Identification and quantification of diffuse groundwater pollution in a mineralised watershed
Received
23rd March 2023
, Accepted 15th January 2024
First published on 31st January 2024
Abstract
A tracer injection and synoptic sampling experiment was carried out in a tributary catchment of the River Tywi (Carmarthenshire, Wales) during a very low streamflow event to locate and quantify sources of groundwater metal pollution from the abandoned Nantymwyn Pb mine. High resolution sampling at 22 stream locations and 12 inflows along a 2 km stretch of the Nant y Bai was deployed. This high spatial resolution sampling technique allowed point and diffuse sources of metal pollution to be identified enabling their contribution to the overall stream load to be quantified. It was found that the highest proportion of filtered Zn load originated from diffuse groundwater sources from the upper mine tailings (43%), and that the highest proportion of filtered Pb load originated from diffuse groundwater sources from the lower mine tailings (40%), with smaller inputs from a field on the River Tywi floodplain. These results demonstrate that tracer dilution and synoptic sampling is a powerful tool to locate and apportion diffuse sources of groundwater metal pollution in small, mineralised watersheds.
Water impact
Abandoned metal mines often pollute nearby watercourses, with harmful impacts on flora, fauna, and potentially humans too. The funding and political will to treat these mines is limited, and targeted remediation is necessary. Locating and quantifying diffuse groundwater and point metal sources will allow improvements to water quality whilst maximising the benefit of any expenditure.
|
Introduction
After the large scale closure of metal and coal mines from the 1900s onwards, abandoned mines have become a major source of metal pollution to rivers globally.1,2 Locating, classifying, and quantifying sources of potentially toxic elements from abandoned metal mines is challenging due to the historical legacy of poorly-mapped mine workings, variations in streamflow and rainfall, and the remote location of mines. Quantification of pollution sources is an essential first step to the successful remediation of polluted mine sites and watercourses.3 Wales, for example, has over 1300 known abandoned metal mines, many abandoned before any legal requirements to map the subterranean workings, let alone plan for any future polluted water outflows causing negative effects on biota downstream.4 Metal pollution from abandoned mines can have a deleterious impact on flora and fauna and can bioaccumulate through the food chain.5–7 Decades of erosion and degradation of surface and subsurface workings have increased the difficulty of managing these sites, which adversely affect over 700 km of river length in western Wales, and cause over 15% of watercourse chemical quality failures in western Wales.8,9
In order to locate source areas of pollutants within a river, a spatial profile of loadings (pollutant concentration multiplied by flow) is required. The tracer dilution and synoptic sampling technique,10 originally developed by the U.S. Geological Survey, has the potential to be used for mine impacted groundwater source apportionment in the more temperate climates of the UK.11,12 The tracer dilution technique is used to calculate streamflow (Q) from the dilution of an injected conservative tracer. As Q values are derived from the downstream changes in tracer concentration, and the tracer has been thoroughly mixed into the river over several days, these flow values are not affected by irregular stream cross-sections, as the velocity-area method would be, and include flow in the hyporheic zone, unlike a weir, or the gulp-injection method.11 Combined with pollutant concentration data collected at the same time and location as the flow data, this method produces high spatial resolution profiles of pollutant loads that can be used to apportion sources of pollution.
Apportionment of mine pollution sources to streams under low flow conditions is important to identify diffuse subterranean sources of metal pollutants and also to consider the potential impacts on river water quality of future low flows, which are predicted to become more extreme and frequent due to climate change.13,14 Failure to consider low and extremely low streamflow events in source apportionment studies and in subsequent mine site remedial activities may result in persistent metal pollution after remediation.15
The aim of this paper is to assess the utility of the tracer dilution and synoptic sampling approach in identifying and quantifying sources of metal pollution, including diffuse groundwater sources, during very low streamflows, using the Nant y Bai stream at the abandoned Nantymwyn mine (Carmarthenshire, Wales) as an exemplar catchment.
