A highly specific and ultrasensitive fluorescent probe for basal lysosomal HOCl detection based on chlorination induced by chlorinium ions (Cl+)†
Received
24th December 2016
, Accepted 3rd April 2017
First published on 3rd April 2017
Abstract
The development of techniques for detecting HOCl at the subcellular level is very important to elucidate its cellular functions. Due to its relatively low concentration, it is still a great challenge to specifically track the basal HOCl in normal cells. In this paper, based on the unique chlorination of HOCl by the initiation of chlorinium ions (Cl+) in an acidic medium, we have developed a simple pH-mediated lysosome-targetable fluorescent probe Lyso-HOCl for the specific detection of HOCl over other bioactive molecules at higher concentration (500 μM). Our results show that Lyso-HOCl possesses a detection limit of 8.0 pM, and can quantitatively detect HOCl at the picomolar level. The ultrasensitive and ultrafast response property of probe Lyso-HOCl offers a good opportunity to monitor the basal HOCl and the fluctuation of endogenous HOCl levels in the lysosomes of macrophages (Raw 264.7 cells), and we thus anticipate that this probe would provide a promising tool for further unraveling the biological functions of HOCl in subcellular lysosomes.
Introduction
Reactive oxygen species (ROS) play a variety of roles in biological processes including signal transduction, inflammation, carcinogenesis, and neurodegenerative injury.1–3 Hypochlorous acid (HOCl), an important ROS, is endogenously generated from hydrogen peroxide (H2O2) and chloride ions (Cl−) by the catalysis of myeloperoxidase (MPO) in living systems, and plays crucial roles in the immune defense against invasive bacteria and other pathogens despite its relatively low concentration.4,5 However, a large number of investigations have shown that excessive HOCl is involved in various diseases, such as inflammatory diseases, cardiovascular diseases, nephropathies, acute lung injuries, cystic fibrosis, neurodegenerative disorders, and even cancers.6–8 On the other hand, recent evidence has demonstrated that lysosomal functions are subtly regulated by ROS including HOCl.9,10 Moreover, lysosomes are one of the most significant sources of HOCl, especially in macrophages.11,12 Therefore, the determination of basal lysosomal HOCl is of great significance for unraveling its physiological and pathological processes.
Fluorescence imaging with the help of fluorescent probes makes it easier to understand the distribution of biologically-relevant species in living systems, even in subcellular organelles including mitochondria and lysosomes.13–16 Recently, many excellent fluorescent probes have been developed to track bioactive molecules for understanding their biological functions.17–27 Although several efforts have been made to exploit fluorescent probes for HOCl, all of them can only detect increased HOCl concentrations by external stimulation owing to their unsatisfactory sensitivity and the very low actual concentrations of HOCl in normal cells.28–38 Thus, it is still a great challenge to specifically monitor the basal intracellular HOCl level without exogenous stimulation. Moreover, only a few lysosome-targetable small molecular fluorescent probes for HOCl have been developed.12,39,40 All of them possess poor water-solubility (30–50% organic solvent as co-solvent) and are composed of the morpholine moiety as the guiding unit.12,39,40 However, the morpholine group exhibits an “alkalizing effect” on the lysosomes so that longer incubation times with such probes can cause an increase in lysosomal pH and induce cell apoptosis.41–44 Moreover, an ideal fluorescent probe for HOCl should exhibit a fast response towards HOCl because of its short lifetime, and be facilely prepared in high yield.
Based on the above-mentioned considerations, we have developed a simple pH-mediated lysosome-targetable fluorescent probe Lyso-HOCl for the determination of HOCl in a completely aqueous solution (Scheme 1). We speculated that probe Lyso-HOCl containing a pH-responsive phenol moiety would target the lysosomes, and that its similar structure to rhodamine would allow the specific detection of HOCl based on its unique chlorination induced by chlorinium ions (Cl+) in an acidic medium. Probe Lyso-HOCl was easily synthesized using commercially available 1,6-dihydroxynaphthalene and 2-(4-diethylamino-2-hydroxybenzoyl) benzoic acid in high yield (93.2%). The results demonstrated that probe Lyso-HOCl exhibits excellent selectivity towards HOCl over other bioactive molecules. Additionally, Lyso-HOCl possesses a detection limit of 8.0 pM, and can quantitatively detect HOCl at the picomolar level. More importantly, Lyso-HOCl with pH-responsive properties was successfully applied for the imaging of basal HOCl and the fluctuation of endogenous/exogenous HOCl levels in the lysosomes of macrophages (Raw 264.7 cells).
