DOI:
10.1039/D3VA00313B
(Critical Review)
Environ. Sci.: Adv., 2024,
3, 154-176
Metal oxide-based electrochemical sensors for pesticide detection in water and food samples: a review
Received
6th October 2023
, Accepted 4th December 2023
First published on 19th December 2023
Abstract
The increasing need for food and agricultural resources necessitates using pesticides to protect plants, but this approach also poses pesticide poisoning and environmental hazards. Although designing an effective pesticide detection method is challenging, various technologies collaborate to develop an effective electrochemical sensor for detection of various pesticides. This review article examines the various metal oxides, their synthesis techniques, and their applications in electrochemical sensors, particularly for environmental applications, to detect pesticides in a variety of contaminated environmental samples. Metal oxides have unique properties that make them useful for pesticide detection because of their more active sites and electrical, optical, and semiconducting properties. Samarium molybdate-based electrode materials are considered the most promising direction for the development of electrode materials for pesticide sensors due to their economy, chemical stability, multiple valences, low detection limit, high sensitivity, and high electrocatalytic activity performance. In addition, this study investigates the current research trend in the detection of pesticides using metal oxide-based sensors in environmental samples, and researchers should expect new research perspectives and ideas. Overall, the metal oxide-based pesticide detection sensors will surely aid in meeting the growing demands for food and environmental monitoring and protection.
Environmental significance
Pesticides protect agricultural crops from insects, weeds, and illnesses. Despite this, a considerable fraction of these pesticides fail to reach their targets, resulting in unintended consequences such as soil contamination and poisoning of ground and surface water resources. This article comprehensively reviews pesticide monitoring in environmental samples using metal oxide electrocatalysts. Metal oxide-based electrochemical pesticide detection provides sensitive, selective, and rapid detection methodologies with significant environmental implications. This methodology allows for more effective monitoring, risk assessment, and the promotion of sustainable agricultural practices, all of which contribute to protecting and preserving our environment.
|
1. Introduction
The word “pesticide” is frequently used to describe several groups of fungicides, wood preservers, rodenticides, household disinfectants, and garden chemicals used to kill pests or develop resistance.1 Pesticides from various functional groups are used to grow crops worldwide. Pesticide residues are frequently found in water, air, and soil due to their ubiquitous use. Most pesticide compounds have been categorized according to their level of hazard, mode of operation, chemical composition, application timing, and application methods. The advent of chemical pesticides has significantly enhanced agricultural crop yields in recent decades.2–4 Moreover, the uncontrolled and excessive use of pesticides has resulted in food contamination and environmental, aquatic, and agricultural pollution. Vegetables, fruits, processed foods, air, soil, and water are some of the sources of pesticide residues that can enter the food chain.5 Dietary exposure to agricultural pesticides is a serious public health risk, especially in developing nations, as it can have immediate and long-term consequences on human health. Chemical pesticides can harm human health by being cytotoxic, carcinogenic, and mutagenic.6,7 The consequences of pesticides on the environment and human health are shown in Fig. 1.
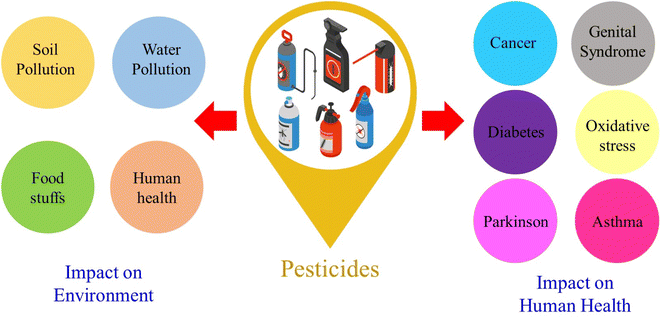 |
| Fig. 1 The schematic diagram of pesticide impacts on the environment and human health. | |
Pesticides are classified into two types: inorganic and organic. Inorganic pesticides include inorganic fungicides, arsenic insecticides, fluoride insecticides, and inorganic herbicides, whereas organonitrogen pesticides, organophosphates, and organochlorine pesticides are among the latter.8–13 Organophosphates are the most common type of plant pesticide and include chlorfenvinphos, fenitrothion, malathion, fonofos, methamidophos, monocrotophos, phosalone, methyl parathion, chlorpyriphos, diazinon, dichlorvos, and demeton-S-methyl.14–25 Organophosphate pesticides control pests in orchards, vegetables, cultivation, and industrial plantations. These pesticides are made up of any organic compound that contains phosphorus. Organophosphate pesticides cause hyperarousal conditions and muscle paralysis.26 Along with organophosphate pesticides, organonitrogen pesticides, which include carbamates, phenyl urea, triazines, and their derivatives such as propoxur, aminocarb, simazine, carbaryl, propazine, and atrazine, are important pesticides.27–33 Organonitrogen pesticides are viewed as less ecologically stable than organophosphate pesticides, but when they enter the digestive system, they pose a risk to human health.34 Various commercial methods have been developed to detect pesticides, such as fluorescence analysis,35 liquid chromatography,36 mass spectrophotometry,37 chromatography,38 high-performance liquid chromatography,39 gas chromatography,40etc. For instance, Sahoo et al.41 demonstrated pesticide nano sensing using zinc oxide quantum dots, employing optical and electrochemical techniques for detecting water samples. Amde et al.42 also introduced a nanofluid containing zinc oxide nanoparticles in an ionic liquid. This allows for single-drop liquid microextraction of fungicides from environmental waters prior to high-performance liquid chromatographic analysis. Despite the high accuracy and sensitivity of these methods, uneconomical and more detection time and advanced instruments are not suitable for rapid and real-time analysis in practical applications. On the other hand, electrochemical sensors have become an alternative method for detecting pesticides due to their low cost, sensitivity, rapid response, and easy miniaturization.43,44 Umamaheswari et al.45 successfully developed a smartphone-based portable sensor for mesotrione that is appropriate for use in nonlaboratory environments. Future commercialization of this technology would make it possible to use it in real-time monitoring applications. Furthermore, researchers have enhanced these desirable characteristics by introducing suitable electrocatalysts through electrode modification.
As previously stated, the electrocatalyst has a significant effect on electrochemical performance. Hence, researchers are interested in developing a novel material with improved physicochemical properties for various fields. Metal oxides play a key role in electrochemical applications due to their porous nature, which leads to a high surface area, more active sites, and electrical, optical, and semiconducting properties.46–50 The chemical flexibility and crystal structural properties of metal oxide nanomaterials pave the way for various applications such as energy storage, optoelectronics, sensors, infrared detectors, and other technologies.51–56 Transition metals have piqued the interest of researchers because they typically have multiple valence states and can easily form a redox cycle between high and low oxidation states.57,58 Their mixed oxidation states and enriched performances benefit energy storage, photoelectrochemical conversion, and other technologies. For example, George et al.59 developed a review of metal oxide-based electrochemical detection of chemical and biochemical species in biological samples. Hua et al.60 discussed heavy metal removal from wastewater using nanosized metal oxides for review. Thus, the size of the particles also reveals the unique and outstanding properties of metal oxides. According to previous literature, sensors utilizing metal oxide materials have been proposed for detecting heavy metal ions, and chemical and biochemical compounds in environmental and biological samples.61,62 For instance, some literature studies have reported on detecting pesticides with different metal oxide-modified electrodes, as shown in Table 1. Because of the physiochemical characteristics, the metal oxide-modified electrodes largely boost the sensitivity and reproducibility of the electrochemical sensor. In the realm of electrochemical sensors, metal oxide-based materials can be used. To the best of the authors' knowledge, no review papers have been published on the various metal oxide (neodymium molybdate, samarium molybdate, bismuth vanadate, dysprosium vanadate, lanthanum stannate, iron oxide) based electrochemical detection of pesticides (methyl parathion, carbendazim, paraoxon, fenitrothion, 2,4,6-trichlorophenol, carbofuran) in water and food samples.
Table 1 Some literature studies reported on the detection of pesticides with different nanomaterial-modified electrodes
Materials |
Methods |
Linear range (μM) |
LOD (μM) |
Pesticides |
Reference |
MnCo2O4 |
DPV |
0.015–435 |
0.002 |
Paraoxon-ethyl |
174
|
PrCoO3 |
DPV |
0.001–84 |
0.002 |
Carbendazim |
175
|
Graphene oxide |
i–t |
1–47 |
1.2 |
Fenuron |
176
|
AgWO4 |
DPV |
1–1108 |
0.066 |
Crisquat |
177
|
SrTiO3 |
DPV |
0.01–434 |
0.003 |
Trichlorophenol |
155
|
rGO/Cu/CuO |
DPV |
0.05–20 |
0.005 |
Carbaryl |
178
|
CuO |
CV |
0.01–30 |
0.003 |
Fenamiphos |
178
|
TiO2 |
DPV |
5–150 |
0.02 |
2,4-Dichlorophenol |
179
|
Fe3O4 |
CV |
0.05–100 |
0.02 |
Chlorpyrifos |
180
|
Bi2Sn2O7 |
CV |
0.05–500 |
0.014 |
Omethoate |
181
|
Pr2(WO4)3 |
i–t |
0.01–313 |
0.005 |
Fenitrothion |
182
|
This review discusses a variety of electrochemical (bio)sensing analyses for highly sensitive pesticide detection. Different nanomaterials have been used to amplify signals from electrochemical analysis techniques. The current review focuses on using metal oxides to develop various (bio)sensing approaches. Metal oxides produce highly successful results when used in electrochemical sensing because they are biocompatible and can detect biological activity during absorption. The authors define metal oxides, their preparation methods, characteristics, and structures in this review. Finally, this study will discuss various electrode material-based electrochemical sensors for pesticide detection and electrode materials that are most efficient for pesticide detection in water and food samples. Moreover, the potential challenges and prospects for pesticide detection are investigated.
2. Metal oxides
Metal oxides can be categorized into three classes based on their chemical properties and structural characteristics. These three main categories are acidic, basic, and amphoteric oxides.63 Metal oxides are compounds consisting of metal-bound oxygen atoms exhibiting admirable physicochemical properties. The metal oxides can be prepared in various sizes from micro to nanometers.64 A metal oxide is considered a conventional model due to its excellent nature, such as abundant active sites, larger specific surface area, various electronic states, excellent electronic conductivity, enhanced electrochemical behavior, and thermal properties.62,65 The metal cation and the oxygen anion coordination located in the metal oxide surface leads to the formation of the relative acidity and basicity of the atom. The metal oxide's acidity and basicity sites significantly impact its catalytic properties. Infrared spectroscopy, calorimetry, and other methods were used to identify the acidic and basic sites of metal oxides.66,67
Metal oxide nanoparticles or materials modified or coated with functional groups, ligands, polymers, or other molecules to impart specific characteristics or functions are referred to as functionalized metal oxides.68 The purpose of these modifications is frequently to improve the material's performance in a variety of applications, such as biomedical, sensing, and catalysis.69 This functionalization adds novel performance, improves electrocatalytic characteristics, and allows for more specific interactions with pesticide analytes, resulting in increased sensitivity, selectivity, and overall performance.70 For example, Krishna et al.71 developed metal oxides with surface modification for highly sensitive electrochemical detection of heavy metals, organophosphates, and nutrients in water samples. The detecting agents ZrO2, MnO2, and MgO, incorporated into the ink, exhibit specific affinities for lead, fenitrothion, and nitrite, respectively. Metal oxides are immobilized on a GCE that has been modified with nitrogen–sulfur co-doped activated carbon-coated carbon nanotubes. The resulting proposed sensor demonstrates excellent electrocatalytic properties for fenitrothion detection with a detection limit of 1.69 nM, showing reliability in water samples.
Metal oxides are used as food additives to improve the visual appeal, texture, and nutritional content of numerous food products.72 Metal oxide nanoparticles (NPs), such as titanium dioxide (TiO2), silicon dioxide (SiO2), zinc oxide (ZnO), and iron oxide (Fe2O3) are used as food additives in a variety of ways. These metal oxides are used for various functions, including food coloring, anti-caking, antimicrobial, and micronutrient supplements.73 TiO2 is a common white pigment,74 SiO2 is utilized as an anti-caking agent and thickener,75 ZnO is used as a nutrient supplement,76 and Fe2O3 nanoparticles are used as natural food coloring agents in the food industry.77 For example, Garcia et al.78 previously discussed the metal oxide-based food additives in food packaging, applications, migration, and regulations. In nanotechnology, advancements can result in the development of novel metal oxide formulations that offer improved bioavailability and targeted nutrition delivery.
Metal oxides can also undergo photo-assisted adsorption and deportation to control their semiconductor properties.79 The reactivity towards electromagnetic radiation, multiple valences, and the oxidation state of metal oxides make them more promising materials for catalytic reactions such as electrochemical redox reactions, isotopic exchange, photocatalysis, and various other applications currently under investigation by researchers.80 Sivalingam et al.81 demonstrated that the Co3O4 material possesses a redox activity characteristic that can be used for energy storage and sensing applications. The outstanding properties of metal oxides make them excellent candidates in several fields like electrochemical and bio-sensors, electrocatalysis, energy storage, optoelectronics, photoelectrochemical conversion, and surface-enhanced Raman spectroscopy.51,54,82–84 Therefore, metal oxides are considered one of the crucial materials in the field of electrochemical sensors.
2.1. Synthesis techniques of metal oxides
Metal oxides that have received the most attention for electrochemical detection of pesticides in aqueous systems are titanium iron, copper, manganese, and zinc oxides.85–87 They can be found in a variety of forms, including particles, rods, cubes, tubes, and others. Metal oxides' shape and size both have an impact on their electrocatalytic performance. Over the last decade, researchers have focused on developing efficient synthetic methods for producing highly stable, shape-controlled, and monodispersed metal oxide nanomaterials.60 In general, synthesis procedures can be divided into two types: (1) physical methods such as high-energy ball milling, severe plastic deformation, ultrasound shot peening, inert gas condensation, and (2) chemical methods such as controlled chemical coprecipitation, pulse electrode position, chemical vapor condensation, liquid flame spray, microemulsion, gas-phase reduction, liquid-phase reduction, etc.88 Hydrothermal, thermal decomposition and coprecipitation synthesis procedures are widely used because of their high yields and scalability, making them simple to execute.89 Metal oxide characterization research focused on crystal structure, size, morphology, and specific surface area properties. Moreover, several electrochemical sensor-based modified electrodes were introduced to determine pesticides in environmental samples. Balamurugan et al.90 synthesized the dysprosium stannate electrode material using a simple coprecipitation method for electrochemical detection of carbofuran. Ganesan et al.91 developed neodymium molybdate by a hydrothermal approach and used it to construct an electrochemical sensor for methyl parathion detection. Kokulnathan et al.92 synthesized samarium molybdate using a sonochemical method for electrochemical detection of carbendazim. Compared to all other synthesis methods, coprecipitation, hydrothermal, and sonochemical methods are the most efficient and beneficial due to low cost, environmental friendliness, high yield, mild conditions, less pollution, and effective reaction. The most commonly used metal oxide synthesis methods for pesticide detection are summarized in Table 2.