Methodology
Study area
One of over 1300 abandoned metal mines in Wales, Nantymwyn Pb mine is located in the upper catchment of the River Tywi, Carmarthenshire (52°5′12′′N; 3°46′20′′W) (Fig. 1), and was mined sporadically from pre-Roman times until abandonment in 1932.16,17 It is located on Bala (Upper Ordovician) shales and grits, with mineral veins carrying galena (PbS) and sphalerite (ZnS) crossing the site in a north-easterly direction.18 These veins along the Abergwesyn Fault have only been worked at Nantymwyn mine, elsewhere in the county they are steeply dipping, with short sections of mineralisation less than a metre wide.19 An automatic rain gauge on a nearby River Tywi flow gauging station received a mean annual rainfall of 1711 mm between 1968–2017.20 An 1830 underground plan of the mine notes “old workings” which were not mapped, and in the 1920s when the mine was undergoing modernisation, maps of the workings were often found to be inaccurate.21,22 The 20 hectares of the site include mine waste dumps of differing metal concentration levels (XRF measurements showed means of 1.53% Pb and 0.18% Zn at the upper part of the site, and 3.59% Pb and 0.33% Zn at the lower part of the site23) and grain size depending on which periods of operation they were formed under. Studies have shown that metals such as Pb from the mine bioaccumulate in fauna,5 and Zn has been ranked the second highest risk water pollutant in the UK out of 71 chemicals.24 The 2.6 km Nant y Bai stream, with a 277 hectare catchment, flows through the mine waste dumps for the last 1.9 km of its reach. The smaller Nant y Mwyn stream is further south and receives flow from two visible mine adits. Mine pollution from these two streams cause the River Tywi to fail Water Framework Directive (WFD) standards for 25 km downstream of the site.8 The River Tywi was noted as being polluted by metal mines in 1861.25 Forty years after mine closure, the South West Wales River Authority26 recorded the Nant y Bai as being fishless and polluted by Pb and Zn. Successor organisations have periodically reported on pollution from the mine and its impact on flora and fauna in the streams and the River Tywi, but there has been no systematic investigation of sources.4,9,27 Nantymwyn Pb mine has been identified as one of the highest priority metal mines in Wales for remediation,4 but this cannot proceed successfully without a clear understanding of the sources of metals across the site. Due to the high spatial resolution stream metal loads possible with the synoptic sampling and tracer injection approach, areas of diffuse groundwater pollution otherwise undetected can be located and quantified, and then considered as part of a whole site remediation plan.11
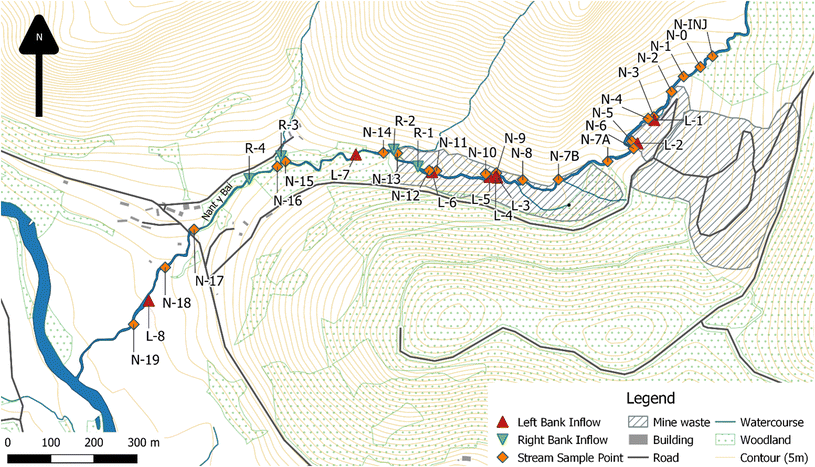 |
| Fig. 1 Nantymwyn Pb mine, stream (N-) and inflow (L-/R-) sample locations.55 | |
Tracer injection and synoptic sampling
In order to collect accurate streamflow and metal loading data, the measured watercourse must have a steady flow state for the duration of the tracer injection and synoptic sampling experiment. Therefore, a summer low flow period was chosen to maximise the chance of constant low flows in the stream.
Following U.S. Geological Survey standard practice,10,28,29 constant-rate tracer injection and synoptic sampling was conducted on the Nant y Bai in July 2019. There are two main advantages of the technique over more traditional methods of streamflow gauging [e.g. velocity-area, gulp injection].11,30 First, as the tracer is injected over a period of days it becomes fully mixed in the stream and therefore captures flow in irregular channel sections and the hyporheic zone. Secondly, as the streamflow is calculated using the tracer concentration in the stream, sampling can be carried out rapidly at each sample site, maximising the number of samples that can be taken and increasing the spatial resolution of sampling. A tracer injection tank was erected at the top of the studied section of the Nant y Bai (point N-INJ on Fig. 1) and filled with approximately 850 L of stream water, to which 35 kg of NaBr was added and thoroughly dissolved. Sodium bromide was selected as the tracer as it is known to behave conservatively in circumneutral waters31 and has been previously used successfully for this purpose in the UK.11 This solution was injected into the stream at a constant rate of 70 mL min−1 for five days using a computer-controlled pump, and terminated at the completion of the synoptic sampling. The injection site (N-INJ in Fig. 1) was above the mine workings as shown by historical aerial photographs. Samples of the solution in the injection tank were taken over the course of the injection period, confirming that the concentration remained within ±1.8% of its starting concentration during the experiment. A TempHion Multi-Parameter Water Quality Sensor with an Ag/AgCl solid-state electrode Br probe32 was used to monitor Br levels in the stream at the end of the studied reach and synoptic sampling began when the tracer concentration had reached a plateau concentration, indicating the Br concentration in the stream was at a steady state. Synoptic samples were taken at 34 sites in the Nant y Bai catchment: at 22 stream sites and 12 inflow sites, as shown in Fig. 1. These sites were selected during an earlier field visit, where six months of spot sampling data showed an increase of metal concentrations in the stream; before and after spoil heaps; and where water was visibly entering the watercourse for example as small tributaries and river bank seeps. For the visible inflows, a sample was taken from the inflow and in the main stream above and below the inflow, the latter a distance downstream that allowed for complete mixing between the two flows.
