PFAS occurrence and distribution in yard waste compost indicate potential volatile loss, downward migration, and transformation†
Received
2nd December 2023
, Accepted 30th January 2024
First published on 30th January 2024
Abstract
We discovered high concentrations of PFAS (18.53 ± 1.5 μg kg−1) in yard waste compost, a compost type widely acceptable to the public. Seventeen out of forty targeted PFAS, belonging to six PFAS classes were detected in yard waste compost, with PFCAs (13.51 ± 0.99 μg kg−1) and PFSAs (4.13 ± 0.19 μg kg−1) being the dominant classes, comprising approximately 72.5% and 22.1% of the total measured PFAS. Both short-chain PFAS, such as PFBA, PFHxA, and PFBS, and long-chain PFAS, such as PFOA and PFOS, were prevalent in all the tested yard waste compost samples. We also discovered the co-occurrence of PFAS with low-density polyethylene (LDPE) and polyethylene terephthalate (PET) plastics. Total PFAS concentrations in LDPE and PET separated from incoming yard waste were 7.41 ± 0.41 μg kg−1 and 1.35 ± 0.1 μg kg−1, which increased to 8.66 ± 0.81 μg kg−1 in LDPE and 5.44 ± 0.56 μg kg−1 in PET separated from compost. An idle mature compost pile revealed a clear vertical distribution of PFAS, with the total PFAS concentrations at the surface level approximately 58.9–63.2% lower than the 2 ft level. This difference might be attributed to the volatile loss of short-chain PFCAs, PFAS's downward movement with moisture, and aerobic transformations of precursor PFAS at the surface.
Environmental significance
The presence of PFAS in various ecosystems is a critical issue, with growing evidence of their harmful effects. We discovered significant PFAS contamination in yard waste compost, a concern given its extensive use in agriculture. PFAS, often co-existing with plastics in compost, underscores the urgent need for creative strategies to minimize risks such as water pollution and exposure to humans and wildlife through land application. We also uncovered PFAS's vertical movement and potential transformation in compost, pointing to possible volatile loss and downward migration. This insight is vital for comprehending PFAS fate in compost and fostering innovative removal techniques. The outcome will transform compost into a tool for enhancing soil health, rather than a conduit for persistent organic pollutants.
|
1. Introduction
The pervasive presence of per- and polyfluoroalkyl substances (PFAS) in the environment is concerning due to their potential long-lasting and harmful effects on humans, animals, and other organisms.1–5 Because of the widespread and unrestricted historical use of PFAS in daily life products and injudicious disposal, PFAS have become pervasive in the environment.6,7 A recent study identified 160 different PFAS in biosolids and compost with a total PFAS concentration of 580–1300 μg kg−1.8 One pathway of PFAS transport to agricultural lands is via biosolids and compost application.9,10 For instance, approximately 320–920 μg kg−1 of perfluoroalkyl acids (PFAAs) were identified in agricultural land that received municipal biosolids for more than ten years.11 Approximately 50% of sewage sludge in Europe and North America is processed for agricultural use,12 and 35% of US households utilize compost for gardening.13,14 Besides the negative impact on the soil microbial community,15–18 plant uptake of PFAS has been investigated, which can create significant health consequences for humans and livestock.19–24 Over time, PFAS can leach from compost into surface runoff, contaminating natural water bodies, and can also percolate through soil, polluting groundwater.8,9 This led many US states, such as Maine and Vermont, to ban PFAS-containing compostable materials (e.g., sewage sludge) for agricultural use.25,26
We critically summarized the PFAS concentration in compost from different studies (Table S1†) and identified no single studies on pure yard waste compost, possibly because of the minimal chance of PFAS contamination of yard waste. Studies reported 0.03–133.30 μg kg−1 PFAS in biosolid and manure compost,8,27 31.5–70.8 μg kg−1 in municipal organic compost (i.e., food waste, leaves, grass, and horse manure),28 8.6–16.3 μg kg−1 in green waste compost (i.e., kitchen waste, yard trimmings),29 and 6.8–11.84 μg kg−1 in commercially available compost (i.e., organic feed unknown).30 Though these studies investigated PFAS in different types of compost, exploring PFAS in pure yard waste compost remains limited. Typically perceived as the purest type of compost, yard waste compost is widely used in many households for gardening. Thus, we investigated forty PFAS in mature yard waste compost, investigated incoming and composted plastics to understand plastics-PFAS co-occurrence, and investigated an idle mature compost pile to elucidate the vertical distribution profile of PFAS. The findings of this study will elucidate the extent of PFAS contamination in yard waste compost and inform the development of global remediation strategies for PFAS in solid materials.
2. Materials and methods
2.1. Yard waste compost
The City of Fargo (North Dakota, USA) residents can drop off yard waste at various locations year-round for free collection by the city authority. The Fargo yard waste compost site accepts tree leaves and grass from the residents. Unfortunately, there has been a tendency to dump unwanted substances on the site (e.g., plastics, paper, packaging) alongside yard waste, mainly due to the lack of awareness and supervision. Yard waste composting typically takes 3–4 months.31 The mature compost from the 13-acre Fargo compost site is then piled together for subsequent screening. We collected mature compost in October 2020 just after final screening with a Komptech Trommel Screen, which ensured proper homogenization. When the compost site has a surplus of mature compost, they store it inside the locked fence (Fig. S1†). We also collected yard waste compost from the surface and 2 ft depth of the compost storage pile from three locations at least six feet apart (composts A, B, and C) in July 2021. The compost storage pile, approximately 1500 cubic feet in volume, has been idle inside a locked fence (100 ft × 100 ft) for over six months.