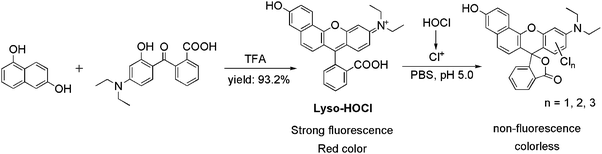 |
| Scheme 1 The synthesis and recognition mechanism of probe Lyso-HOCl for HOCl. | |
Results and discussion
Design and synthesis of Lyso-HOCl
Lysosomes are substantially more acidic (pH 4.5–5.5) than other subcellular organelles of cells.45,46 This property can be employed as an endogenous stimulus for the activation of recognition properties and fluorescence signals.43,46,47 Thus, we reasoned that a pH-responsive fluorescent probe could be competent for targeting the lysosomes of cells. Simultaneously, to construct an excellent fluorescent probe for specific detection of HOCl, it is of great significance to find an appropriate recognition receptor.28 Excitingly, previous studies have proven that HOCl can rapidly chlorinate xanthene dyes.33,48–50 This feature of HOCl is quite unique among other ROS and reactive nitrogen species (RNS). Therefore, we envisioned that a pH-responsive fluorescent dye containing the xanthene moiety might be an ideal probe for the specific determination of lysosomal HOCl. It is reported that seminaphthorhodafluor dye containing a similar xanthene moiety is especially useful as a pH indicator because of its excellent photophysical properties including high quantum yield, visible-light excitation, dual-emission, and big molar absorptivity.51–54 Herein, we synthesized a seminaphthorhodafluor dye as a fluorescent probe for HOCl (Lyso-HOCl). Probe Lyso-HOCl was easily prepared from 1,6-dihydroxynaphthalene and 2-(4-diethylamino-2-hydroxybenzoyl) benzoic acid in trifluoroacetic acid (TFA) as a solvent (yield: 93.2%), which was superior to the reported methods using ZnCl2 as a catalyst at 160 °C.55
Properties of fluorescent probe Lyso-HOCl for HOCl
The photophysical properties of Lyso-HOCl were investigated in a completely aqueous solution because of its outstanding water-solubility. In the absence of HOCl, the probe solution exhibits a very strong fluorescence emission peak at 587 nm (Φ = 0.032) with excitation at 520 nm under the simulated physiological conditions in the lysosomes (PBS, pH = 5.0) (Fig. S1, ESI†). Upon addition of 5 μM (1 equiv.) HOCl, a rapid fluorescence decrease at 587 nm (almost non-fluorescence) was observed, and the instant response time (<5 s) makes it convenient to monitor HOCl with a short lifetime in living systems. Meanwhile, it's worth mentioning that this phenomenon of great fluorescence quenching might be ascribed to the formation of a spirolactone56,57 structure containing a similar rhodamine moiety induced by chlorination based on the highly reactive chlorinating agent, chlorinium ions (Cl+), produced from the decomposition of HOCl in the acidic solution,58,59 which is different from the chlorination of fluorescein dyes by HOCl.37,48,49 To disclose the recognition mechanism of Lyso-HOCl for HOCl, a weak basic solution (pH = 7.4) was chosen as the contrastive solution (Fig. 1, detailed information about the effects of different pH values is shown in Fig. S2, ESI†). Lyso-HOCl showed negligible fluorescence changes in the presence of HOCl (pKa = 7.6 for HOCl)30 under basic conditions, which is in good agreement with the conclusions reported by Zhao et al.56,60,61 This feature would be beneficial for tracking specifically the HOCl level in the lysosomes of cells.43 The possible reason is that HOCl can more easily decompose into Cl+ under acidic conditions than under basic conditions, which indirectly demonstrated that the chlorination of HOCl is attributed to the initiation of Cl+.62,63 To further confirm the sensing mechanism of Lyso-HOCl for HOCl, the reaction of Lyso-HOCl with HOCl was conducted under the same conditions as described above. The reaction products were subjected to electrospray ionization mass spectral analysis. Peaks corresponding to monochloride, dichloride, and trichloride were observed in the high resolution mass spectrum (HRMS) (Fig. S2, ESI†). Thus, in agreement with previous reports,56–63 probe Lyso-HOCl most likely undergoes the proposed mechanism as shown in Scheme 1.