Table 2 A summary of used metal oxide synthesis methods for pesticide detection
Materials |
Synthesis method |
Morphology |
Pesticides |
Reference |
Fe3O4 |
Hydrothermal |
Quasi-spherical |
Malathion |
183
|
TiO2 |
Sonochemical |
Long tube |
Paraquat |
184
|
Dy2Sn2O7 |
Coprecipitation |
Nanoplatelet |
Carbofuran |
90
|
CeO2 |
Sonochemical |
Spherical |
Hydrazine |
185
|
AgWO4 |
Hydrothermal |
Nanoparticle |
Crisquat |
177
|
NiCo2O4 |
Hydrothermal |
Microsphere |
Paraoxon ethyl |
186
|
PrCoO3 |
Hydrothermal |
Nanoflake |
Carbendazim |
175
|
SrTiO3 |
Hydrothermal |
Micro-pebble |
2,4,6-Trichlorophenol |
155
|
Fe3O4 |
Coprecipitation |
Nanoparticle |
Triazine |
187
|
2.2. Important properties of metal oxides in developing electrochemical sensing
Metal oxides are economical, easily synthesized, biocompatible, and loaded with a catalyst.93 Thus, they are potential materials for altering electrodes. Furthermore, metal oxides have tuneable properties due to their flexible structure. The remarkable characteristic of metal oxides is their capacity to immobilize the electrocatalyst, which improves a sensor's selectivity and sensitivity against environmental contaminants by loading the electrocatalyst in a metal oxide.59 For example, cerium oxide electrode materials have exhibited outstanding electrocatalytic activity because of their mixed-valence oxidation state conversion between Ce(III) and Ce(IV), which helped with fast electron transport.94 Furthermore, Fe3O4 electrode materials have unique properties such as high mobility of oxygen ions, superparamagnetic, low toxicity, biocompatibility, small sizes, and extraordinary catalytic activities in oxidative processes, which lead to increased sensitivity and stability of pesticide detection.95,96 Spinel-type oxides demonstrate exceptional structural durability. They also possess a distinctive pore volume and offer a greater number of electrochemically active sites. These properties arise from the synergistic effect of diverse metal ion configurations, allowing for the utilization of bimetallic catalysts in electrochemical pesticide sensors.97 Metal oxides, such as TiO2, ZnO, and SnO2, exhibit high sensitivity to various pesticides due to their semiconducting nature.98–100 Bao et al.101 developed a TiO2 material that demonstrated excellent electrocatalytic performance due to its textural properties, pore dimension, pore volume, distribution, and specific surface area. For these reasons, metal oxides have been used to create a variety of electrochemical sensors that can be utilized in various environmental monitoring applications.93,102 Electrode modification with metal oxide materials has numerous applications in sensing heavy metals, phenolics, pesticides, and other harmful inorganic compounds.103–106 These types of metal oxide electrodes, compared to other unique modified electrodes, have a promising future for detecting environmental toxins.
2.3. Classification of metal oxides on the basis of their importance for different pesticides
Metal oxides have significance in a variety of applications, including pesticides. Metal oxides can be classified according to their importance for various pesticides based on their distinct properties and functions. Antifungal metal oxides: copper oxides (CuO and Cu2O) are known for their antifungal properties. Fungicides containing them are used to control several fungal diseases in crops. Copper-based pesticides are efficient against a wide range of fungi.107 Herbicide metal oxides: aluminium oxide (Al2O3) nanoparticles have also shown promise in herbicide formulations. They can improve the adhesion and penetration of herbicides on plant surfaces. Iron oxide (Fe2O3) nanoparticles have been investigated for use in herbicides. They can be utilized as herbicidal substance carriers, enhancing their transport and effectiveness. Titanium dioxide (TiO2) is used as a pesticide carrier and can enhance the adhesion of the active ingredient to the target surface.108,109 Insecticide metal oxides: some insecticides contain zinc oxide (ZnO). It can act as a carrier for other active substances and is used in pest control formulations. Magnesium oxide (MgO) can improve the efficacy of insecticides by serving as a transporter or synergist. Silicon dioxide (SiO2) is frequently used as an enhancer or transport in pesticide formulations. It can improve active component stability, dispersibility, and effectiveness.110,111
2.4. Diverse tuning approaches for enhancing metal oxide electrochemical sensing
Metal oxides are versatile materials with specific electrochemical properties that allow them to be used in a wide range of sensing applications. Researchers use several tuning approaches to improve their performance. These approaches are aimed at modifying the structure, composition, and surface properties of metal oxides. In this section, we look at various tuning strategies for improving the electrochemical sensing capabilities of metal oxides. Scheme 1 shows the schematic diagram of several tuning approaches for improving metal oxide electrochemical sensing applications.
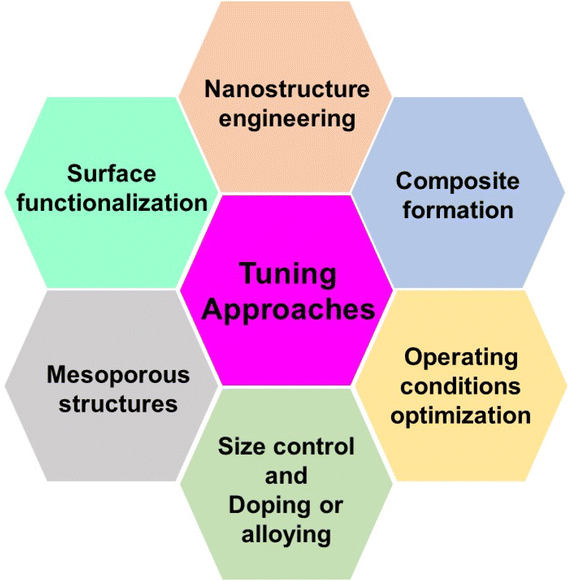 |
| Scheme 1 The schematic diagram of several tuning approaches for improving metal oxides–electrochemical sensing applications. | |
(1) Nanostructure engineering: the surface area and active sites are improved using nanostructure engineering to increase electrochemical reactivity. Techniques like sol–gel synthesis, hydrothermal methods, or template-assisted processes are used. Nanostructured metal oxides have higher sensitivity and faster response times because of their increased surface area and improved charge transfer kinetics.112,113
(2) Surface functionalization: the surface is modified with specific functional groups to improve selectivity. Organic molecules, polymers, or biomolecules are attached to the surface of the metal oxide. Functionalization enhances the affinity of metal oxides towards target analytes, improving selectivity and reducing interference from other species.114,115
(3) Mesoporous structures: mesoporous metal oxides can improve mass transport and accessibility of analytes to the electrode surface. Mesoporous metal oxide structures are prepared using sacrificial or template techniques. The mesoporous structure improves sensor performance by accelerating the diffusion of target species to the electrode surface.116,117
(4) Size control: the size of metal oxide particles is modified to enhance their electrochemical performance. The particle size is precisely controlled during synthesis through variations in reaction conditions. The tunable features of size-controlled metal oxides enhance the electrochemical sensors' sensitivity, selectivity, and response times.118,119
(5) Doping or alloying: the electronic and chemical properties are modified to optimize sensor performance. This is done by introducing doping elements or forming alloys with metal oxides during synthesis. The range of applications for metal oxide sensors can be increased by doping with elements like transition metals, enhancing selectivity, conductivity, and catalytic activity.120–122
(6) Operating condition optimization: the experimental parameters such as pH, temperature, and applied potential are adjusted. The operational conditions are investigated and optimized methodically for the best sensor efficiency. It is ensured that the sensor operates under ideal conditions, which enhances the signal-to-noise ratio and overall performance.123–125
(7) Composite formation: the advantages of different materials are combined to achieve a synergistic effect. Metal oxides are integrated with other materials, such as incorporate polymers, carbon materials, or conductive nanomaterials. Composite metal oxides enhance conductivity, stability, and sensitivity, offering improved overall performance compared to individual components.126–128
3. Metal oxide-based electrochemical sensors for pesticide detection
Electrochemical sensors have been developed regularly with the advancement of electrical devices and metal oxides, notably in pesticide monitoring.129 However, due to the lower electroactivity of electrochemical sensors, the electrochemical detection of pesticides without a modified electrode is challenging. Because of this, modified electrodes are needed for signal measurability in pesticide detection. Metal oxides are being used as electrode materials due to their enhanced electrocatalytic activity, portability, and accuracy.130–132 Thus, various reviews have thoroughly clarified the preparation, mechanism, and applications of metal oxide-based enhanced electrochemical detection.59,61,112,133,134 This section mainly discusses the various pesticide monitoring-based electrochemical sensors created in the area using a range of metal oxides. Metal oxides are being presented as an appealing choice for designing and fabricating effective electrochemical sensors due to their superior physical and chemical properties.
3.1. Methyl parathion sensor
Methyl parathion (MPN) is an organophosphorus pesticide widely used as a fungicide and insecticide in industry and agriculture because of its high toxicity and effectiveness.135 MPN has a substantial impact on agricultural product harvesting. The significant toxicity of MPN residue can readily pose severe safety threats to food safety and the ecological system.136 Therefore, developing an effective, easy, economical, and highly sensitive detection MPN monitoring technology is critical. Ganesan et al.91 synthesized a porous 3D flower-like neodymium molybdate (pf-Nd2Mo3O9) by a hydrothermal approach to construct an electrochemical sensor for MPN detection. The structure and shape of the pf-Nd2Mo3O9 material were confirmed by X-ray diffraction (JCPDS no. 33-936) and TEM investigation (Fig. 2A). A cyclic voltammetry (CV) sensor for MPN organophosphate pesticide detection was developed using the pf-Nd2Mo3O9 electrode material. This procedure used differential pulse voltammetry (DPV) with a glassy carbon electrode (GCE). The electrochemical sensor for detecting MPN was developed by immersing an electrode in an electrolytic solution containing the required pesticides. Fig. 2B depicts the variation in the current as a function of the amount of the pf-Nd2Mo3O9 modified GCE. The peak current response was higher after modifying the pf-Nd2Mo3O9/GCE, compared to the unmodified GCE because of its high surface area (0.18 cm2), superior charge transit, and more active sites. These results show that modifying pf-Nd2Mo3O9/GCE can provide superior electrocatalytic performance for MPN detection. According to the linearity study, the electrochemical detection of MPN has a concentration range of 0.5 to 300 μM. The electrochemical detection mechanism of MPN reveals that the cathodic peak (R1) is attributed to the direct reduction of MPN by hydroxylamine (4e− & 4H+ transfer process), while the redox couple (O1 & R2) results from the reversible behavior of hydroxylamine to nitroso derivatives (2e− & 4H+ transfer process)91,137 (Fig. 2D). Fig. 2C depicts the DPV cure of pf-Nd2Mo3O9/GCE for different MPN concentrations vs. obtained current response. According to the DPV studies, the calculated sensitivity and limit of detection values were 1.88 μA μM−1 cm−2 and 5.7 nM. The investigated technique was used to measure the amount of MPN in water and food samples. These results demonstrate that the technique produces accurate results when used to monitor MPN in a variety of contaminated food and water samples.
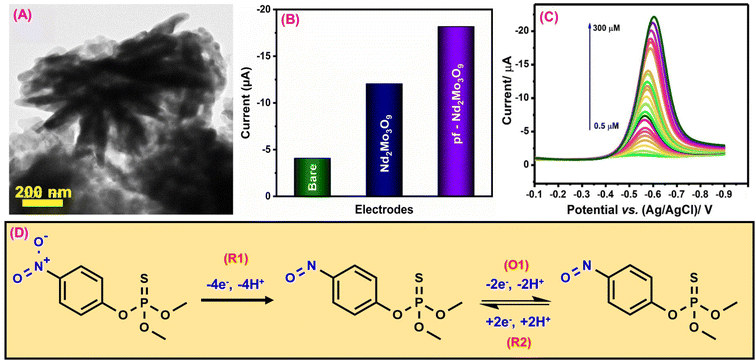 |
| Fig. 2 (A) TEM image of pf-Nd2Mo3O9. (B) CV investigation of the bare GCE (green), Nd2Mo3O9 (blue), and pf-Nd2Mo3O9 (purple). (C) DPV signal of pf-Nd2Mo3O9 for MPN detection. (D) Electrochemical detection mechanism of MPN103 (modified). ((A and C) License number: 5542351439158). | |
3.2. Carbendazim sensor
Carbendazim (CRM) is a benzimidazole fungicide whose benzimidazole ring is tough to break, leading to environmental harm.138 CRM is employed in agriculture during pre and post-harvest for pathogen protection, which will harm fruits and crops.139 The CRM residue can cause eye irritation, contact with derma, liver disease, skin inflammation, and chromosome changes in organisms which results in adversative environmental impact.140 CRM is a carcinogen for human health due to its toxicity even though it has agricultural and economic benefits. Compared to other techniques, the electrochemical approach has proven to be reliable for CRM detection. Kokulnathan et al.92 effectively synthesized a samarium molybdate (Sm3(MoO4)3) electrocatalyst for sensitive electrochemical CRM detection in real-world environmental samples (Fig. 3A). A simple sonochemical approach for producing a Sm3(MoO4)3 nanomaterial that is more stable, anti-interference and sensitive for CRM detection by an electrochemical sensor has been developed.92 The structure and shape of the Sm3(MoO4)3 material were confirmed by X-ray diffraction (JCPDS no. 34-0512), and FE-SEM (2D nanosheets) investigation (Fig. 3B and C). The Sm3(MoO4)3 modified screen-printed electrode (SPCE) current response (6.6 μA) was two fold higher than that of the unmodified SPCE due to extraordinary properties of Sm3(MoO4)3, such as chemical stability, abundant reserves, and multiple valences. Joseph & Santhoshkumar et al.141,142 discussed the electro-oxidation mechanism of CRM. They found that the process is quasi-irreversible and involves the transfer of two electrons and two protons. This transfer leads to the formation of the benzimidazole radical and methyl carbamate. The benzimidazole radical undergoes dimerization, and the resulting dimer undergoes reduction, eventually producing alcohol. Therefore, in CRM, the imidazole-2-yl-carbamate component passes through oxidation, and a two-electron and two-proton reaction produces the imidazole carbonyl derivative (Fig. 4). Fig. 3D reveals the DPV results of Sm3(MoO4)3/SPCE for different CRM concentrations vs. obtained current response and linearity studies (R2 = 0.9902). According to the DPV studies, the calculated sensitivity and limit of detection values are 13.34 μA μM−1 cm−2 and 1 nM. The performance of the proposed sensor suggests that Sm3(MoO4)3 has a promising future for determining CRM in environmentally hazardous samples.
