Fate of nutrients and trace contaminants in a large shallow soda lake. Spatial gradients and underlying processes from the tributary river to the reed belt†
Received
13th April 2023
, Accepted 22nd July 2023
First published on 16th August 2023
Abstract
Shallow lakes provide a multitude of ecosystem functions, but they are particularly vulnerable to natural and anthropogenic disturbances. Understanding the driving factors determining the fate and spatial distribution of nutrients and pollutants in such systems is fundamental to assess the impact of ongoing or future external pressures endangering their ecological integrity. This study investigates the fate of trace contaminants transported into the large shallow Lake Neusiedl, including contaminants representative of different patterns of sources and emission pathways and of environmental behavior, namely metals, pharmaceuticals, an artificial sweetener and perfluoroalkyl substances. Further, it examines the horizontal spatial distribution of nutrients, ions and physico-chemical parameters with an unprecedented detailed focus on the internal variability within the large reed belt. As described in the past e.g. for chloride, evaporation was identified as the process leading to a substantial concentration enrichment of the industrial chemical PFOA and the sweetener acesulfame K from the tributary river into the open lake. This is particularly relevant in view of the predicted future increase of evapotranspiration due to climate change. In contrast, the observed loss of diclofenac, but also of PFOS and carbamazepine suggests that the well-mixed, humic-rich and alkaline Lake Neusiedl offers favorable conditions for the photodegradation of otherwise very persistent chemicals. Another important finding, in the context of possible modifications in lake water levels due to climate change, is the fundamental role played by the connectivity between open lake and reed belt but also by the presence and characteristics of inner water areas within the reed belt region in determining the hydrochemistry of the lake system. By revealing systematic spatial patterns and by focusing on the underlying factors and processes, the understanding offered by this study is of high value for the conservation of shallow lakes.
Environmental significance
Lake Neusiedl is the largest endorheic lake in Central Europe, it is surrounded by the largest contiguous reed area in Europe and by being extremely shallow, it is highly vulnerable to anthropogenic disturbance. In this work, we reveal clear and significant spatial patterns of hydro-chemical parameters and trace contaminants and shed light on the factors and mechanisms determining their fate and behavior in the complex lake system. We demonstrate among others the essential roles of the evaporation process and of the connectivity between open lake and reed belt in determining the good health and quality status of the lake. This level of understanding is essential to estimate the future impact of climate-change driven water level fluctuations, anthropogenic emissions and water management in shallow lakes.
|
Introduction
Shallow lakes provide worldwide a disproportionate contribution to biodiversity, nutrient cycling, food production and recreation services, yet at the same time they are more vulnerable than deep lakes to natural or anthropogenic disturbances.1 They are more sensitive to meteorological and hydrological fluctuations than deep stratified lakes, thus being more dramatically exposed to negative impacts of climate change2–5 and are particularly responsive to emission loads of nutrients and trace contaminants.6,7 While most shallow lakes usually lack stable vertical gradients due to their polymictic character, they can show pronounced horizontal differences in chemistry and sediment composition due to wind action and sheltering effects in the littoral zone.8–11 Such spatial differences, combined with pronounced short-term temporal variability, represent a challenge for monitoring impacts of external pressures on hydrochemistry and biology.
This is also true for Lake Neusiedl, the largest endorheic lake in Central Europe. With an average depth of 1–1.5 m, it is extremely shallow. A distinctive trait of Lake Neusiedl is the 4 km wide reed (Phragmites australis) belt, which occupies more than half of its surface. This makes it the largest contiguous reed area in Europe after the Danube Delta, with major international importance as a wetland and bird sanctuary.12,13 The limnological study of this system has predominantly focused so far on the open lake area, while little attention has been paid to the chemical features and biological communities of the reed belt.14–16 The fact that the whole network of water quality monitoring stations is located in the open lake area is further illustrative of the almost exclusive focus on the pelagic zone of the lake system.17 Nevertheless, mass balance analyses suggest that the exchange between reed belt and pelagic zone is of eminent importance for the water quality of the lake.18 According to their calculations, the reed belt represents a sink for suspended solids and nutrients, yet at the same time, depending on water level and connectivity, release processes can export nutrients to the open lake area and significantly contribute to internal loading. In their investigation of spatial changes in the water quality of the Austrian part of Neusiedl Lake via multivariate statistical methods,14 mostly focused on the spatial variability within the open lake and on the local impact of anthropogenic activities. However, by including also sampling points in the channels, they revealed significant differences in water chemistry between the reed belt and open water. While increasing evidence indicates that the water chemism in the reed belt differs substantially from that in the open lake, spatial patterns and driving factors are still only partially understood. The water balance of the lake is highly sensitive to climatic changes19 and, despite large uncertainties, predicted longer droughts in the future3,20,21 might dramatically alter the lake's physico-chemical characteristics and the connection between the pelagic zone and its reed belt. Therefore, an enhanced understanding of the lake system functioning in this respect is of the utmost importance.