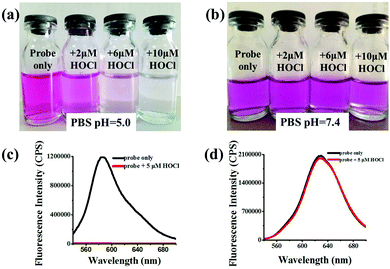 |
| Fig. 1 (a) The color changes of Lyso-HOCl (20 μM) in the presence of different concentrations of HOCl in a completely aqueous solution containing PBS (10 mM, pH = 5.0). (b) The color changes of Lyso-HOCl (20 μM) in the presence of different concentrations of HOCl in a completely aqueous solution containing PBS (10 mM, pH = 7.4). (c) The fluorescence changes of Lyso-HOCl (5 μM) in the absence and presence of HOCl (5 μM) in a completely aqueous solution containing PBS (10 mM, pH = 5.0). (d) The fluorescence changes of Lyso-HOCl (5 μM) in the absence and presence of HOCl (5 μM) in a completely aqueous solution containing PBS (10 mM, pH = 7.4). | |
In the absorption spectra, the free probe solution shows a strong hunchbacked absorption band (absorption peaks around 515 nm and 550 nm). When 5 μM (1 equiv.) HOCl was added to the solution of Lyso-HOCl, the above-mentioned absorption band disappeared (Fig. S3, ESI†) and the color of the solution turned from red to colorless (Fig. 1a). Therefore, our proposed probe Lyso-HOCl has another powerful superiority that HOCl detection was visible to the naked eye, which would be beneficial for further applications in vitro.
Determination of HOCl
Then, the quantitative analysis of HOCl with the aid of probe Lyso-HOCl was studied in detail. Continuous addition of HOCl to a solution of Lyso-HOCl resulted in a gradual decrease of the fluorescence band centered at around 587 nm. More importantly, a linear relationship between the fluorescence intensity at 587 nm and HOCl concentrations in the range of 300 to 1800 pM were obtained (Fig. 2). Therefore, Lyso-HOCl possesses excellent sensitivity and can quantitatively detect HOCl at the picomolar level.28,64–66 The detection limit (based on 3σ/k, where σ is the standard deviation of five blank measurements, and k is the slope of the linear equation) was determined to be as low as 8 pM, which makes the probe available for monitoring the basal HOCl level in the lysosomes of cells (Fig. 2). The ultrasensitivity of Lyso-HOCl for HOCl was ascribable to the lower cyclization energy barrier of the probe by the introduction of the naphthol moiety57 and the production of highly reactive chlorinating agent Cl+ in the acidic solution.
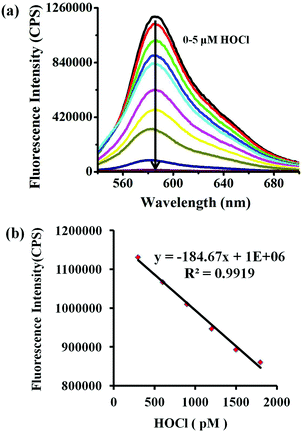 |
| Fig. 2 (a) The fluorescence spectra of the probe (5 μM) with the addition of increasing concentrations of HOCl (0–5 μM) in a completely aqueous solution containing PBS (10 mM, pH = 5.0). (b) A plot of fluorescence intensity at 587 nm vs. increasing concentrations of HOCl (300–1800 pM). The excitation wavelength was 520 nm, and the excitation and emission slit widths were 3 nm. Each spectrum was acquired 1 min after HOCl addition at 25 °C. | |
Selectivity of Lyso-HOCl for HOCl
We then examined the specificity of probe Lyso-HOCl towards HOCl under the same analytical conditions as previously noted. As shown in Fig. 3, nearly no fluorescence spectra changes were observed in the presence of other biologically-relevant species including K+, Ca2+, Mg2+, Na+, Zn2+, Al3+, NO3−, NO2−, Cu2+, Fe3+, cysteine (Cys), H2O2, tert-butyl hydroperoxide (TBHP), hydroxyl radical (˙OH), tert-butoxy radical (˙OtBu), NO, Cl−, ClO2−, ClO3−, ClO4−, glutathione (GSH, 5 mM), 1O2, O2−, and ONOO− at a higher concentration (500 μM), which fully demonstrated that Lyso-HOCl possessed excellent selectivity. The high specificity of Lyso-HOCl for HOCl was ascribed to the unique chlorination of HOCl among other various species.