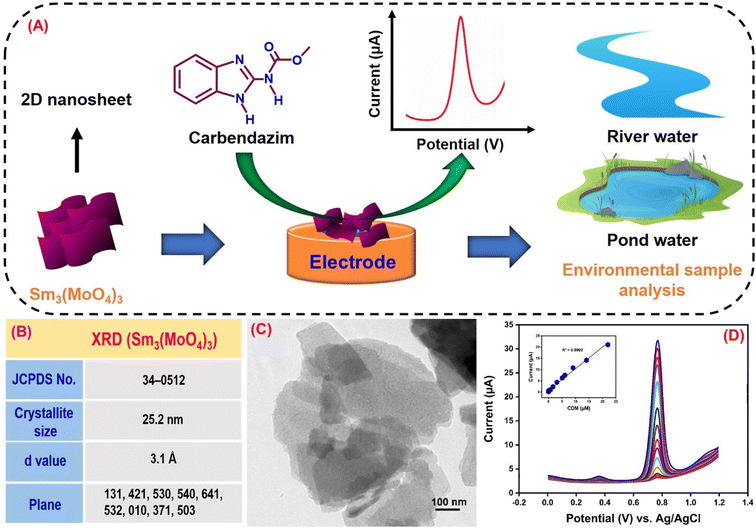 |
| Fig. 3 (A) Graphical illustration of Sm3(MoO4)3 as an electrode material for a CRM sensor. (B) Crystal structure studies of Sm3(MoO4)3. (C) Morphological studies of Sm3(MoO4)3. (D) DPV curves of Sm3(MoO4)3 for CRM detection79 (modified). ((C and D) License number: 5547980179571). | |
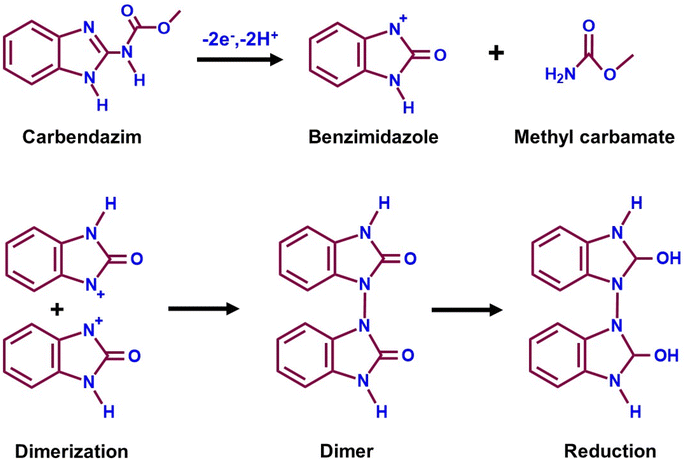 |
| Fig. 4 The possible electrochemical detection mechanism of CRM. | |
3.3. Paraoxon sensor
Paraoxon is an organophosphate pesticide commonly used in agriculture to control insects/pests and produce a huge amount of crops while harming environmental and biological systems.143 This insecticide, mainly sprayed on beans, wheat, cotton and fruits, can affect the neurological systems of all living things, including watersheds, humans, and animals.144 Gopi et al.145 constructed an effective electrochemical sensor based on BiVO4 as an electrocatalyst for detecting hazardous paraoxon pesticide. The material characterization revealed that the synthesized BiVO4 had superior conductivity, sufficient oxygen vacancies, and good chemical stability. Fig. 5A shows the crystal structure of the synthesized BiVO4 material.146 TEM image confirmed the open walnut-like structure branched dendrites of BiVO4 (Fig. 5B).147 In this process, DPV with a SPCE was used. The electro-reduction current response of paraoxon towards BiVO4/SPCE and the bare SPCE was investigated using the CV technique (Fig. 5C). This study showed that, compared to the bare SPCE, the modified BiVO4 material had a high current response and minimum potential window, indicating that the BiVO4 material had excellent electroconductivity for paraoxon detection. The possible electrochemical redox process of paraoxon is depicted in Fig. 6, and is more similar to previously described literature.148Fig. 5D shows the DPV signals for paraoxon concentrations using BiVO4/SPCE. The limit of detection and sensitivity for paraoxon were calculated to be 0.034 μM and 0.0345 μA μM−1 cm−2. Compared to conventional detection materials, the BiVO4/SPCE sensor performs admirably.
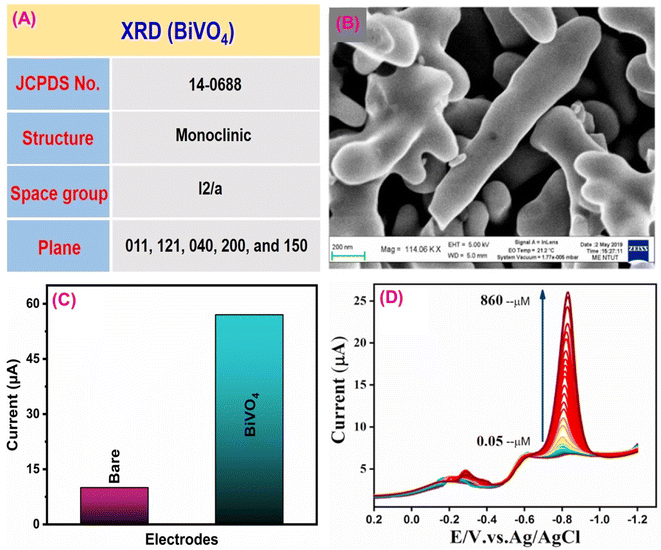 |
| Fig. 5 (A) XRD data of BiVO4. (B) TEM data of BiVO4. (C) CV signal of the bare SPCE and BiVO4/SPCE towards paraoxon detection. (D) DPV signal of BiVO4/SPCE for detection of various concentrations of paraoxon112 (modified). ((B and D) License number: 5542341491022). | |
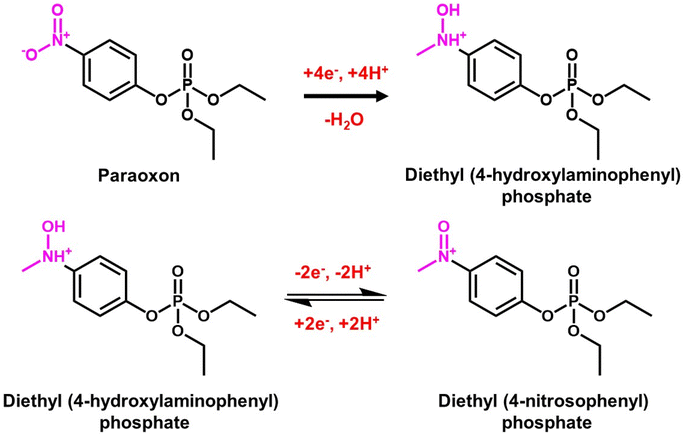 |
| Fig. 6 The electrochemical oxidation mechanism of the paraoxon sensor. | |
3.4. Fenitrothion sensor
Fenitrothion (FN) is an organophosphate compound that is widely used as an insecticide in current agricultural practices. However, prolonged exposure to FN poses significant risks to human health and can lead to pollution of food, water, and land sources. It serves an effective solution for controlling flies, cockroaches, and mosquitoes in agricultural contexts and public health initiatives when applied as a residual contact spray.149–151 Given these concerns, Ganesan et al.152 proposed a green approach for developing dysprosium vanadate (DyVO4) for FN insecticide detection. The crystal structure of the DyVO4 electrode material has been verified using the JCPDS no. 074-0775 reference (Fig. 7A). They developed a DyVO4 electrode material through a hydrothermal approach, which attained a 3D hierarchical micro flower-like morphology (Fig. 7B). Morphological characterization is more critical in the electrochemical sensor since both their shape and size have an impact on their electrocatalytic performance. The DyVO4 modified GCE current response (−52 μA) was four-fold higher than that of the unmodified GCE due to extraordinary properties of DyVO4, such as excellent stability, multilayer architectures, variety of active states, presence of oxygen sites, and higher energy density (Fig. 7C). The R1 reduction peak results from the transfer of four electrons (+4e−) and four protons (+4H+) from FN's nitro group (NO2–FN) to the hydroxylamine group (NHOH–FN). Additionally, the reverse scan revealed the presence of the redox peaks O1 and R2, which are associated with the conversion of nitroso–FN (NO–FN) from NHOH–FN (−2e−, −2H+) and its subsequent reduction back to NHOH–FN (+2e−, +2H+).152 According to the DPV studies, the calculated sensitivity and limit of detection values are 14.2 μA μM−1 cm−2 and 1.4 nM (Fig. 7D). The results of this sensor demonstrate that DyVO4 is a promising tool for monitoring FN in environmental pollutant samples.
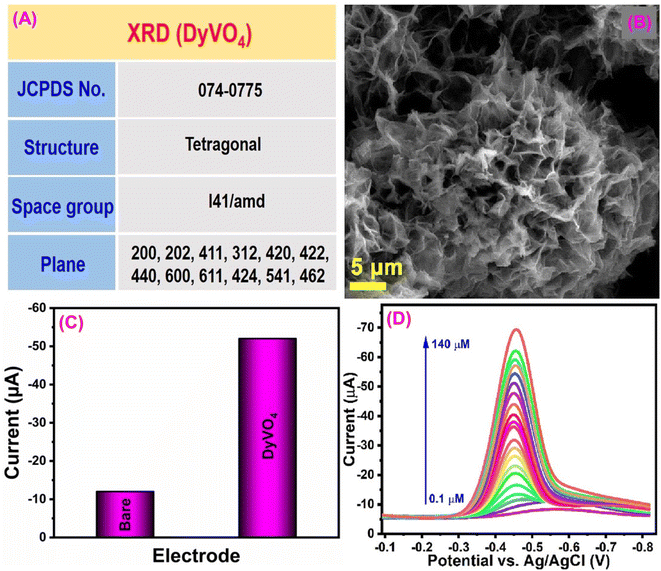 |
| Fig. 7 (A) Crystal structure analysis of DyVO4. (B) Morphological studies of DyVO4. (C) CV response of the bare GCE and DyVO4/SPCE towards FN detection. (D) DPV response of DyVO4/GCE for detection of various concentrations of FN119 (modified). ((B and D) License number: 5550950069266). | |
3.5. 2,4,6-Trichlorophenol sensor
2,4,6-Trichlorophenol (TCL) is a chlorinated organic compound that has been identified as one of the most hazardous organic pollutants due to its extensive development and use in industrial and agricultural operations.153,154 TCL is widely utilized in a variety of sectors, including medicines, disinfectants, insecticides, fungicides, pesticides, and intermediates in the manufacturing of various dyes, as well as wood preservatives.155 The untreated discharge of TCL-contaminated water into the aquatic environment can harm drinking water and human health.155,156 Ramadhass et al.157 created a sensor containing lanthanum stannate (Ln2Sn2O7) that was synthesized using a simple coprecipitation method and evaluated its electrochemical properties to detect TCL (Fig. 8A). Furthermore, the morphological investigation using FE-SEM determined the structure of the Ln2Sn2O7 material (hexagonal nanosheets). On the other hand, the crystal structure (cubic and hkl planes) investigation with XRD was used to analyze the physicochemical properties of Ln2Sn2O7.158 An increase in the oxidation current response as compared to bare shows that Ln2Sn2O7 has catalytic activity for the electrochemical oxidation of TCL (Fig. 8B). It is possible that TCL behaves similarly to the 4-nitrophenol oxidation mechanism.157,159 The proposed Ln2Sn2O7 electrode-based TCL sensor exhibits a wide linear range (0.1–650 μM), a low-level limit of detection (0.074 μM), and excellent sensitivity (1.5 μA μM−1 cm−2) (Fig. 8C). This sensor attained higher levels of storage stability, reproducibility, and repeatability than the diagnostic techniques that have been previously reported,160–162 and it offers substantial technological breakthroughs. The proposed Ln2Sn2O7 electrode-based TCL sensors are the best option for environmental sample monitoring based on the reasonable results of the real sample determination.
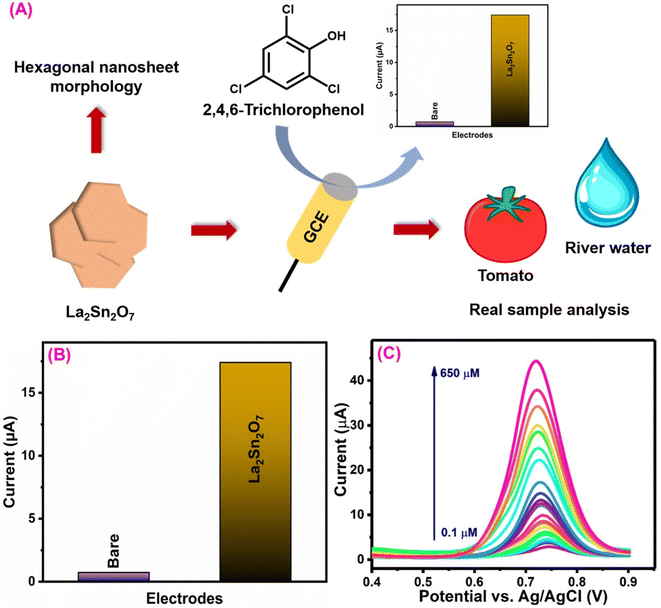 |
| Fig. 8 (A) Schematic diagram for the synthesis of the La2Sn2O7 nanosheet and its electrochemical detection studies. (B) CV current response of the bare GCE and La2Sn2O7/GCE towards TCL detection. (C) DPV response of La2Sn2O7/GCE for detection of various concentrations of TCL124 (modified). ((C) License number: 5570091012633). | |
3.6. Carbofuran sensor
Carbofuran (CBF), often known as furadan, is one of the most significant carbamate pesticides. It can shorten the growth time of crops and boost agricultural yields.163,164 Because of their high insecticidal mobility, relatively low ecological persistence, and great efficacy in eradicating insects and pests, carbamate compounds are a wide range of pesticides used extensively around the world.165 CBF is mainly used to control insects in pumpkins, tomatoes, bananas, mangoes, maize, soybeans, rice, potatoes, corn, and brinjals. It can overstimulate the neurological system, leading to death, respiratory paralysis, disorientation, dizziness, and drowsiness.166 Rajiv et al.167 designed a biogenic iron oxide/magnetic glassy carbon electrode (Fe3O4/MGCE) for electrochemical detection of CBF in various vegetable samples. Artocarpus heterophyllus seed extract was used to prepare Fe3O4 nanoparticles. They attempted a green chemical synthesis of Fe3O4 nanoparticles without the use of any surfactant or other reducing reagent. Furthermore, the synthesized Fe3O4 was characterized and validated using several analytical techniques.167 The electrochemical catalytic activity of the Fe3O4 on the modified electrode surface must boost the electron transfer process, leading to CBF oxidation (Fig. 9A). Fig. 9C depicts the detailed electrochemical oxidation detection mechanism of CBF.166 The Fe3O4-based CBF sensor has attained excellent sensitivity (6.53 ± 0.084 μA μM−1 cm−2), a wide linear range (0.3 to 38.5 μM), and a low limit of detection (0.01 μM) (Fig. 9B). The benefits of the suggested sensor, such as high sensitivity, reproducibility, storage stability, and selectivity have resulted in a significant result for real-world detection of CBF in various food samples. After reviewing the existing literature, it is summarized that the metal oxide-based electrode material is suitable for the electrochemical detection of pesticides in water and food samples (Table 4). Overall, compared to other electrode materials in this study, the Sm3(MoO4)3-based electrode materials are more attractive due to their economy, low limit of detection, high sensitivity, and high electrocatalytic activity performance.