In comparison to suspended sediments, nutrients and salts, significantly less is known regarding the pollution level and the fate of metals and organic trace contaminants in the Lake Neusiedl. In the 1990s, the contamination level with heavy metals and their uptake by reed was investigated.22,23 In the framework of a study aimed to evaluate the potential impact of external sources of artificial water dotation to the lake,24 examined the occurrence and concentration level of approximately 20 organic chemicals in the estuary of the Wulka river (the main tributary) and at one spot in the open lake, in which they detected different substances at quantifiable levels.25,26 shed light on the processes behind the accumulation and distribution of mercury in sediments, macrophytes and fish. Despite their relevance, such studies build a fragmented and insufficient knowledge basis to understand the fate of the noteworthy loads of pollutants transported into the lake by the Wulka river.27,28 It is known e.g. that salts such as chloride undergo a concentration enrichment in the lake in years of low water level, due to the combined effect of evaporation and absence of outflow.18 Whether this also applies to persistent trace contaminants has however not been investigated so far.
This work contributes to bridging the above-mentioned knowledge gaps by specifically addressing two main research questions. Firstly, it investigates which fate trace contaminants transported by the Wulka river undergo in the lake. The scope includes a selection of contaminants representative of different patterns of sources and emission pathways and of environmental behavior, namely six metals, two pharmaceuticals, an artificial sweetener and two perfluoroalkyl substances. Secondly, it examines which horizontal differences the water chemism presents between open lake and reed belt area and which role different processes and factors play in determining the spatial variability. For the first time, an extensive monitoring survey was carried out targeting explicitly the reed belt of Lake Neusiedl. To examine its internal variability and gain understanding of the underlying driving factors, the sampling points were strategically selected to include both channels and open water areas within the reed belt, as well as to cover different degrees of connectivity with the open lake. The results provide a so far lacking solid data basis, which: (i) enables testing the underlying hypothesis that the water chemical composition in the reed belt and in the open lake is determined by the connectivity between these two regions of the lake and by local factors such as sedimentation, evaporative concentration and biological processes and (ii) offers quantitative evidence of the role of these phenomena.
Data and methods
Test areas and sampling sites
Situated between Austria and Hungary at 116 m asl, Lake Neusiedl shows a rather small ratio of catchment/lake area of approximately 1120 km2/320 km2. Characteristic traits are high pH values (8.5–9), high soda concentrations (alkalinity 7–15 mmol L−1, sodium concentration 250–600 mg L−1) and high inorganic turbidity due to calcite and dolomite/protodolomite precipitation29 and to the constant turbulence caused by wind and waves.30 Without a natural outlet, the lake is a nearly closed system with precipitation accounting for 79% of its water input and evapotranspiration being responsible for 90% of its water loss, respectively.31 The only relevant tributary is the river Wulka, with a mean discharge of 1 m3 s−1. Owing to rather low precipitations in the 400 km2 large river catchment, in average the discharge of treated municipal wastewater accounts for approximately 50% of the river baseflow discharge, reaching even higher shares in dry seasons. Two test areas were selected at the western shore near the village Mörbisch (M) and at the eastern shore near Illmitz (IL), respectively (Fig. 1, coordinates in Table A1 of the Appendix†). In both areas, the sampling sites were located along transects from the open lake to the inner area of the reed belt.
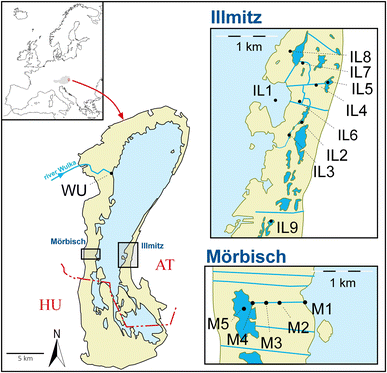 |
| Fig. 1 Map of Lake Neusiedl and of the sampling sites: river Wulka estuary (one sampling site) and the two test areas Illmitz (nine sampling sites) and Mörbisch (five sampling sites). | |
Table 1 describes the degree of connectivity of each sampling site with the open lake, with decreasing connectivity from level C1 (open lake itself) to C5 (completely isolated, with no connection to the open lake via a channel). The three intermediate levels are defined as follows: C2 (near lake – closely connected to the open lake via a broad reed channel), C3 (medium connectivity – connected to the open lake via a narrow reed channel with a distance < 600 m to the open lake) and C4 (low connectivity – connected to the open lake via a narrow reed channel with a distance > 600 m to the open lake). In addition, the sites differ from each other with respect to their exposure, namely their location within (or proximity to) large water areas within the inner reed belt. These categories are defined as well in Table 1, with decreasing exposure from level E1 (open lake) to E5 (small water area in the inner reed belt without any proximity to large open areas). To investigate the input via the Wulka river, a sampling site was located in the estuary just before the river flows into the open lake, after having passed through the reed belt.
