isoxazole aldehydes/ketones (24) (Scheme 11).30 The key to this reaction is the choice of the oxidant and the solvent. Moreover, the substrate scope of methyloximes (22) and long-chain enols (23) have also been fully examined in the presence of the 5 mol% of Pd(OAc)2, 1 equiv. chloranil, 1 equiv. KI, and 1 equiv. TBAB under CH3CN conditions, thus furnishing the desired products 24 in good to excellent yields with high stereo- and regioselectivities. Additionally, this method displayed a wide tolerance for various functional groups on both the methyloximes and long-chain enols moieties. Mechanistically, enol migration insertion to heteroaryl palladium species produces the alkyl palladium(II) species Int-14, which undergoes rapid β-H elimination and reinsertion to change the position of the palladium on the alkyl chain giving alkyl palladium intermediate Int-15. Afterwards, successive additions and elimination of HPdX produce the corresponding alkylation products 24.
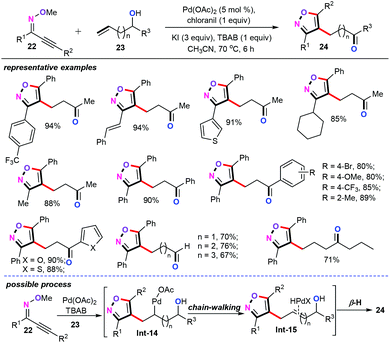 |
| Scheme 11 Palladium-catalyzed cascade cyclization and alkylation reaction. | |
In a subsequent study, 4-sulfenylisoxazole derivatives (27) were efficiently assembled through a palladium-catalyzed three component cascade annulation/sulfenylation using readily available Na2S2O3 as an odorless sulfenylation reagent (Scheme 12).31 In this transformation, one C–O bond and two C–S bonds were formed in the cascade process. This approach shows a broad substrate scope and good functional compatibility, including alkoxyl, halogen, trifluoromethyl, and trifluoromethylsulfanyl groups. However, aliphatic halides were not compatible with this cascade S-transfer reaction. Notably, this cascade observation is the first successful case of the direct sulfenylation of acetylinic oximes (25) using Na2S2O3 as an odorless sulfenylation reagent. More importantly, the reaction shows excellent chemoselectivity, excellent atom and step economy, and eco-friendliness, without any additive, base, and ligand, thus providing a promising application in synthetic and pharmaceutical chemistry.
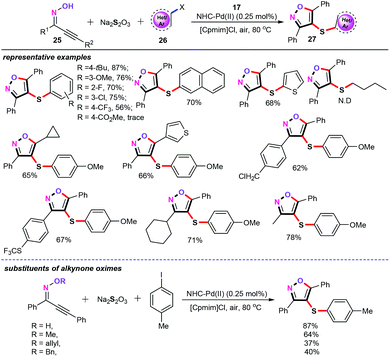 |
| Scheme 12 Palladium-catalyzed cascade annulation/sulfenylation reaction. | |
Control experiments demonstrated that neither diaryl disulfides nor aryl thiols were possible intermediates in this three-component cascade transformation. In addition, the free radical pathway was also absolutely ruled out in our catalytic system. As a result, a plausible mechanism is described in Scheme 13. Initially, trans-oxypalladation of acetylinic oximes gives the heteroaryl palladium intermediate Int-16. Synchronously, aryl halides reacted with Na2S2O3 affording thiosulfate complex Int-17,32 which undergoes ligand exchange with Int-16 furnishing palladium thiosulfate complex Int-18. Afterwards, the subsequent release of SO3 and reductive elimination generate the target products 27. And above all, the ionic liquid [Cpmim]Cl (1-cyanopropyl-3-methyl imidazolium chloride) plays a crucial role in this cascade sulfenylation reaction. It not only acts as a green reaction medium, but also provides excess Cl− to eliminate hydrochloride from acetylinic oximes 25, furnishing the heteroaryl palladium intermediate Int-16.
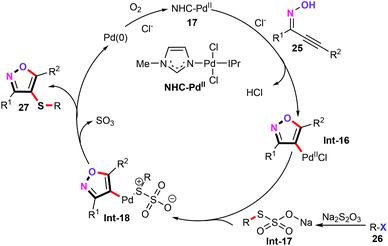 |
| Scheme 13 Proposed mechanism for the cascade annulation/sulfenylation reaction. | |
Interestingly, this aerobic oxidation cascade annulation strategy was successfully applied to more sophisticated catalytic systems. For instance, our group accomplished a palladium-catalyzed aerobic oxidation aminocarbonylation of O-methyloximes (28) and amines (29) with PEG-400 as an environmentally benign medium (Scheme 14).33 Particularly, this novel approach has opened up a new channel for the quick assembly of diverse synthetically challenging 4-carboxamide isoxazole derivatives (30) with high atom- and step-economy. It is noteworthy that Pd(Py)2Cl2 could efficiently catalyze this cascade aminocarbonylation in the absence of phosphine ligands or other additional additives. Among a variety of solvents, PEG-400 proved to be the suitable solvent for this chemical transformation. Under the optimized conditions, both alkynone O-methyloximes and a vast array of primary amines were well accommodated in the transformation, affording the corresponding 4-carboxamide isoxazoles in moderate to good yields. However, aromatic amine and secondary amine were not compatible with the current catalytic system. Notably, with 1 atm CO as the carbonyl source, O2 or air as the terminal oxidant, ionic liquid as the green additive, and PEG-400 as the green solvent make this cascade aminocarbonylation green and practical.
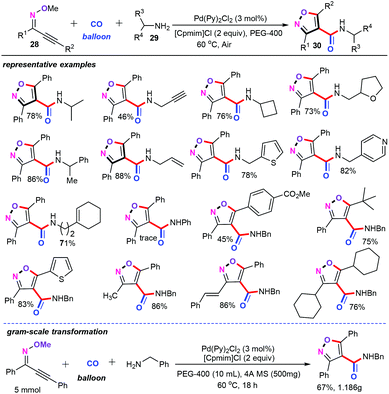 |
| Scheme 14 Palladium-catalyzed cascade annulation/aminocarbonylation reaction. | |
Mechanistic studies suggested that a Pd(0)/Pd(II) catalytic pathway is involved in this aminocarbonylation reaction. Based on the experimental results, we suggested a plausible mechanism as shown in Scheme 15. Similarly, migratory insertion of CO into the C–Pd bond of the vinylpalladium intermediate Int-19 gives the acylpalladium species Int-20. Subsequently, ligand exchange of acylpalladium species Int-20 with primary amines leads to carbamoylpalladium intermediate Int-21,34 which undergoes a reductive elimination process to produce the aminocarbonylation products 30.
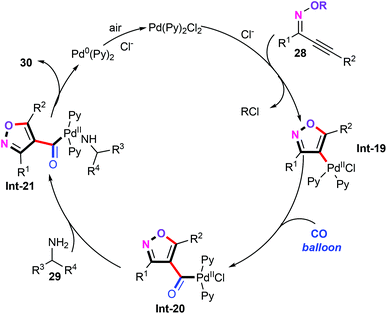 |
| Scheme 15 Proposed mechanism for the cascade annulation/aminocarbonylation reaction. | |
In recent years, the use of metal nanoparticle catalysts in cascade coupling reactions has rapidly increased. As emphasized in the literature, the dominant metal nanoparticle employed in this area is the palladium nanoparticle.35 For example, Vadde and Jonnalagadda and co-workers developed a one-pot Cu-free nano-Pd catalyzed sequential acyl Sonogashira coupling-intramolecular cyclization for the synthesis of various substituted isoxazoles (34) in an environmentally benign PEG-400/water system (Scheme 16).36 The Pd-NHC catalyst precursors can be synthesized from Pd(OAc)2 and NHC ligands (33) in the presence of DBU in THF. It is worth mentioning that an in situ generation of catalytic palladium nanoparticles from Pd-NHC afforded significantly better results than the direct use of commercially available palladium catalysts such as PdCl2, and Pd(OAc)2. Notably, recycling experiments showed that the obtained nano-Pd catalyst could be recycled up to five times without remarkable loss of catalytic activity. Thus, recoverability, environmental benignity, and high catalytic activity were some added advantages of this novel approach. As for the plausible reaction mechanism, the oxidative addition of the acyl chloride (31) to nano-Pd(0) gives a Pd(II) species Int-21. Then, in the presence of a pyrrolidine base, alkyne (32) coordination forms Pd–alkyne species.
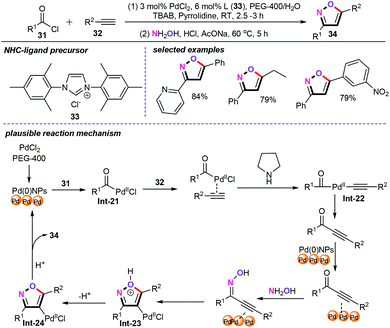 |
| Scheme 16 PdNP-catalyzed regioselective one-pot synthesis of isoxazoles in PEG-400. | |
Despite the undisputable advances that have been made in palladium-catalyzed cascade annulation/functionalization, the synthetic methodologies for the preparation of valuable 4-haloisoxazoles have remained relatively rare. In this study, Larock and co-workers established a metal-free electrophilic cyclization of alkynyl O-methyl oximes for the assembly of structurally diverse 4-bromo- and 4-iodoisoxazole derivatives with Br2 and ICl as the electrophiles.21 In 2016, Thongsornkleeb and co-workers disclosed a metal-free chlorinative cyclization of alkynyl O-methyl oximes (35) for the synthesis of 4-chloroisoxazoles in moderate to excellent yields (Scheme 17).37 With the combination of NCS (N-chlorosuccinimide)/TMSCl (chlorotrimethylsilane) as the coupling system, the procedure was compatible with both electron-deficient and electron-rich alkynyl O-methyl oximes as coupling partners in CH3NO2 at room temperature. From the mechanistic point of view, chlorine (Cl2) and hydrochloric acid (HCl) were generated in situ and were confirmed as the possible intermediates in this protocol. Notably, 4-bromo- and 4-iodoisoxazoles were also investigated using N-bromosuccinimide (NBS) and N-iodosuccinimide (NIS) as the halogenation reagents, respectively.
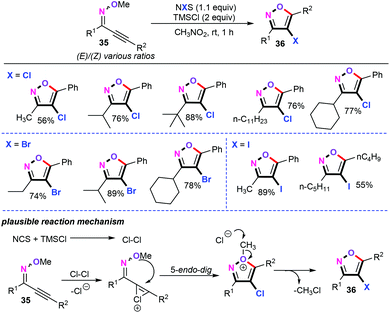 |
| Scheme 17 The direct synthesis of 4-haloisoxazoles by the NXS/TMSCl catalytic system. | |
Gratifyingly, our group also exploited a palladium-catalyzed synthetic strategy for the preparation of various valuable 4-bromoisoxazoles derivatives (38) from readily available O-methyl oximes (37) in basic ionic liquids (Scheme 18).38 It was found that Pd(TFA)2 (5 mol%) can efficiently catalyze this bromination reaction in the presence of CuBr2 (2 equiv.) as the brominated reagent, Na2S2O8 (2 equiv.) as the oxidant, and ionic liquid [Cpmim]Cl as the solvent at 100 °C in open air, furnishing a vast array of 4-bromoisoxazoles in good to excellent yields with high atom efficiency. Moreover, several different solvents were examined and the results showed that the use of various conventional solvents such as DMF, DMSO, toluene, 1,4-dioxane, and NMP significantly decreased the yields. Presumably, the Pd(II)/Pd(IV) catalytic cycle may be involved in this cascade cyclization/bromination process. Similar to the previous reports, the heteroaryl palladium(II) catalytic species Int-25 can be oxidized into the palladium(IV) active species Int-26 in the presence of 2 equiv. Na2S2O8 in this approach.