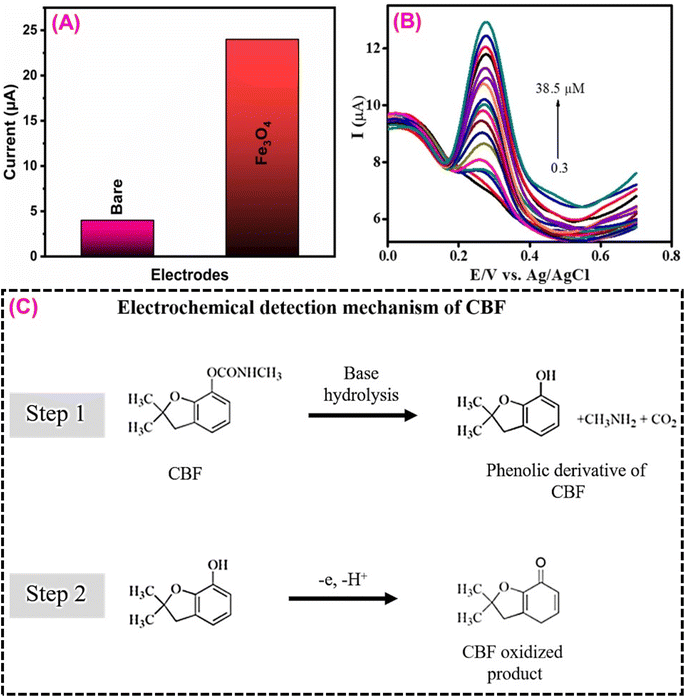 |
| Fig. 9 (A) CV current signal of Fe3O4/MGCE and Fe3O4/MGCE towards CBF detection. (B) DPV signal of Fe3O4/MGCE for the CBF concentrations from 0.3–38.5 μM. (C) Possible electrochemical detection mechanism of CBF134 (modified). ((B) License number: 5570091331923). | |
4. Environmental sample analysis
Examining the practical applicability is crucial for electrochemical pesticide detection. The electrochemical techniques were also used to detect pesticides in real-world samples. The proposed approach was used to determine pesticides in water and food samples to ensure their applicability in real-world analyses. Zeng et al.168 used the spiking method to evaluate the practical use of the developed sensor in detecting simazine in various water samples. This investigation showed that tap, river, and pond water samples had a high recovery rate.169 Prabhu et al.170 reported a synthesis of ZnO@Cu nanoparticles with the CTAB surfactant for electrochemical detection of 2,4-dichlorophenol in environmental samples. This study used square wave voltammetry (SWV) with a carbon paste electrode (CPE). The real-time application of the suggested ZnO@Cu/CPE towards 2,4-dichlorophenol was tested using environmental samples such as water, vegetables, and fruits. Furthermore, a one-step co-electrodeposition method was developed for fabricating ZrO2-decorated graphene-based electrochemical sensors for methyl parathion detection in vegetable samples. This sensor demonstrated excellent stability, reproducibility, and a new and promising approach for pesticide analysis.171 Moghaddam et al.172 created a Pt/NiCo2O4 electrode material with distinct structural properties using a hydrothermal approach and subsequently used it as a new platform for carbendazim detection. DPV and liquid chromatography-mass spectrometry (LC-MS) with a SPCE were used for this practical study. This sensor was used to determine pesticides in tomato and lettuce samples to ensure its applicability in real-world analyses. Then, Narayanan et al.173 developed a hybrid nanomaterial CuO/Nd2O3 using a one-step coprecipitation approach and investigated a novel platform for non-enzymatic malathion pesticide detection. This sensor electrode material exhibits intriguing electrochemical properties due to its nano-size, stability, and high surface area. The vegetable & fruit extract samples were tested using the DPV technique at varied concentrations of malathion pesticide. The proposed sensor results indicate that the approach is highly accurate in real-world applications. The electrochemical detection of pesticides in environmental samples using metal oxide electrode materials is presented in Table 3.
Table 3 A summary of metal oxide electrode material-based electrochemical detection of pesticides in water and food samples
Materials |
Pesticides |
Real samples |
Recovery (%) |
RSD |
Reference |
GeO2@Pd |
Simazine |
Water |
92–111 |
0.42 to 1.58 |
169
|
ZnO@Cu |
2,4-Dichlorophenol |
Water |
98.03–99.85 |
0.218–0.946 |
170
|
Vegetables & fruits |
97.08–105.52 |
0.786–2.810 |
ZrO2 |
Methyl parathion |
Vegetables |
96.5–104.4 |
1.0–4.2 |
171
|
CuO NFs |
Chlorpyrifos |
Fruits and vegetables |
95.98–106.72 |
2.25–4.82 |
188
|
Pt/NiCo2O4 |
Carbendazim |
Vegetables |
97.3–103 |
1.9–2.8 |
172
|
CuO–Nd2O3 |
Malathion |
Vegetables & fruit extract |
94.0–101 |
1.41–9.26 |
173
|
CuO/TiO2 |
Methyl parathion |
Ground water |
98.80–106.72 |
2.13–3.45 |
85
|
TiO2/CTAB |
Aminotriazole |
Water |
96.23–99.56 |
0.17–1.26 |
189
|
Cu/RGO |
Carbendazim |
Pond water |
99.7–102.2 |
— |
190
|
SiO2–MnO2 |
Paraoxon |
Water |
97.9–104.4 |
2.1–8.8 |
191
|
Fe3O4@SiO2 |
Imidacloprid |
Water, fruits & vegetables |
94.80–109.40 |
1.22–10.39 |
192
|
Ag/ZnO |
Phoxim |
Water & vegetables |
94.3–105.7 |
4.08–4.97 |
193
|
5. Future perspectives and challenges
Pesticides have been utilized to boost agricultural yields worldwide throughout the past century. However, their environmental residues are extremely toxic to nontarget species such as humans and mammals. Thus, reliable technologies for precisely determining these pesticides are reported in the literature. Electrochemical methods, such as voltammetry, DPV, SWV, and amperometry, are the most widely used technology for detecting electroactive chemicals such as pesticides. The creation of metal oxide-based sensors with improved selectivity, sensitivity, and durability by overcoming the drawbacks of enzyme-based sensors has recently sparked increased interest in developing enzyme-free sensors. The rapid advances in environmental technology may be seen in two areas: expanding worldwide awareness of the effects on the environment and public health and commercialization and large-scale production techniques for creating electrochemical sensors. As efforts are undertaken to address environmental concerns and enhance public health outcomes, these sectors are making substantial progress. The usage of a smartphone-based sensor enables the technology's possible commercialization. This means that the sensor might be manufactured on a bigger scale, sold, and used by individuals or organizations to monitor pesticides in real time. This commercialization potential suggests that the technology may become more accessible and widespread in the future. Various methods for constructing electrochemical detection using new metal oxide electrode materials without supporting other materials have been proposed over the last few decades. These sensors, which incorporate greater stability and sensitivity, were designed using several materials' remarkable chemical, physical, and electrical features. Furthermore, using metal oxide electrode materials can potentially satisfy multi-detection requirements in real-world applications. Combining optical and electrochemical sensors into a dual-sensor system results in enhancing applicability and minimizing false positive signals possible. While only a limited number of preliminary studies have explored using metal oxide-based sensors for rapidly detecting pesticides in food, agriculture, health, and other domains, such platforms are essential for addressing future human health challenges and promoting a better quality of life.
Table 4 Important findings and summary of the present results
Metal oxide |
Important finding |
Ref. |
Neodymium molybdate |
The hydrothermal method was utilized to create 3D flower-like neodymium molybdate (pf-Nd2Mo3O9), which was then used to build an electrochemical sensor for methyl parathion (MPN) detection |
91
|
As a result of their large surface area (0.18 cm2), superior charge transit, and more active sites, the peak current response was greater after modifying pf-Nd2Mo3O9/GCE compared to the unmodified glassy carbon electrode (GCE) |
Samarium molybdate |
Samarium molybdate (Sm3(MoO4)3) with a significant level of active sites used for electrochemical detection of carbendazim (CRM) in environmental samples |
92
|
Due to a variety of extraordinary features of Sm3(MoO4)3, such as chemical stability, abundant reserves, and multivalence, the Sm3(MoO4)3 modified screen-printed electrode (SPCE) current response (6.6 μA) was two-fold greater than that of the unmodified SPCE |
Bismuth vanadate |
BiVO4 as an electrocatalyst in a high-performance electrochemical sensor for the detection of hazardous paraoxon pesticide |
145
|
The synthesized BiVO4 exhibited higher conductivity, enough oxygen vacancies, and chemical stability |
The modified BiVO4 material displays a strong current response and a small potential window when compared to bare SPCE, showing that the BiVO4 material has superior electroconductivity for paraoxon detection |
Dysprosium vanadate |
Due to DyVO4's extraordinary features, such as superior stability, multilayer structures, a diversity of active states, the presence of oxygen sites, and increased energy density, the current responsiveness of the DyVO4 modified GCE (−52 μA) was four times that of the unmodified GCE |
152
|
Lanthanum stannate |
The La2Sn2O7 sensor exhibits a lower charge transfer resistance compared to the unmodified electrode, leading to an increased anodic current response |
157
|
TCL's high sensitivity, excellent selectivity and a wide linear range allows for a low detection limit |
Iron oxide |
This sensor utilizes Fe3O4 nanoparticles that are synthesized using an eco-friendly and sustainable approach, employing a seed extract derived from Artocarpus heterophyllus |
167
|
The electrochemical catalytic activity of Fe3O4 on the modified electrode surface must boost the electron transfer process leading to CBF oxidation |
There are several challenges associated with this approach. The characterization of these electrodes can be tedious and time-consuming. Additionally, there is uncertainty surrounding the mechanism of the electrode process, and the stability and toxicity of metal oxides pose further concerns. Incorporating metal oxides into molecularly imprinted polymer-based sensors can enhance the sensing of analyte molecules through guest–host interactions, but it may also introduce instability in some instances. Moreover, the preparation of electrodes can be costly and time-consuming, which can affect consistency. Synthetic routes for metal oxides often require special conditions, impacting their size, shape, stability, and, ultimately, the reproducibility of the sensor. Other parameters, such as electrolyte, temperature, and humidity, also influence the sensing abilities of pesticides. The stability and function of the sensor can be impacted by temperature and moisture, lowering its sensitivity and reaction time. The electrolyte can influence the target pesticide's chemical reactivity and solubility, which will affect the sensor response. Nevertheless, despite these challenges, the development of such sensing devices plays a vital role in improving various aspects of our lives. These devices significantly impact addressing issues related to environmentally hazardous materials, ensuring food safety, enhancing pharmaceutical and clinical diagnostics, and enabling effective security surveillance. Looking ahead, it is hoped that future research will yield promising results in producing cheaper and more environmentally friendly electrode materials, further advancing electrochemical sensing applications.
6. Conclusions
Accurate and direct pollution detection is a constant concern in environmental monitoring, health, life, and food safety. Rapid identification of pollutants in the environment is difficult, due to a lack of convenient and reliable detection methods. Electrochemical sensors are potential tools for detecting a wide range of biochemical targets, heavy metal ions, and environmental contaminants. This has a wide range of applications in environmental monitoring and other domains. The less expensive technology that benefits the public, particularly for agricultural uses, should be employed to put this research to good use. Metal oxides also have a bright technical future because they are economical and simple to prepare, and have various composition and preparation variables that can be used. The review article provides a comprehensive summary of recent advancements in metal oxide synthesis methods, properties, mechanisms, and the use of metal oxide-based electrochemical sensors for detecting pesticides in environmental samples. Furthermore, the study focuses on discussing various metal oxides (including neodymium molybdate, samarium molybdate, bismuth vanadate, dysprosium vanadate, lanthanum stannate, and iron oxide) employed in the electrochemical detection of pesticides (such as methyl parathion, carbendazim, paraoxon, fenitrothion, 2,4,6-trichlorophenol, and carbofuran) in water and food samples. Based on this study's findings, the Sm3(MoO4)3-based electrode material outshines other metal oxide electrode materials due to their economy, low detection limit, high sensitivity, and high electrocatalytic activity performance. Furthermore, developing an electrochemical sensor based on new metal oxides is a promising topic for improving sensitivity and selectivity. Using metal oxide-based portable sensors is likely to change commercial sensor technology in the future.
Conflicts of interest
The authors declare that they have no known competing financial interests or personal relationships that could have appeared to influence the work reported in this paper.
Acknowledgements
The authors gratefully acknowledge the financial support from the National Science and Technology Council, Taiwan, ROC, under the contracts NSTC 112-2218-E-006-025 and NSTC 112-2218-E-002-052 for this research. This research was also supported in part by the Higher Education Sprout Project, Ministry of Education to the Headquarters of University Advancement at National Cheng Kung University (NCKU).
References
-
A. Özkara, D. Akyıl and M. Konuk, in Environmental Health Risk-Hazardous Factors to Living Species, IntechOpen, 2016 Search PubMed
.