Synoptic samples were collected in a downstream to upstream direction, to avoid contaminating downstream samples with disturbed sediments and from higher Br concentrations upstream.33 At each synoptic sample point a water sample was taken in a 1 L bottle, following the Environment Agency standard protocol of rinsing the sample bottle with sample site water three times,34 and then taken to a field laboratory for preparation for analysis as soon as practicable in the same hour. At the field laboratory, the sample's pH and temperature were recorded, and three 25 mL vials filled from the larger bottle, again rinsing the vial three times with sample water. One vial was filled with the sample as collected and fixed with HNO3 (for total metal concentrations analysis), one filled with the sample after being filtered through a 0.45 μm membrane and fixed with HNO3 (for filtered metal concentrations analysis), and one sample was filtered through a 0.45 μm membrane (for bromide analysis)35 sample vials were then sealed and stored in a fridge at 4 °C.
Laboratory analysis
Total and filtered concentrations of elements (primarily for Pb, Zn, Cd, and Br, but also for 17 others (Be, Na, Mg, Al, K, Ca, V, Cr, Mn, Fe, Co, Ni, Cu, As, Ag, Sb, and Tl)) were determined by Inductively Coupled Plasma Mass Spectrometry (using an Agilent Technologies ICP MS 7900 with autosampler). Br concentrations were determined additionally by ion chromatography, as Br can have a memory effect on ICP-MS.36 After initial assessment of the total and filtered concentrations of the elements analysed, three elements were selected for source apportionment analysis: Pb, Zn, and Cd, all of which fail WFD Environmental Quality Standards (EQS) from sample site N-3 onwards, and cause WFD EQS failure within the River Tywi for up to 25 km downstream of the site.37
Estimating streamflow
Streamflow was measured at each synoptic sample site by the observed dilution of the injected Br. Streamflow estimation at each site relates the injected tracer to the observed dilution, where Q (L s−1) is streamflow.10,29,38
Q
INJ (mL min−1) |
Injection rate |
C
INJ (μg L−1) |
Concentration of the injected tracer |
C
p (μg L−1) |
Tracer plateau concentration at the synoptic sampling site |
C
B (μg L−1) |
Background tracer concentration in stream water above the injection point |
Loading analysis
The studied stream was divided into 21 segments, demarcated by the 22 stream synoptic sampling sites. Loads L (mg s−1) of Pb, Zn, and Cd were calculated as the product of the streamflow estimate and the metal concentration at the synoptic site, as below:
With metal loads calculated for each synoptic site, the cumulative instream load can be calculated, which is the sum of all the increases in metal loads between each stream segment. Comparing the cumulative instream load to the metal load calculated for the individual or grouped stream segments, highlights locations in the stream where metal attenuation (through chemical reactions) or metal addition (through sources) are occuring.11 Furthermore, the percent contribution of each source can be calculated as:
where L1 and L2 are the cumulative instream load at the upstream and downstream points of a selected stream segment, and Δload is the change in metal load within the segment.11
Q (L s−1) |
Stream discharge |
C
m (mg L−1) |
Concentration of the element |
Stream segment contributions can be combined to show the cumulative impacts of similar areas of pollution, for instance where the watercourse flows through surface mine waste.10
Results and discussion
Streamflow, pH, and metal concentrations
Calculated streamflows for each of the 21 stream sites, and the corresponding Br tracer concentration values are shown in Fig. 2. Streamflow increased with distance downstream from the tracer injection point from 3.71 L s−1 at N-0 to 15.35 L s−1 at N-19, the end of the studied reach. The largest inflow on the day of sampling was the Nant y Glo (R-3), responsible for 16% of the total streamflow increase. This was also the only inflow responsible for more than a 10% increase in streamflow. Stream segments with sampled inflows accounted for 63% of the total streamflow measured in the stream, indicating that at least 37% of the streamflow on the day of sampling came from subsurface or unidentified diffuse surface water inputs.
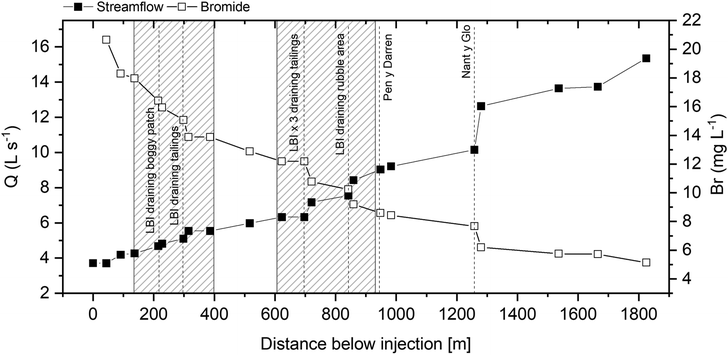 |
| Fig. 2 Downstream changes in Br concentration and streamflow (Q) along the Nant y Bai. Major inflows are marked with dashed lines, and areas of mine waste with hashed boxes. | |
Stream pH values varied between 6.45 to 6.91, with the inflow pH values slightly lower, between 6.25 to 6.78; both are shown in Fig. 3. The River Tywi has historically suffered from acidification, from both coniferous plantations and from industrial pollution.39–41 The latter has reduced substantially in the last thirty years,1,42,43 and the while the wider mine site is used for conifer plantation, with approximately 40% of the Nant y Bai catchment used for this purpose, due to the mine waste near the Nant y Bai there are only a few coniferous trees growing in the immediate vicinity of the stream.