We also separated and collected bulk plastics from incoming fresh yard waste and composted yard waste. All the compost and plastic samples were collected in LC-MS grade methanol-cleaned plastic zip-lock bags and stored in a refrigerator at 4 °C before analysis and extraction. After proper homogenization, a certain mass of the composts was extracted using the ASTM D3987-12 protocol,32 and the ammonium and nitrate concentrations in the extracts were quantified to calculate the maturity index (NH4+/NO3−) of the compost samples.33 The extracts were filtered through 0.45 μm Whatman filter papers (Sigma Aldrich, MO, USA) and the organic carbon (as dissolved organic matter) of the extracts was measured using a Shimadzu TOC Analyzer (Text S1 and Table S2†). All the collected compost samples were fully mature (maturity index <0.5) (Table S3†). The total carbon, organic carbon, and total inorganic carbon concentrations of all the compost samples were quantified using a Shimadzu TOC Solid Sample Analyzer (Text S2 and Table S2†). Monthly total precipitation and average temperature data at the compost site from Jan 2020 to Dec 2021 are provided in Table S4.†
2.2. PFAS extraction and analysis
Homogenized compost samples were sieved twice through a 1.5 mm pore-sized LC-MS grade methanol-cleaned aluminum net. Ten grams of compost from each compost were taken in triplicates in pre-cleaned 50 mL polypropylene tubes. Then, 100 μL mass-labeled PFAS internal standard (MPFAC-HIF-ES, Wellington Laboratories, Guelph, ON, Canada) was added to the sample and adequately mixed (Table S5†). The targeted analytes, extracted internal standards, and non-extracted internal standards were similar to the EPA draft method 1633 (ref. 34) (Table S6†). 10 mL of LC-MS grade methanol (Fisher Scientific, USA) was added to the sample tubes for extraction on a shaker (Thermo Scientific, MA, USA) at 200 rpm for 24 hours, followed by room temperature centrifugation at 10
000 rpm for 2 hours. After centrifugation, methanol was poured into pre-cleaned 10 mL polypropylene tubes and concentrated to 1 mL with a nitrogen blowdown evaporator. For PFAS extraction from plastics, plastics were first dried at room temperature and dusted off loose organics using compressed air. Plastics were cut down into small pieces (∼10 mm), and a certain mass was extracted by following the same protocol for compost. A detailed characterization procedure for these plastics, which includes crack analysis on the surface, can be found in the ESI (Text S3, S4, and Table S7†). We also prepared procedural blank samples to assess background contamination during extraction (Text S5†). The extraction recovery for the mass-labeled PFAS internal standards was 58–126%, similar to previous studies that used a low concentration of ammonium salts with methanol for PFAS extraction from compost (Table S1†).
We targeted forty PFAS (demonstrated in the EPA draft method 1633 (ref. 34)) in compost and plastics from seven PFAS classes. These include eleven perfluorocarboxylic acids (PFCAs), eight perfluorosulfonic acids (PFSAs), three fluorotelomer carboxylic acids (FTCAs), three fluorotelomer sulfonic acids (FTSs), seven perfluoroalkane sulfonyl fluorides (PASFs) or sulfanomido compounds, five perfluoroether carboxylic acids (PFECAs), and three perfluoroether sulfonic acids (PFESAs). The PFAS were quantified using an ultra-performance liquid chromatograph (UPLC) coupled with a Triple Quadrupole mass spectrometer (Vanqish UPLC-Altis MS, Thermo Scientific, USA) in the negative ionization mode. UPLC separation was carried out using a Phenomenex Luna Omega C18 column (2.1 × 100 mm) following an already published protocol (Text S6 and Table S8†).35,36 The measured PFAS mass was normalized by the dry mass of compost. Statistical analysis and plotting were performed using OriginPro (version 2023b, 10.05). Two-way multivariate analysis (MANOVA) was performed to understand the impact of sampling depth and moisture on the distribution of PFAS. We also conducted a one-way analysis of variance (ANOVA, α ≤ 0.05) for the total carbon and dissolved organic matter (DOM) at the surface and at 2 ft depth in composts A–C to find the correlation with PFAS distribution. Additionally, linear regression analysis (significant at α ≤ 0.05) of DOM versus total PFAS at varying depths was conducted to understand the correlation between DOM and total PFAS distribution. Pearson correlation was performed to test for the co-occurrence of different PFAS at different depths. Furthermore, we calculated the ratio of PFAS concentrations at 2 ft to the PFAS concentrations at the surface level. Linear regression analysis was performed between these values and the water solubility and vapor pressure values of PFAS to elucidate the correlation (significant at α ≤ 0.05).
3. Results and discussion
3.1. PFAS in yard waste compost
Out of the forty targeted PFAS, seventeen compounds spanning six PFAS classes (excluding FTCAs) were found in yard waste compost (Table S9† and Fig. 1). Dominantly, 72.5% were PFCAs and 22.1% were PFSAs (Fig. 1). Prior research indicates that 60–70% of the targeted PFAS in municipal organic and biosolid composts were PFCAs and PFSAs, together termed perfluoroalkyl acids (PFAAs).13,28 In the yard waste compost, short-chain PFAAs, such as perfluorobutanoic acids (PFBA), perfluorohexanoic acids (PFHxA), and perfluorobutane sulfonic acids (PFBS), were detected at high concentrations. Specifically, concentrations of 11.15 ± 1.5 μg kg−1 PFBA, 1.34 ± 0.07 μg kg−1 PFHxA, and 1.53 ± 0.12 μg kg−1 PFBS were detected (Fig. 1). Correspondingly, previous studies reported high concentrations of PFBA (0.15–12.04 μg kg−1), PFHxA (1.06–1.39 μg kg−1), and PFBS (0.79–7.63 μg kg−1) in commercial and municipal organic waste compost28,30 (Table S1†). Long-chain PFAS, including PFOA (0.44 ± 0.04 μg kg−1), linear PFOS (0.86 ± 0.08 μg kg−1), branched PFOS (0.43 ± 0.06 μg kg−1), and PFHpS (1.15 ± 0.045 μg kg−1), were also detected in the yard waste compost (Fig. 1). Notably, US chemical manufacturers voluntarily phased out PFOA and PFOS in 2002 and 2015, respectively.37 Similar to our findings, studies identified long-chain PFAAs (PFCAs > C7 and PFSAs > C5) in various composts13,29 (Table S1†). Additionally, considerable concentrations of 6