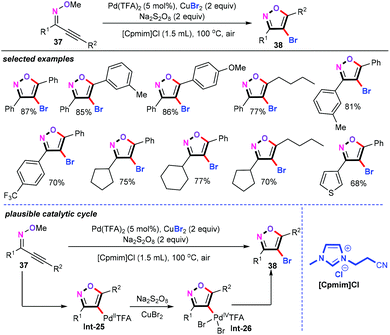 |
| Scheme 18 Palladium-catalyzed cascade cyclization/bromination. | |
In 2015, based on their program on sustainable C–H activation, the Takenaka and Sasai group developed a palladium-catalyzed direct C–H arylation of isoxazoles (39) for the preparation of a library of 5-arylisoxazoles (41) in good yields with high regioselectivity (Scheme 19).39 In the presence of 5 mol% of PdCl2(MeCN)2, 10 mol% of 1,2-bis(diphenylphosphino)benzene (DPPBz) and 2 equiv. of AgF in N,N-dimethylacetamide (DMA) at 100 °C for 24 h, both electron-donating (Me, MeO) and electron-withdrawing (CF3, COMe, CO2Me, CN) substituents on the phenyl ring of aryl and heteroaryl iodides were amenable for this transformation. Further screening of the reaction conditions revealed that both DPPBz as the ligand and AgF as the additive were indispensable for this chemical transformation. Moreover, this protocol also displayed a wide tolerance for various functional groups on the isoxazole moiety, such as alkyl, cycloalkyl, aryl, vinyl, and hydrogen atom, and good yields of the corresponding products were obtained. Additionally, the intermolecular competition KIE experiments (kH/kD = 3.4) indicated that the C–H activation was involved in the rate-limiting step.
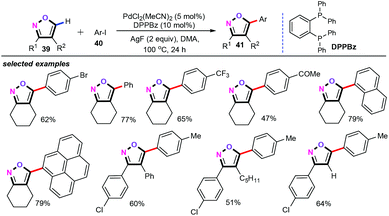 |
| Scheme 19 Palladium-catalyzed direct C–H arylation of isoxazoles. | |
In the proposed mechanism (Scheme 20), oxidative addition of the aryl iodides 40 with the Pd(0) complex forms aryl palladium intermediates Int-27. Then, anion exchange of the resulting Int-27 species with AgF affords the fluoride-palladium intermediates Int-28. Afterwards, C–H bond activation of isoxazoles 39 produces palladium complex Int-29. Subsequently, the reductive elimination delivers the target 5-arylisoxazole products. More importantly, the Pd–I intermediate Int-27 was also successfully synthesized and applied in this coupling reaction.
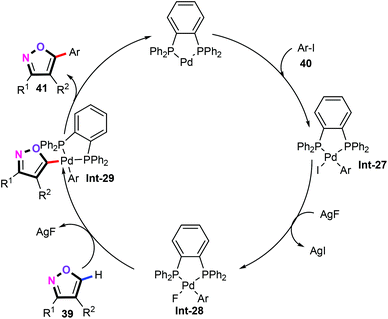 |
| Scheme 20 Plausible catalytic cycle for palladium-catalyzed direct C–H arylation of isoxazoles. | |
Recently, Jurberg and co-workers developed a palladium-catalyzed cross-coupling strategy for the synthesis of 3,5-disubstituted isoxazoles using 5-(pseudo)halogenated isoxazoles as the platform molecule (Scheme 21).40 With triflate substituted isoxazoles (42) as the optimal substrate, the well-known Sonogashira and Suzuki cross-coupling can be easily achieved, thus generating the corresponding alkynylation products (44) and arylation (46) products in moderate to good yields.
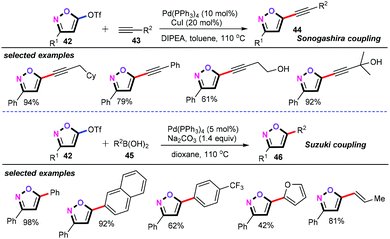 |
| Scheme 21 Palladium-catalyzed coupling of 5-(pseudo)halogenated isoxazoles. | |
3 Gold catalysts
In 2010, Miyata and co-workers reported a gold-catalyzed domino reaction of alkynyl oxime ethers (47) to access synthetically challenging trisubstituted isoxazoles (Scheme 22).41 In the presence of 5 mol% of AuCl3 as the catalyst and DCE as the solvent under reflux conditions for 2 h, oxime ethers (47) underwent a subsequent 5-endo cyclization and an allyl oxonium Claisen type [3.3]-σ rearrangement delivering the corresponding products 48 in moderate yields. In addition, a variety of functional groups such as furyl, trifluoromethyl, ester, allyl, hydroxyl, and silyloxymethyl (OTBS) were compatible with this protocol. Moreover, the newly developed reaction can be further applied for the straightforward synthesis of fused isoxazole heterocycles.
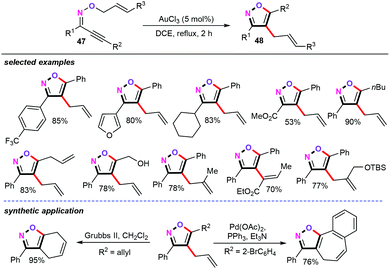 |
| Scheme 22 Gold-catalyzed cyclization/Claisen type rearrangement reaction. | |
A plausible mechanism of this domino reaction was then proposed, as shown in Scheme 23. Similar to the palladium-catalyzed reaction pathway, nucleophilic addition of alkynyl oxime ethers (47) gives the heteroaryl gold catalytic species Int-30. Subsequently, intermediate Int-30 undergoes the Claisen-type [3.3]-σ rearrangement process, affording gold intermediate Int-32. Finally, further aromatization of gold intermediate Int-32 generates 4-allylisoxazoles and liberates the catalytic gold species.
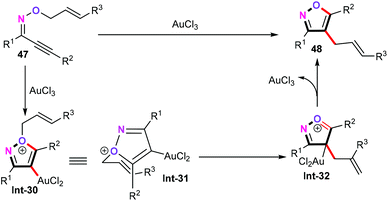 |
| Scheme 23 Plausible catalytic cycle for gold-catalyzed domino reaction. | |
In the same year, Perumal and co-workers developed an AuCl3-catalyzed cycloisomerization of α,β-acetylenic oximes (49) (Scheme 24).42 Using 1 mol% AuCl3 as the catalyst, with no ligands or additives, in CH2Cl2 at 30 °C for 30 min under a N2 atmosphere, a broad range of functional groups such as OMe, F, OH, CN, and SiMe3 were all well tolerated, and afforded the corresponding 3-substituted, 5-substituted or 3,5-disubstituted isoxazole products 50 in good to excellent yields. Control experiments revealed that no reaction was observed in the absence of gold catalysts. Moreover, other gold catalysts such as AuBr3, AuCl, and Me3PAuCl/AgBF4 were also screened, and negative results were observed.
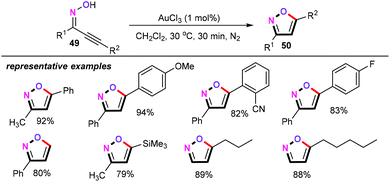 |
| Scheme 24 Gold-catalyzed cycloisomerization of α,β-acetylenic oximes. | |
Three years later, the Wong and Che group investigated the cyclometallated gold-catalyzed cycloisomerization of alkynyl oximes for the synthesis of isoxazoles (Scheme 25).43 Obviously, six-membered ring cyclometallated gold complexes (52e–52h) show a higher catalytic activity than five-membered ring cyclometallated gold complexes (52a–52d) for the formation of 3,5-diphenylisoxazole. In the presence of 1 mol% 52e as the catalyst and 2 mol% AgOTf as the additive in MeOH/CH2Cl2 (1
:
1) at room temperature for 2 h, the scope and limitations of the alkynyl oximes were then comprehensively investigated.
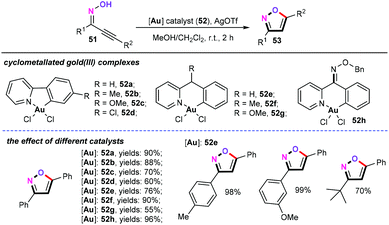 |
| Scheme 25 The cyclometallated gold-catalyzed cycloisomerization of alkynyl oxime. | |
In 2014, Ryu and co-workers developed a gold-catalyzed cascade cyclization–oxidative alkynylation and cyclization–fluorination of alkynyl O-methyl oximes (54) (Scheme 26).44 Further screening of the reaction conditions revealed that both (PPh3)AuNTf2 and K3PO4 were indispensable for this protocol. The authors also explained that the role of K3PO4 is to abstract the acetylenic proton of the terminal alkyne and produce a gold-acetylide species. Using a combination of 10 mol% of (PPh3)AuNTf2, 2 equiv. of K3PO4, 2.5 equiv. of Selectfluor as the catalytic system, the electron-withdrawing alkynes (55) proceed smoothly at room temperature, thus generating two coupling products in relatively lower yields. However, alkynes bearing electron-donating groups on the phenyl ring were not amenable for this cascade heterocyclization reaction. Unfortunately, this approach can only deliver two regioisomers.
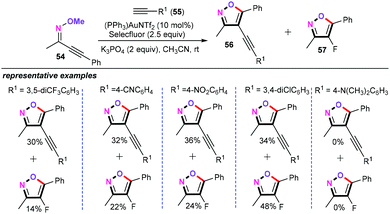 |
| Scheme 26 Gold-catalyzed cascade cyclization/alkynylation and cyclization/fluorination reaction. | |
Subsequently, the same group also reported gold-catalyzed cascade cyclization/fluorination of alkynyl O-methyl oximes (58) (Scheme 27).45 Thus, the authors found that the combination of 5 mol% of (IPr)AuCl, 5 mol% of AgOTs, 2.5 equiv. of Selectfluor, and 2 equiv. of NaHCO3 in CH3CN at room temperature proved to be optimal for the cyclization/fluorination process. Moreover, the optimization conditions revealed that gold(I) complexes bearing bulky biphenyl-based phosphine ligands were not effective for this transformation. As for the other alternative fluorinating reagents such as N-fluorobenzenesulfonimide (NFSI) or N-fluoropyridinium tetrafluoroborate (NFPY-BF4), they failed to deliver the desired products. It is rather remarkable that NaHCO3 as the base had a highly significant impact on the outcome of this reaction. Alkynyl O-methyl oximes bearing a wide range of functional groups, such as nitro, trifluoromethyl, methoxy and halides, reacted with Selectfluor smoothly to produce the 4-fluoroisoxazole products 59 in good to excellent yields (even 100% yields).
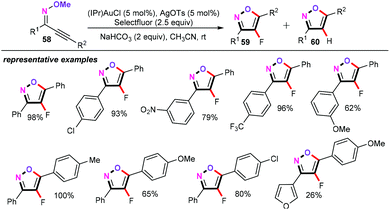 |
| Scheme 27 Gold-catalyzed cascade cyclization/fluorination reaction. | |
Several control experiments were carried out to gain mechanistic insights into the details of this process. For instance, in their NMR experiment, the authors observed the generation of CH3OTs, which revealed that the anion OTs− can act as a demethylating reagent in this cascade procedure. Significantly, the isoxazolyl-gold complex Int-35 was further synthesized and characterized by X-ray crystallography analysis, which may provide direct evidence for this type of catalytic cycle. Based on the above observations and the previous studies,46 a plausible reaction mechanism was subsequently postulated in Scheme 28. With Au(I) as the catalyst, the initial cyclization of O-methyl oximes 58 leads to the vinyl Au(I) complex Int-33, which undergoes demethylation with the aid of the anion OTs− forming the Au(I) complex Int-34. Subsequently, oxidation by Selectfluor generates the cationic Au(III) intermediate Int-35 bearing both isoxazole and fluoride groups. Finally, a reductive elimination produces the desired 4-fluoroisoxazoles 59 and the active Au(I) catalytic species.