- A. Rhouati, M. Berkani, Y. Vasseghian and N. Golzadeh, MXene-based electrochemical sensors for detection of environmental pollutants: a comprehensive review, Chemosphere, 2022, 291, 132921 CrossRef CAS
.
- M. A. Khan, F. Ramzan, M. Ali, M. Zubair, M. Q. Mehmood and Y. Massoud, Emerging Two-Dimensional Materials-Based Electrochemical Sensors for Human Health and Environment Applications, Nanomaterials, 2023, 13, 780 CrossRef CAS PubMed
.
- M. Stoytcheva, V. Gochev and Z. Velkova, Electrochemical biosensors for direct determination of organophosphorus pesticides: a review, Curr. Anal. Chem., 2016, 12, 37–42 CrossRef CAS
.
- R. Umapathi, S. M. Ghoreishian, S. Sonwal, G. M. Rani and Y. S. Huh, Portable electrochemical sensing methodologies for on-site detection of pesticide residues in fruits and vegetables, Coord. Chem. Rev., 2022, 453, 214305 CrossRef CAS
.
- V. N. Rakitsky, V. A. Koblyakov and V. S. Turusov, Nongenotoxic (epigenetic) carcinogens: pesticides as an example. A critical review, Teratog., Carcinog., Mutagen., 2000, 20, 229–240 CrossRef CAS PubMed
.
- U. Saleem, S. Ejaz, M. Ashraf, M. O. Omer, I. Altaf, Z. Batool, R. Fatima and M. Afzal, Mutagenic and cytotoxic potential of endosulfan and lambda-cyhalothrin—in vitro study describing individual and combined effects of pesticides, J. Environ. Sci., 2014, 26, 1471–1479 CrossRef CAS
.
- J. Fenik, M. Tankiewicz and M. Biziuk, Properties and determination of pesticides in fruits and vegetables, TrAC, Trends Anal. Chem., 2011, 30, 814–826 CrossRef CAS
.
- A. Bertero, M. Chiari, N. Vitale, M. Zanoni, E. Faggionato, A. Biancardi and F. Caloni, Types of pesticides involved in domestic and wild animal poisoning in Italy, Sci. Total Environ., 2020, 707, 136129 CrossRef CAS
.
- H. Karimi-Maleh, F. Karimi, L. Fu, A. L. Sanati, M. Alizadeh, C. Karaman and Y. Orooji, Cyanazine herbicide monitoring as a hazardous substance by a DNA nanostructure biosensor, J. Hazard. Mater., 2022, 423, 127058 CrossRef CAS
.
- J. W. Song and H. N. Lim, Synthesis of carbamoyl fluorides via a selective fluorinative Beckmann fragmentation, Org. Lett., 2021, 23, 5394–5399 CrossRef CAS
.
- G. Velmurugan, K. Swaminathan, S. Mohanraj, M. Dhivakar, G. Veerasekar, T. Alexander, M. Cherian, N. G. Palaniswami and T. Pradeep, Association of co-accumulation of arsenic and organophosphate insecticides with diabetes and atherosclerosis in a rural agricultural community: KMCH-NNCD-I study, Acta Diabetol., 2020, 57, 1159–1168 CrossRef CAS PubMed
.
- J. M. Reiff, M. Ehringer, C. Hoffmann and M. H. Entling, Fungicide reduction favors the control of phytophagous mites under both organic and conventional viticulture, Agric., Ecosyst. Environ., 2021, 305, 107172 CrossRef CAS
.
- S. Singh, V. Kumar, R. Kanwar, A. B. Wani, J. P. K. Gill, V. K. Garg, J. Singh and P. C. Ramamurthy, Toxicity and detoxification of monocrotophos from ecosystem using different approaches: a review, Chemosphere, 2021, 275, 130051 CrossRef CAS
.
- P. d. J. Bastidas-Bastidas, J. Leyva-Morales, C. Olmeda-Rubio, J. Pineda-Landeros and I. Martínez-Rodríguez, Comparison of two methods for multi-residue analysis of organophosphorus pesticides in agricultural products with high and low moisture content, Revista Bio Ciencias, 2019, 6, e654 Search PubMed
.
- W. Huo and S. Yin, Determination of methamidophos, fonofos, parathion methyl and parathion in apple by solid-phase extraction and gas chromatography-mass spectrometry, J. Food Saf. Qual., 2017, 8, 3565–3570 Search PubMed
.
- M. H. Dehghani, Z. S. Niasar, M. R. Mehrnia, M. Shayeghi, M. A. Al-Ghouti, B. Heibati, G. McKay and K. Yetilmezsoy, Optimizing the removal of organophosphorus pesticide malathion from water using multi-walled carbon nanotubes, Chem. Eng. J., 2017, 310, 22–32 CrossRef CAS
.
- A. A. Ensafi, F. Rezaloo and B. Rezaei, Electrochemical determination of fenitrothion organophosphorus pesticide using polyzincon modified-glassy carbon electrode, Electroanalysis, 2017, 29, 2839–2846 CrossRef CAS
.
- G. Rosello-Marquez, R. M. Fernández-Domene and J. Garcia-Anton, Organophosphorus pesticides (chlorfenvinphos, phosmet and fenamiphos) photoelectrodegradation by using WO3 nanostructures as photoanode, J. Electroanal. Chem., 2021, 894, 115366 CrossRef CAS
.
-
H. M. Thompson and C. H. Walker, in Nondestructive Biomarkers in Vertebrates, CRC Press, 2020, pp. 37–62 Search PubMed
.
- W. da Silva, M. E. Ghica and C. M. Brett, Choline oxidase inhibition biosensor based on poly (brilliant cresyl blue)–deep eutectic solvent/carbon nanotube modified electrode for dichlorvos organophosphorus pesticide, Sens. Actuators, B, 2019, 298, 126862 CrossRef CAS
.
- P. Wang, H. Li, M. M. Hassan, Z. Guo, Z.-Z. Zhang and Q. Chen, Fabricating an acetylcholinesterase modulated UCNPs-Cu2+ fluorescence biosensor for ultrasensitive detection of organophosphorus pesticides-diazinon in food, J. Agric. Food Chem., 2019, 67, 4071–4079 CrossRef CAS
.
- A. Aswathi, A. Pandey and R. K. Sukumaran, Rapid degradation of the organophosphate pesticide-chlorpyrifos by a novel strain of Pseudomonas nitroreducens AR-3, Bioresour. Technol., 2019, 292, 122025 CrossRef CAS
.
- S. Zhao, W. Xu, W. Zhang, H. Wu, C. Guang and W. Mu, In-depth biochemical identification of a novel methyl parathion hydrolase from Azohydromonas australica and its high effectiveness in the degradation of various organophosphorus pesticides, Bioresour. Technol., 2021, 323, 124641 CrossRef CAS PubMed
.
- M. Shakourian, Y. Yamini and M. Safari, Facile magnetization of metal–organic framework TMU-6 for magnetic solid-phase extraction of organophosphorus pesticides in water and rice samples, Talanta, 2020, 218, 121139 CrossRef CAS
.
- S. Sabzevari and J. Hofman, A worldwide review of currently used pesticides' monitoring in agricultural soils, Sci. Total Environ., 2022, 812, 152344 CrossRef CAS PubMed
.
- M. Liu, J. Wei, Y. Wang, H. Ouyang and Z. Fu, Dopamine-functionalized upconversion nanoparticles as fluorescent sensors for organophosphorus pesticide analysis, Talanta, 2019, 195, 706–712 CrossRef CAS PubMed
.
- T. M. Oliveira, F. W. Ribeiro, C. P. Sousa, G. R. Salazar-Banda, P. de Lima-Neto, A. N. Correia and S. Morais, Current overview and perspectives on carbon-based (bio) sensors for carbamate pesticides electroanalysis, TrAC, Trends Anal. Chem., 2020, 124, 115779 CrossRef CAS
.
- Q.-Y. Wang, J. Yang, X. Dong, Y. Chen, L.-H. Ye, Y.-H. Hu, H. Zheng and J. Cao, Zirconium metal–organic framework assisted miniaturized solid phase extraction of phenylurea herbicides in natural products by ultra-high-performance liquid chromatography coupled with quadrupole time-of-flight mass spectrometry, J. Pharm. Biomed. Anal., 2020, 180, 113071 CrossRef CAS
.
- F. Suo, X. You, Y. Ma and Y. Li, Rapid removal of triazine pesticides by P doped biochar and the adsorption mechanism, Chemosphere, 2019, 235, 918–925 CrossRef CAS
.
- J. Juárez-Gómez, M. T. Ramírez-Silva, D. Guzmán-Hernández, M. Romero-Romo and M. Palomar-Pardavé, Construction and optimization of a novel acetylcholine ion-selective electrode and its application for trace level determination of propoxur pesticide, J. Electrochem. Soc., 2020, 167, 087501 CrossRef
.
- I. Al Yahyai and H. A. Al-Lawati, A review of recent developments based on chemiluminescence detection systems for pesticides analysis, Luminescence, 2021, 36, 266–277 CrossRef CAS PubMed
.
- J. Xu, H. Olvera-Vargas, F. Y. H. Teo and O. Lefebvre, A comparison of visible-light photocatalysts for solar photoelectrocatalysis coupled to solar photoelectro-Fenton: application to the degradation of the pesticide simazine, Chemosphere, 2021, 276, 130138 CrossRef CAS PubMed
.
- M. I. Khan, M. A. Shoukat, S. A. Cheema, H. N. Arif, N. K. Niazi, M. Azam, S. Bashir, I. Ashraf and R. Qadri, Use, contamination and exposure of pesticides in Pakistan: a review, Pak. J. Agric. Sci., 2020, 57, 1 Search PubMed
.
- Y. Zhu, M. Wang, X. Zhang, J. Cao, Y. She, Z. Cao, J. Wang and A. Abd El-Aty, Acetylcholinesterase immobilized on magnetic mesoporous silica nanoparticles coupled with fluorescence analysis for rapid detection of carbamate pesticides, ACS Appl. Nano Mater., 2022, 5, 1327–1338 CrossRef CAS
.
- K. Granby, J. H. Andersen and H. B. Christensen, Analysis of pesticides in fruit, vegetables and cereals using methanolic extraction and detection by liquid chromatography–tandem mass spectrometry, Anal. Chim. Acta, 2004, 520, 165–176 CrossRef CAS
.
- M. A. Z. Chowdhury, A. Fakhruddin, M. N. Islam, M. Moniruzzaman, S. H. Gan and M. K. Alam, Detection of the residues of nineteen pesticides in fresh vegetable samples using gas chromatography–mass spectrometry, Food Control, 2013, 34, 457–465 CrossRef
.
- M. M. Rahman, A. Abd El-Aty, S. W. Kim, S. C. Shin, H. C. Shin and J. H. Shim, Quick, easy, cheap, effective, rugged, and safe sample preparation approach for pesticide residue analysis using traditional detectors in chromatography: a review, J. Sep. Sci., 2017, 40, 203–212 CrossRef CAS PubMed
.
- M. Asensio-Ramos, J. Hernández-Borges, T. M. Borges-Miquel and M. Á. Rodríguez-Delgado, Ionic liquid-dispersive liquid–liquid microextraction for the simultaneous determination of pesticides and metabolites in soils using high-performance liquid chromatography and fluorescence detection, J. Chromatogr. A, 2011, 1218, 4808–4816 CrossRef CAS PubMed
.
- X. Pang, J. Qiu, Z. Zhang, P. Li, J. Xing, X. Su, G. Liu, C. Yu and R. Weng, Wide-scope multi-residue analysis of pesticides in beef by gas chromatography coupled with quadrupole Orbitrap mass spectrometry, Food Chem., 2023, 407, 135171 CrossRef CAS PubMed
.
- D. Sahoo, A. Mandal, T. Mitra, K. Chakraborty, M. Bardhan and A. K. Dasgupta, Nanosensing of pesticides by zinc oxide quantum dot: an optical and electrochemical approach for the detection of pesticides in water, J. Agric. Food Chem., 2018, 66, 414–423 CrossRef CAS
.
- M. Amde, Z.-Q. Tan, R. Liu and J.-F. Liu, Nanofluid of zinc oxide nanoparticles in ionic liquid for single drop liquid microextraction of fungicides in environmental waters prior to high performance liquid chromatographic analysis, J. Chromatogr. A, 2015, 1395, 7–15 CrossRef CAS PubMed
.
- R. Umapathi, C. V. Raju, S. M. Ghoreishian, G. M. Rani, K. Kumar, M.-H. Oh, J. P. Park and Y. S. Huh, Recent advances in the use of graphitic carbon nitride-based composites for the electrochemical detection of hazardous contaminants, Coord. Chem. Rev., 2022, 470, 214708 CrossRef CAS
.
- L. Killedar, D. Ilager, S. J. Malode and N. P. Shetti, Fast and facile electrochemical detection and determination of fungicide carbendazim at titanium dioxide designed carbon-based sensor, Mater. Chem. Phys., 2022, 285, 126131 CrossRef CAS
.
- U. Rajaji, S. Chinnapaiyan, S.-M. Chen, M. Govindasamy, J. I. d. Oliveira Filho, W. Khushaim and V. Mani, Design and fabrication of yttrium ferrite garnet-embedded graphitic carbon nitride: a sensitive electrocatalyst for smartphone-enabled point-of-care pesticide (mesotrione) analysis in food samples, ACS Appl. Mater. Interfaces, 2021, 13, 24865–24876 CrossRef CAS
.
- S. Tajik, H. Beitollahi, Z. Dourandish, P. Mohammadzadeh Jahani, I. Sheikhshoaie, M. B. Askari, P. Salarizadeh, F. G. Nejad, D. Kim and S. Y. Kim, Applications of non-precious transition metal oxide nanoparticles in electrochemistry, Electroanalysis, 2022, 34, 1065–1091 CrossRef CAS
.
- I. Hussain, S. Sahoo, D. Mohapatra, M. Ahmad, S. Iqbal, M. S. Javed, S. Gu, N. Qin, C. Lamiel and K. Zhang, Recent progress in trimetallic/ternary-metal oxides nanostructures: misinterpretation/misconception of electrochemical data and devices, Appl. Mater. Today, 2022, 26, 101297 CrossRef
.
- G. Maduraiveeran, M. Sasidharan and W. Jin, Earth-abundant transition metal and metal oxide nanomaterials: synthesis and electrochemical applications, Prog. Mater. Sci., 2019, 106, 100574 CrossRef CAS
.