:
2 fluorotelomer sulfonic acid (6
:
2 FTS, 0.30 ± 0.02 μg kg−1) and N-ethyl perfluorooctane sulfonamido acetic acid (EtFOSAA, 0.12 ± 0.008 μg kg−1) were identified (Fig. 1). Fluorotelomer sulfonic acids can transform into PFAAs in composts.30 This conversion involves the oxidation of carbon–fluorine bonds within fluorotelomer-based PFAS, yielding PFAAs.30,38 Hence, some detected PFAAs in compost might stem from fluorotelomers' transformation. Additionally, fluorotelomer alcohols (FTOHs) can transform into FTCAs during yard waste composting, similar to the transformation of 6
:
2 FTOH into 5
:
3 FTCA and 4
:
3 FTCA during sewage sludge composting.39
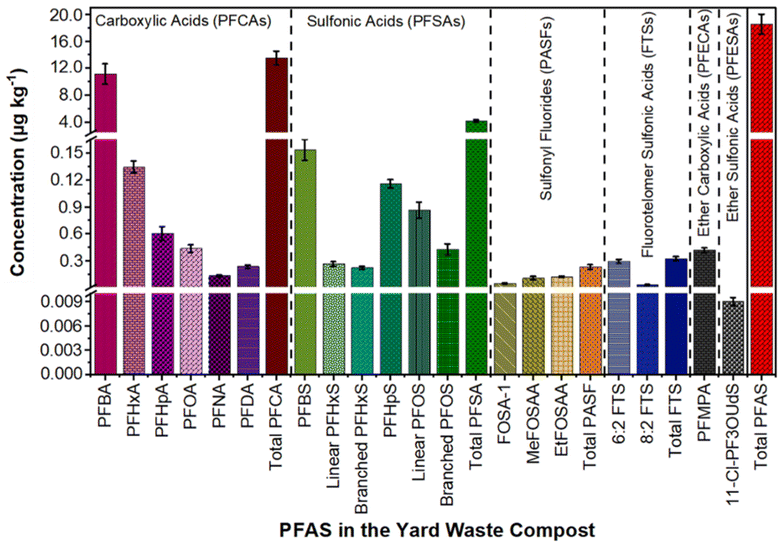 |
| Fig. 1 Concentrations of seventeen different PFAS from six classes in yard waste compost. Among these, only perfluoro-3-methoxy propanoic acid (PFMPA) was detected in the PFECA class, and 11-chloroeicosafluoro-3-oxaundecane-1-sulfonic acid (11-Cl-PF3OUdS) was detected in the PFESA class. The average and standard deviation for each compound were calculated from six measurements, and extended details are available in Table S9.† Perfluoroalkane sulfonyl fluorides (PASFs) represent three sulfonamido compounds. | |
3.2. Plastics and PFAS co-occurrence
LDPE and PET were identified as prevalent plastics in yard waste compost (Fig. S2†). This is because PET water bottles often end up in yard waste, and many individuals dispose of yard waste using LDPE bags. When examining the carbonyl index, vinyl index, and crystallinity before and after composting, negligible chemical degradation was observed (Fig. S3†); however, these plastics can act as carriers of adsorbed PFAS in the environment.40–43 We identified seven PFAS in the incoming plastics and ten in the compost-separated plastics (Fig. 2). The incoming LDPE contained more total PFAS (7.41 ± 0.41 μg kg−1) than the incoming PET (1.35 ± 0.1 μg kg−1) (Fig. 2). This discrepancy is because LDPE, with its high flexibility, rough surface, and strong hydrophobicity, adsorbs more organic pollutants than PET.44–46 Additionally, the shape of PET bottles remains largely unchanged, limiting the surface area available for PFAS adsorption. PFAS concentrations varied between incoming and compost-separated LDPE and PET, suggesting PFAS enrichment on plastics during composting. The total PFAS in compost-separated LDPE and PET increased to 8.66 ± 0.81 μg kg−1 and 5.44 ± 0.56 μg kg−1, respectively. Notably, the mass of PFAS per kg in the combined LDPE and PET plastics is about 23.8% lower than the mass found in a kg of compost. Both types of plastics showed a dominance of short-chain PFAS (Fig. 2). Besides the original PFAS in incoming LDPE, PFHxA (0.38 ± 0.01 μg kg−1), MeFOSAA (0.1 ± 0.02 μg kg−1), and EtFOSAA (0.18 ± 0.03 μg kg−1) were adsorbed during composting (Fig. 2). This enrichment may be due to the composting process creating microscopic cracks in plastics, enhancing their surface area (Fig. S4†).47,48
 |
| Fig. 2 Ten out of forty analyzed PFAS were detected in low-density polyethylene (LDPE) and polyethylene terephthalate (PET) separated from incoming and composted yard waste. Extended forms of the PFAS compounds are available in Table S9.† | |
3.3. Vertical distribution of PFAS in a compost storage pile
The compost PFAS concentration at the surface level was significantly lower compared to the PFAS level at 2 ft depth, indicating potential volatilization loss or downward movement with moisture. The total PFAS concentration at 2 ft depth was 2.5, 2.1, and 2.5 times of the total PFAS concentrations at the surface level for composts A, B, and C (Fig. 3, S5 and S6†). Similarly, the total PFAS concentration at 1 ft depth was 57.65% greater than the surface level in compost A (Fig. S5 and S6†). Additionally, the concentration of two dominant PFAS classes, such as PFCAs and PFSAs, at 2 ft depths were 2.8–3.6 and 1.4–1.7 times of the surface level concentrations (Fig. 3). In compost A, at 2 ft depth, PFBA had the highest concentration (2.95 ± 0.08 μg kg−1), trailed by PFBS (1.88 ± 0.1 μg kg−1); a trend mirrored in composts B and C (Fig. S7–S9†). Of the short chain PFCAs, PFBA and PFPeA concentrations at 2 ft depth were approximately 5.9–9.3 times of the surface level concentrations in composts A–C (Fig. 3). Downward movement of short-chain PFAS was reported in soil columns because of the high water solubility from their terminal functional groups' water affinity.49,50 Thus, the precipitation (e.g., rainfall, snow) at the compost site will directly affect the downward movement of water-soluble PFAS in the compost piles. The compost moisture content ranged from 28.1 to 29.9% at the surface and 41.3 to 44.8% at 2 ft depths, similar to the PFAS distribution trend with depth (Table S3†). The ratio of PFAS concentration at 2 ft depths to the surface was positively correlated with the water solubility of PFAS (Fig. 4A and Table S10†), which suggests that the water solubility of PFAS impacted their downward movement in the compost pile.