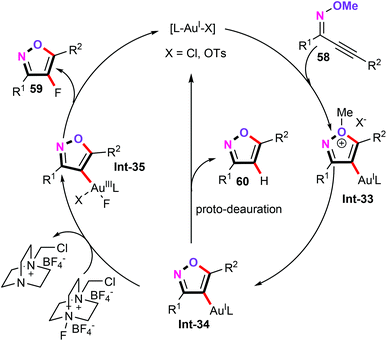 |
| Scheme 28 Plausible mechanism for the gold-catalyzed cascade cyclization/fluorination reaction. | |
But so far, limited strategies have been achieved towards the alkoxyboration of the alkynes.47 As their extension studies, in 2016, Blum and co-workers disclosed a gold-catalyzed or uncatalyzed cascade oxyboration reaction of alkynyl oximes (61) for the synthesis of 4-borylated isoxazoles (64) (Scheme 29).48 This cascade reaction is an operationally simple one-pot, three-step procedure, including boric esterification, oxyboration, and further functional transformation, delivering a wide range of 4-borylated isoxazoles in excellent yields (ranging from 85% to 98%). More importantly, both the metal-catalyzed and the uncatalyzed oxyboration pathways are tolerated with some sensitive functional groups such as furyl, bromo, nitro, and ester groups, which are not compatible with the previous borylation strategies. Notably, this cascade procedure begins with alkynyl oximes 61, which can easily convert into the corresponding boric ester intermediate 62 using catecholborane reagent (HBcat). Then, with the metal-catalyzed and the uncatalyzed oxyboration conditions, two pathways afford the borylated isoxazoles 63. However, compared with the metal-catalyzed process, the catalyst-free conditions required higher temperatures and longer reaction times (almost 1–21 days).
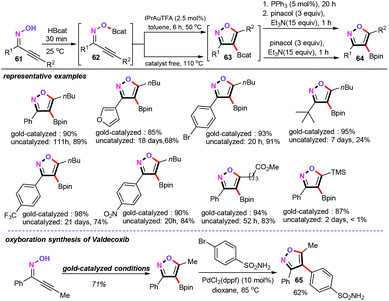 |
| Scheme 29 Oxyboration of alkynyl oximes for the synthesis of 4-borylated isoxazoles. | |
4 Iron catalysts
In recent years, iron-catalyzed or mediated chemical transformations have emerged as a powerful tool for generating new carbon–carbon and carbon–heteroatom bonds and, hence, have become the most attractive research area in both contemporary organic synthesis and industrial processes.49
In 2010, Campagne and co-workers developed the one-pot synthesis of substituted isoxazoles (68) from the propargylic alcohols (66) (Scheme 30).50 Et3N as the base may play an important role in yielding different heteroaromatic motifs with high regioselectivity. With 2.5 mol% FeCl3 as the catalyst, the disubstituted isoxazoles were obtained in moderate to good yields. Both aliphatic alkynes and aromatic alkynes were perfectly accommodated under the optimized reaction conditions. From a mechanistic point of view, propargylic hydroxylamines Int-36in situ generated from propargylic alcohols with N-sulfonyl-protected hydroxyl amines (67) were believed to be the key intermediate in this chemical transformation.
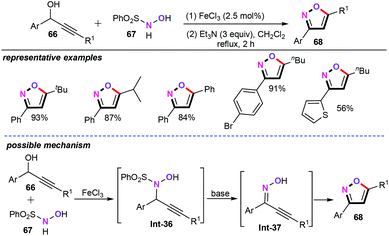 |
| Scheme 30 Iron-catalyzed protocol for the one-pot synthesis of isoxazoles. | |
In 2013, Zeni and co-workers demonstrated an elegant iron-mediated intramolecular cyclization of alkynone O-methyloximes (69) with diorganyl diselenides (70) for the assembly of a library of 4-organoselenylisoxazoles (71) (Scheme 31).51 This selenylation approach smoothly proceeded in the presence of 1.5 equiv. of FeCl3 in CH2Cl2 at room temperature for 5 min, providing the desired 4-organoselenylisoxazoles in moderate yields with high regioselectivity. Diaryl diselenides bearing either electron-donating groups such as methyl, or electron-withdrawing groups, such as trifluoromethyl, on the phenyl ring were well accommodated. Significantly, dialkyl diselenides such as dibutyl diselenides were also compatible with the developed conditions. Notably, the newly obtained 4-organoselenylisoxazoles can react with nbutyllithium, followed by trapping with several electrophiles, to afford the functionalized isoxazoles in moderate to good yields. In addition, the notable features of this method include short reaction time, wide substrate scope, and the ability to carry out the reaction under room temperature conditions without the need for additives. However, the equivalent dosage of FeCl3 catalyst may be the limitation of this protocol.
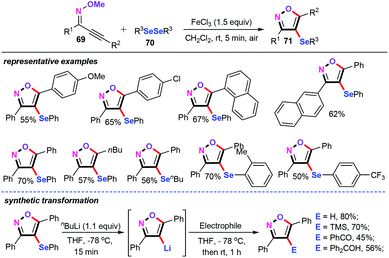 |
| Scheme 31 FeCl3-mediated intramolecular cyclization of alkynone O-methyloximes. | |
Based on the obtained mechanistic studies and the previous literature reports, a plausible mechanism for the FeCl3-mediated intramolecular cyclization reaction was proposed, as demonstrated in Scheme 32. Firstly, when FeCl3 and diorganyl diselenide (R3Se)2 were allowed to react in CH2Cl2 at room temperature, reactive species R3SeFeCl2 (Int-38) and R3SeCl (Int-39) were obtained. For path a, electrophilic addition of alkynes with R3SeCl forms the selenonium intermediate Int-40, which can undergo sequential anti-nucleophilic attack and the elimination of methyl chloride giving the desired products 71. Alternatively, the coordination of the iron species R3SeFeCl2 with the carbon–carbon triple bond of the alkynes forms the iron complex Int-42. Similarly, the anti-nucleophilic attack of the oxygen atom affords the isoxazolyliron species Int-43, which undergoes a reductive elimination procedure to produce the target products 4-selenylisoxazoles.
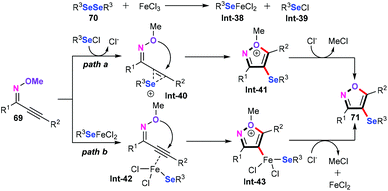 |
| Scheme 32 Plausible mechanism for the FeCl3-mediated intramolecular cyclization reaction. | |
Notably, 2H-azirines as the structurally flexible organic synthons have been used for the preparation of polyfunctionalized heterocycles such as isoxazoles or oxazoles under transition metal-catalyzed or photochemical conditions.52 In this context, Zhao and Du and co-workers have extended the above-mentioned strategies to the iron-catalyzed ring expansion of 2H-azirine from enaminones (72) to access isoxazoles (73) in a one-pot manner (Scheme 33).53 Using FeCl2 as an inexpensive, nontoxic, and efficient catalyst, a wide range of functional groups such as methoxyl, nitro, and benzyl were well tolerated under this catalytic system, giving the desired products 73 in satisfactory yields. Furthermore, the observations of control experiments suggested that 2H-azirine Int-44 may be the possible intermediate in this chemical transformation. Then, the coordination of FeCl2 with the nitrogen atom of intermediate Int-44 gives the iron–azirine complex Int-45. Finally, the sequential 6π-electron electrocyclization and demetallization of intermediate Int-46 generate the trisubstituted isoxazoles.
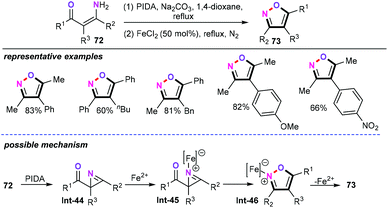 |
| Scheme 33 Iron-catalyzed ring expansion of 2H-azirine from enaminones. | |
In addition, the Xu group reported an iron-mediated cascade cyclization approach for the synthesis of diverse isoxazoles from alkynes with iron nitrate as the nitration reagent (Scheme 34).54 Further optimization of the reaction conditions showed that the kinds of additives were indispensable for this type of transformation. With KI as the additive, the self-coupling of alkynes can smoothly proceed in the presence of 1.5 equiv. Fe(NO3)3·9H2O in THF at 60 °C for 16 h under a nitrogen atmosphere, generating the corresponding products 75 in moderate yields with high regioselectivity.
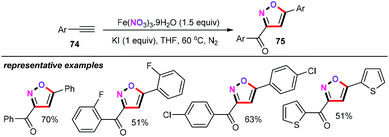 |
| Scheme 34 Iron-mediated self-coupling of alkynes. | |
Particularly interesting is the fact that when KI and t-BuCN were selected as the additives, the cross-coupling of terminal alkynes (76) with electron-deficient alkynes (77) were also perfectly accommodated under the similar reaction conditions (Scheme 35).54 The substrate scopes and limitations of both terminal alkynes and electron-deficient alkynes were then comprehensively evaluated. Remarkably, propiolamide and alkynyl sulfonate were also tolerated well, albeit giving the corresponding isoxazole derivatives 78 in lower yields.
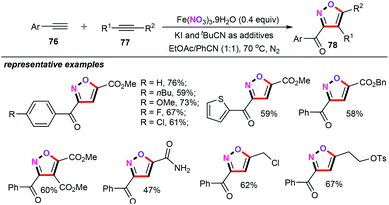 |
| Scheme 35 Iron-mediated cross-coupling of terminal alkynes with electron-deficient alkynes. | |
5 Copper catalysts
Owing to its Earth-abundance, low cost, and relative insensitivity to water and air, the metal copper can be applied as a tunable and multifunctional catalyst for the preparation of value-added organic molecules in a more eco-friendly and economic manner.55 In this regard, Maiti and Chanda and co-workers developed a Cu-catalyzed microwave assisted 1,3-dipolar cycloaddition reaction of readily available aromatic aldehydes (79) with various alkynes (81) in water for the preparation of structurally diverse 3,5-disubstituted isoxazoles (82) (Scheme 36).56 This new approach provides a rapid and highly effective synthetic strategy for constructing polyfunctionalized isoxazoles with good functional group tolerance. And, a wide array of functional groups such as halide, nitro, ester, hydroxyl, and trimethylsilyl groups were perfectly accommodated for this transformation, affording the corresponding products in good to excellent yields.