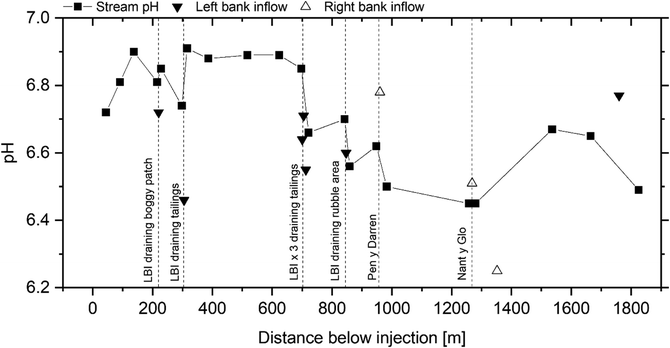 |
| Fig. 3 Downstream changes in pH at stream and inflow sites. Major inflows are marked with dashed lines. | |
The WFD and drinking water standards for Pb, Zn and Cd are shown in Table 1. Concentrations of these metals increased over the upstream area of mine waste, and substantially after the inflow at L-2, coincident with the Zn and Cd peak concentrations (Fig. 4). This sharp increase in the concentration of all three metals at N-6 occurs after a left bank inflow at L-2 demonstrating high concentrations, with filtered Zn at 10
274 μg L−1, filtered Pb at 1924 μg L−1, and filtered Cd at 37.4 μg L−1. The main stream after this input has a filtered Pb concentration of 217 μg L−1, a filtered Zn concentration of 1124 μg L−1, and a filtered Cd concentration of 4.6 μg L−1. The concentration of all three metals decrease after this inflow until the downstream area of mine waste where Pb concentrations increase. Two non-mine-impacted tributaries enter the Nant y Bai between the end of the downstream area of mine waste and the sampling point at N-17, and these have a diluting effect on all three metals. The mean filtered concentration of Pb over the studied reach was 272 μg L−1 (range 2.6–1924 μg L−1), the mean filtered concentration of Zn was 1058 μg L−1 (range 5.4–10
274 μg L−1) and the mean filtered concentration of Cd was 4.5 μg L−1 (range 0.7–37.4 μg L−1). Pb and Zn exceeded the respective EQS for 88% of the studied reach, and Cd was in exceedance of the EQS for the entire length of the studied reach. The filtered and total concentrations were similar for all three metals of concern, indicating that metal transport was primarily in the filtered (potentially dissolved) fraction.
Table 1 WFD Environmental Quality Standards for the River Tywi, and the drinking water standards for England and Wales44,45
Element |
WFD EQS (μg L−1) |
England & Wales drinking water (μg L−1) |
Zinc (Zn) (bioavailable) |
12.9 |
— |
Cadmium (Cd) |
≤0.08 |
5 |
Lead (Pb) (bioavailable) |
1.2 |
10 |
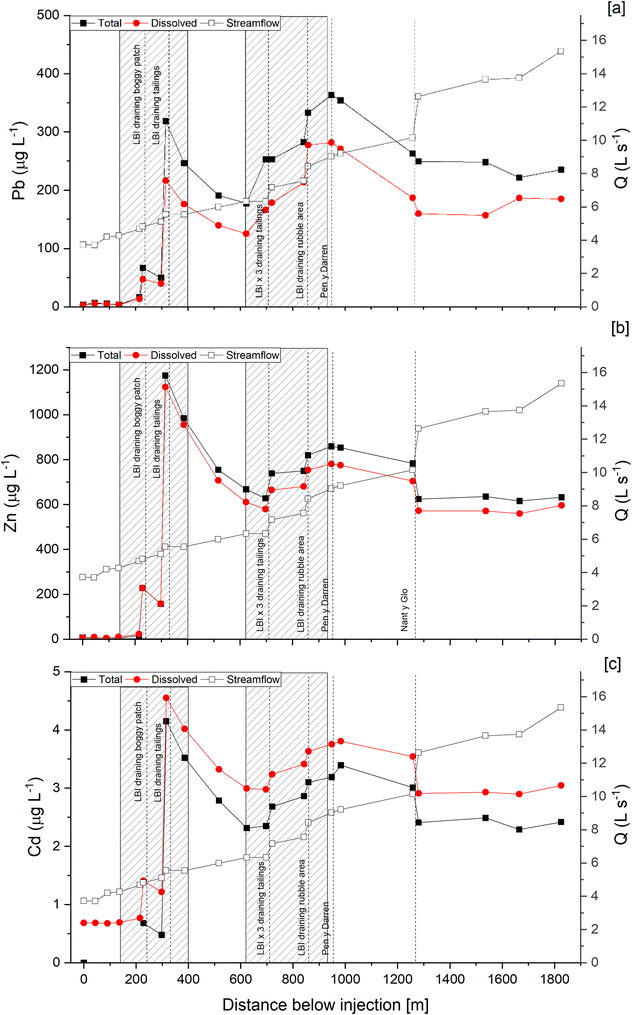 |
| Fig. 4 Downstream changes in total and filtered Pb stream concentrations (a), total and filtered Zn concentrations (b), and total and filtered Cd concentrations (c), with flow (Q) superimposed. | |
Metal loadings and patterns
The loading of metals for each stream segment, and the cumulative stream load at that point, are shown in Fig. 4. The single largest load increase over any measured stretch of stream for all three metals was at N-6, downstream of an ephemeral stream in the upper tailings. This contributed 45% of filtered Zn, 25% of filtered Pb, and 35% of filtered Cd to the stream. Other large sources (>10%) include: a) N-12, within which an inflow at L-6 contributes 18% of the filtered Pb, 10% filtered Zn, and 9% filtered Cd, and b) N-19 which contributes 12% of both filtered Zn and Cd, and 7% of filtered Pb, though that section does not have any visible inflows.