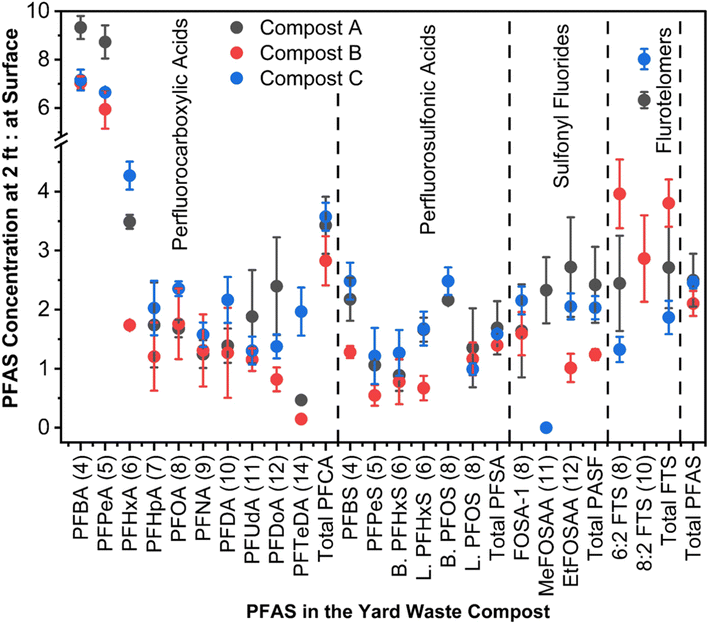 |
| Fig. 3 The ratio of PFAS concentrations at 2 ft depths to the surface in the yard waste compost. To calculate the ratio, PFAS concentrations at 2 ft depths were divided by the PFAS concentrations at the surface for composts A, B, and C. Nineteen out of the forty analyzed PFAS were detected in these compost samples. | |
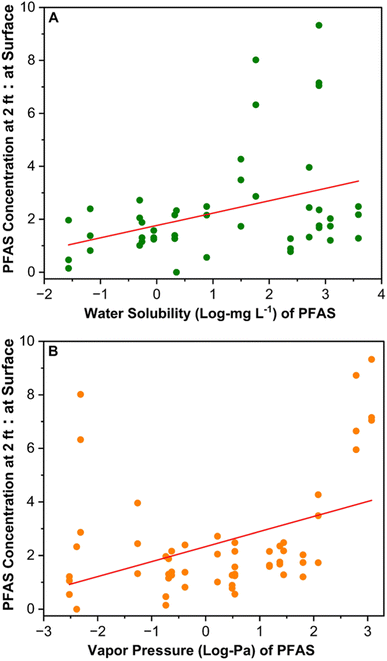 |
| Fig. 4 Linear regression analysis between the ratio of PFAS concentrations at 2 feet depth to surface level and their water solubility (A) and vapor pressure (B). To calculate the ratio, PFAS concentrations at 2 ft depth were divided by the concentrations at the surface for composts A, B, and C. The median value of water solubility and vapor pressure of PFAS were used for the analysis and plots. Median value for each PFAS were derived by consolidating data from 82 peer-reviewed sources for water solubility and 77 peer-reviewed sources for vapor pressure (Fig. S10†). | |
Besides downward movement with moisture, volatile loss and transformation might explain PFAS reduction at the pile's surface. Literature reveals that short-chain PFAS (e.g., PFBA, PFPeA, PFHxA) with high vapor pressure are more volatile than their long-chain counterparts (Fig. S10†). These short-chain PFAS compounds sorb to water vapor, increasing their long-range transport potential.51–53 A strong positive correlation between the vapor pressure of PFAS and the ratio of PFAS concentration at 2 ft depth to the surface in the compost samples was observed (Fig. 4B and Table S11†). This suggests the possibility of volatile loss of PFAS with high vapor pressure from the compost surface, leading to reduced PFAS levels at the surface. PFAA precursors such as fluorotelomer sulfonates and sulfonamides are semi-volatile due to their large molecular size and strong intermolecular forces, limiting evaporation.51,54 These precursors have low water affinity due to their longer carbon chains, making them more likely to sorb on solids.55,56 However, we found that semi-volatile PFAA precursors such as FOSA-1's concentrations at the surface level were 40–60% less compared to the 2 ft level, EtFOSAA was 45–60% less, and 6
:
2 FTS was 25–80% less at the surface than at 2 ft (Fig. 3 and S7–S9†). Besides the downward movement with moisture and volatile loss, this high percent loss from the surface could be attributed to the biological transformation of PFAA precursors into short-chain PFAAs at the top compost layer (0–10 cm) under oxygen-rich conditions.57 Studies indicate that this biotransformation is more prevalent in surface soil than in deeper layers.57–59
Long-chain PFAS were less mobile in the compost pile than short-chain PFAS and PFAS precursors. The change of long-chain PFAS concentrations at the compost surface was notable, but less than the change in short-chain and precursor PFAS concentrations (Fig. 3). The distribution of long-chain PFAS is influenced by both moisture and DOM in the solid medium.49,61–67 Interestingly, DOM at 2 ft depth (7.82–14.21 g kg−1) was significantly (α ≤ 0.05) greater than the DOM at the compost surface (3.57–4.78 g kg−1), showing a strong correlation with the total PFAS at varying depths (Tables S12, S13 and Fig. S5†). However, there was no significant variation in the total carbon content across depths in composts A–C (Tables S2, S14 and Fig. S6†). The fate of PFAS is also influenced by their structural variations, such as isomers. Research indicates that linear PFAS isomers tend to adhere to soil and sediments, whereas branched isomers are more prone to movement.60 This difference is linked to the greater polarity of branched isomers relative to linear ones. Our findings revealed that branched PFOS levels were 2.5 times greater at 2 ft depth than at the surface, suggesting water-assisted downward movement (Fig. 3). Conversely, linear PFOS levels were similar at both depths, indicating sorption by compost. PFAS binding affinity on organics can also be affected by other chemical factors such as functional groups, aromaticity, and humification.50,68–70 Furthermore, biogeochemical elements like iron deposits on compost can also influence PFAS adsorption, especially with iron oxides binding short-chain PFAS via surface complexation or ion exchange.50,71 Redox gradients alter the distribution and form of iron oxides with depth, which could affect PFAS adsorption variability.72–74 Although theoretically, stronger surface adsorption of PFAS might occur by biogeochemical factors that could slow down PFAS's downward movement, this study did not show such evidence.
Composts at greater depths had notably more moisture than surface layers (Table S3†). Depth and moisture content were interdependent, significantly impacting the concentration of PFAS species at 0 and 2 ft depths (Table S15†). Statistical analyses showed significant correlations among certain short-chain PFAS (i.e., PFBA, PFBS, PFPeA, PFHxA, and PFHpA) and between them and compounds like 6
:
2 FTS, 8
:
2 FTS, and FOSA-1, suggesting a shared origin (Fig. 5). Likewise, long-chain PFAS (i.e., PFNA, PFDA, and PFUdA) were significantly correlated with one another and with certain short-chain compounds and precursors (i.e., PFHpA, 8
:
2 FTS, and EtFOSAA), hinting at a common origin (Fig. 5). However, PFSAs showed no distinct correlation in yard waste compost, indicating different sources. Prior research has noted strong correlations among various PFAS in liquid and solid mediums, pointing to shared origins.75–78 A leading contributor of PFAS in yard waste is plastics, which often end up in yard waste due to the lack of public awareness and monitoring. Paper, paper products, and packaging materials can also act as sources of PFAS in yard waste compost. Moreover, the water used to maintain the moisture content of yard waste can act as a source of PFAS in compost. The proximity of the compost site near the Fargo landfill increases the likelihood of PFAS entering the compost through atmospheric deposition, particularly due to the potential presence of volatile PFAS in emissions from landfill materials. Given these varied sources, it is essential to conduct an in-depth investigation to pinpoint the precise origins of PFAS in yard waste compost.