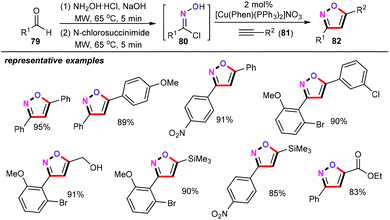 |
| Scheme 36 Cu-Catalyzed microwave assisted 1,3-dipolar cycloaddition reaction. | |
Additionally, transition-metal-catalyzed cross-coupling reactions involving diazo compounds also gained much attention in recent years. In 2018, a novel and atom-economical [3 + 2] cycloaddition reaction of β-keto esters (83) with nitrile oxides (Int-49) (generated in situ from copper carbene (Int-47) and tert-butyl nitrite (tBuONO)), delivering fully substituted isoxazoles (85) with high regio- and stereoselectivity, was successfully described by Wan and Bao and co-workers (Scheme 37).57 Gratifyingly, the authors found that the addition of Mg(OTf)2 (10 mol%) as the additive was crucial for achieving high yields. Further screening of the reaction conditions revealed that 2.5 equiv. of DABCO as the base was indispensable for this chemical transformation. The substrate scope was then evaluated, and both the β-keto esters and diazo compounds showed a wide substrate applicability under the optimized conditions. Detailed mechanistic investigations revealed that copper catalyst can accelerate the transfer of the diazo compounds to form nitrile oxide Int-49 intermediates in this reaction.
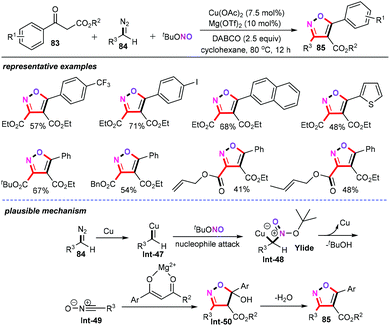 |
| Scheme 37 Copper-catalyzed three-component cascade [3 + 2] cycloaddition reaction. | |
In 2019, Zhao and Yuan and co-workers disclosed an attractive and elegant copper-catalyzed three-component [3 + 2] cycloaddition reaction of alkynes (86), tert-butyl nitrite, and diazo compounds (87) for the preparation of synthetically versatile isoxazole derivatives (88) (Scheme 38).58 It was found that 10 mol% Cu(OAc)2 can efficiently catalyze this three-component cascade cycloaddition reaction in the presence of 1 equiv. DABCO as the base in toluene at 130 °C for 6–10 h. A wide range of functional groups, such as halogen, pyridyl, thienyl, ester, cycloalkyl, and carbonyl groups, were tolerated well under the current catalytic system, affording the corresponding products 88 in moderate yields. Moreover, electron-rich alkyne substrates such as ethynyltrimethylsilane was also compatible with this protocol. Mechanistically, similar to the previous findings, nitrile oxides generated in situ from copper carbene and diazo compounds were the key intermediate for this chemical transformation.
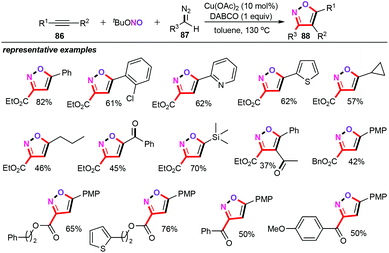 |
| Scheme 38 Copper-catalyzed three-component [3 + 2] cycloaddition reaction of alkynes. | |
Copper nitrate was envisioned as an effective nitration reagent and catalyst to assemble more elaborated heterocyclic structural motifs. Based on their previous research,59 the Xu group disclosed a copper-mediated regioselective cascade annulation approach from two different alkynes for the formation of pharmacologically interesting polysubstituted isoxazoles (91) (Scheme 39).60 Other types of nitrogen sources such as Fe(NO3)3, Co(NO3)2, KNO3, and tBuONO were also evaluated; however, no positive results were obtained. Thus, copper nitrate as the nitrogen source was essential for the success of this chemical transformation. Moreover, PhCN as the ideal solvent had a profound effect on the efficiency of this synthetic strategy. This cascade annulation approach can be performed in the presence of 2 equiv. of Cu(NO3)2 in PhCN at 60 °C for 2.5 h under N2 atmospheric conditions, furnishing the corresponding polysubstituted isoxazoles 91 in moderate to good yields with excellent regioselectivities. Importantly, dimeric isoxazole products could also be achieved from dipropargyl amine. Notably, the newly obtained isoxazoles can be conveniently converted into the muscarinic antagonist 92 and the major depressive disorder reagent 93 by the simple synthetic transformation,61 showing potential applications in pharmaceutical chemistry.
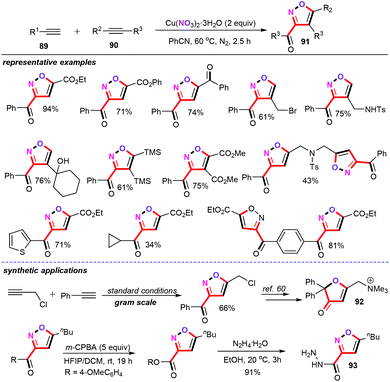 |
| Scheme 39 Copper-mediated cascade annulation approach from two different alkynes. | |
6 Other metal catalysts
6.1 Silver catalysts
Silver has been widely utilized for the straightforward and rapid assembly of structurally diverse functionalized heterocyclic scaffolds. In this research area, Miyata and co-workers explored a silver-catalyzed cyclization of alkynyl oxime ethers (94) for the construction of various functionalized disubstituted isoxazoles (95) (Scheme 40).62 Further investigations demonstrated that PhOH as the proton source is essential for the protonation process. Other types of proton sources such as MeOH and AcOH were shown to be particularly less effective for this transformation. Under the optimized reaction conditions, both aromatic and aliphatic alkynyl oxime ethers were effective substrates in this catalytic reaction, thus, giving the corresponding disubstituted isoxazoles products in synthetically useful yields with high levels of positional selectivity. Interestingly, this approach provided a facile and environmentally benign way to access the biologically active isoxazolecarboxylic acid 96, which can be used as the Mycobacterium tuberculosis phosphatase PtpB inhibitor.63
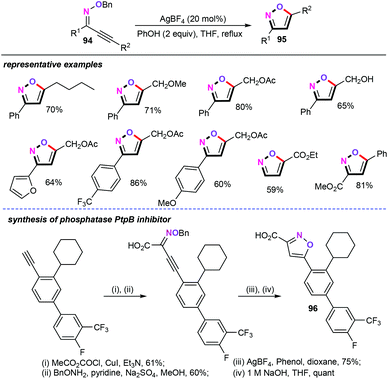 |
| Scheme 40 Silver-catalyzed cyclization of alkynyl oxime ethers. | |
Based on the preliminary mechanistic studies, a plausible mechanism was then postulated in Scheme 41. Firstly, the silver-catalyzed 5-endo-dig cyclization gives an oxonium silver intermediate Int-52, followed by sequential protonation and the aromatization process generating the desired disubstituted isoxazoles.
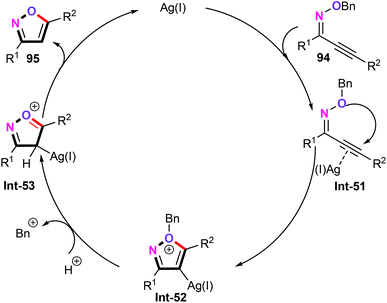 |
| Scheme 41 Plausible mechanism for silver-catalyzed cyclization. | |
6.2 Aluminium catalysts
Recently, Yang and co-workers described an elegant and practical AlCl3-mediated cascade cyclization/sulfenylation of alkynone O-methyloximes (97) with an electrophilic source for the synthesis of 4-sulfenyl isoxazoles (100) (Scheme 42).64 In the presence of 1 equiv. AlCl3 in MeNO2 at 60 °C for 3–7 h, a whole variety of functional groups of both alkynone O-methyloximes and N-arylsulfanylsuccinimides (98) such as alkyl, alkoxyl, halo, ester, nitro, trimethylsilyl, and thienyl were well tolerated, delivering the corresponding products in good yields. Moreover, this protocol can be performed in a gram scale despite a slight decrease in the reaction efficiency. Additionally, the substrate scope of the dialkyl disulfides (99) was then evaluated under similar conditions, albeit with a slight decrease in the yields.
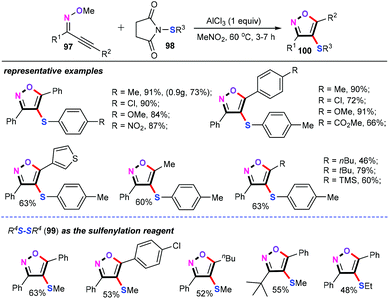 |
| Scheme 42 AlCl3-mediated cascade cyclization/sulfenylation. | |
Detailed mechanistic studies indicated that the sulfenium cation Int-54 was the key active species in this cascade process. Based on the obtained results, a plausible mechanism of this cascade cyclization/sulfenylation is described in Scheme 43. Initially, the activation of N-arylsulfanylsuccinimides (98) with AlCl3 forms Int-54. After this, the electrophile attack of alkynes gives the sulfonium cation Int-55. Subsequently, intramolecular nucleophilic cyclization of the oxygen atom affords intermediate Int-57, which can undergo demethylation in the presence of Cl− to furnish the desired 4-sulfenyl isoxazoles 100.
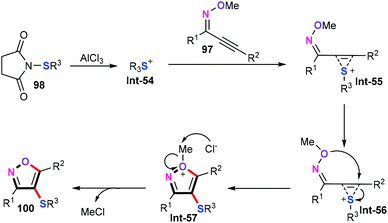 |
| Scheme 43 Plausible mechanism for AlCl3-mediated cascade cyclization/sulfenylation. | |
6.3 Ruthenium catalysts
In 2014, Fokin and co-workers developed a ruthenium-catalyzed cycloaddition reaction of haloalkynes (102) with nitrile oxides for the assembly of 4-haloisoxazoles (103) with high atom- and step-economy (Scheme 44).65 Generally, alkynyl nitrile oxides Int-58 can be generated in situ in the presence of DIPEA (diisopropylethylamine) as the base at ambient temperature. Subsequent studies indicated that [CpRuCl(cod)] was identified as the most active catalyst for this transformation. Under the optimized conditions, the substrate scope and limitations of haloalkynes were then investigated. Satisfactorily, various structurally diverse haloalkynes such as propiolic amides, esters, ketones, and phosphonates were good candidates, and produced the expected products in moderate to good yields with high regioselectivities. Interestingly, 1-choro-, 1-bromo-, and 1-iodoalkynes also performed well under the optimized conditions. Notably, the obtained 4-haloisoxazoles can be further derivatized by palladium-catalyzed cross-coupling reactions.66,67
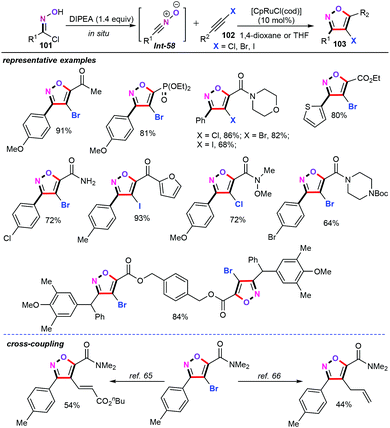 |
| Scheme 44 Ruthenium-catalyzed cycloaddition reaction of haloalkynes with nitrile oxides. | |
6.4 Platinum catalysts
In 2013, Ferreira and co-workers developed a highly efficient platinum-catalyzed cyclization of both propargylic N-hydroxylamines (104) and propargylic amino ethers (105) to furnish regioisomeric isoxazoles (106 and 107) (Scheme 45).68 Additional investigations of the monodentate phosphorus ligands revealed that the P(OPh)3 was essential for this chemical transformation, while other ligands such as PCy3, PPh3, and P(nBu)3 did not improve the yields. Moreover, an array of acid additives were also evaluated, and trifluoroacetic acid (TFA) was found to be the optimal acid for this transformation. This robust platinum-catalyzed system displayed excellent reactivity, and a wide range of sensitive functional groups, such as vinyl, silyloxymethyl, sulfinyl, and sulfoxide groups, were well tolerated under the current catalytic system. Notably, this methodology could be further applied to rapidly access medicinal active molecules.69 Detailed mechanistic studies indicated that the platinum carbene intermediate Int-59 is involved in this chemical transformation.