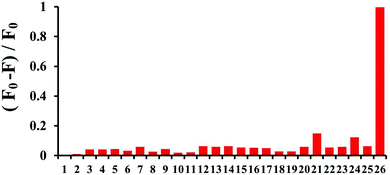 |
| Fig. 3 The fluorescence response of Lyso-HOCl (5 μM) in the presence of different analytes (500 μM except for the specific labels) in a completely aqueous solution containing PBS (10 mM, pH = 5.0). (1) Blank; (2) K+; (3) Ca2+; (4) Mg2+; (5) Na+; (6) Zn2+; (7) Al3+; (8) NO3−; (9) NO2−; (10) Cu2+; (11) Fe3+; (12) Cys; (13) H2O2; (14) TBHP; (15) ˙OH; (16) ˙OtBu; (17) NO; (18) Cl−; (19) ClO2−; (20) ClO3−; (21) ClO4−; (22) GSH (5 mM); (23) 1O2; (24) O2−; (25) ONOO−; (26) HOCl (5 μM). The excitation wavelength was 520 nm, and the excitation and emission slit widths were 3 nm. The bars represent the fluorescence intensities at 587 nm and each spectrum was acquired 1 min after HOCl addition at 25 °C. | |
Imaging applications of Lyso-HOCl
Prior to the imaging experiments, the cytotoxicity of Lyso-HOCl was evaluated through 3-(4,5-dimethylthiazol-2-yl)-2,5-diphenyltetrazolium bromide (MTT) assays in Raw 264.7 macrophage cells. The results revealed that Lyso-HOCl exhibited almost no toxicity or low toxicity (Fig. S4, ESI†). The intracellular location of Lyso-HOCl inside cells was examined through co-incubation with Lyso-HOCl and Lyso-Tracker Blue (a commercially available lysosomal marker) in live Raw 264.7 macrophage cells (Fig. 4). As shown in Fig. 4c, the images of the green and blue channels merged well. The changes in the fluorescence intensity profiles of the linear regions of interest were closely synchronous (Fig. 4e). Moreover, the intensity scatter plot of the green and blue channels exhibited a close correlation with a high overlap coefficient of 0.91 (Fig. 4f). These results demonstrated that Lyso-HOCl was specifically located in the lysosomes of live Raw 264.7 macrophage cells.
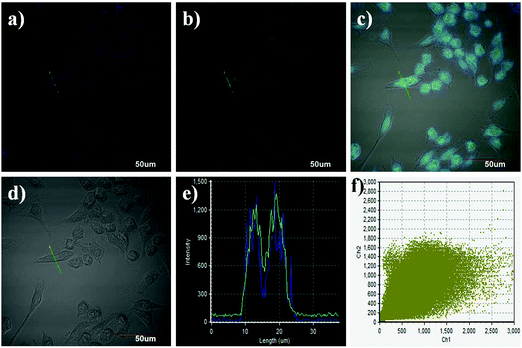 |
| Fig. 4 Confocal fluorescence images of live Raw 264.7 macrophage cells co-incubated with Lyso-HOCl (10 μM) and Lyso-Tracker Blue (100.0 nM) for 20 min. (a) The blue channel for the Lyso-Tracker fluorescence; (b) the green channel for the probe fluorescence; (c) merged image of (a), (b) and (d); (d) bright-field image; (e) fluorescence intensity profiles of the region of interest (ROI) across the cells co-stained with Lyso-HOCl and Lyso-Tracker Blue; (f) intensity scatter plot of green and blue channels. Incubation was performed at 37 °C under a humidified atmosphere containing 5% CO2. The scale bar is 50 μm. | |
Subsequently, Lyso-HOCl was used to monitor HOCl in live Raw 264.7 macrophage cells. As shown in Fig. 5a (for detailed data of Fig. 5, see Fig. S5 in the ESI†), the control Raw 264.7 macrophage cells showed a medium intracellular fluorescence. As expected, the Raw 264.7 macrophages cells pre-treated with N-acetylcysteine (NAC; a scavenger of HOCl)67 exhibited a stronger fluorescence (Fig. 5b), implying that Lyso-HOCl was sensitive enough to detect the basal HOCl level in Raw 264.7 macrophages cells without external stimulation. Additionally, the Raw 264.7 macrophages cells pre-treated with phorbol 12-myristate 13-acetate (PMA, a ROS stimulant that induces the production of HOCl)68 revealed a weaker fluorescence (Fig. 5c). These results suggest that Lyso-HOCl is capable of tracking the basal HOCl levels and the fluctuation of endogenous HOCl levels in live Raw 264.7 macrophage cells, which is of significance for exploring the physiological and pathological functions of HOCl. It is especially notable that Lyso-HOCl is the first fluorescent probe for imaging the basal HOCl level in normal cells. Finally, Lyso-HOCl was also successfully applied to monitor the changes in HOCl levels in the presence of exogenous HOCl in live Raw 264.7 macrophage cells (Fig. S6, ESI†).