Table 1 Characterization of the sampling sites with respect to their connectivity to the open lake and their location within or proximity to open water areas
Test area |
Sampling site |
Connectivity to open lake degree – description |
Location within or proximity to large open water areas degree – description |
Illmitz |
IL1 |
C1 |
Open lake |
E1 |
Open lake |
IL2 |
C2 |
Near-lake |
E3 |
Small water open area (less than 5000 m2) with close proximity to a large open water area |
IL3 |
C3 |
Medium connectivity |
E4 |
Small open water area (less than 5000 m2) with proximity to a large open water area |
IL4 |
C3 |
Medium connectivity |
E4 |
Small open water area (less than 5000 m2) with proximity to a large open water area |
IL5 |
C4 |
Low connectivity |
E2 |
Large open water area of several hectares within the inner reed belt |
IL6 |
C4 |
Low connectivity |
E5 |
Small open water area (less than 5000 m2) with no proximity to a large open water area |
IL7 |
C4 |
Low connectivity |
E2 |
Large open water area of several hectares within the inner reed belt |
IL8 |
C4 |
Low connectivity |
E2 |
Large open water area of several hectares within the inner reed belt |
IL9 |
C5 |
Isolated |
E2 |
Large open water area of several hectares within the inner reed belt |
Mörbisch |
M1 |
C1 |
Open lake |
E1 |
Open lake |
M2 |
C3 |
Medium connectivity |
E3 |
Small water open area (less than 5000 m2) with close proximity to a large open water area |
M3 |
C3 |
Medium connectivity |
E3 |
Small water open area (less than 5000 m2) with close proximity to a large open water area |
M4 |
C4 |
Low connectivity |
E2 |
Large open water area of several hectares within the inner reed belt |
M5 |
C4 |
Low connectivity |
E2 |
Large open water area of several hectares within the inner reed belt |
Sampling strategy, scope of analyzed parameters and chemical analyses
The scope of the analyses includes in the first place general physico-chemical parameters (water temperature: WT, dissolved oxygen: DO, electric conductivity: EC, pH), main ions (calcium: Ca2+, magnesium: Mg2+, sodium: Na+, potassium: K+, chloride: Cl−, sulphate–sulphur: SO4–S), acid-neutralizing capacity (ANC), phosphorus (total: TP, dissolved: DP, soluble reactive: SRP, soluble unreactive: SUP, particulate: PP), nitrogen (total: TN, dissolved organic: DON, nitrate–nitrogen: NO3–N, nitrite–nitrogen: NO2–N, ammonium–nitrogen: NH4–N, particulate: PN), soluble reactive silica (SRSi), total organic carbon (TOC), dissolved organic carbon (DOC), suspended solids (particulate matter: PM, particulate inorganic matter: PIM, particulate organic matter: POM), and chlorophyll-a (Chl a). For the analysis of these hydro-chemical parameters, sampling campaigns were carried out at all sites in October 2017, in March, July and October 2018, and in April 2019. In each of the first four campaigns, samples were taken three times at a weekly interval, while the last campaign consisted of one single sampling (exact dates are reported in Table A2†). As depicted in Fig. 2, in the study period the lakes' water level fluctuated over about 30 cm (115.3–115.6 m asl) and the sampling campaigns covered the whole range from the lowest to the highest water level conditions. The scope of trace contaminants included metals (cadmium: Cd, copper: Cu, lead: Pb, mercury: Hg, nickel: Ni, zinc: Zn), two industrial organic chemicals (perfluorooctanoic acid: PFOA and perfluorooctanesulfonic acid: PFOS), the sweetener acesulfame K and two pharmaceuticals (carbamazepine and diclofenac). These contaminants were selected to cover different sources and emission patterns (point and diffuse pathways, legacy or more recent pollution) and environmental behavior (e.g. different levels of persistence, biodegradability and adsorbability). For these analyses, samples were taken in both test areas at a selected subset of sites and dates from November 2017 to September 2019 (Table A3†). In the same period of time, other monitoring campaigns analyzed these contaminants in the Wulka river before its inflow in the reed belt estuary.32–35 With exception of total metals, which are likely partially deposited with suspended sediments in this area, for these contaminants no alteration is expected to occur within the reed belt estuary. Therefore, such measurements (Tables A4 and A5†) were included in this study to better assess the input via the river. Owing to the reduced data availability for metals and trace contaminants in the lake compared to the hydro-chemical parameters, to increase the sample size for the statistical analyses the data were grouped into the following three categories according to the connectivity of the sampling sites: “open lake”, “high-mid connected” and “low connected-isolated”. Table A6† indicates the resulting final sample sizes.