Lesser but still important sources (>5%) of filtered Zn include the area above N-12 which contributes 10%, with an inflow from the lower mine waste area at L-6. The final measured stretch flows through farmland which may have been used by the mine, as historical paintings show ore loading activities nearby.46 This segment, N-19, provides 8% of the filtered Zn. N-9, the first stretch in the lower mine waste area provides 6% of the filtered Zn.
Between the two areas of mine waste, from N-7A to N-9, the Pb load declined 12% for total and 11% for filtered, indicating both physical and chemical attenuation processes causing this decrease. Over the whole studied reach 28% of the total Pb load added to the reach is attenuated, 24% of the Zn, and 13% of the Cd.
Major source areas of metal pollution
Identification and classification of the source areas is key to successful and cost-effective mine pollution remediation.3 Several diffuse sources of mine pollution were identified along the studied reach, characterised in two groups: visible surface run-off from mine waste, and diffuse groundwater inflows. The surface run-off from diffuse sources is of particular interest as the tracer injection experiment was conducted under steady state conditions, meaning that these sources were not directly linked to rainfall run-off as is common.28 Studies at other sites have shown that even after remediation these surface run-off diffuse sources can cause EQS failure during and after storm events.47 Older methods for ore processing, often relied on water, either for direct power, or as part of the processing action, but leave minimal traces of the physical infrastructure many decades after they ceased to operate.48
The stream segments identified as metal sources to the stream were classified into four groups, dependent upon their location and the nature of the source area: mining inflow (MI), non-mine inflow (NMI), mining diffuse (MD), and non-mining diffuse (NMD) (Fig. 5). Segments with a sampled inflow were put into the inflow groups, and this was subdivided into mining, when the segment was in an area with mine waste, or where historical aerial photos showed mine waste had been revegetated, or non-mine where there was no mine waste, in the segment. Stream segments with no sampled inflow were allocated to the diffuse group, and again subdivided into mining and non-mining groups. The largest source of Zn, Pb, and Cd was MD, contributing 55% of the filtered Zn load, 42% of the filtered Pb load, and 49% of the filtered Cd load. By their nature, diffuse groundwater sources can only be identified in areas between sampling points, and it is likely that over a segment there will be areas of attenuation as well as increase.49 This means that any figure for metal load increases is a net increase over the stream segment, and if the source is to be considered for direct remediation, the segment will need to be analysed further. MI was responsible for 40% of the filtered Pb load, but just 22% of the filtered Cd and 14% of the Zn.
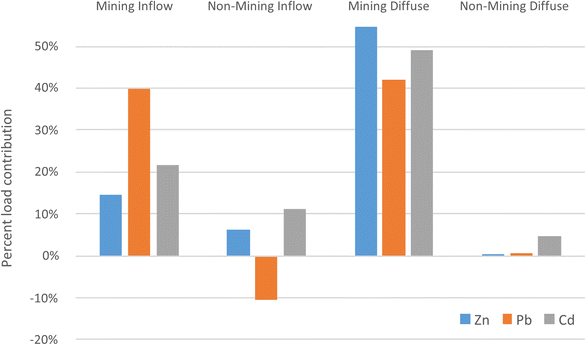 |
| Fig. 5 Percent contribution and grouping of source areas (mining inflow (MI), non-mine inflow (NMI), mining diffuse (MD), and non-mining diffuse (NMD)), to overall metal loads, filtered Zn, Pb, and Cd. | |
Informing remediation
The results from this synoptic sampling and tracer injection experiment locate and quantify sources of metal pollution during baseflow stream conditions, and while any future remediation should consider all likely flow conditions at a site, preliminary predictions can be made for improvements to water quality after remediation. To demonstrate improvements at the end of the studied reach that could be possible now that the sources at low baseflow conditions have been identified, the data from the experiment were applied to the Department for Environment, Food and Rural Affairs (DEFRA, UK) Source Apportionment Spreadsheet.50 This tool is designed to automatically calculate the importance of point and diffuse pollution sources in non-coal mining impacted catchments after inputting flow and concentration data for sampling points in a stream.50 It can then also estimate improvements in water quality at these points to model theoretical remediation strategies.50
The largest group source of pollution, mining diffuse (MD), is largely (78%) in the upper reach of the stream between 90–500 m below the tracer injection point. The mine waste here could be consolidated, reprofiled, and capped, in a similar manner to the work conducted at the Frongoch Pb Mine.51,52 Although not the only method used, this remediation strategy reduced the total Zn concentrations in the Frongoch stream by 20%.52 Using the DEFRA Source Apportionment Spreadsheet, this reduction of 20% in total Zn was input for the upper reach of the stream, and used to predict downstream concentrations in the Nant y Bai. However, with these proposed changes, the predicted decrease in total Zn concentration in the Nant y Bai was only 3% which would be of limited use to improve water quality.