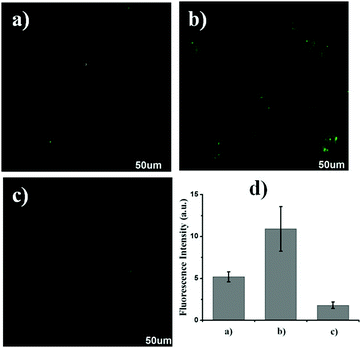 |
| Fig. 5 Confocal fluorescence images of live Raw 264.7 macrophages cells: (a) Raw 264.7 macrophages cells incubated with Lyso-HOCl (10 μM) for 20 min; (b) Raw 264.7 macrophages cells incubated with Lyso-HOCl (10 μM) for another 20 min after pre-incubation with 200 μM NAC for 60 min; (c) Raw 264.7 macrophages cells incubated with Lyso-HOCl (10 μM) for another 20 min after pre-incubation with 1.0 μg mL−1 PMA for 60 min; (d) relative fluorescence intensity of cells in panels (a)–(c). The provided images of Raw 264.7 macrophage cells are representative ones (n = 10 fields of cells). Incubation was performed at 37 °C under a humidified atmosphere containing 5% CO2. The scale bar is 50 μm. | |
Experimental section
Materials and general methods
All the chemicals used in this paper were obtained from commercial suppliers and used without further purification. Ultrapure water was prepared through a Sartorius Arium 611DI system and used throughout the experiment. 1H NMR and 13C NMR spectra were recorded on a Bruker AV-400 spectrometer with chemical shifts reported in ppm (in DMSO-d6, TMS as internal standard). Fluorescence emission spectra were measured on a Horiba FluoroMax-4 spectrophotometer. The fluorescence images of Raw 264.7 macrophage cells were observed under an Olympus FV1000-IX81 confocal fluorescence microscope, and the excitation wavelength was 488 nm. All the fluorescence spectra were uncorrected. All pH measurements were performed using a Sartorius basic pH-meter PB-10.
Synthesis of probe Lyso-HOCl
1,6-Dihydroxynaphthalene (3.2034 g, 20 mmol) and 2-(4-diethylamino-2-hydroxybenzoyl) benzoic acid (6.2670 g, 20 mmol) were dissolved in 15 mL trifluoroacetic acid (TFA). The mixture was refluxed and stirred for 24 hours. After the removal of TFA, the pure probe Lyso-HOCl was obtained as a dark-red powder by recrystallization from ethyl acetate (7.6
200 g, 93.2%). 1H-NMR (400 MHz, DMSO-d6) δ (×10−6): 1.203(t, J = 6.8 Hz, 6H), 3.570 (s, 4H), 6.794–7.125 (m, 4H), 7.258 (s, 1H), 7.342–7.414 (m, 2H), 7.540 (d, J = 8.4 Hz, 1H), 7.779–7.875 (m, 2H), 8.168 (d, J = 7.2 Hz, 1H), 8.636 (d, J = 8.4 Hz, 1H), 10.659 (s, 1H). 13C-NMR (100 MHz, DMSO-d6) δ (×10−6): 12.94, 45.30, 97.37, 110.55, 115.01, 116.88, 117.93, 120.02, 124.08, 125.32, 130.39, 130.79, 134.80, 158.11, 158.46, 158.81, 159.16, 168.14. ESI-MS calcd for C26H20NO4 [M+H]+ 438.1700, found 438.1702.
Conclusions
In conclusion, we have developed a simple pH-mediated lysosome-targetable fluorescent probe Lyso-HOCl for HOCl. Lyso-HOCl exhibits preeminent selectivity toward HOCl over other biologically-relevant species, which might be ascribed to the unique chlorination of HOCl via the initiation of Cl+. Moreover, Lyso-HOCl can quantitatively detect HOCl in the range of 300 to 1800 pM with a detection limit of 8 pM. Experiments on bioimaging applications demonstrate that Lyso-HOCl can specifically locate in the lysosomes of live Raw 264.7 macrophage cells and monitor the basal intracellular HOCl level without exogenous stimulation. Moreover, its ability to track the changes in HOCl levels in the presence of exogenous or endogenous HOCl in live cells was further demonstrated. More importantly, this proposed recognition mechanism will open up new exciting opportunities for designing highly selective and ultrasensitive fluorescent probes for HOCl. The present study offers an easy-to-synthesize specific fluorescent probe for the detection of HOCl at the picomolar level and an excellent tool for exploring the physiological and pathological functions of basal lysosomal HOCl in live cells at a lower concentration. Further endeavors are being made to develop ratiometric lysosomal fluorescent probes based on the proposed recognition mechanism in our laboratory.