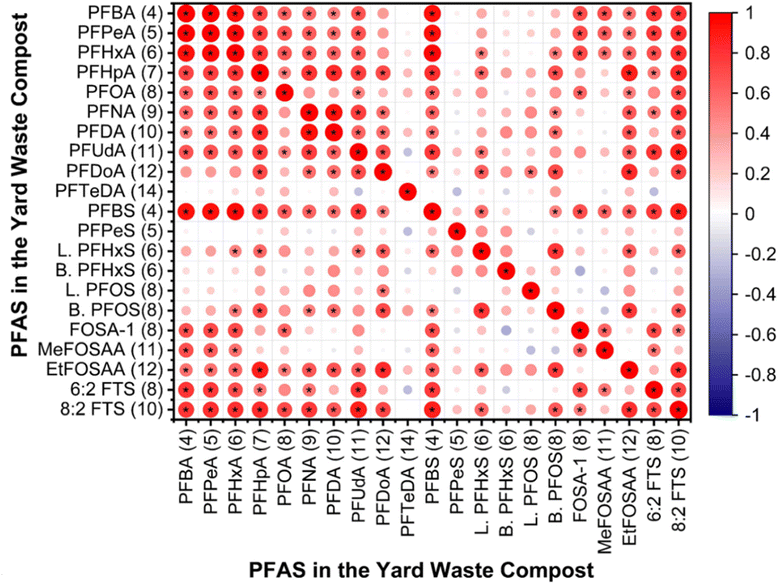 |
| Fig. 5 Pearson correlation (significant at α ≤ 0.05) among the detected PFAS at the surface level (i.e., 0 ft) and at 2 ft depths in yard waste composts A, B, and C. The red circle with ‘*’ denotes a significant correlation between the corresponding pairs of the PFAS. The red color in the right-side bar indicates the intensity of a strong correlation, and the blue color denotes the intensity of a weak correlation among different PFAS. | |
Conclusions
This novel investigation revealing substantial PFAS contamination and plastic-PFAS co-occurrence in yard waste compost calls for attention to mitigate risks from land application. With total PFAS detected at 18.53 ± 1.5 μg kg−1 (Fig. 1), concentrations comparable to those in biosolids-derived composts, demanding rigorous testing and regulations for this popular soil amendment. The prevalence of short-chain PFAS, such as PFBA, and the downward movement of PFAS with moisture through the 2 ft compost profile demonstrate the potential for groundwater leaching and surface water pollution via runoff (Fig. S11†) when PFAS-laden composts are spread in gardens and on agricultural fields. Enriching PFAS on plastics during composting also identifies an overlooked exposure pathway to humans and wildlife. The adsorption of PFAS on plastics in compost can lead to two outcomes. Removing these plastics before processing reduces PFAS contamination risks. Yet, due to the low profitability of composting, this step is skipped, leading to the fragmentation of PFAS-laden plastics into micro- and nanoplastics during compost grinding. This results in PFAS being distributed along with these tiny plastic particles, which can elevate the environmental hazards associated with PFAS. While surface volatilization may reduce short-chain PFAS levels, continuous environmental recirculation limits this as a permanent solution. The precise quantification of PFAS downward migration, volatile loss, and transformation in a compost pile is outside the scope of this study. With the mounting evidence on PFAS uptake in crops, implementing science-based testing, treatment, and regulatory guidance is imperative to sustain the circular benefits of composting without perpetuating toxic PFAS pollution. Deriving innovative solutions for PFAS removal (e.g., cost-effective engineered adsorbents) will transform compost into a driver of healthy soils and sustainable food systems rather than a source of persistent organic pollutants.
Conflicts of interest
The authors declare no conflict of interest.
Acknowledgements
We acknowledge the Environmental Research and Education Foundation (EREF) for supporting Biraj Saha with PhD scholarship. Biraj Saha was also partially supported by the Civil, Construction, and Environmental Engineering Department at North Dakota State University as a graduate assistant. The authors would like to acknowledge the help of Scott Olson from the City of Fargo Solid Waste Division for his help with sample collection.
References
- C. Lau, J. L. Butenhoff and J. M. Rogers, The developmental toxicity of perfluoroalkyl acids and their derivatives, Toxicol. Appl. Pharmacol., 2004, 198(2), 231–241 CrossRef CAS PubMed.
- K. A. Barzen-Hanson, S. C. Roberts, S. Choyke, K. Oetjen, A. McAlees and N. Riddell,
et al., Discovery of 40 Classes of Per- and Polyfluoroalkyl Substances in Historical Aqueous Film-Forming Foams (AFFFs) and AFFF-Impacted Groundwater, Environ. Sci. Technol., 2017, 51(4), 2047–2057 CrossRef CAS PubMed.
- M. Ateia, D. Chiang, M. Cashman and C. Acheson, Total Oxidizable Precursor (TOP) Assay-Best Practices, Capabilities and Limitations for PFAS Site Investigation and Remediation, Environ. Sci. Technol. Lett., 2023, 10(4), 292–301 CrossRef CAS PubMed.
- M. Kotthoff, J. Müller, H. Jürling, M. Schlummer and D. Fiedler, Perfluoroalkyl and polyfluoroalkyl substances in consumer products, Environ. Sci. Pollut. Res., 2015, 22(19), 14546–14559 CrossRef CAS PubMed.
- A. C. Maizel, S. Shea, A. Nickerson, C. Schaefer and C. P. Higgins, Release of Per- and Polyfluoroalkyl Substances from Aqueous Film-Forming Foam Impacted Soils, Environ. Sci. Technol., 2021, 55(21), 14617–14627 CrossRef CAS PubMed.
- J. P. Benskin, B. Li, M. G. Ikonomou, J. R. Grace and L. Y. Li, Per- and Polyfluoroalkyl Substances in Landfill Leachate: Patterns, Time Trends, and Sources, Environ. Sci. Technol., 2012, 46(21), 11532–11540 CrossRef CAS PubMed.
- Z. S. Wei, T. Y. Xu and D. Y. Zhao, Treatment of per- and polyfluoroalkyl substances in landfill leachate: status, chemistry and prospects, Environ. Sci.: Water Res. Technol., 2019, 5(11), 1814–1835 RSC.
- G. Munoz, A. M. Michaud, M. Liu, S. Vo Duy, D. Montenach and C. Resseguier,
et al., Target and Nontarget Screening of PFAS in Biosolids, Composts, and Other Organic Waste Products for Land Application in France, Environ. Sci. Technol., 2022, 56(10), 6056–6068 CrossRef CAS PubMed.
- K. Röhler, A. A. Haluska, B. Susset, B. Liu and P. Grathwohl, Long-term behavior of PFAS in contaminated agricultural soils in Germany, J. Contam. Hydrol., 2021, 241, 103812 CrossRef PubMed.
- I. L. Pepper, M. L. Brusseau, F. J. Prevatt and B. A. Escobar, Incidence of Pfas in soil following long-term application of class B biosolids, Sci. Total Environ., 2021, 793, 148449 CrossRef CAS PubMed.
- J. W. Washington, H. Yoo, J. J. Ellington, T. M. Jenkins and E. L. Libelo, Concentrations, distribution, and persistence of perfluoroalkylates in sludge-applied soils near Decatur, Alabama, USA, Environ. Sci. Technol., 2010, 44(22), 8390–8396 CrossRef CAS PubMed.
- L. Nizzetto, M. Futter and S. Langaas, Are Agricultural Soils Dumps for Microplastics of Urban Origin?, Environ. Sci. Technol., 2016, 50(20), 10777–10779 CrossRef CAS PubMed.