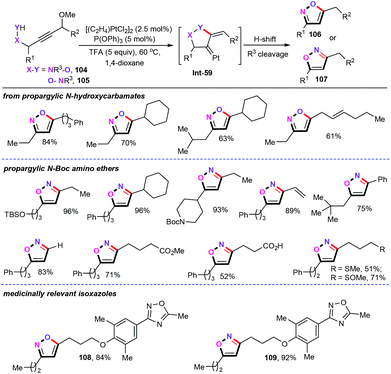 |
| Scheme 45 Platinum-catalyzed cyclizations for the synthesis of regioisomeric isoxazoles. | |
6.5 Scandium catalysts
In 2017, Patel and co-workers described a scandium-catalyzed domino process of terminal aryl alkenes and alkynes with tert-butyl nitrite for the straightforward preparation of functionalized isoxazolines (110) and isoxazole (111) derivatives (Scheme 46).70 By using 5 mol% of Sc(OTf)3 as the catalyst and 0.5 equiv. of quinoline as the base, together with DCE (1,2-dichloroethane) as the solvent, the unactivated olefins were allowed to react with tert-butyl nitrite, producing the isoxazoline products 110 in moderate yields. Additionally, this protocol showed excellent substrate scope and functional group compatibility on the aromatic ring moiety, including halogen, nitro, and carboxylic acid ester groups. However, the substrate scope and limitations of the aliphatic olefins were not investigated under the optimized conditions. Gratifyingly, under the same reaction conditions, a wide variety of alkynes can be successfully coupled with tert-butyl nitrite, albeit providing the corresponding isoxazole derivatives 111 in lower yields. A plausible mechanism involving the NO˙ radical from the tert-butyl nitrite was then proposed based on the observations of control experiments.
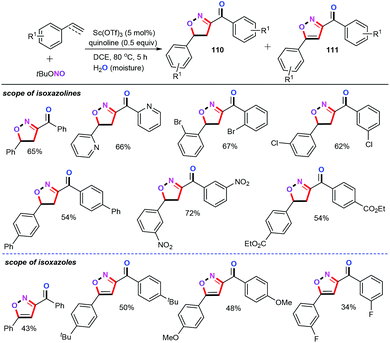 |
| Scheme 46 Scandium-catalyzed cascade cyclization of alkenes and alkynes with tert-butyl nitrite. | |
6.6 Rhodium catalysts
Recently, Nakamura and co-workers discovered a rhodium(III)-catalyzed carboxylate-directed C–H functionalization of isoxazoles (112) with alkynes (113) (Scheme 47).71 In the presence of 5 mol% of [Cp*RhCl2]2 as the catalyst and 1 equiv. of Ag2CO3 as the oxidant in DMF at 100 °C for 2 h in open air, this new protocol provided a facile and efficient synthetic pathway for preparing functionalized pyranoisoxazolones (114) in good to excellent yields with high regioselectivities and exceptional functional group tolerance. Specifically, both symmetrical and unsymmetrical aliphatic internal alkynes were perfectly accommodated, furnishing the desired products in good yields. In addition, this approach showed high levels of oxidant dependency. When the oxidant Ag2CO3 was replaced by Cu(OAc)2·H2O under the similar reaction conditions, the carboxylate-direct isoxazoles were allowed to react with various alkyl and aryl alkynes to obtain the corresponding isoquinoline derivatives (115) in moderate yields. Further investigation showed that the hydroarylation process of isoxazolyl-4-carboxylic acids with alkynes could also proceed efficiently in the presence of [Cp*Rh(CH3CN)3][SbF6]2 (2.5 mol%) as the catalyst, AdCO2H (1 equiv.) as the additive, and K2CO3 (0.3 equiv.) as the base, affording the corresponding products 116 in good yields. However, symmetrical aliphatic internal alkynes were not compatible with the current catalytic system.
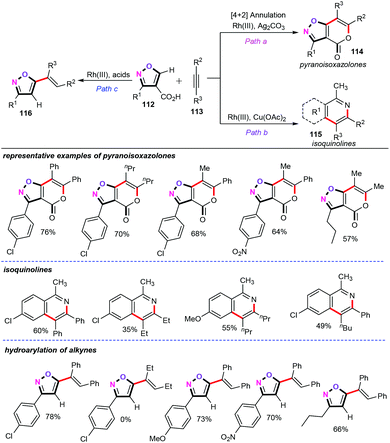 |
| Scheme 47 Rhodium(III)-catalyzed carboxylate-directed C–H functionalizations of isoxazoles with alkynes. | |
6.7 Indium catalysts
Very recently, the Li, Wu, and Hu group successfully accomplished a novel indium-catalyzed 1,3,4-trifunctionalization cascade of 1,3-enynes (117) with sodium sulfinates (118) and t-BuONO (TBN) for the synthesis of diversiform 5-sulfonylisoxazoles (119) (Scheme 48).72 Initially, a variety of metal catalysts such as InCl3, In(OAc)3, In(OTf)3, CuBr2, and FeCl3 were examined, which were shown to be less effective than InBr3 in this transformation. When the reaction was performed under an O2 atmosphere, however, no desired product was detected. Thus, the authors optimized the reaction as follows: InBr3 (10 mol%), MeCN (2 mL) at 60 °C under an argon atmosphere for 8 h. It is noteworthy that a wide range of 1,3-enynes including terminal and substituted internal 1,3-enynes were compatible with the optimized conditions. A variety of functional groups such as alkyl, halogen, trifluoromethyl, methoxyl, nitro, ester, and heterocyclic groups were well tolerated, and delivered the desired products in moderate to good yields with excellent regioselectivities. Control experiments indicated that this synthetic method might undergo a free radical pathway. Based on the current experimental results and the previous reports,73 a tentative reaction mechanism is then proposed. Free radical reaction of sodium sulfinate 118 with t-BuONO forms a sulfonyl radical (Int-61), a NO radical and t-BuONa. And then, radical addition of the sulfonyl radical with 1,3-enynes affords two resonant radical intermediates: alkyl radical Int-62 and allenyl radical Int-63.74 Subsequently, the radical cross coupling of allenyl radical Int-63 with the NO radical generates the allenyl intermediate Int-64, which undergoes successive isomerization and intramolecular nucleophilic cyclization to produce the desired products 119 in the presence of InBr3. The authors also demonstrated that InBr3 plays an important role in this cascade pathway. It not only acts as a Lewis acid to activate the sulfonyl radical but also facilitates the nucleophilic cyclization process.
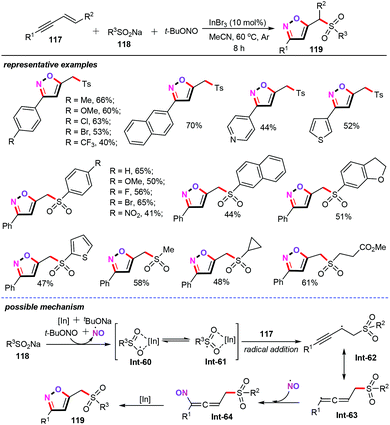 |
| Scheme 48 Indium-catalyzed 1,3,4-trifunctionalization cascade of 1,3-enynes for the synthesis of 5-sulfonylisoxazoles. | |
6.8 Iron and palladium co-catalysts
Bimetallic cooperative catalyzed couplings were also identified as one of the most powerful and fascinating catalytic systems for the synthesis of heteroaromatic scaffolds in the chemical and pharmaceutical industries.75 In this context, the Campagne and Vrancken group demonstrated a Fe/Pd-co-catalyzed cascade one-pot, four-step cyclization of propargylic alcohols (120) for the assembly of functionalized trisubstituted isoxazoles (122) (Scheme 49).76 A range of aryl iodides (121) were allowed to react with propargylic hydroxylamines Int-65 in the presence of Fe/Pd cooperative catalysis conditions, and afforded the desired products in acceptable yields with high regioselective. However, a minor drawback in this synthetic strategy is the requirement of more synthetic steps for this process. A plausible mechanism was then proposed based on the obtained results and the previous literature precedents. Initially, an iron-catalyzed propargylic substitution by N-protected hydroxylamine forms intermediate Int-65. After this, nucleopalladation of the aryl palladium complex generated in situ from aryl iodides gives vinylpalladium intermediate Int-66. Subsequently, a reductive elimination process affords the trisubstituted isoxazoline Int-67. Finally, the subsequent hydrogenation deprotection and oxidative aromatization furnish the target products 122.
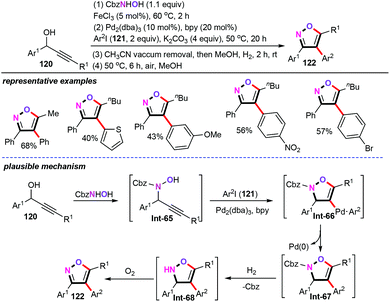 |
| Scheme 49 Iron and palladium-co-catalyzed cascade cyclization of trisubstituted isoxazoles. | |
7. Summary
Isoxazoles and their derivatives have been identified as important and synthetically useful aromatic heterocycles. In particular, polysubstituted isoxazole architectures display significant biological and pharmaceutical activities. As a consequence, extensive studies have been established to developing novel and efficient synthetic methodologies for the assembly of structurally diverse isoxazole scaffolds in the past years. Generally, there are four representative synthetic strategies typically applied for the preparation of these heterocyclic frameworks, including [3 + 2] cycloaddition reactions of alkynes with nitrile oxides, transition metal-catalyzed C–H functionalization of isoxazoles, condensation reactions from β-dicarbonyls with hydroxylamine, and metal-free and metal-catalyzed cycloisomerizations. Among these elegant transformations, transition-metal-catalyzed cascade cyclization/functionalization of alkynes has emerged as flourishing and fascinating research fields for the straightforward synthesis of various high value-added functionalized isoxazoles in a step- and atom-economical manner. In particular, a variety of alkynes including alkynone oxime ethers, acetylinic oximes, propargylic amino ethers, alkynyl nitrile oxides, and propargylic alcohols have been investigated for these cascade cyclization/functionalization process. And a wide array of metal catalysts, such as palladium, copper, iron, gold, silver, and other metals have been typically employed in recent years. It is worth mentioning that palladium catalysts occupy a privileged position mainly due to their remarkable versatility and high catalytic activity. Some of the described approaches in this review manifested that the developed metal catalyzed or mediated cascade cyclization reaction could provide rapid and straightforward approaches for the preparation of structurally flexible pharmaceuticals and biologically active isoxazole molecules by further structural modification and synthetic transformations. Undoubtedly, metal catalyzed or mediated cyclization/functionalization of alkynes represents the most straightforward and efficient synthetic methodologies for the rapid and precise construction of these important frameworks.
Despite the significant achievements, there remain some challenges and problems that need to be overcome. More importantly, metal-free synthetic methodologies also present an important and alternative development trend in this field in the recent five years.77 More environmentally friendly synthetic methodologies, such as photocatalytic synthesis, electrochemical synthesis, and enzymatic synthesis would be fascinating research fields in the future. In light of the ever-increasing importance of complicated isoxazole skeletons in pharmaceutical agents, preclinical candidates, and material aspects, the development of green and efficient alternatives to the construction of these special heterocyclic architectures in an eco-friendly manner will become an important and promising research area. This review will provide a new perspective for the synthesis of functionalized isoxazoles and can be further applied by a large number of synthetic and pharmaceutical chemists. We also believe that the exploration of metal catalyzed or mediated synthesis of isoxazoles from alkynes will continue to be flourishing and captivating.