Acknowledgements
We gratefully acknowledge financial support from the National Natural Science Foundation of China (21607053, 21107029, and 21275018), Shandong Province Key Research and Development Project (2016GSF117010), Postdoctoral Science Foundation of China (2013M541953, 2016M592127), Outstanding Young Scientists Award Fund of Shandong Province (BS2013HZ007), Shandong Provincial Natural Science Foundation (ZR2014DM011), Shandong Provincial Key Research Project (2015GSF117025), Beijing National Laboratory for Molecular Sciences (BNLMS) (20140121), Research Fund for the Doctoral Program of Higher Education of China (RFDP) (20121101110049) and the 111 Project (B07012).
Notes and references
- X. Chen, F. Wang, J. Y. Hyun, T. Wei, J. Qiang, X. Ren, I. Shin and J. Yoon, Chem. Soc. Rev., 2016, 45, 2976–3016 RSC.
- X. Chen, X. Tian, I. Shin and J. Yoon, Chem. Soc. Rev., 2011, 40, 4783–4804 RSC.
- C. Nathan, J. Clin. Invest., 2003, 111, 769–778 CrossRef CAS PubMed.
- Z. M. Prokopowicz, F. Arce, R. Biedron, C. L. Chiang, M. Ciszek, D. R. Katz, M. Nowakowska, S. Zapotoczny, J. Marcinkiewicz and B. M. J. Chain, J. Immunol., 2010, 184, 824–835 CrossRef CAS PubMed.
- J. Shepherd, S. A. Hilderbrand, P. Waterman, J. W. Heinecke, R. Weissleder and P. Libby, Chem. Biol., 2007, 14, 1221–1231 CrossRef CAS PubMed.
- I. P. David and J. D. Michael, Chem. Res. Toxicol., 2001, 14, 1453–1464 CrossRef.
- I. P. David and J. D. Michael, Biochemistry, 2006, 45, 8152–8162 CrossRef PubMed.
- Y. W. Yap, M. Whiteman and N. S. Cheung, Cell. Signalling, 2007, 19, 219–228 CrossRef CAS PubMed.
- D. J. Klionsky and S. D. Emr, Science, 2000, 290, 1717–1721 CrossRef CAS PubMed.
- T. Shintani and D. J. Klionsky, Science, 2004, 306, 990–995 CrossRef CAS PubMed.
- S. J. Klebanoff, J. Leukocyte Biol., 2005, 77, 598–625 CrossRef CAS PubMed.
- L. Yuan, L. Wang, B. K. Agrawalla, S. J. Park, H. Zhu, B. Sivaraman, J. Peng, Q. H. Xu and Y. T. Chang, J. Am. Chem. Soc., 2015, 137, 5930–5938 CrossRef CAS PubMed.
- W. Sun, S. Guo, C. Hu, J. Fan and X. Peng, Chem. Rev., 2016, 116, 7768–7817 CrossRef CAS PubMed.
- H. Zhu, J. Fan, B. Wang and X. Peng, Chem. Soc. Rev., 2015, 44, 4337–4366 RSC.
- X. Li, X. Gao, W. Shi and H. Ma, Chem. Rev., 2014, 114, 590–659 CrossRef CAS PubMed.
- H. Zhu, J. Fan, J. Du and X. Peng, Acc. Chem. Res., 2016, 49, 2115–2126 CrossRef CAS PubMed.
- J. Chao, Z. Li, Y. Zhang, F. Huo, C. Yin, Y. Liu, Y. Li and J. Wang, J. Mater. Chem. B, 2016, 4, 3703–3712 RSC.
- B. Liu, X. Hu, J. Chai, J. Zhu, B. Yang and Y. Li, J. Mater. Chem. B, 2016, 4, 3358–3364 RSC.
- W. Chen, C. Liu, B. Peng, Y. Zhao, A. Pacheco and M. Xian, Chem. Sci., 2013, 4, 2892–2896 RSC.
- P. Jin, C. Jiao, Z. Guo, Y. He, S. Zhu, H. Tian and W. Zhu, Chem. Sci., 2014, 5, 4012–4016 RSC.
- H. Xiao, P. Li, X. Hu, X. Shi, W. Zhang and B. Tang, Chem. Sci., 2016, 7, 6153–6159 RSC.
- Y. Gong, X. Zhang, G. Mao, L. Su, H. Meng, W. Tan, S. Feng and G. Zhang, Chem. Sci., 2016, 7, 2275–2285 RSC.
- W. Chen, E. W. Rosser, T. Matsunaga, A. Pacheco, T. Akaike and M. Xian, Angew. Chem., Int. Ed., 2015, 54, 13961–13965 CrossRef CAS PubMed.