- R. Kim Lazcano, Y. J. Choi, M. L. Mashtare and L. S. Lee, Characterizing and Comparing Per- and Polyfluoroalkyl Substances in Commercially Available Biosolid and Organic Non-Biosolid-Based Products, Environ. Sci. Technol., 2020, 54(14), 8640–8648 CrossRef CAS PubMed.
- Z. Zhang, D. Sarkar, J. K. Biswas and R. Datta, Biodegradation of per- and polyfluoroalkyl substances (PFAS): A review, Bioresour. Technol., 2022, 344, 126223 CrossRef CAS PubMed.
- H. Liu, W. Hu, X. Li, F. Hu, Y. Liu and T. Xie,
et al., Effects of perfluoroalkyl substances on root and rhizosphere bacteria: Phytotoxicity, phyto-microbial remediation, risk assessment, Chemosphere, 2022, 289, 133137 CrossRef CAS PubMed.
- J.-Y. Wu, F.-G. Ding, Z.-W. Shen, Z.-L. Hua and L. Gu, Linking microbiomes with per- and poly-fluoroalkyl substances (PFASs) in soil ecosystems: Microbial community assembly, stability, and trophic phylosymbiosis, Chemosphere, 2022, 305, 135403 CrossRef CAS PubMed.
- Y. Sun, C. Duan, N. Cao, X. Li, X. Li and Y. Chen,
et al., Effects of microplastics on soil microbiome: The impacts of polymer type, shape, and concentration, Sci. Total Environ., 2022, 806, 150516 CrossRef CAS PubMed.
- F. Zhu, Y. Yan, E. Doyle, C. Zhu, X. Jin and Z. Chen,
et al., Microplastics altered soil microbiome and nitrogen cycling: The role of phthalate plasticizer, J. Hazard. Mater., 2022, 427, 127944 CrossRef CAS PubMed.
- W. Mei, H. Sun, M. Song, L. Jiang, Y. Li and W. Lu,
et al., Per- and polyfluoroalkyl substances (PFASs) in the soil–plant system: Sorption, root uptake, and translocation, Environ. Int., 2021, 156, 106642 CrossRef CAS PubMed.
- B. Xu, W. Qiu, J. Du, Z. Wan, J. L. Zhou and H. Chen,
et al., Translocation, bioaccumulation, and distribution of perfluoroalkyl and polyfluoroalkyl substances (PFASs) in plants, iScience, 2022, 25(4), 104061 CrossRef CAS PubMed.
- M. C. S. Costello and L. S. Lee, Sources, Fate, and Plant Uptake in Agricultural Systems of Per- and Polyfluoroalkyl Substances, Curr. Pollut. Rep., 2020 DOI:10.1007/s40726-020-00168-y.
- H. Eun, E. Yamazaki, S. Taniyasu, A. Miecznikowska, J. Falandysz and N. Yamashita, Evaluation of perfluoroalkyl substances in field-cultivated vegetables, Chemosphere, 2020, 239, 124750 CrossRef CAS PubMed.
- I. Azeem, M. Adeel, M. A. Ahmad, N. Shakoor, G. D. Jiangcuo and K. Azeem,
et al., Uptake and Accumulation of Nano/Microplastics in Plants: A Critical Review, Nanomaterials, 2021, 11(11), 2935 CrossRef CAS PubMed.
- W. Wang, G. Rhodes, J. Ge, X. Yu and H. Li, Uptake and accumulation of per- and polyfluoroalkyl substances in plants, Chemosphere, 2020, 261, 127584 CrossRef CAS PubMed.
-
Maine Department of Environmental Protection, State of Maine Research and Collaboration Regarding PFAS Treatment and Destruction, 2021, Public Law 2021, Chapter 641, effective August 8, 2022, https://www.maine.gov/dep/spills/topics/pfas/#Treat. Access on 15 Jan 2024 Search PubMed.
-
S. Michael and C. M. J. Heard Snow, An Emerging Containment of (Legal) Concern: PFAS Legal Issues at the State and Federal Leve, William and Marry Law School Scholarship Repository, 2020, https://scholarship.law.wm.edu/vcpclinic, Accessed on June 10 2022 Search PubMed.
- R. Kim Lazcano, C. de Perre, M. L. Mashtare and L. S. Lee, Per- and polyfluoroalkyl substances in commercially available biosolid-based products: The effect of treatment processes, Water Environ. Res., 2019, 91(12), 1669–1677 CrossRef CAS PubMed.
- Y. J. Choi, R. Kim Lazcano, P. Yousefi, H. Trim and L. S. Lee, Perfluoroalkyl Acid Characterization in U.S. Municipal Organic Solid Waste Composts, Environ. Sci. Technol. Lett., 2019, 6(6), 372–377 CrossRef CAS.
- R. C. Brändli, T. Kupper, T. D. Bucheli, M. Zennegg, S. Huber and D. Ortelli,
et al., Organic pollutants in compost and digestate. Part 2. Polychlorinated dibenzo-p-dioxins, and -furans, dioxin-like polychlorinated biphenyls, brominated flame retardants, perfluorinated alkyl substances, pesticides, and other compounds, J. Environ. Monit., 2007, 9(5), 465–472 RSC.
- A. K. Sivaram, L. Panneerselvan, A. Surapaneni, E. Lee, K. Kannan and M. Megharaj, Per- and polyfluoroalkyl substances (PFAS) in commercial composts, garden soils, and potting mixes of Australia, Environ. Adv., 2022, 7, 100174 CrossRef CAS.
- M. Pergola, A. Persiani, A. M. Palese, V. Di Meo, V. Pastore and C. D'Adamo,
et al., Composting: The way for a sustainable agriculture, Appl. Soil Ecol., 2018, 123, 744–750 CrossRef.
-
ASTM, Standard Practice for Shake Extraction of Solid Waste with Water, 2006, https://webstore.ansi.org/standards/astm/astmd398706, Accessed on Feb 20 2023 Search PubMed.
- J. Huang, Z. Yu, H. Gao, X. Yan, J. Chang and C. Wang,
et al., Chemical structures and characteristics of animal manures and composts during composting and assessment of maturity indices, PLoS One, 2017, 12(6), e0178110 CrossRef PubMed.
-
Draft Method 1633 for 40 PFAS Compounds, https://www.epa.gov/cwa-methods/cwa-analytical-methods-and-polyfluorinated-alkyl-substances-pfas#draft-method-1633, Accessed on June 01 2022 Search PubMed.
- R. Li, S. Alomari, T. Islamoglu, O. K. Farha, S. Fernando and S. M. Thagard,
et al., Systematic Study on the Removal of Per- and Polyfluoroalkyl Substances from Contaminated Groundwater Using Metal-Organic Frameworks, Environ. Sci. Technol., 2021, 55(22), 15162–15171 CrossRef CAS PubMed.