Conflicts of interest
The authors declare no competing financial interest.
Acknowledgements
We thank the Ministry of Science and Technology of the People's Republic of China (2016YFA0602900), the National Natural Science Foundation of China (21971072), the Fundamental Research Funds for the Central Universities (2018ZD16), and the Pearl River S&T Nova Program of Guangzhou (201906010072) for financial support.
References
- L. Claisen and O. Lowman, Zur Kenntniss des Benzoylacetons, Ber. Dtsch. Chem. Ges., 1888, 21, 1149–1157 CrossRef.
-
(a) Y. Kanda, T. Takahashi, Y. Araki, T. Konoike, S.-I. Mihara and M. Fujimoto, Discovery and synthesis of a potent sulfonamide ETB selective antagonist, Bioorg. Med. Chem. Lett., 2000, 10, 1875–1878 CrossRef CAS PubMed;
(b) J. Sun, C. Lin, X. Qin, X. Dong, Z. Tu, F. Tang, C. Chen and J. Zhang, Synthesis and biological evaluation of 3,5-disubstituted-4-alkynylisoxozales as a novel class of HSP90 inhibitors, Bioorg. Med. Chem. Lett., 2015, 25, 3129–3134 CrossRef CAS PubMed;
(c) J. Zhu, J. Mo, H. Lin, Y. Chen and H.-P. Sun, The recent progress of isoxazole in medicinal chemistry, Bioorg. Med. Chem., 2018, 26, 3065–3075 CrossRef CAS PubMed.
- For selected reviews:
(a)
B. J. Wakefield, in Science of Synthesis: Houben-Weyl Methods of Molecular Transformations, ed. E. Schaumann, Georg Thieme Verlag, Stuttgart, New York, 2004, vol. 11, pp. 229–288 Search PubMed;
(b)
A. M. S. Silva, A. C. Tome, T. M. V. D. Pinho e Melo and J. Elguero, in Modern Heterocyclic Chemistry, ed. J. Alvarez-Builla, J. J. Vaquero and J. Barluenga, WileyVCH, Weinheim, 2011, pp. 727–808 Search PubMed.
-
(a) R. G. Micetich and R. Raap, Penicillins from 3- and 5-phenylisothiazole-4-carboxylic acids and alkoxy derivatives, J. Med. Chem., 1968, 11, 159–160 CrossRef CAS PubMed;
(b) A. Severin, K. Tabei, F. Tenover, M. Chung, N. Clarke and A. Tomasz, High level Oxacillin and Vancomycin resistance and altered cell wall composition in staphylococcus aureus carrying the staphylococcal mecA and the enterococcal vanA gene complex, J. Biol. Chem., 2004, 279, 3398–3407 CrossRef CAS PubMed.
- M. R. Jensen, J. Schoepfer, T. Radimerski, A. Massey, C. T. Guy, J. Brueggen, C. Quadt, A. Buckler, R. Cozens, M. J. Drysdale, C. Garcia-Echeverria and P. Chène, NVP-AUY922: a small molecule HSP90 inhibitor with potent antitumor activity in preclinical breast cancer models, Breast Cancer Res., 2008, 10, R33 CrossRef PubMed.
- R. D. Taylor, M. MacCoss and A. D. G. Lawson, Rings in drugs, J. Med. Chem., 2014, 57, 5845–5859 CrossRef CAS PubMed.
-
(a) P. Vitale and A. Scilimati, Recent developments in the chemistry of 3-arylisoxazoles and 3-aryl-2-isoxazolines, Adv. Heterocycl. Chem., 2017, 122, 1–41 CrossRef CAS;
(b) L. Li, T.-D. Tan, Y.-Q. Zhang, X. Liu and L.-W. Ye, Recent advances in transition-metal-catalyzed reactions of alkynes with isoxazoles, Org. Biomol. Chem., 2017, 15, 8483–8492 RSC.
-
(a) T. M. V. D. Pinho e Melo, Recent advances on the synthesis and reactivity of isoxazoles, Curr. Org. Chem., 2005, 9, 925–958 CrossRef CAS;
(b) J.-J. Wen, Y. Zhu and Z.-P. Zhan, The synthesis of aromatic heterocycles from propargylic compounds, Asian J. Org. Chem., 2012, 1, 108–129 CrossRef CAS.
-
(a) T. M. V. D. Pinho e Melo, 4-Isoxazolines: scaffolds for organic synthesis, Eur. J. Org. Chem., 2010, 3363–3376 CrossRef CAS;
(b) F. Heaney, Nitrile oxide/alkyne cycloadditions: a credible platform for synthesis of bioinspired molecules by metal-free molecular clicking, Eur. J. Org. Chem., 2012, 3043–3048 CrossRef CAS;
(c) A. V. Gulevich, A. S. Dudnik, N. Chernyak and V. Gevorgyan, Transition metal-mediated synthesis of monocyclic aromatic heterocycles, Chem. Rev., 2013, 113, 3084–3213 CrossRef CAS;
(d) S. Roscales and J. Plumet, Metal-catalyzed 1,3-dipolar cycloaddition reactions of nitrile oxides, Org. Biomol. Chem., 2018, 16, 8446–8461 RSC.
-
(a) Y. Fall, C. Reynaud, H. Doucet and M. Santelli, Ligand-free-palladium-catalyzed
direct 4-arylation of isoxazoles using aryl bromides, Eur. J. Org. Chem., 2009, 4041–4050 CrossRef CAS;
(b) D. Roy, S. Mom, S. Royer, D. Lucas, J. C. Hierso and H. Doucet, Palladium-catalyzed direct arylation of heteroaromatics with activated aryl chlorides using a sterically relieved ferrocenyl-diphosphane, ACS Catal., 2012, 2, 1033–1041 CrossRef CAS;
(c) B. Chappell, N. Dedman and S. Wheeler, Studies on the palladium-catalysed direct alkenylation of 1,2-azoles, Tetrahedron Lett., 2011, 52, 3223–3225 CrossRef CAS;
(d) I. I. F. Boogaerts and S. P. Nolan, Carboxylation of C-H bonds using N-heterocyclic carbene gold(I) complexes, J. Am. Chem. Soc., 2010, 132, 8858–8859 CrossRef CAS PubMed.
-
(a) T. M. V. Pinho e Melo, Recent advances on the synthesis and reactivity of isoxazoles, Curr. Org. Chem., 2005, 9, 925–958 CrossRef CAS;
(b) N. T. Patil and Y. Yamamoto, Coinage metal-assisted synthesis of heterocycles, Chem. Rev., 2008, 108, 3395–3442 CrossRef CAS PubMed.
-
(a) W. Jia-Jie, Y. Zhu and Z.-P. Zhan, The synthesis of aromatic heterocycles from propargylic compounds, Asian J. Org. Chem., 2012, 1, 108–129 CrossRef;
(b) T. Morita, S. Yugandar, S. Fuse and H. Nakamura, Recent progresses in the synthesis of functionalized isoxazoles, Tetrahedron Lett., 2018, 59, 1159–1171 CrossRef CAS.
- F. Hu and M. Szostak, Recent developments in the synthesis and reactivity of isoxazoles: metal catalysis and beyond, Adv. Synth. Catal., 2015, 357, 2583–2614 CrossRef CAS.
- For selected reviews, see:
(a) W. Wu and H. Jiang, Haloalkynes: a powerful and versatile building block in organic synthesis, Acc. Chem. Res., 2014, 47, 2483–2504 CrossRef CAS;
(b)
J. Li and H. Jiang, in Noble Metal Noble Value: Ru-, Rh-, Pd-catalyzed Heterocycle Synthesis, ed. X. Wu, World Scientific Publishing Co Pte Ltd., Singapore, 2016, p. 351 Search PubMed. For selected examples, see:
(c) L. Huang, Q. Wang, X. Liu and H. Jiang, Switch of selectivity in the synthesis of α-methylene-γ-lactones: palladium-catalyzed intermolecular carboesterification of alkenes with alkynes, Angew. Chem., Int. Ed., 2012, 51, 5696–5700 CrossRef CAS PubMed;
(d) J. Li, W. Yang, S. Yang, L. Huang, W. Wu, Y. Sun and H. Jiang, Palladium-catalyzed cascade annulation to construct functionalized β- and γ-lactones in ionic liquids, Angew. Chem., Int. Ed., 2014, 53, 7219–7222 CrossRef CAS PubMed;
(e) L. Ouyang, J. Li, J. Zheng, J. Huang, C. Qi, W. Wu and H. Jiang, Access to α-amino acid esters through palladium-catalyzed oxidative amination of vinyl ethers with hydrogen peroxide as the oxidant and oxygen source, Angew. Chem., Int. Ed., 2017, 56, 15926–15930 CrossRef CAS PubMed.
- J. Li, S. Yang, W. Wu and H. Jiang, Recent Advances in Pd-catalyzed cross-coupling reaction in ionic liquids, Eur. J. Org. Chem., 2018, 1284–1306 CrossRef CAS.
- J. Li, S. Yang, W. Wu and H. Jiang, Palladium-catalyzed cascade cyclization/alkynylation reactions, Chem. – Asian J., 2019, 14, 4114–4128 CrossRef CAS.
-
(a)
E. J. Nigishi, Handbook of Organopalladium Chemistryf or Organic Synthesis, Wiley-VCH, Weinheim, 2002, vol. 2 Search PubMed;
(b) L.-N. Guo, X.-H. Duan and Y.-M. Liang, Palladium-catalyzed cyclization of propargylic compounds, Acc. Chem. Res., 2011, 44, 111–122 CrossRef CAS PubMed;
(c) X.-F. Wu, H. Neumann and M. Beller, Update 1 of: macrolactonizations in the total synthesis of natural products, Chem. Rev., 2013, 113, 1–35 CrossRef CAS PubMed;
(d) J. Ye and S. Ma, Palladium-catalyzed cyclization reactions of allenes in the presence of unsaturated carbon-carbon bonds, Acc. Chem. Res., 2014, 47, 989–1000 CrossRef CAS PubMed;
(e) G. Yin, X. Mu and G. Liu, Palladium(II)-catalyzed oxidative difunctionalization of alkenes: bond forming at a high-valent palladium center, Acc. Chem. Res., 2016, 49, 2413–2423 CrossRef CAS PubMed;
(f) D. Wang, A. B. Weinstein, P. B. White and S. S. Stahl, Ligand-promoted palladium-catalyzed aerobic oxidation reactions, Chem. Rev., 2018, 118, 2636–2679 CrossRef CAS PubMed.
- Z. She, D. Niu, L. Chen, M. A. Gunawan, X. Shanja, W. H. Hersh and Y. Chen, Synthesis of trisubstituted isoxazoles by palladium(II)-catalyzed cascade cyclization-alkenylation of 2-alkyn-1-one O-methyl oximes, J. Org. Chem., 2012, 77, 3627–3633 CrossRef CAS PubMed.
- D.-D. Li, T.-T. Yuan and G.-W. Wang, Synthesis of isoindolinones viapalladium-catalyzed C-H activation of N-methoxybenzamides, Chem. Commun., 2011, 47, 12789–12791 RSC.
- X. Liu, D. Hong, Z. She, W. H. Hersh, B. Yoo and Y. Chen, Complementary regioselective synthesis of 3,5-disubstituted isoxazoles from ynones, Tetrahedron, 2018, 74, 6593–6606 CrossRef CAS.