- S. Wang and X. Wang, Microwave-assisted one-pot synthesis of metal/metal oxide nanoparticles on graphene and their electrochemical applications, Electrochim. Acta, 2011, 56, 3338–3344 CrossRef CAS
.
- S. Yan, K. Abhilash, L. Tang, M. Yang, Y. Ma, Q. Xia, Q. Guo and H. Xia, Research advances of amorphous metal oxides in electrochemical energy storage and conversion, Small, 2019, 15, 1804371 CrossRef PubMed
.
- A. Huang, Y. He, Y. Zhou, Y. Zhou, Y. Yang, J. Zhang, L. Luo, Q. Mao, D. Hou and J. Yang, A review of recent applications of porous metals and metal oxide in energy storage, sensing and catalysis, J. Mater. Sci., 2019, 54, 949–973 CrossRef CAS
.
- F.-t. Li, J. Ran, M. Jaroniec and S. Z. Qiao, Solution combustion synthesis of metal oxide nanomaterials for energy storage and conversion, Nanoscale, 2015, 7, 17590–17610 RSC
.
- X. Yu, T. J. Marks and A. Facchetti, Metal oxides for optoelectronic applications, Nat. Mater., 2016, 15, 383–396 CrossRef CAS
.
- M. Kim, B.-H. Kwon, C. W. Joo, M. S. Cho, H. Jang, Y. j. Kim, H. Cho, D. Y. Jeon, E. N. Cho and Y. S. Jung, Metal oxide charge transfer complex for effective energy band tailoring in multilayer optoelectronics, Nat. Commun., 2022, 13, 75 CrossRef CAS PubMed
.
- D. Nunes, A. Pimentel, A. Gonçalves, S. Pereira, R. Branquinho, P. Barquinha, E. Fortunato and R. Martins, Metal oxide nanostructures for sensor applications, Semicond. Sci. Technol., 2019, 34, 043001 CrossRef CAS
.
- H. Khamh, E. Sachet, K. Kelly, J.-P. Maria and S. Franzen, As good as gold and better: conducting metal oxide materials for mid-infrared plasmonic applications, J. Mater. Chem. C, 2018, 6, 8326–8342 RSC
.
- Q. Liu, A. Zhang, R. Wang, Q. Zhang and D. Cui, A review on metal-and metal oxide-based nanozymes: properties, mechanisms, and applications, Nano-Micro Lett., 2021, 13, 1–53 CrossRef
.
-
P. A. Cox, Transition Metal Oxides: An Introduction to Their Electronic Structure and Properties, Oxford University Press, 2010 Search PubMed
.
- J. M. George, A. Antony and B. Mathew, Metal oxide nanoparticles in electrochemical sensing and biosensing: a review, Microchim. Acta, 2018, 185, 1–26 CrossRef CAS
.
- M. Hua, S. Zhang, B. Pan, W. Zhang, L. Lv and Q. Zhang, Heavy metal removal from water/wastewater by nanosized metal oxides: a review, J. Hazard. Mater., 2012, 211, 317–331 CrossRef
.
- L. Manjakkal, D. Szwagierczak and R. Dahiya, Metal oxides based electrochemical pH sensors: current progress and future perspectives, Prog. Mater. Sci., 2020, 109, 100635 CrossRef CAS
.
- A. S. Agnihotri, A. Varghese and M. Nidhin, Transition metal oxides in electrochemical and bio sensing: a state-of-art review, Appl. Surf. Sci. Adv., 2021, 4, 100072 CrossRef
.
- J. Chen, Q. Liu, X.-Z. Song, S.-Y. Zhou, B. Li, L.-F. Xie, G.-C. Yang, C.-Y. Cao, S. Lu and D. Zhang, Effect of multi-type oxides on the catalytic pyrolysis behaviors of 5-amino-1H-tetrazole, Fuel, 2023, 331, 125736 CrossRef CAS
.
- L. Rassaei, F. Marken, M. Sillanpää, M. Amiri, C. M. Cirtiu and M. Sillanpää, Nanoparticles in electrochemical sensors for environmental monitoring, TrAC, Trends Anal. Chem., 2011, 30, 1704–1715 CrossRef CAS
.
- P. Kofstad, Defects and transport properties of metal oxides, Oxid. Met., 1995, 44, 3–27 CrossRef CAS
.
- M. Tamura, K.-i. Shimizu and A. Satsuma, Comprehensive IR study on acid/base properties of metal oxides, Appl. Catal., A, 2012, 433, 135–145 CrossRef
.
- P. Lauriol-Garbey, G. Postole, S. Loridant, A. Auroux, V. Belliere-Baca, P. Rey and J. Millet, Acid–base properties of niobium-zirconium mixed oxide catalysts for glycerol dehydration by calorimetric and catalytic investigation, Appl. Catal., B, 2011, 106, 94–102 CAS
.
- S. D. Bukkitgar, S. Kumar, S. Singh, V. Singh, K. R. Reddy, V. Sadhu, G. B. Bagihalli, N. P. Shetti, C. V. Reddy and K. Ravindranadh, Functional nanostructured metal oxides and its hybrid electrodes-recent advancements in electrochemical biosensing applications, Microchem. J., 2020, 159, 105522 CrossRef CAS
.
- J. Gong, Z. Guan and D. Song, Biosensor based on acetylcholinesterase immobilized onto layered double hydroxides for flow injection/amperometric detection of organophosphate pesticides, Biosens. Bioelectron., 2013, 39, 320–323 CrossRef CAS PubMed
.
- T. Kokulnathan, T.-J. Wang, F. Ahmed and N. Arshi, Fabrication of flower-like nickel cobalt-layered double hydroxide for electrochemical detection of carbendazim, Surf. Interfaces, 2023, 36, 102570 CrossRef CAS
.
- K. Jangid, R. P. Sahu, S. Sakib, I. Zhitomirsky and I. K. Puri, Surface-Modified Metal Oxides for Ultrasensitive Electrochemical Detection of Organophosphates, Heavy Metals, and Nutrients, ACS Appl. Nano Mater., 2022, 5, 17183–17193 CrossRef CAS
.
- E. P. o. F. Additives and N. S. a. t. Food, Scientific opinion on the re-evaluation of iron oxides and hydroxides (E 172) as food additives, EFSA J., 2015, 13, 4317 Search PubMed
.
- E. Ganasan, H. Mohd Yusoff, A. A. Azmi, P. W. Chia, S. S. Lam, S.-Y. Kan, R. K. Liew, K. Venkateswarlu and C. K. Teo, Food additives for the synthesis of metal nanoparticles: a review, Environ. Chem. Lett., 2023, 21, 525–538 CrossRef CAS
.
- M.-H. Ropers, H. Terrisse, M. Mercier-Bonin and B. Humbert, Titanium dioxide as food additive, Appl. Titanium Dioxide, 2017, 10 DOI:10.5772/intechopen.68883
.
- M. Mutsuga, K. Sato, Y. Hirahara and Y. Kawamura, Analytical methods for SiO2 and other inorganic oxides in titanium dioxide or certain silicates for food additive specifications, Food Addit. Contam., 2011, 28, 423–427 CrossRef CAS PubMed
.
- Y. Wang, L. Yuan, C. Yao, L. Ding, C. Li, J. Fang, K. Sui, Y. Liu and M. Wu, A combined toxicity study of zinc oxide nanoparticles and vitamin C in food additives, Nanoscale, 2014, 6, 15333–15342 RSC
.
-
S. A. Akarsu and A. D. Ömür, Nanoparticles as Food Additives and their Possible Effects on Male Reproductive Systems, Nanotechnology in Reproduction, 2023, p. 13 Search PubMed
.
- C. V. Garcia, G. H. Shin and J. T. Kim, Metal oxide-based nanocomposites in food packaging: applications, migration, and regulations, Trends Food Sci. Technol., 2018, 82, 21–31 CrossRef CAS
.
- G. Busca, Spectroscopic characterization of the acid properties of metal oxide catalysts, Catal. Today, 1998, 41, 191–206 CrossRef CAS
.
- I. E. Wachs, Recent conceptual advances in the catalysis science of mixed metal oxide catalytic materials, Catal. Today, 2005, 100, 79–94 CrossRef CAS
.
- S. Ramesh, K. Karuppasamy, D. Vikraman, E. Kim, L. Sanjeeb, Y.-J. Lee, H.-S. Kim, J.-H. Kim and H. S. Kim, Hierarchical Co3O4 decorated nitrogen-doped graphene oxide nanosheets for energy storage and gas sensing applications, J. Ind. Eng. Chem., 2021, 101, 253–261 CrossRef CAS
.
- T.-W. Chen, T.-W. Tseng, J. Yu and R. Yu, A Facile Synthesis of the Nickel Oxide Nanoparticles for the Effective Electrochemical Detection of Hydrogen peroxide in Contact Lens Solution, Int. J. Electrochem. Sci., 2020, 15, 8181–8189 CrossRef
.
- H. Chen and S. Yang, Hierarchical nanostructures of metal oxides for enhancing charge separation and transport in photoelectrochemical solar energy conversion systems, Nanoscale Horiz., 2016, 1, 96–108 RSC
.
- X. Fan, P. Wei, G. Li, M. Li, L. Lan, Q. Hao and T. Qiu, Manipulating hot-electron injection in metal oxide heterojunction array for ultrasensitive surface-enhanced Raman scattering, ACS Appl. Mater. Interfaces, 2021, 13, 51618–51627 CrossRef CAS
.
- X. Tian, L. Liu, Y. Li, C. Yang, Z. Zhou, Y. Nie and Y. Wang, Nonenzymatic electrochemical sensor based on CuO-TiO2 for sensitive and selective detection of methyl parathion pesticide in ground water, Sens. Actuators, B, 2018, 256, 135–142 CrossRef CAS
.
- A. K. Ravi, N. Punnakkal, S. P. Vasu, B. G. Nair and S. B. TG, Manganese dioxide based electrochemical sensor for the detection of nitro-group containing organophosphates in vegetables and drinking water samples, J. Electroanal. Chem., 2020, 859, 113841 CrossRef CAS
.
-
M. Milenković, S. Jevtić, B. Laban, B. Petković and A. Jokić, Nano-Fe2O3 particles as voltammetric signal amplifiers in sensing of heavy metals and pesticides, 2023 Search PubMed.
- L. Li, M. Fan, R. C. Brown, J. Van Leeuwen, J. Wang, W. Wang, Y. Song and P. Zhang, Synthesis, properties, and environmental applications of nanoscale iron-based materials: a review, Crit. Rev. Environ. Sci. Technol., 2006, 36, 405–431 CrossRef CAS
.
- Y. Ju-Nam and J. R. Lead, Manufactured nanoparticles: an overview of their chemistry, interactions and potential environmental implications, Sci. Total Environ., 2008, 400, 396–414 CrossRef CAS
.
- B. Thirumalraj, A. Krishnapandi, S.-M. Chen, S. MSP and H. Choe, Rational design and interlayer effect of dysprosium-stannate nanoplatelets incorporated graphene oxide: a versatile and competent electrocatalyst for toxic carbamate pesticide detection in vegetables, ACS Sustain. Chem. Eng., 2020, 8, 17882–17892 CrossRef CAS
.
- M. Ganesan, R. K. Devi, A.-H. Liao, K.-Y. Lee, G. Gopalakrishnan and H.-C. Chuang, 3D-flower-like porous neodymium molybdate nanostructure for trace level detection of organophosphorus pesticide in food samples, Food Chem., 2022, 396, 133722 CrossRef CAS PubMed
.
- T. Kokulnathan, E. A. Kumar and T.-J. Wang, Synthesis of two-dimensional nanosheet like samarium molybdate with abundant active sites: real-time carbendazimin analysis in environmental samples, Microchem. J., 2020, 158, 105227 CrossRef CAS
.
- G. M. Nair, T. Sajini and B. Mathew, Advanced green approaches for metal and metal oxide nanoparticles synthesis and their environmental applications, Talanta Open, 2022, 5, 100080 CrossRef
.
- S. Maheshwaran, M. Akilarasan, S.-M. Chen, E. Tamilalagan, E. Keerthiga, A. A. Alothman, K. N. Alqahtani and P.-S. Ganesh, Synthesis of nickel-doped ceria nanospheres for in situ profiling of warfarin sodium in biological media, Bioelectrochemistry, 2022, 146, 108166 CrossRef CAS
.
- B. Arumugam, S. Palanisamy, G. Janagaraj, S. K. Ramaraj, M. Chiesa and C. Subramaianan, Real-time detection of 2, 4, 6-trichlorophenol in environmental samples using Fe3O4 nanospheres decorated graphitic carbon nitride nanosheets, Surf. Interfaces, 2023, 102930 CrossRef CAS
.
- C. Yu, L. Gou, X. Zhou, N. Bao and H. Gu, Chitosan–Fe3O4 nanocomposite based electrochemical sensors for the determination of bisphenol A, Electrochim. Acta, 2011, 56, 9056–9063 CrossRef CAS
.
- A. Muthumariappan, K. Sakthivel, S.-M. Chen, T.-W. Chen, A. M. Elgorban, M. S. Elshikh and N. Marraiki, Evaluating an effective electrocatalyst for the rapid determination of triptan drug (Maxalt™) from (mono and binary) transition metal (Co, Mn, CoMn, MnCo) oxides via electrochemical approaches, New J. Chem., 2020, 44, 605–613 RSC
.
- M. Daizy, M. Ali, M. Bacchu, M. A. S. Aly and M. Khan, ZnO hollow spheres arrayed molecularly-printed-polymer based selective electrochemical sensor for methyl-parathion pesticide detection, Environ. Technol. Innovation, 2021, 24, 101847 CrossRef CAS
.
- R. Li, T. Zhe, F. Bai, Z. Xu, M. Li, T. Bu, F. Li, H. Fang, L. Wang and X. Lü, Hierarchical SnO2 nanoparticles designed based on in situ derivatization strategy for rapid and sensitive imidacloprid detection, Microchem. J., 2023, 190, 108691 CrossRef CAS
.
- Z. Arham, A. K. Ramli, M. Natsir and M. Nurdin, Photoelectrode Properties Based on TiO2 Photocatalyst Doped Non-Metallic Agent as Initial Model for Detection and Degradation of Pesticide Pollutants, Environments, 2023, 3, 4 Search PubMed
.
- S. J. Bao, C. M. Li, J. F. Zang, X. Q. Cui, Y. Qiao and J. Guo, New nanostructured TiO2 for direct electrochemistry and glucose sensor applications, Adv. Funct. Mater., 2008, 18, 591–599 CrossRef CAS
.