- R. Li, S. Alomari, R. Stanton, M. C. Wasson, T. Islamoglu and O. K. Farha,
et al., Efficient Removal of Per- and Polyfluoroalkyl Substances from Water with Zirconium-Based Metal–Organic Frameworks, Chem. Mater., 2021, 33(9), 3276–3285 CrossRef CAS.
-
USEPA, EPA Announces New Drinking Water Health Advisories for PFAS Chemicals, $1 Billion in Bipartisan Infrastructure Law Funding to Strengthen Health Protections, 2022, https://www.epa.gov/newsreleases/epa-announces-new-drinking-water-health-advisories-pfas-chemicals-1-billion-bipartisan, Accessed on Mar 01 2023 Search PubMed.
- C. M. Butt, D. C. G. Muir and S. A. Mabury, Biotransformation pathways of fluorotelomer-based polyfluoroalkyl substances: A review, Environ. Toxicol. Chem., 2014, 33(2), 243–267 CrossRef CAS PubMed.
- W. Qiao, J. Miao, H. Jiang and Q. Yang, Degradation and effect of 6:2 fluorotelomer alcohol in aerobic composting of sludge, Biodegradation, 2021, 32(1), 99–112 CrossRef CAS PubMed.
- F. Wang, K. M. Shih and X. Y. Li, The partition behavior of perfluorooctanesulfonate (PFOS) and perfluorooctanesulfonamide (FOSA) on microplastics, Chemosphere, 2015, 119, 841–847 CrossRef CAS PubMed.
- N. Islam, T. Garcia da Fonseca, J. Vilke, J. M. Gonçalves, P. Pedro and S. Keiter,
et al., Perfluorooctane sulfonic acid (PFOS) adsorbed to polyethylene microplastics: Accumulation and ecotoxicological effects in the clam Scrobicularia plana, Mar. Environ. Res., 2021, 164, 105249 CrossRef CAS PubMed.
- A. Bakir, S. J. Rowland and R. C. Thompson, Competitive sorption of persistent organic pollutants onto microplastics in the marine environment, Mar. Pollut. Bull., 2012, 64(12), 2782–2789 CrossRef CAS PubMed.
- J. W. Scott, K. G. Gunderson, L. A. Green, R. R. Rediske and A. D. Steinman, Perfluoroalkylated Substances (PFAS) Associated with Microplastics in a Lake Environment, Toxics, 2021, 9(5), 106 CrossRef CAS PubMed.
- B. C. Crone, T. F. Speth, D. G. Wahman, S. J. Smith, G. Abulikemu and E. J. Kleiner,
et al., Occurrence of per- and polyfluoroalkyl substances (PFAS) in source water and their treatment in drinking water, Crit. Rev. Environ. Sci. Technol., 2019, 49(24), 2359–2396 CrossRef PubMed.
- E. Hu, H. Yuan, Y. Du and X. Chen, LDPE and HDPE Microplastics Differently Affect the Transport of Tetracycline in Saturated Porous Media, Materials, 2021, 14(7), 1757 CrossRef CAS PubMed.
- L. Fu, J. Li, G. Wang, Y. Luan and W. Dai, Adsorption behavior of organic pollutants on microplastics, Ecotoxicol. Environ. Saf., 2021, 217, 112207 CrossRef CAS PubMed.
- C. Scopetani, D. Chelazzi, A. Cincinelli, T. Martellini, V. Leiniö and J. Pellinen, Hazardous contaminants in plastics contained in compost and agricultural soil, Chemosphere, 2022, 293, 133645 CrossRef CAS PubMed.
- E. O. Esan, L. Abbey and S. Yurgel, Exploring the long-term effect of plastic on compost microbiome, PLoS One, 2019, 14(3), e0214376 CrossRef CAS PubMed.
- Y. Wang, U. Munir and Q. Huang, Occurrence of per- and polyfluoroalkyl substances (PFAS) in soil: Sources, fate, and remediation, Soil & Environmental Health., 2023, 1(1), 100004 Search PubMed.
- T. M. H. Nguyen, J. Bräunig, K. Thompson, J. Thompson, S. Kabiri and D. A. Navarro,
et al., Influences of Chemical Properties, Soil Properties, and Solution pH on Soil–Water Partitioning Coefficients of Per- and Polyfluoroalkyl Substances (PFASs), Environ. Sci. Technol., 2020, 54(24), 15883–15892 CrossRef CAS PubMed.
- C. A. Barton, M. A. Botelho and M. A. Kaiser, Solid Vapor Pressure and Enthalpy of Sublimation for Perfluorooctanoic Acid, J. Chem. Eng. Data, 2008, 53(4), 939–941 CrossRef CAS.
- X. Liu, Understanding semi-volatile organic compounds in indoor dust, Indoor Built Environ., 2022, 31(2), 291–298 CrossRef CAS PubMed.
- I. K. Dimzon, J. Westerveld, C. Gremmel, T. Frömel, T. P. Knepper and P. De Voogt, Sampling and simultaneous determination of volatile per- and polyfluoroalkyl substances in wastewater treatment plant air and water, Anal. Bioanal. Chem., 2017, 409(5), 1395–1404 CrossRef CAS PubMed.
- A. M. Piekarz, T. Primbs, J. A. Field, D. F. Barofsky and S. Simonich, Semivolatile Fluorinated Organic Compounds in Asian and Western U.S. Air Masses, Environ. Sci. Technol., 2007, 41(24), 8248–8255 CrossRef CAS PubMed.
- J.-N. Uwayezu, L. W. Y. Yeung and M. Bäckström, Sorption of Perfluorooctane Sulfonic Acid Including Its Isomers to Soils: Effects of pH, Natural Organic Matter and Na2SO4, Front. Environ. Chem., 2022, 3, 905170 CrossRef.
- D. Zhou, M. L. Brusseau, Y. Zhang, S. Li, W. Wei and H. Sun,
et al., Simulating PFAS adsorption kinetics, adsorption isotherms, and nonideal transport in saturated soil with tempered one-sided stable density (TOSD) based models, J. Hazard. Mater., 2021, 411, 125169 CrossRef CAS PubMed.
- J. Zhou, G. Zhao, M. Li, J. Li, X. Liang and X. Yang,
et al., Three-dimensional spatial distribution of legacy and novel poly/perfluoroalkyl substances in the Tibetan Plateau soil: Implications for transport and sources, Environ. Int., 2022, 158, 107007 CrossRef CAS PubMed.
- S. Mejia Avendaño and J. Liu, Production of PFOS from aerobic soil biotransformation of two perfluoroalkyl sulfonamide derivatives, Chemosphere, 2015, 119, 1084–1090 CrossRef PubMed.
- S. Brendel, É. Fetter, C. Staude, L. Vierke and A. Biegel-Engler, Short-chain perfluoroalkyl acids: environmental concerns and a regulatory strategy under REACH, Environ. Sci. Eur., 2018, 30(1), 9 CrossRef PubMed.