- J. P. Waldo and R. C. Larock, Synthesis of isoxazoles via electrophilic cyclization, Org. Lett., 2005, 7, 5203–5205 CrossRef CAS.
- W. Wu and H. Jiang, Palladium-catalyzed oxidation of unsaturated hydrocarbons using molecular oxygen, Acc. Chem. Res., 2012, 45, 1736–1748 CrossRef CAS PubMed.
-
(a) X. Tang, W. Wu, W. Zeng and H. Jiang, Copper-catalyzed oxidative carbon-carbon and/or carbon-heteroatom bond formation with O2 or internal oxidants, Acc. Chem. Res., 2018, 51, 1092–1105 CrossRef CAS PubMed;
(b) J. Li, S. Yang, W. Wu and H. Jiang, Recent developments in palladium-catalyzed C-S bond formation, Org. Chem. Front., 2020, 7, 1395–1417 RSC.
- J. Li, M. Hu, C. Li, C. Li, J. Li, W. Wu and H. Jiang, Palladium-catalyzed cascade cyclization/alkynylation and alkenylation of alkynone O-methyloximes with terminal alkynes, Adv. Synth. Catal., 2018, 360, 2707–2719 CrossRef CAS.
- J. Li, R. Huang, C. Li, S. Lin, W. Wu and H. Jiang, Assembly of functionalized 4-alkynylisoxazoles by palladium-catalyzed three-component cascade cyclization/alkynylation, Chem. – Asian J., 2019, 14, 2309–2315 CrossRef CAS PubMed.
- C. M. R. Volla and J.-E. Bäckvall, Palladium-catalyzed aerobic domino oxidative carbocyclization-alkynylation of allenynes, Angew. Chem., Int. Ed., 2013, 52, 14209–14213 CrossRef CAS PubMed.
- C. Li, J. Li, F. Zhou, C. Li and W. Wu, Palladium-catalyzed cascade annulation/allylation of alkynyl oxime ethers with allyl halides: rapid access to fully substituted isoxazoles, J. Org. Chem., 2019, 84, 11958–11970 CrossRef CAS PubMed.
-
(a) S. Ma and Z. Yu, “Walking” of the C-C π bond over long distances in Pd-catalyzed reactions of 2,3-allenoic acids with ω-1-alkenyl halides, Angew. Chem., Int. Ed., 2003, 42, 1955–1957 CrossRef CAS PubMed;
(b) T. Kochi, T. Hamasaki, Y. Aoyama, J. Kawasaki and F. Kakiuchi, Chain-walking strategy for organic synthesis: catalytic cycloisomerization of 1,n-dienes, J. Am. Chem. Soc., 2012, 134, 16544–16547 CrossRef CAS PubMed.
-
(a) J. Li, S. Yang, H. Jiang, W. Wu and J. Zhao, Palladium-catalyzed coupling of alkynes with unactivated alkenes in ionic liquids: a regio- and stereoselective synthesis of functionalized 1,6-dienes and their analogues, J. Org. Chem., 2013, 78, 12477–12486 CrossRef CAS PubMed;
(b) J. Li, S. Yang, L. Huang, H. Chen and H. Jiang, Highly efficient and practical synthesis of functionalized 1,5-dienes via Pd(II)-catalyzed halohomoallylation of alkynes, RSC Adv., 2013, 3, 11529–11532 RSC;
(c) J. Li, W. Hu, C. Li, S. Yang, W. Wu and H. Jiang, Palladium-catalyzed cascade reaction of haloalkynes with unactivated alkenes for assembly of functionalized oxetanes, Org. Chem. Front., 2017, 4, 373–376 RSC.
- W. Wu, C. Li, F. Zhou, J. Li, X. Xu and H. Jiang, Synthesis of β-isoxazole carbonyl derivatives and their analogues via palladium-catalyzed sequential C(sp2)-O/C(sp2)-C(sp3) bond formations, Adv. Synth. Catal., 2019, 361, 3813–3823 CrossRef CAS.
- J. Li, Y. Wu, M. Hu, C. Li, M. Li, D. He and H. Jiang, A palladium-catalyzed three-component cascade S-transfer reaction in ionic liquids, Green Chem., 2019, 21, 4084–4089 RSC.
- Z. Qiao, J. Wei and X. Jiang, Direct cross-coupling access to diverse aromatic sulfide: palladium-catalyzed double C-S bond construction using Na2S2O3 as a sulfurating reagent, Org. Lett., 2014, 16, 1212–1215 CrossRef CAS PubMed.
- J. Li, J. Yu, W. Xiong, H. Tang, M. Hu, W. Wu and H. Jiang, A palladium-catalyzed oxidative aminocarbonylation reaction of alkynone O-methyloximes with amines and CO in PEG-400, Green Chem., 2020, 22, 465–470 RSC.
- S. Liu, H. Wang, X. Dai and F. Shi, Organic ligand-free carbonylation reactions with unsupported bulk Pd as catalyst, Green Chem., 2018, 20, 3457–3462 RSC.
-
(a) A. Fihri, M. Bouhrara, B. Nekoueishahraki, J.-M. Basset and V. Polshettiwar, Chem. Soc. Rev., 2011, 40, 5181–5203 RSC;
(b) C. Deraedt and D. Astruc, Acc. Chem. Res., 2014, 47, 494–503 CrossRef CAS PubMed.
- N. S. Thirukovela, R. Balaboina, V. Botla, R. Vadde, S. B. Jonnalagadda and C. S. Vasam, One-pot regioselective synthesis of substituted pyrazoles and isoxazoles in PEG-400/water medium by Cu-free nano-Pd catalyzed sequential acyl Sonogashira coupling-intramolecular cyclization, Catal. Sci. Technol., 2019, 9, 6471–6481 RSC.
- W. Kaewsri, C. Thongsornkleeb, J. Tummatorn and S. Ruchirawat, Isomerizable (E/Z)-alkynyl-O-methyl oximes employing TMSCl-NCS in chlorinative cyclization for the direct synthesis of 4-chloroisoxazoles, RSC Adv., 2016, 6, 48666–48675 RSC.
- J. Li, S. Lin, R. Huang, C. Li and S. Yang, Palladium-catalyzed cascade cyclization/bromination of 2-alkynone O-methyl oximes in ionic liquid, Chin. J. Org. Chem., 2019, 39, 1417–1423 CrossRef.
- M. Shigenobu, K. Takenaka and H. Sasai, Palladium-catalyzed direct C-H arylation of isoxazoles at the 5-position, Angew. Chem., Int. Ed., 2015, 54, 9572–9576 CrossRef CAS PubMed.
- A. A. G. Fernandes, A. F. da Silva, C. Y. Okada Jr., V. Suzukawa, R. A. Cormanich and I. D. Jurberg, General platform for the conversion of isoxazole-5-ones to 3,5-disubstituted isoxazoles via nucleophilic substitutions and palladium catalyzed cross-coupling strategies, Eur. J. Org. Chem., 2019, 3022–3034 CrossRef CAS.
- M. Ueda, A. Sato, Y. Ikeda, T. Miyoshi, T. Naito and O. Miyata, Direct synthesis of trisubstituted isoxazoles through gold-catalyzed domino reaction of alkynyl oxime ethers, Org. Lett., 2010, 12, 2594–2597 CrossRef CAS PubMed.
- C. Praveen, A. Kalyanasundaram and P. T. Perumal, Gold(III)-catalyzed synthesis of isoxazoles by cycloisomerization of α,β-acetylenic oximes, Synlett, 2010, 777–781 CAS.
- K. K.-Y. Kung, V. K.-Y. Lo, H.-M. Ko, G.-L. Li, P.-Y. Chan, K.-C. Leung, Z. Zhou, M.-Z. Wang, C.-M. Che and M.-K. Wong, Cyclometallated gold(III) complexes as effective catalysts for synthesis of propargylic amines, chiral allenes and isoxazoles, Adv. Synth. Catal., 2013, 355, 2055–2070 CrossRef CAS.
- D.-H. Song and J.-S. Ryu, Synthesis of 3,4,5-trisubstituted isoxazoles through gold-catalyzed cascade cyclization-oxidative alkynylation and cyclization-fluorination of 2-alkynone O-methyloximes, Bull. Korean Chem. Soc., 2014, 35, 2635–2644 CrossRef CAS.
- Y. Jeong, B.-I. Kim, J. K. Lee and J.-S. Ryu, Direct synthesis of 4-fluoroisoxazoles through gold-catalyzed cascade cyclization-fluorination of 2-alkynone O-methyl oximes, J. Org. Chem., 2014, 79, 6444–6455 CrossRef CAS PubMed.
-
(a) J. Qian, Y. Liu, J. Zhu, B. Jiang and Z. Xu, A novel synthesis of fluorinated pyrazoles via gold(I)-catalyzed tandem aminofluorination of alkynes in the presence of selectfluor, Org. Lett., 2011, 13, 4220–4223 CrossRef CAS;
(b) W. Wang, J. Jasinski, G. B. Hammond and B. Xu, Fluorine-enabled cationic gold catalysis: functionalized hydration of alkynes, Angew. Chem., Int. Ed., 2010, 49, 7247–7252 CrossRef CAS.
- J. J. Hirner, D. J. Faizi and S. A. Blum, Alkoxyboration: ring-closing addition of B-O σ bonds across alkynes, J. Am. Chem. Soc., 2014, 136, 4740–4745 CrossRef CAS PubMed.
- K. N. Tu, J. J. Hirner and S. A. Blum, Oxyboration with and without a catalyst: borylated isoxazoles via B–O σ-bond addition, Org. Lett., 2016, 18, 480–483 CrossRef CAS.
-
(a) F. Jia and Z. Li, Iron-catalyzed/mediated oxidative transformation of C-H bonds, Org. Chem. Front., 2014, 1, 194–214 RSC;
(b) A. Correa, O. G. Mancheño and C. Bolm, Iron-catalysed carbon-heteroatom and heteroatom-heteroatom bond forming processes, Chem. Soc. Rev., 2008, 37, 1108–1117 RSC;
(c) Y. Liang, Y.-F. Liang and N. Jiao, Cu- or Fe-catalyzed C-H/C-C bond nitrogenation reactions for the direct synthesis of N-containing compounds, Org. Chem. Front., 2015, 2, 403–415 RSC.
- O. Debleds, E. Gayon, E. Ostaszuk, E. Vrancken and J.-M. Campagne, A versatile iron-catalyzed protocol for the one-pot synthesis of isoxazoles or isoxazolines from the same propargylic alcohols, Chem. – Eur. J., 2010, 16, 12207–12213 CrossRef CAS.
- A. Sperança, B. Godoi and G. Zeni, Iron(III) chloride/diorganyl diselenides: a tool for intramolecular cyclization of alkynone O-methyloximes, J. Org. Chem., 2013, 78, 1630–1637 CrossRef.
-
(a) S. Brahma and J. K. Ray, Synthesis of azirines containing aldehyde functionality and their utilization as synthetic tools for five membered oxazoles and isoxazoles, J. Heterocycl. Chem., 2008, 45, 311–317 CrossRef CAS;
(b) S. Rajam, R. S. Murthy, A. V. Jadhav, Q. Li, C. Keller, C. Carra, T. C. S. Pace, C. Bohne, B. S. Ault and A. D. Gudmundsdottir, Photolysis of (3-methyl-2H-azirin-2-yl)-phenylmethanone: direct detection of a triplet vinylnitrene intermediate, J. Org. Chem., 2011, 76, 9934–9945 CrossRef CAS.