- W. Chen, S. Li, J. Wang, K. Sun and Y. Si, Metal and metal-oxide nanozymes: bioenzymatic characteristics, catalytic mechanism, and eco-environmental applications, Nanoscale, 2019, 11, 15783–15793 RSC
.
- S. Sawan, R. Maalouf, A. Errachid and N. Jaffrezic-Renault, Metal and metal oxide nanoparticles in the voltammetric detection of heavy metals: a review, TrAC, Trends Anal. Chem., 2020, 131, 116014 CrossRef CAS
.
- G. Ziyatdinova, A. Zhupanova and H. Budnikov, Electrochemical sensors for the simultaneous detection of phenolic antioxidants, J. Anal. Chem., 2022, 77, 155–172 CrossRef CAS
.
-
C. Prasse and T. Ternes, Removal of organic and inorganic pollutants and pathogens from wastewater and drinking water using nanoparticles–a review, Nanoparticles in the Water Cycle: Properties, Analysis and Environmental Relevance, 2010, pp. 55–79 Search PubMed
.
- Y. Yang, H. Tu, A. Zhang, D. Du and Y. Lin, Preparation and characterization of Au–ZrO2–SiO2 nanocomposite spheres and their application in enrichment and detection of organophosphorus agents, J. Mater. Chem., 2012, 22, 4977–4981 RSC
.
- W. Huang, H. Fang, S. Zhang and H. Yu, Optimised green synthesis of copper oxide nanoparticles and their antifungal activity, Micro Nano Lett., 2021, 16, 374–380 CrossRef CAS
.
- P. F. P. Barbosa, E. G. Vieira, L. R. Cumba, L. L. Paim, A. P. R. Nakamura, R. D. A. Andrade and D. R. do Carmo, Voltammetric techniques for pesticides and herbicides detection-an overview, Int. J. Electrochem. Sci., 2019, 14, 3418–3433 CrossRef CAS
.
-
M. Rani, S. Choudhary, J. Yadav, Keshu and U. Shanker, in Handbook of Green and Sustainable Nanotechnology: Fundamentals, Developments and Applications, Springer, 2022, pp. 1–26 Search PubMed
.
- M. Jameel, M. Shoeb, M. T. Khan, R. Ullah, M. Mobin, M. K. Farooqi and S. M. Adnan, Enhanced insecticidal activity of thiamethoxam by zinc oxide nanoparticles: a novel nanotechnology approach for pest control, ACS Omega, 2020, 5, 1607–1615 CrossRef CAS
.
- S. Abinaya and H. P. Kavitha, Magnesium Oxide Nanoparticles: Effective Antilarvicidal and Antibacterial Agents, ACS Omega, 2023, 8, 5225 CrossRef PubMed
.
- M. Anu Prathap, B. Kaur and R. Srivastava, Electrochemical sensor platforms based on nanostructured metal oxides, and zeolite-based materials, Chem. Rec., 2019, 19, 883–907 CrossRef CAS
.
-
R. Viter and I. Iatsunskyi, in Nanomaterials Design for Sensing Applications, Elsevier, 2019, pp. 41–91 Search PubMed
.
- B. Maleki, M. Baghayeri, M. Ghanei-Motlagh, F. M. Zonoz, A. Amiri, F. Hajizadeh, A. Hosseinifar and E. Esmaeilnezhad, Polyamidoamine dendrimer functionalized iron oxide nanoparticles for simultaneous electrochemical detection of Pb2+ and Cd2+ ions in environmental waters, Measurement, 2019, 140, 81–88 CrossRef
.
-
H. A. Murthy, A. N. Wagassa, C. Ravikumar and H. Nagaswarupa, in Functionalized Nanomaterial-Based Electrochemical Sensors, Elsevier, 2022, pp. 369–392 Search PubMed
.
- A. Walcarius, Mesoporous materials-based electrochemical sensors, Electroanalysis, 2015, 27, 1303–1340 CrossRef CAS
.
- R. Bhattacharjee, S. Tanaka, S. Moriam, M. K. Masud, J. Lin, S. M. Alshehri, T. Ahamad, R. R. Salunkhe, N.-T. Nguyen and Y. Yamauchi, Porous nanozymes: the peroxidase-mimetic activity of mesoporous iron oxide for the colorimetric and electrochemical detection of global DNA methylation, J. Mater. Chem. B, 2018, 6, 4783–4791 RSC
.
- V. Sudha, G. Murugadoss and R. Thangamuthu, Structural and morphological tuning of Cu-based metal oxide nanoparticles by a facile chemical method and highly electrochemical sensing of sulphite, Sci. Rep., 2021, 11, 3413 CrossRef CAS PubMed
.
- G.-Q. Yuan, H.-F. Jiang, C. Lin and S.-J. Liao, Shape-and size-controlled electrochemical synthesis of cupric oxide nanocrystals, J. Cryst. Growth, 2007, 303, 400–406 CrossRef CAS
.
- D. Vasu, A. K. Keyan, S. Sakthinathan, C.-L. Yu, B.-Z. Hsu, T.-W. Chiu and J. Wu, Enhanced electrocatalytic activity of non-metal-doped transition metal oxides for an electrochemical detection of furazolidone, Electrocatalysis, 2022, 13, 348–360 CrossRef CAS
.
- Y. Kim, S. Yang, Y. Kang, B.-K. Kim and H. Lee, Transition metal doped Sb@SnO2 nanoparticles for photochemical and electrochemical oxidation of cysteine, Sci. Rep., 2018, 8, 12348 CrossRef
.
- A. Anusiya, B. Jansi Rani, G. Ravi, R. Yuvakkumar, S. Ravichandran, V. Ganesh and B. Saravanakumar, Transition-metal element (Ni, Co)-doped MgO microflowers for electrochemical biosensor applications, JOM, 2019, 71, 279–284 CrossRef CAS
.
- S. Maheshwaran, S. Kogularasu, S.-M. Chen, W.-H. Chen, Y.-Y. Lee and G.-P. Chang-Chien, Ultra-trace detection of sulfathiazole, an anti-infective agent and environmental contaminant, using electrochemical sensing with holmium vanadate-graphene oxide nanocomposites, J. Taiwan Inst. Chem. Eng., 2023, 153, 105233 CrossRef CAS
.
- N. A. Bohari, S. Siddiquee, S. Saallah, M. Misson and S. E. Arshad, Optimization and analytical behavior of electrochemical sensors based on the modification of indium tin oxide (ITO) using PANI/MWCNTs/AuNPs for mercury detection, Sensors, 2020, 20, 6502 CrossRef CAS
.
- S. Maheshwaran, R. Balaji, S.-M. Chen, E. Tamilalagan, N. Chandrasekar, S. Ethiraj and M. S. Samuel, Construction of graphene oxide wrapped gadolinium vanadate nanocomposites as an efficient electrocatalyst for the amperometric sensing of sulfadiazine, Process Saf. Environ. Prot., 2022, 168, 77–87 CrossRef CAS
.
- S. Maheshwaran, E. Tamilalagan, S.-M. Chen, M. Akilarasan, Y.-F. Huang, N. AlMasoud, K. M. Abualnaja and M. Ouladsmne, Rationally designed f-MWCNT-coated bismuth molybdate (f-MWCNT@BMO) nanocomposites for the voltammetric detection of biomolecule dopamine in biological samples, Microchim. Acta, 2021, 188, 315 CrossRef CAS PubMed
.
- M. Akilarasan, E. Tamilalagan, S.-M. Chen, S. Maheshwaran, C.-H. Fan, M. A. Habila and M. Sillanpää, Rational synthesis of rare-earth lanthanum molybdate covered reduced graphene oxide nanocomposites for the voltammetric detection of moxifloxacin hydrochloride, Bioelectrochemistry, 2022, 146, 108145 CrossRef CAS PubMed
.
- S. Maheshwaran, R. Balaji, S.-M. Chen, Y.-C. Liao, N. Chandrasekar, S. Ethiraj and M. S. Samuel, Fabrication of 2D–0D Ti3AlC2@SmVO4 heterojunction nanocomposites for ultrasensitive electrochemical detection of sulfathiazole in environmental samples, J. Environ. Chem. Eng., 2022, 10, 108956 CrossRef CAS
.
- Q. He, B. Wang, J. Liang, J. Liu, B. Liang, G. Li, Y. Long, G. Zhang and H. Liu, Research on the construction of portable electrochemical sensors for environmental compounds quality monitoring, Mater. Today Adv., 2023, 17, 100340 CrossRef CAS
.
- J. W. Wong, J. Wang, J. S. Chang, W. Chow, R. Carlson, Ł. Rajski, A. R. Fernández-Alba, R. Self, W. K. Cooke and C. M. Lock, Multilaboratory Collaborative study of a nontarget data acquisition for target analysis (nDATA) workflow using liquid chromatography-high-resolution accurate mass spectrometry for pesticide screening in fruits and vegetables, J. Agric. Food Chem., 2021, 69, 13200–13216 CrossRef CAS
.
- C. Zhang, X. Liang, Y. Lu, H. Li and X. Xu, Performance of CuAl-LDH/Gr nanocomposite-based electrochemical sensor with regard to trace glyphosate detection in water, Sensors, 2020, 20, 4146 CrossRef CAS
.
- A. Cioffi, M. Mancini, V. Gioia and S. Cinti, Office paper-based electrochemical strips for organophosphorus pesticide monitoring in agricultural soil, Environ. Sci. Technol., 2021, 55, 8859–8865 CrossRef CAS PubMed
.
- G. B. Subbaiah, K. V. Ratnam, S. Janardhan, K. Shiprath, H. Manjunatha, M. Ramesha, N. K. Prasad, S. Ramesh and T. A. Babu, Metal and Metal Oxide Based Advanced Ceramics for Electrochemical Biosensors-A Short Review, Front. Mater., 2021, 8, 682025 CrossRef
.
- X.-Y. Yu, Z.-G. Liu and X.-J. Huang, Nanostructured metal oxides/hydroxides-based electrochemical sensor for monitoring environmental micropollutants, Trends Environ. Anal. Chem., 2014, 3, 28–35 CrossRef
.
- R. Li, M. Shang, T. Zhe, M. Li, F. Bai, Z. Xu, T. Bu, F. Li and L. Wang, Sn/MoC@NC hollow nanospheres as Schottky catalyst for highly sensitive electrochemical detection of methyl parathion, J. Hazard. Mater., 2023, 130777 CrossRef CAS
.
- M. Guo, F. Li, Q. Ran, G. Zhu, Y. Liu, J. Han, G. Wang and H. Zhao, Facile fabrication of Zr-based metal–organic framework/Ketjen black-carbon nanotubes composite sensor for highly sensitive detection of methyl parathion, Microchem. J., 2023, 190, 108709 CrossRef CAS
.
- R. Karthik, J. V. Kumar, S.-M. Chen, T. Kokulnathan, T.-W. Chen, S. Sakthinathan, T.-W. Chiu and V. Muthuraj, Development of novel 3D flower-like praseodymium molybdate decorated reduced graphene oxide: an efficient and selective electrocatalyst for the detection of acetylcholinesterase inhibitor methyl parathion, Sens. Actuators, B, 2018, 270, 353–361 CrossRef CAS
.
- I. Suresh, S. Selvaraj, N. Nesakumar, J. B. B. Rayappan and A. J. Kulandaiswamy, Nanomaterials based non-enzymatic electrochemical and optical sensors for the detection of carbendazim: a review, Trends Environ. Anal. Chem., 2021, 31, e00137 CrossRef CAS
.
- P. Noyrod, O. Chailapakul, W. Wonsawat and S. Chuanuwatanakul, The simultaneous determination of isoproturon and carbendazim pesticides by single drop analysis using a graphene-based electrochemical sensor, J. Electroanal. Chem., 2014, 719, 54–59 CrossRef CAS
.
- Y. Li, X. Chen, H. Ren, X. Li, S. Chen and B.-C. Ye, A novel electrochemical sensor based on molecularly imprinted polymer-modified C-ZIF67@Ni for highly sensitive and selective determination of carbendazim, Talanta, 2022, 237, 122909 CrossRef CAS PubMed
.
- P. Santhoshkumar, B. Thirumalraj, B. Sriram, K. Karuppasamy, D. Vikraman, A. Kathalingam, H. Choe and H.-S. Kim, Mesoporous SnSe2-grafted N-doped carbon composites with integrated flaky structure for electrochemical sensing of carbendazim, Ceram. Int., 2022, 48, 16023–16032 CrossRef CAS
.
- X. B. Joseph, J. N. Baby, S.-F. Wang, B. Sriram and M. George, Interfacial superassembly of Mo2C@NiMn-LDH frameworks for electrochemical monitoring of carbendazim fungicide, ACS Sustain. Chem. Eng., 2021, 9, 14900–14910 CrossRef CAS
.
- J. A. Hondred, J. C. Breger, N. J. Alves, S. A. Trammell, S. A. Walper, I. L. Medintz and J. C. Claussen, Printed graphene electrochemical biosensors fabricated by inkjet maskless lithography for rapid and sensitive detection of organophosphates, ACS Appl. Mater. Interfaces, 2018, 10, 11125–11134 CrossRef CAS
.
- G. G. Pimenta, M. E. de Queiroz, R. P. Victor, L. M. Noronha, A. A. Neves, A. F. d. Oliveira and F. F. Heleno, DLLME-GC/ECD Method for the Residual Analysis of Parathion-Methyl and its Application in the Study of the UV-Photodegradation Process, J. Braz. Chem. Soc., 2017, 28, 2045–2053 CAS
.
- P. K. Gopi, D. B. Ngo, S.-M. Chen, C. H. Ravikumar and W. Surareungchai, High-performance electrochemical sensing of hazardous pesticide paraoxon using BiVO4 nano dendrites equipped catalytic strips, Chemosphere, 2022, 288, 132511 CrossRef CAS PubMed
.
- Y. Hu, D. Li, Y. Zheng, W. Chen, Y. He, Y. Shao, X. Fu and G. Xiao, BiVO4/TiO2 nanocrystalline heterostructure: a wide spectrum responsive photocatalyst towards the highly efficient decomposition of gaseous benzene, Appl. Catal., B, 2011, 104, 30–36 CrossRef CAS
.
- X. Zhang, Z. Ai, F. Jia, L. Zhang, X. Fan and Z. Zou, Selective synthesis and visible-light photocatalytic activities of BiVO4 with different crystalline phases, Mater. Chem. Phys., 2007, 103, 162–167 CrossRef CAS
.