The synoptic sampling and tracer injection experiment combined with the data from the Source Apportionment DEFRA spreadsheet also allows for the remediation of point sources to be modelled. The passive mine water treatment system at Force Crag Pb and Zn mine in England removes on average 97% of the Zn from the circumneutral minewaters.53 To model a similar removal rate of a theoretical system at Nantymwyn, a 97% reduction in total Zn from inputs L-1 and L-2 was modelled by the Source Apportionment DEFRA spreadsheet, which predicted a reduction in Zn concentrations at point N-10 of 73%. With additional work to gauge the cost and difficulty of treating or consolidating sources, this dataset can be used to predict the water quality improvements these will offer, and therefore give a balanced view of the best combination of remediation in terms of cost, difficulty, and metal reduction. While any remediation of the site, whether by passive or active treatment systems, or by reducing diffuse sources from the mine waste and subsurface inflows, would require data from higher baseflow conditions in summer and winter using the bromide tracer and synoptic sampling technique,54 as well as monitoring of storm events, this demonstrates the ability of a synoptic sampling and tracer injection experiment to guide remediation of a site.
Conclusions
In July 2019 a tracer injection and synoptic sampling experiment was carried out on a 2 km section of the Nant y Bai, a stream impacted by the abandoned Nantymwyn Pb Mine, to investigate sources of mine pollution at a high spatial resolution. Diffuse and point subsurface and surface inflows of both Pb and Zn were identified and quantified over the two main areas of mine waste, as well as inputs in vegetated areas, at a much higher spatial resolution than is provided by conventional spot sampling. The experiment was conducted during very low flows for the catchment, with minimal rainfall, and demonstrated that groundwater diffuse sources of metals from an abandoned mine continue to affect watercourses despite the limited surface run-off during low baseflow conditions. The use of tracer injection and synoptic sampling allows for streamflow and water chemistry to be established along a watercourse at a single point in time.
The data from the tracer injection and synoptic sampling can be used both to quantify sources of pollution, and to model possible remediation scenarios. This powerful method can be utilised similarly at other sites where pollutant flows are poorly understood, or where sources cannot be located by visual identification or traditional spot sampling. The high spatial resolution data from the approach of tracer injection and synoptic sampling is key to informing remediation plans.
Author contributions
Aaron Todd: investigation, visualization, writing – original draft preparation, & editing. Iain Robertson: investigation, funding acquisition, writing – review & editing. Patrick Byrne: investigation, methodology, funding acquisition, resources, writing – review & editing. Rory Walsh: investigation, writing – review & editing. Tom Williams: investigation, writing – review & editing. Paul Edwards: investigation, writing – review & editing.
Conflicts of interest
There are no conflicts to declare.
Acknowledgements
The authors thank the Knowledge Economy Skills Scholarships (KESS 2) for funding AT's PhD, allowing this research to be undertaken. We also thank Ilaria Frau and NRW staff for their assistance with fieldwork. PB acknowledges receipt of a Natural Environment Research Council award (NE/S009507/1).
References
- M. J. Whelan, C. Linstead, F. Worrall, S. J. Ormerod, I. Durance, A. C. Johnson, D. Johnson, M. Owen, E. Wiik, N. J. K. Howden, T. P. Burt, A. Boxall, C. D. Brown, D. M. Oliver and D. Tickner, Sci. Total Environ., 2022, 843, 157014 CrossRef CAS PubMed.
- M. G. Macklin, C. J. Thomas, A. Mudbhatkal, P. A. Brewer, K. A. Hudson-Edwards, J. Lewin, P. Scussolini, D. Eilander, A. Lechner, J. Owen, G. Bird, D. Kemp and K. R. Mangalaa, Science, 2023, 381, 1345–1350 CrossRef CAS PubMed.
- B. A. Kimball, R. L. Runkel, K. Walton-Day and K. E. Bencala, Appl. Geochem., 2002, 17, 1183–1207 CrossRef CAS.
-
Environment Agency Wales, Metal Mines Strategy for Wales, Environment Agency Wales, Cardiff, 2002 Search PubMed.