- K. Schulz, M. R. Silva and R. Klaper, Distribution and effects of branched versus linear isomers of PFOA, PFOS, and PFHxS: A review of recent literature, Sci. Total Environ., 2020, 733, 139186 CrossRef CAS PubMed.
- M. L. Brusseau and B. Guo, PFAS concentrations in soil versus soil porewater: Mass distributions and the impact of adsorption at air-water interfaces, Chemosphere, 2022, 302, 134938 CrossRef CAS PubMed.
- M. L. Brusseau, Estimating the relative magnitudes of adsorption to solid-water and air/oil-water interfaces for per- and poly-fluoroalkyl substances, Environ. Pollut., 2019, 254, 113102 CrossRef CAS PubMed.
- T. M. H. Nguyen, J. Bräunig, R. S. Kookana, S. L. Kaserzon, E. R. Knight and H. N. P. Vo,
et al., Assessment of Mobilization Potential of Per- and Polyfluoroalkyl Substances for Soil Remediation, Environ. Sci. Technol., 2022, 56(14), 10030–10041 CrossRef CAS PubMed.
- D. Mussabek, K. M. Persson, R. Berndtsson, L. Ahrens, K. Nakagawa and T. Imura, Impact of the Sediment Organic vs. Mineral Content on Distribution of the Per- and Polyfluoroalkyl Substances (PFAS) in Lake Sediment, Int. J. Environ. Res. Public Health, 2020, 17(16), 5642 CrossRef CAS PubMed.
- E. K. Savvidou, B. Sha, M. E. Salter, I. T. Cousins and J. H. Johansson, Horizontal and Vertical Distribution of Perfluoroalkyl Acids (PFAAs) in the Water Column of the Atlantic Ocean, Environ. Sci. Technol. Lett., 2023, 10(5), 418–424 CrossRef CAS PubMed.
- C.-d Gan, M.-y Peng, H.-b Liu and J.-y Yang, Concentration and distribution of metals, total fluorine, per- and poly-fluoroalkyl substances (PFAS) in vertical soil profiles in industrialized areas, Chemosphere, 2022, 302, 134855 CrossRef CAS PubMed.
- L. Qi, R. Li, Y. Wu, X. Lin and G. Chen, Effect of solution chemistry on the transport of short-chain and long-chain perfluoroalkyl carboxylic acids (PFCAs) in saturated porous media, Chemosphere, 2022, 303, 135160 CrossRef CAS PubMed.
- H. Campos Pereira, M. Ullberg, D. B. Kleja, J. P. Gustafsson and L. Ahrens, Sorption of perfluoroalkyl substances (PFASs) to an organic soil horizon – Effect of cation composition and pH, Chemosphere, 2018, 207, 183–191 CrossRef CAS PubMed.
- F. Li, X. Fang, Z. Zhou, X. Liao, J. Zou and B. Yuan,
et al., Adsorption of perfluorinated acids onto soils: Kinetics, isotherms, and influences of soil properties, Sci. Total Environ., 2019, 649, 504–514 CrossRef CAS PubMed.
- H. Campos-Pereira, J. Makselon, D. B. Kleja, I. Prater, I. Kögel-Knabner and L. Ahrens,
et al., Binding of per- and polyfluoroalkyl substances (PFASs) by organic soil materials with different structural composition – Charge- and concentration-dependent sorption behavior, Chemosphere, 2022, 297, 134167 CrossRef CAS PubMed.
- X. Tan, P. Dewapriya, P. Prasad, Y. Chang, X. Huang and Y. Wang,
et al., Efficient Removal of Perfluorinated Chemicals from Contaminated Water Sources Using Magnetic Fluorinated Polymer Sorbents, Angew. Chem., Int. Ed., 2022, 61(49), e202213071 CrossRef CAS PubMed.
- A. Thompson, D. G. Rancourt, O. A. Chadwick and J. Chorover, Iron solid-phase differentiation along a redox gradient in basaltic soils, Geochim. Cosmochim. Acta, 2011, 75(1), 119–133 CrossRef CAS.
- A. E. O'Connor, J. L. Luek, H. McIntosh and A. J. Beck, Geochemistry of redox-sensitive trace elements in a shallow subterranean estuary, Mar. Chem., 2015, 172, 70–81 CrossRef.
- A. K. Tokranov, D. R. LeBlanc, H. M. Pickard, B. J. Ruyle, L. B. Barber and R. B. Hull,
et al., Surface-water/groundwater boundaries affect seasonal PFAS concentrations and PFAA precursor transformations, Environ. Sci.: Processes Impacts, 2021, 23(12), 1893–1905 RSC.
- P. Zhao, X. Xia, J. Dong, N. Xia, X. Jiang and Y. Li,
et al., Short- and long-chain perfluoroalkyl substances in the water, suspended particulate matter, and surface sediment of a turbid river, Sci. Total Environ., 2016, 568, 57–65 CrossRef CAS PubMed.
- K. A. Pike, P. L. Edmiston, J. J. Morrison and J. A. Faust, Correlation Analysis of Perfluoroalkyl Substances in Regional U.S. Precipitation Events, Water Res., 2021, 190, 116685 CrossRef CAS PubMed.
- W. S. Chambers, J. G. Hopkins and S. M. Richards, A Review of Per- and Polyfluorinated Alkyl Substance Impairment of Reproduction, Front. toxicol., 2021, 3, 732436 CrossRef PubMed.
- J. Kim, X. Xin, B. T. Mamo, G. L. Hawkins, K. Li and Y. Chen,
et al., Occurrence and Fate of Ultrashort-Chain and Other Per- and Polyfluoroalkyl Substances (PFAS) in Wastewater Treatment Plants, ACS ES&T Water, 2022, 2(8), 1380–1390 Search PubMed.
Footnote |
† Electronic supplementary information (ESI) available: Supporting texts on compost carbon analysis, dissolved organic matter analysis, FTIR analysis of plastics, advanced image analysis of plastics surface, quality assurance and quality control (QA/QC), UPLC–MS/MS analysis; supporting tables on PFAS concentrations in different compost types, moisture content and maturity indices, carbon content, mass-labeled PFAS internal standards, FTIR operating conditions, UPLC–MS/MS operating conditions, extended forms of PFAS, one-way ANOVA and multivariate two-way ANOVA analysis, linear regression analysis; supporting figures of an idle compost pile, FTIR spectra of plastics and indices, cracks on plastic surface, total PFAS versus DOM at different depths, total PFAS versus total carbon at different depths, concentrations of the investigated PFAS at different depths, and runoff from the compost site. See DOI: https://doi.org/10.1039/d3em00538k |
|
This journal is © The Royal Society of Chemistry 2024 |
Click here to see how this site uses Cookies. View our privacy policy here.