- B. Mutharani, P. Ranganathan, S.-M. Chen and C. Karuppiah, Enzyme-free electrochemical detection of nanomolar levels of the organophosphorus pesticide paraoxon-ethyl by using a poly(N-isopropyl acrylamide)-chitosan microgel decorated with palladium nanoparticles, Microchim. Acta, 2019, 186, 1–11 CrossRef
.
- A. A. Ensafi, R. Noroozi, N. Zandi and B. Rezaei, Cerium (IV) oxide decorated on reduced graphene oxide, a selective and sensitive electrochemical sensor for fenitrothion determination, Sens. Actuators, B, 2017, 245, 980–987 CrossRef CAS
.
- A. Kumaravel and M. Chandrasekaran, A biocompatible nano TiO2/Nafion composite modified glassy carbon electrode for the detection of fenitrothion, J. Electroanal. Chem., 2011, 650, 163–170 CrossRef CAS
.
- J. Vinoth Kumar, R. Karthik, S.-M. Chen, K. Natarajan, C. Karuppiah, C.-C. Yang and V. Muthuraj, 3D flower-like gadolinium molybdate catalyst for efficient detection and degradation of organophosphate pesticide (fenitrothion), ACS Appl. Mater. Interfaces, 2018, 10, 15652–15664 CrossRef CAS
.
- M. Ganesan, R. K. Devi, S.-M. Chen and S. K. Ravi, Sustainable synthesis of hierarchical dysprosium vanadate 3D-micro flowers for electrochemical evaluation of organophosphate pesticide in food samples, Chem. Eng. J., 2023, 466, 143111 CrossRef CAS
.
- J. A. Buledi, A. R. Solangi, S. Q. Memon, S. I. Haider, S. Ameen, N. H. Khand, A. Bhatti and N. Qambrani, Nonenzymatic electrochemical detection of 2, 4, 6-trichlorophenol using CuO/Nafion/GCE: a practical sensor for environmental toxicants, Langmuir, 2021, 37, 3214–3222 CrossRef CAS
.
- W. Zheng, R. Su, X. Lin and J. Liu, Nanochannel array modified three-dimensional graphene electrode for sensitive electrochemical detection of 2, 4, 6-trichlorophenol and prochloraz, Front. Chem., 2022, 10, 954802 CrossRef CAS PubMed
.
- M. Akilarasan, E. Tamilalagan, S.-M. Chen, S. Maheshwaran, T.-W. Chen, A. M. Al-Mohaimeed, W. A. Al-Onazi and M. S. Elshikh, An eco-friendly low-temperature synthetic
approach towards micro-pebble-structured GO@SrTiO3 nanocomposites for the detection of 2, 4, 6-trichlorophenol in environmental samples, Microchim. Acta, 2021, 188, 1–10 CrossRef
.
- L. Wang, Y. Liu, R. Yang, J. Li and L. Qu, AgNPs–PDA–GR nanocomposites-based molecularly imprinted electrochemical sensor for highly recognition of 2, 4, 6-trichlorophenol, Microchem. J., 2020, 159, 105567 CrossRef
.
- R. K. Devi, M. Ganesan, T.-W. Chen, S.-M. Chen, R. A. Rasheed, W. A. Al-Onazi, M. S. Elshikh, X. Liu and J. Yu, Hexagonal nanosheets of pyrrochlore-type lanthanum stannate for sensitive detection of chlorinated pesticide in food and environmental samples, Food Chem., 2023, 404, 134516 CrossRef
.
- J.-Y. Yang, Y.-C. Su and X.-Y. Liu, Hydrothermal synthesis, characterization and optical properties of La2Sn2O7: Eu3+ micro-octahedra, Trans. Nonferrous Met. Soc. China, 2011, 21, 535–543 CrossRef CAS
.
- K.-Y. Hwa, A. Ganguly, A. Santhan and T. S. K. Sharma, Vanadium selenide decorated reduced graphene oxide nanocomposite: a co-active catalyst for the detection of 2, 4, 6–trichlorophenol, Chemosphere, 2021, 282, 130874 CrossRef CAS PubMed
.
- X. Zhu, J. Liu, Z. Zhang, N. Lu, X. Yuan and D. Wu, Green synthesis of a bromocresol purple/graphene composite and its application in electrochemical determination of 2, 4, 6-trichlorophenol, Anal. Methods, 2015, 7, 3178–3184 RSC
.
- X. Zheng, S. Liu, X. Hua, F. Xia, D. Tian and C. Zhou, Highly sensitive detection of 2, 4, 6-trichlorophenol based on HS-β-cyclodextrin/gold nanoparticles composites modified indium tin oxide electrode, Electrochim. Acta, 2015, 167, 372–378 CrossRef CAS
.
- T. Zhang, L. Wang, C. Gao, C. Zhao, Y. Wang and J. Wang, Hemin immobilized into metal–organic frameworks as an electrochemical biosensor for 2, 4, 6-trichlorophenol, Nanotechnology, 2018, 29, 074003 CrossRef
.
- A. Ogram, Y.-P. Duan, S. Trabue, X. Feng, H. Castro and L.-T. Ou, Carbofuran degradation mediated by three related plasmid systems, FEMS Microbiol. Ecol., 2000, 32, 197–203 CrossRef CAS
.
- R. F. Turco and A. Konopka, Biodegradation of carbofuran in enhanced and non-enhanced soils, Soil Biol. Biochem., 1990, 22, 195–201 CrossRef CAS
.
- C.-C. Yu, G. M. Booth, D. J. Hansen and J. Larsen, Fate of carbofuran in a model ecosystem, J. Agric. Food Chem., 1974, 22, 431–434 CrossRef CAS PubMed
.
- V. Mariyappan, M. Keerthi and S.-M. Chen, Highly selective electrochemical sensor based on gadolinium sulfide rod-embedded RGO for the sensing of carbofuran, J. Agric. Food Chem., 2021, 69, 2679–2688 CrossRef CAS PubMed
.
- P. Rajiv, R. Manikandan, S. Sangeetha, P. Vanathi and S. Dhanasekaran, Fabrication of biogenic iron oxide and their efficiency to detect carbofuran in vegetable samples, Inorg. Chem. Commun., 2022, 142, 109649 CrossRef CAS
.
- Z. Zeng, X. Wang, T. Yang, Y. Li, X. Liu, P. Zhang, B. Feng and T. Qing, Transition metal-doped germanium oxide nanozyme with enhanced enzyme-like activity for rapid detection of pesticide residues in water samples, Anal. Chim. Acta, 2023, 1245, 340861 CrossRef CAS
.
- Z. Zeng, X. Wang, T. Yang, Y. Li, X. Liu, P. Zhang, B. Feng and T. Qing, Transition metal-doped germanium oxide nanozyme with enhanced enzyme-like activity for rapid detection of pesticide residues in water samples, Anal. Chim. Acta, 2023, 340861 CrossRef CAS
.
- K. Prabhu, S. J. Malode, R. M. Kulkarni and N. P. Shetti, Electro-sensing base for hazardous pesticide 2, 4-DCP and its quantification in real samples at ZnO@Cu core-shell nanoparticles in the presence of cationic surfactant, Mater. Chem. Phys., 2022, 278, 125705 CrossRef CAS
.
- J. Gong, X. Miao, H. Wan and D. Song, Facile synthesis of zirconia nanoparticles-decorated graphene hybrid nanosheets for an enzymeless methyl parathion sensor, Sens. Actuators, B, 2012, 162, 341–347 CrossRef CAS
.
- H. Mahmoudi-Moghaddam, H. A. Javar and Z. Garkani-Nejad, Fabrication of platinum-doped NiCo2O4 nanograss modified electrode for determination of carbendazim, Food Chem., 2022, 383, 132398 CrossRef CAS
.
- M. Narayanan, A. Amalraj and P. Perumal, A copper oxide functionalized neodymium oxide hybrid nanostructure interconnected with modified glassy carbon electrode (CuO–Nd2O3/GCE) for electrochemical determination of malathion pesticide, J. Mater. Res., 2023, 38, 416–428 CrossRef CAS
.
- T. Kokulnathan, T.-J. Wang, Y.-Y. Wang, V. Suvina and F. Ahmed, Three-dimensional manganese cobaltate: a highly conductive electrocatalyst for paraoxon-ethyl detection, Microchim. Acta, 2022, 189, 315 CrossRef CAS PubMed
.
- R. Sundaresan, V. Mariyappan, T.-W. Chen, S.-M. Chen, N. Karuppusamy and M. Akilarasan, Highly sensitive electrochemical sensor based on perovskite type PrCoO3 for the detection of carbendazim, Int. J. Electrochem. Sci., 2021, 16, 210829 CrossRef CAS
.
- V. Mani, R. Devasenathipathy, S.-M. Chen, T.-Y. Wu and K. Kohilarani, High-performance electrochemical amperometric sensors for the sensitive determination of phenyl urea herbicides diuron and fenuron, Ionics, 2015, 21, 2675–2683 CrossRef CAS
.
- S. Maheshwaran, V. Renganathan, S.-M. Chen, R. Balaji, C. Kao, N. Chandrasekar, S. Ethiraj, M. S. Samuel and M. Govarthanan, Hydrothermally constructed AgWO4-rGO nanocomposites as an electrode enhancer for ultrasensitive electrochemical detection of hazardous herbicide crisquat, Chemosphere, 2022, 299, 134434 CrossRef CAS
.
- P. Hashemi, N. Karimian, H. Khoshsafar, F. Arduini, M. Mesri, A. Afkhami and H. Bagheri, Reduced graphene oxide decorated on Cu/CuO-Ag nanocomposite as a high-performance material for the construction of a non-enzymatic sensor: application to the determination of carbaryl and fenamiphos pesticides, Mater. Sci. Eng., C, 2019, 102, 764–772 CrossRef CAS PubMed
.
- S. Zhao, C. Hou, L. Shao, W. An and W. Cui, Adsorption and in situ photocatalytic synergy degradation of 2, 4-dichlorophenol by three-dimensional graphene hydrogel modified with highly dispersed TiO2 nanoparticles, Appl. Surf. Sci., 2022, 590, 153088 CrossRef CAS
.
- F. Soltani-Nezhad, A. Saljooqi, A. Mostafavi and T. Shamspur, Synthesis of Fe3O4/CdS–ZnS nanostructure and its application for photocatalytic degradation of chlorpyrifos pesticide and brilliant green dye from aqueous solutions, Ecotoxicol. Environ. Saf., 2020, 189, 109886 CrossRef CAS
.
- M. Wang, L. Hou, X. Chen and T. Lin, Homogeneous photoelectrochemical biosensor for sensitive detection of omethoate via ALP-mediated pesticide assay and Bi2S3@Bi2Sn2O7 heterojunction as photoactive material, Anal. Bioanal. Chem., 2022, 414, 7277–7289 CrossRef CAS PubMed
.
- R. Sundaresan, V. Mariyappan, T.-W. Chen, S.-M. Chen, M. Akilarasan, X. Liu and J. Yu, One-dimensional rare-earth tungstate nanostructure encapsulated reduced graphene oxide electrocatalyst-based electrochemical sensor for the detection of organophosphorus pesticide, J. Nanostruct. Chem., 2023, 1–14 Search PubMed
.
- P. Hu, Y. Zhou, A. Qileng, H. Liang, B. Kong, W. Liu and Y. Liu, The Cohesion of Cascade Enzyme Reaction with Metal–Organic Framework Composite for the Sensitive Detection of Malathion and In situ Imaging of Vegetables, Sens. Actuators, B, 2023, 133591 CrossRef CAS
.
- C. B. Marien, T. Cottineau, D. Robert and P. Drogui, TiO2 nanotube arrays: influence of tube length on the photocatalytic degradation of paraquat, Appl. Catal., B, 2016, 194, 1–6 CrossRef CAS
.
- N. Gowthaman, H. N. Lim, V. Balakumar and S. Shankar, Ultrasonic synthesis of CeO2@organic dye nanohybrid: environmentally benign rabid electrochemical sensing platform for carcinogenic pollutant in water samples, Ultrason. Sonochem., 2020, 61, 104828 CrossRef CAS
.
- B. Kulkarni, V. Suvina, K. Pramoda and R. G. Balakrishna, Picomolar, Electrochemical Detection of Paraoxon Ethyl, by Strongly Coordinated NiCo2O4-SWCNT Composite as an Electrode Material, J. Electroanal. Chem., 2023, 117175 CrossRef CAS
.
- P. K. Boruah, G. Darabdhara and M. R. Das, Polydopamine functionalized graphene sheets decorated with magnetic metal oxide nanoparticles as efficient nanozyme for the detection and degradation of harmful triazine pesticides, Chemosphere, 2021, 268, 129328 CrossRef CAS PubMed
.
- G. Xu, D. Huo, C. Hou, Y. Zhao, J. Bao, M. Yang and H. Fa, A regenerative and selective electrochemical aptasensor based on copper oxide nanoflowers-single walled carbon nanotubes nanocomposite for chlorpyrifos detection, Talanta, 2018, 178, 1046–1052 CrossRef CAS
.
- S. J. Malode, N. P. Shetti and K. R. Reddy, Highly sensitive electrochemical assay for selective detection of aminotriazole based on TiO2/poly(CTAB) modified sensor, Environ. Technol. Innovation, 2021, 21, 101222 CrossRef CAS
.
- C. Tian, S. Zhang, H. Wang, C. Chen, Z. Han, M. Chen, Y. Zhu, R. Cui and G. Zhang, Three-dimensional nanoporous copper and reduced graphene oxide composites as enhanced sensing platform for electrochemical detection of carbendazim, J. Electroanal. Chem., 2019, 847, 113243 CrossRef CAS
.
- X. Wu, P. Wang, S. Hou, P. Wu and J. Xue, Fluorescence sensor for facile and visual detection of organophosphorus pesticides using AIE fluorogens-SiO2-MnO2 sandwich nanocomposites, Talanta, 2019, 198, 8–14 CrossRef CAS
.
- W. Xie, Y. Ju, J. Zhang, Y. Yang, Y. Zeng, H. Wang and L. Li, Highly sensitive and specific determination of imidacloprid pesticide by a novel Fe3O4@SiO2@MIPIL fluorescent sensor, Anal. Chim. Acta, 2022, 1195, 339449 CrossRef CAS PubMed
.
- X. Zhai, F. Xu, Y. Li, F. Jun, S. Li, C. Zhang, H. Wang and B. Cao, A highly selective and recyclable sensor for the electroanalysis of phosphothioate pesticides using silver-doped ZnO nanorods arrays, Anal. Chim. Acta, 2021, 1152, 338285 CrossRef CAS PubMed
.
|
This journal is © The Royal Society of Chemistry 2024 |
Click here to see how this site uses Cookies. View our privacy policy here.