- Y. Tang, X. Kong, A. Xu, B. Dong and W. Lin, Angew. Chem., Int. Ed., 2016, 55, 3356–3359 CrossRef CAS PubMed.
- W. Chen, A. Pacheco, Y. Takano, J. J. Day, K. Hanaoka and M. Xian, Angew. Chem., Int. Ed., 2016, 55, 9993–9996 CrossRef CAS PubMed.
- K. Xu, D. Luan, X. Wang, B. Hu, X. Liu, F. Kong and B. Tang, Angew. Chem., Int. Ed., 2016, 55, 12751–12754 CrossRef CAS PubMed.
- W. Zhang, P. Li, F. Yang, X. Hu, C. Sun, W. Zhang, D. Chen and B. Tang, J. Am. Chem. Soc., 2013, 135, 14956–14959 CrossRef CAS PubMed.
- Y. Yue, F. Huo, C. Yin, J. O. Escobedo and R. M. Strongin, Analyst, 2016, 141, 1859–1873 RSC.
- Q. Xu, C. H. Heo, G. Kim, H. W. Lee, H. M. Kim and J. Yoon, Angew. Chem., Int. Ed., 2015, 54, 4890–4894 CrossRef CAS PubMed.
- Y. Koide, Y. Urano, K. Hanaoka, T. Terai and T. Nagano, J. Am. Chem. Soc., 2011, 133, 5680–5682 CrossRef CAS PubMed.
- H. Zhu, J. Fan, J. Wang, H. Mu and X. Peng, J. Am. Chem. Soc., 2014, 136, 12820–12823 CrossRef CAS PubMed.
- J. Zhou, L. H. Li, W. Shi, X. H. Gao, X. H. Li and H. M. Ma, Chem. Sci., 2015, 6, 4884–4888 RSC.
- Q. Xu, K.-A. Lee, S. Lee, K. M. Lee, W.-J. Lee and J. Yoon, J. Am. Chem. Soc., 2013, 135, 9944–9949 CrossRef CAS PubMed.
- G. Cheng, J. Fan, W. Sun, J. Cao, C. Hu and X. Peng, Chem. Commun., 2014, 50, 1018–1020 RSC.
- J. J. Hu, N. K. Wong, Q. Gu, X. Bai, S. Ye and D. Yang, Org. Lett., 2014, 16, 3544–3547 CrossRef CAS PubMed.
- W. Zhang, W. Liu, P. Li, J. kang, J. Wang, H. Wang and B. Tang, Chem. Commun., 2015, 51, 10150–10152 RSC.
- J. J. Hu, N. Wong, M. Lu, X. Chen, S. Ye, A. Q. Zhao, P. Gao, R. Y. Kao, J. Shen and D. Yang, Chem. Sci., 2016, 7, 2094–2099 RSC.
- Q. Xu, C. H. Heo, J. A. Kim, H. S. Lee, Y. Hu, D. Kim, K. M. K. Swamy, G. Kim, S. Nam, H. M. Kim and J. Yoon, Anal. Chem., 2016, 88, 6615–6620 CrossRef CAS PubMed.
- Z. Qu, J. Ding, M. Zhao and P. Li, J. Photochem. Photobiol., A, 2015, 299, 1–8 CrossRef CAS.
- M. Ren, B. Deng, K. Zhou, X. Kong, J. Wang, G. Xu and W. Lin, J. Mater. Chem. B, 2016, 4, 4739–4745 RSC.
-
I. Johnson and M. T. Z. Spence, The Molecular Probes Handbook-A Guide to Fluorescent Probes and Labeling Technologies, Life Technologies Corporation, Carlsbad, 11th edn, 2010, p. 519 Search PubMed.
-
R. P. Haugland, A Guide to Fluorescent Probes and Labeling Technologies, Invitrogen Corp., The United States, 2005, pp. 580–588 Search PubMed.
- Y. Li, W. Wu, J. Yang, Y. Yuan, C. Liu, J. Zheng and R. Yang, Chem. Sci., 2016, 7, 1920–1925 RSC.
- H. Zhu, J. Fang, Q. Xu, H. Li, J. Wang, P. Gao and X. Peng, Chem. Commun., 2012, 48, 11766–11768 RSC.
- Q. Wan, S. Chen, W. Shi, L. Li and H. Ma, Angew. Chem., Int. Ed., 2014, 53, 10916–10920 CrossRef CAS PubMed.
- F. Kratz, I. A. Muller, C. Ryppa and A. Warnecke, ChemMedChem, 2008, 3, 20–53 CrossRef CAS PubMed.