- A. Sartorius, M. Johnson, S. Young, M. Bennett, K. Baiker, P. Edwards and L. Yon, Food Addit. Contam.: Part A, 2022, 39, 1074–1085 CrossRef CAS PubMed.
- J. E. Gall, R. S. Boyd and N. Rajakaruna, Environ. Monit. Assess., 2015, 187, 201 CrossRef PubMed.
-
P. B. Tchounwou, C. G. Yedjou, A. K. Patlolla and D. J. Sutton, Molecular, Clinical and Environmental Toxicology, 2012, vol. 101, pp. 133–164 Search PubMed.
-
Natural Resources Wales, Reason for not achieving good Cycle 3 [Microsoft Excel Spreadsheet], Natural Resources Wales, Cardiff, 2022 Search PubMed.
-
Coal Authority, Metal Mine Failing Waterbodies Assessment – Overview Report, The Coal Authority, Mansfield, 2020 Search PubMed.
-
B. A. Kimball, Use of tracer injections and synoptic sampling to measure metal loading from acid mine drainage. US Geological Survey Fact Sheet 245-96, USGS, 1997 Search PubMed.
- P. Byrne, A. Yendell, I. Frau and A. M. L. Todd, Mine Water Environ., 2021, 1, 1–14 Search PubMed.
-
P. Onnis, P. Byrne, K. A. Hudson-Edwards, T. Stott and C. Hunt, in Mine Water – Risk to Opportunity, ed. Ch. Wolkersdorfer, L. Sartz, A. Weber, J. Burgess and G. Tremblay, Pretoria, South Africa, 2018, vol. 2, pp. 843–849 Search PubMed.
- P. Byrne, P. Onnis, R. L. Runkel, I. Frau, S. F. L. Lynch and P. Edwards, Environ. Sci. Technol., 2020, 54, 15742–15750 CrossRef CAS PubMed.
- N. W. Arnell and S. N. Gosling, J. Hydrol., 2013, 486, 351–364 CrossRef.
- W. H. Blake, R. P. D. Walsh, M. J. Barnsley, G. Palmer, P. Dyrynda and J. G. James, Hydrol. Processes, 2003, 17, 1923–1939 CrossRef.
-
G. W. Hall, Nantymwyn Mine, https://www.aditnow.co.uk/Mines/Nantymwyn-Lead-Mine_4295/, (accessed 5 June 2020).
-
G. W. Hall, Metal Mining in Mid Wales 1822–1921, The Welsh Mines Society, Aberystwyth, 2014 Search PubMed.
-
M. J. Al-Atia and J. W. Barnes, in Geochemical Exploration 1974: Proceedings Of The Fifth International Geochemical Exploration Symposium, ed. I. L. Elliot and W. K. Fletcher, Association of Exploration Geochemists, Vancouver, 1975, pp. 341–352 Search PubMed.
-
J. R. Davies, D. I. Schofield, T. H. Sheppard, R. A. Waters, M. Williams and D. Wilson, Geology of the Lampeter district — a brief explanation of the geological map sheet 195 Lampeter, British Geological Survey, Nottingham, 2006 Search PubMed.
-
National River Flow Archive, 60007 – Tywi at Dolau Hirion, https://nrfa.ceh.ac.uk/data/station/meanflow/60007, (accessed 1 February 2021).
-
A. Ralph, OM4077_3 Longitudinal Setn. of the workings on the principle lode in Nantymwyn Lead Mine, 1830 Search PubMed.
-
S. J. S. Hughes, British Mining, 1992, pp. 87–110 Search PubMed.
-
H. De-Quincey, PhD Thesis, Swansea University, 2020 Search PubMed.
- A. C. Johnson, R. L. Donnachie, J. P. Sumpter, M. D. Jürgens, C. Moeckel and M. G. Pereira, Sci. Total Environ., 2017, 599–600, 1372–1381 CrossRef CAS PubMed.
-
Fisheries Preservation Society, On The Pollution Of The Rivers Of The Kingdom; The Enormous Magnitude Of The Evil, And The Urgent Necessity In The Interest Of The Public Health & The Fisheries For Its Suppression By Immediate Legislative Enactment, London, 1868 Search PubMed.
-
South West Wales River Authority, Ninth and Final Statutory Annual Report 1973–1974, South West Wales River Authority, Llanelli, 1974 Search PubMed.
-
S. J. Brown, The Effects of Abandoned Metal Mines at Rhandirmwyn on the Quality of the Upper River Tywi SW/86/20, Welsh Water, Haverfordwest, 1986 Search PubMed.
- P. Byrne, I. Reid and P. J. Wood, Environ. Monit. Assess., 2013, 185, 2817–2832 CrossRef CAS PubMed.
-
F. A. Kilpatrick and E. D. Cobb, Techniques of Water Resources Investigations of the United States Geological Survey, 1985, vol. 3, p. 52 Search PubMed.
- P. Onnis, P. Byrne, K. A. Hudson-edwards, T. Stott and C. O. Hunt, Minerals, 2023, 13(6), 790 CrossRef CAS.