- Y. Zheng, C. Yang, D. Zhang-Negreriea, Y. Du and K. Zhao, One-pot synthesis of isoxazoles from enaminones: an application of Fe(II) as the catalyst for ring expansion of 2H-azirine intermediates, Tetrahedron Lett., 2013, 54, 6157–6160 CrossRef CAS.
- Z. Lai, Z. Li, Y. Liu, P. Yang, X. Fang, W. Zhang, B. Liu, H. Chang, H. Xu and Y. Xu, Iron-mediated synthesis of isoxazoles from alkynes: using iron(III) nitrate as a nitration and cyclization reagent, J. Org. Chem., 2018, 83, 145–153 CrossRef CAS PubMed.
-
(a) S. D. McCann and S. S. Stahl, Copper-catalyzed aerobic oxidations of organic molecules: pathways for two-electron oxidation with a four-electron oxidant and a one-electron redox-active catalyst, Acc. Chem. Res., 2015, 48, 1756–1766 CrossRef CAS;
(b) W.-H. Rao and B.-F. Shi, Recent advances in copper-mediated chelation-assisted functionalization of unactivated C–H bonds, Org. Chem. Front., 2016, 3, 1028–1047 RSC.
- D. R. Meena, B. Maiti and K. Chanda, Cu(I) catalyzed microwave assisted telescopic synthesis of 3,5-disubstituted isoxazoles in green media, Tetrahedron Lett., 2016, 57, 5514–5517 CrossRef CAS.
- R. Chen, A. A. Ogunlana, S. Fang, W. Long, H. Sun, X. Bao and X. Wan,
In situ generation of nitrile oxides from copper carbene and tert-butyl
nitrite: synthesis of fully substituted isoxazoles, Org. Biomol. Chem., 2018, 16, 4683–4687 RSC.
- X.-D. Wang, L.-H. Zhu, P. Liu, X.-Y. Wang, H.-Y. Yuan and Y.-L. Zhao, Copper-catalyzed cascade cyclization reactions of diazo compounds with tert-butyl nitrite and alkynes: one-pot synthesis of isoxazoles, J. Org. Chem., 2019, 84, 16214–16221 CrossRef CAS PubMed.
- M. Gao, Y. Li, Y. Gan and B. Xu, Copper nitrate mediated regioselective [2 + 2 + 1] cyclization of alkynes with alkenes: a cascade approach to isoxazolines, Angew. Chem., Int. Ed., 2015, 54, 8795–8799 CrossRef CAS PubMed.
- Y. Li, M. Gao, B. Liu and B. Xu, Copper nitrate-mediated chemo- and regioselective annulation from two different alkynes: a direct route to isoxazoles, Org. Chem. Front., 2017, 4, 445–449 RSC.
- S. Roscales, N. Bechmann, D. Holger Weiss, M. Köckerling, J. Pietzsch and T. Kniess, Novel valdecoxib derivatives by ruthenium(II)-promoted 1,3-dipolar cycloaddition of nitrile oxides with alkynes-synthesis and COX-2 inhibition activity, Med. Chem. Commun., 2018, 9, 534–544 RSC.
- M. Ueda, Y. Ikeda, A. Sato, Y. Ito, M. Kakiuchi, H. Shono, T. Miyoshi, T. Naito and O. Miyata, Silver-catalyzed synthesis of disubstituted isoxazoles by cyclization of alkynyl oxime ethers, Tetrahedron, 2011, 67, 4612–4615 CrossRef CAS.
- K. A. Rawls, C. Grundner and J. A. Ellman, Design and synthesis of nonpeptidic, small molecule inhibitors for the Mycobacterium tuberculosisprotein tyrosine phosphatase PtpB, Org. Biomol. Chem., 2010, 8, 4066–4070 RSC.
- W.-C. Gao, Y.-F. Cheng, H.-H. Chang, X. Li, W.-L. Wei and P. Yang, Synthesis of 4-sulfenyl isoxazoles through AlCl3-mediated electrophilic cyclization and sulfenylation of 2-alkyn-1-one O-methyloximes, J. Org. Chem., 2019, 84, 4312–4317 CrossRef CAS PubMed.
- J. S. Oakdale, R. K. Sit and V. V. Fokin, Ruthenium-catalyzed cycloadditions of 1-haloalkynes with nitrile oxides and organic azides: synthesis of 4-haloisoxazoles and 5-halotriazoles, Chem. – Eur. J., 2014, 20, 11101–11110 CrossRef CAS PubMed.
- J. P. Waldo and R. C. Larock, The synthesis of highly substituted isoxazoles by electrophilic cyclization: an efficient synthesis of Valdecoxib, J. Org. Chem., 2007, 72, 9643–9647 CrossRef CAS PubMed.
- J. A. Crossley and D. L. Browne, An alkynyliodide cycloaddition strategy for the construction of iodoisoxazoles, J. Org. Chem., 2010, 75, 5414–5416 CrossRef CAS.
- P. A. Allegretti and E. M. Ferreira, Platinum-catalyzed cyclizations via carbene intermediates: syntheses of complementary positional isomers of isoxazoles, Chem. Sci., 2013, 4, 1053–1058 RSC.
- G. D. Diana, D. L. Volkots, T. J. Nitz, T. R. Bailey, M. A. Long, N. Vescio, S. Aldous, D. C. Pevear and F. J. Dutko, Oxadiazoles as ester bioisosteric replacements in compounds related to disoxaril. antirhinovirus activity, J. Med. Chem., 1994, 37, 2421–2436 CrossRef CAS.
- P. Sau, S. K. Santra, A. Rakshit and B. K. Patel,
tert-Butyl nitrite-mediated domino synthesis of isoxazolines and isoxazoles from terminal aryl alkenes and alkynes, J. Org. Chem., 2017, 82, 6358–6365 CrossRef CAS.
- S. Yugandar and H. Nakamura, Rhodium(III)-catalysed carboxylate-directed C-H functionalizations of isoxazoles with alkynes, Chem. Commun., 2019, 55, 8382–8385 RSC.
- X. Yue, M. Hu, X. He, S. Wu and J.-H. Li, A radical-mediated 1,3,4-trifunctionalization cascade of 1,3-enynes with sulfinates and tertbutyl nitrite: facile access to sulfonyl isoxazoles, Chem. Commun., 2020, 56, 6253–6256 RSC.
-
(a) M. Hu, B. Liu, X.-H. Ouyang, R.-J. Song and J.-H. Li, Nitrative cyclization of 1-ethynyl-2-(vinyloxy)benzenes to access 1-[2-(nitromethyl)benzofuran-3-yl] ketones through dioxygen activation, Adv. Synth. Catal., 2015, 357, 3332–3340 CrossRef CAS;
(b) Y. Y. Liu, X. H. Yang, R. J. Song, S. L. Luo and J. H. Li, Oxidative 1,2-carboamination of alkenes with alkyl nitriles and amines toward γ-amino alkyl nitriles, Nat. Commun., 2017, 8, 14720 CrossRef PubMed;
(c) F. Wang, D. Wang, Y. Zhou, L. Liang, R. Lu, P. Chen, Z. Lin and G. Liu, Divergent synthesis of CF3-substituted allenyl nitriles by ligand-controlled radical 1,2- and 1,4-addition to 1,3-enynes, Angew. Chem., Int. Ed., 2018, 57, 7140–7145 CrossRef CAS.
- Y. Song, S. Song, X. Duan, X. Wu, F. Jiang, Y. Zhang, J. Fan, X. Huang, C. Fu and S. Ma, Copper-catalyzed radical approach to allenyl iodides, Chem. Commun., 2019, 55, 11774–11777 RSC.
-
(a) J. M. Lee, Y. Na, H. Han and S. Chang, Cooperative multi-catalyst systems for one-pot organic transformations, Chem. Soc. Rev., 2004, 33, 302–312 RSC;
(b) J. Fu, X. Huo, B. Li and W. Zhang, Cooperative bimetallic catalysis in asymmetric allylic substitution, Org. Biomol. Chem., 2017, 15, 9747–9759 RSC;
(c) G. R. Gupta, J. Shah, K. S. Vadagaonkar, A. G. Lavekar and A. R. Kapdi, Hetero-bimetallic cooperative catalysis for the synthesis of heteroarenes, Org. Biomol. Chem., 2019, 17, 7596–7631 RSC.
- E. Gayon, O. Quinonero, S. Lemouzy, E. Vrancken and J.-M. Campagne, Transition-metal-catalyzed uninterrupted four-step sequence to access trisubstituted isoxazoles, Org. Lett., 2011, 13, 6418–6421 CrossRef CAS PubMed.
- For base-promoted examples, see:
(a) X. Zhou, X. Xu, Z. Shi, K. Liu, H. Gao and W. Li, Enolate-mediated 1,3-dipolar cycloaddition reaction of β-functionalized ketones with nitrile oxides: direct access to 3,4,5-trisubstituted isoxazoles, Org. Biomol. Chem., 2016, 14, 5246–5250 RSC;
(b) F.-T. Gao, Z. Fang, R.-R. Su, P.-X. Rui and X.-G. Hu, Hydroximoyl fluorides as the precursors of nitrile oxides: synthesis, stability and [3 + 2]-cycloaddition with alkynes, Org. Biomol. Chem., 2018, 16, 9211–9217 RSC;
(c) L.-E. Carloni, S. Mohnani and D. Bonifazi, Synthesis of 3,5-disubstituted isoxazoles through a 1,3-dipolar cycloaddition reaction between alkynes and nitrile oxides generated from O-silylated hydroxamic acids, Eur. J. Org. Chem., 2019, 7322–7334 CrossRef CAS. For radical cross-couplings:
(d) D. Wang, F. Zhang, F. Xiao and G.-J. Deng, A three-component approach to isoxazolines and isoxazoles under metal-free conditions, Org. Biomol. Chem., 2019, 17, 9163–9168 RSC;
(e) Y. He, Y.-Y. Xie, Y.-C. Wang, X.-M. Bin, D.-C. Hu, H.-S. Wang and Y.-M. Pan, TEMPO-catalyzed synthesis of 5-substituted isoxazoles from propargylic ketones and TMSN3, RSC Adv., 2016, 6, 58988–58993 RSC. For water-assisted example:
(f) C. Kesornpun, T. Aree, C. Mahidol, S. Ruchirawat and P. Kittakoop, Water-assisted nitrile oxide cycloadditions: synthesis of isoxazoles and stereoselective syntheses of isoxazolines and 1,2,4-oxadiazoles, Angew. Chem., Int. Ed., 2016, 55, 3997–4001 CrossRef CAS PubMed. For I2-catalyzed example:
(g) S. U. Dighe, S. Mukhopadhyay, S. Kolle, S. Kanojiya and S. Batra, Synthesis of 3,4,5-trisubstitutedIsoxazoles from Morita-Baylis-Hillman acetates by an NaNO2/I2-mediated domino reaction, Angew. Chem., Int. Ed., 2015, 54, 10926–10930 CrossRef CAS PubMed. For N-Heterocyclic carbene-catalyzed example:
(h) S. Kankala, R. Vadde and C. S. Vasam, N-Heterocyclic carbene-catalyzed 1,3-dipolar cycloaddition reactions: a facile synthesis of 3,5-di- and 3,4,5-trisubstituted isoxazoles, Org. Biomol. Chem., 2011, 9, 7869–7876 RSC.
|
This journal is © the Partner Organisations 2020 |
Click here to see how this site uses Cookies. View our privacy policy here.