- J. Liu, J. Ren, X. Bao, W. Gao, C. Wu and Y. Zhao, Anal. Chem., 2016, 88, 5865–5870 CrossRef CAS PubMed.
- J. C. Whitin, R. A. Clark, E. R. Simons and H. J. Cohen, J. Biol. Chem., 1981, 256, 8904–8906 CAS.
- J. K. Hurst, J. M. Albrich, T. R. Green, H. Rosen and S. Klebanoff, J. Biol. Chem., 1984, 259, 4812–4821 CAS.
- Q. A. Best, N. Sattenapally, D. J. Dyer, C. N. Scott and M. E. McCarroll, J. Am. Chem. Soc., 2013, 135, 13365–13370 CrossRef CAS PubMed.
- E. Nakata, Y. Nazumi, Y. Yukimachi, Y. Uto, H. Maezawa, T. Hashimoto, Y. Okamoto and H. Hori, Bioorg. Med. Chem. Lett., 2011, 21, 1663–1666 CrossRef CAS PubMed.
- J. E. Whitaker, R. P. Haugland and F. G. Prendergast, Anal. Biochem., 1991, 194, 330–344 CrossRef CAS PubMed.
- K. Honda, E. Nakata, A. Ojida and I. Hamachi, Chem. Commun., 2006, 4024–4026 RSC.
- E. Nakata, Y. Yukimachi, Y. Nazumi, Y. Uto, H. Maezawa, T. Hashimoto, Y. Okamoto and H. Hori, Chem. Commun., 2010, 46, 3526–3528 RSC.
-
R. P. Haugland and J. Whitaker, US Pat., Appl. No. 4945171, 1990 Search PubMed.
- Y.-R. Zhang, X.-P. Chen, S. Jing, J.-Y. Zhang, Q. Yuan, J.-Y. Miao and B.-X. Zhao, Chem. Commun., 2014, 50, 14241–14244 CAS.
- H. N. Kim, M. H. Lee, H. J. Kim, J. S. Kim and J. Yoon, Chem. Soc. Rev., 2008, 37, 1465–1472 RSC.
- P. Nagy and M. T. Ashby, Chem. Res. Toxicol., 2005, 18, 919–923 CrossRef CAS PubMed.
- X. L. Armesto, M. L. Canle, M. I. Fernández, M. V. García and J. A. Santaballa, Tetrahedron, 2000, 56, 1103–1109 CrossRef CAS.
- S.-L. Shen, X. Zhao, X.-F. Zhang, X.-L. Liu, H. Wang, Y.-Y. Dai, J.-Y. Miao and B.-X. Zhao, J. Mater. Chem. B, 2017, 5, 289–295 RSC.
- Z. Tang, X.-L. Ding, Y. Liu, Z.-M. Zhao and B.-X. Zhao, RSC Adv., 2015, 5, 99664–99668 RSC.
- P. B. D. de la Mare, I. C. Hilton and C. A. Vernon, J. Chem. Soc., 1960, 4039–4044 RSC.
- P. B. D. de la Mare, A. D. Ketley and C. A. Vernon, J. Chem. Soc., 1954, 1290–1297 RSC.
- Y.-R. Zhang, Y. Liu, X. Feng and B.-X. Zhao, Sens. Actuators, B, 2017, 240, 18–36 CrossRef CAS.
- B. Zhu, P. Li, W. Shu, X. Wang, C. Liu, Y. Wang, Z. Wang, Y. Wang and B. Tang, Anal. Chem., 2016, 88, 12532–12538 CrossRef CAS PubMed.
- B. Wang, J. Wen, K. Gao, H. Yan, Y. Xu, H. Li, J. Chen, W. Wang and S. Sun, RSC Adv., 2016, 6, 35315–35317 RSC.
- V. Witko-Sarsat, V. Gausson, A. T. Nguyen, M. Touam, T. Drueke, F. Santangelo and B. Descamps-Latscha, Kidney Int., 2003, 64, 82–91 CrossRef CAS PubMed.
- S. E. Gomez-Mejiba, Z. Zhai, M. S. Gimenez, M. T. Ashby, J. Chilakapati, K. Kitchin, R. P. Mason and D. C. Ramirez, J. Biol. Chem., 2010, 285, 20062–20071 CrossRef CAS PubMed.
Footnote |
† Electronic supplementary information (ESI) available. See DOI: 10.1039/c6tb03345h |
|
This journal is © The Royal Society of Chemistry 2017 |
Click here to see how this site uses Cookies. View our privacy policy here.