-
D. A. Dzombak and F. M. M. Morel, Surface Complexation Modeling: Hydrous Ferric Oxide, John Wiley, New York, 1990 Search PubMed.
-
Instrumentation Northwest Inc, AquiStar ® TempHion TM Smart Sensor (ph, ISE, Redox) Instruction Manual, 2012 Search PubMed.
-
B. A. Kimball, R. L. Runkel, T. E. Cleasby and D. A. Nimick, US Geological Survey Professional Paper, 2004, pp. 191–262 Search PubMed.
-
Environment Agency, Chemical and microbiological sampling of water, Environment Agency, Bristol, 9th edn, 2010 Search PubMed.
- G. E. Batley and D. Gardner, Water Res., 1977, 11, 745–756 CrossRef CAS.
- J. Y. Zhang, G. M. Li and W. Guo, At. Spectrosc., 2014, 35, 97–102 CrossRef CAS.
-
Natural Resources Wales, UK Water Quality Sampling Harmonised Monitoring Scheme Detailed Data, https://libcat.naturalresources.wales/folio/?oid=116338.
- R. L. Runkel, K. Walton-Day, B. A. Kimball, P. L. Verplanck and D. A. Nimick, J. Hydrol., 2013, 489, 26–41 CrossRef CAS.
-
National Rivers Authority Welsh Region, Llyn Brianne Acid Waters Project: Final Report, Cardiff, 1992 Search PubMed.
- R. Ward, R. W. Edwards, A. S. Gee and J. H. Stoner, Trans. Inst. Br. Geogr., 1991, 16, 505 CrossRef.
- S. J. Ormerod and I. Durance, J. Appl. Ecol., 2009, 46, 164–174 CrossRef CAS.
- S. Reis, P. Grennfelt, Z. Klimont, M. Amann, H. ApSimon, J. P. Hettelingh, M. Holland, A. C. LeGall, R. Maas, M. Posch, T. Spranger, M. A. Sutton and M. Williams, Science, 2012, 338, 1153–1154 CrossRef CAS PubMed.
-
E. C. Lloyd, M. J. Jenkins and A. P. Rogers, Acid Waters : Llyn Brianne Project Project Record, National Rivers Authority, Bristol, 1996 Search PubMed.
-
The Water Supply (Water Quality) Regulations, UK, 2016 Search PubMed.
-
Water Framework Directive, The Water Framework Directive (Standards and Classification) Directions (England and Wales), UK, 2015 Search PubMed.
-
J. W. Smith, Interior of the mining part of Carreg Mowyn where the lead ore is principally carried in boats through narrow level communications branching from the mines: The boats used in this subterraneous navigation are narrow & sharp at each end & of about 5 tons, The National Library of Wales, Painting, 1792 Search PubMed.
- G. J. P. Thornton and R. P. D. Walsh, Sci. Total Environ., 2001, 278, 45–55 CrossRef CAS PubMed.
-
C. Mills, I. Simpson and W. P. Adderley, Landscape History, 2014, vol. 35, pp. 47–72 Search PubMed.
- B. Palumbo-Roe, J. Wragg and V. J. Banks, J. Soils Sediments, 2012, 12, 1633–1640 CrossRef CAS.
-
DEFRA, Excel source apportionment spreadsheet tool: User instructions, DEFRA, Newcastle upon Tyne, 2018 Search PubMed.
-
P. Edwards, J. F. Murphy, J. I. Jones, C. Morgan, R. P. D. Walsh and J. Gething, in IMWA 2021 – ‘Mine Water Management for Future Generations’, ed. P. Stanley, C. Wolkersdorfer and K. Wolkersdorfer, IMWA, Cardiff, 2021, pp. 145–150 Search PubMed.
-
P. Edwards, T. Williams and P. Stanley, in IMWA 2016 – Mining Meets Water – Conflicts and Solutions, ed. C. Drebenstedt and M. Paul, IMWA, Freiberg, 2016, pp. 546–553 Search PubMed.
-
A. Jarvis, C. Gandy, M. Bailey, J. Davis, P. Orme, J. Malley, H. Potter and A. Moorhouse, in 10th International Conference on Acid Rock Drainage & IMWA Annual Conference, ed. A. Brown, C. Bucknam, J. Burgess, M. Carballo, D. Castendyk, L. Figueroa, L. Kirk, V. McLemore, J. McPhee, M. O'Kane, R. Seal, J. Wiertz, D. Williams, W. Wilson and C. Wolkersdorfer, GECAMIN, Santiago, Chile, 2015 Search PubMed.
- P. Onnis, P. Byrne, K. A. Hudson-Edwards, I. Frau, T. Stott, T. Williams, P. Edwards and C. O. Hunt, Appl. Geochem., 2023, 151, 105623 CrossRef CAS.
-
Ordnance Survey, OS MasterMap Open, EDINA Digimap Ordnance Survey Service, Edinburgh, 2020 Search PubMed.
|
This journal is © The Royal Society of Chemistry 2024 |
Click here to see how this site uses Cookies. View our privacy policy here.