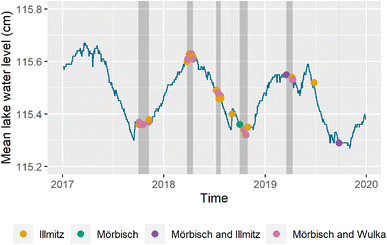 |
| Fig. 2 Mean water level fluctuations of Lake Neusiedl in 2017–2019. Dark grey areas and points indicate the dates at which sampling took place. | |
The sampling was carried out by a boat. Samples were taken in 0.5–2 L high density polyethylene (HDPE) bottles, with exception of samples destined to the analysis of Hg, which were collected in 0.5 L white glass bottles. The samples were immediately taken to the laboratories under cooled conditions. Filtration was carried out by 0.45 μm membrane filters. All analyses were carried out by certified laboratories according to the standard methods reported in Tables A7 and A8.†
Statistical analyses
To first explore the existence of spatial gradients and of common patterns of the analyzed parameters in the littoral zone of the lake (here intended as the boundary between reed belt and open lake), a principal component analysis (PCA) with prior log transformation and standardization (centering and scaling to unit variance) and the analysis of Spearman's correlation were carried out. Owing to the disparity in available sample size for trace contaminants, these two first analyses included exclusively the measured hydro-chemical parameters, for which all 14 sampling sites were sufficiently represented. In a second step, spatial differences within each of the two test areas were investigated in further detail via the Kruskal–Wallis test.36 To assess not only the existence of significant differences within each test area as a whole, but also to analyze which specific sites significantly differ from each other, the post hoc Dunn test37 was applied to perform pairwise comparisons between individual sites within every transect. Distribution-free methods were chosen because for many variables the data do not meet the assumptions required by parametric tests. The measurements for organic contaminants contain censored data, i.e. values below the limit of quantification (LOQ). For these substances, statistical descriptors were estimated with the method “regression on order statistics” (ROS) and data were pre-processed to apply the Kruskal–Wallis test and the post hoc Peto–Peto test for pairwise comparisons according to.38 All data processing and statistical analyses were performed with the software package R.39 In particular, for the analysis of censored data, the NADA2 package was used.40
Results and discussion
All laboratory measurements are available at https://doi.org/10.48436/ez2zc-pqa36. The full detailed results of the statistical analyses are reported in Section A3 of the Appendix: PCA summary statistics (Table A9); correlation matrix (Fig. A1 and A2†); statistical descriptors (mean, median, standard deviation per sampling site or group of sampling sites; Tables A10 and A11†); summary statistics of the Kruskal–Wallis test (Tables A12 and A13†); summary statistics of the post hoc tests for pairwise comparisons (Tables A14–A17†); boxplots for all analyzed parameters (Fig. A3–A31†). Here, the main results are presented and discussed with focus on identifying indicators of the driving underlying processes and mechanisms.
Spatial clusters
The presence of highly correlated variables does not pose per se a formal problem in the PCA procedure. However, including near-redundant variables can lead to overemphasize their contribution and to mask other potentially relevant factors. Since numerous measured hydro-chemical parameters are highly correlated with each other in the data set, this effect was explicitly investigated by performing the procedure with and without the following variables: ANC and all ions but Ca2+ due to correlation coefficients of 0.96–1 with EC; PIM due to a correlation of 0.98 with PM, DON and TOC due to a correlation of 0.95 with DOC. The variance explained by the principal components (PCs) is higher when including all variables. Whereas with the selected subset 2 PCs explain 51% and 3 PCS 66% of the variance respectively, these values rise to 66% and 76% by including all data. The difference is mostly due to the great contribution of ions in determining the variability of water chemistry in Lake Neusiedl, as found by.14 Besides this, the results are in both cases very similar and clearly identify two clusters of data points measured in the open lake and at the isolated site (Fig. 3). Despite a large internal variability, these two regions are separated without any overlap. The other three groups of data points show in contrast extended areas of intersection, yet with a perceivable gradual shift according to the connectivity grade, so that low connected sites overlap only to a minimum extent with the open lake and the same applies to near-lake and isolated sites. Another important insight gained through the PCA is the identification of variables, which play a dominant role in determining spatial clusters. In Fig. 3, the ten most contributing variables are shown. EC, DOC, TN and DP play a dominant role in differentiating the isolated site from the rest, while PM and to a lower extent TP mostly contribute to distinguishing the open lake and near lake from the remaining reed belt area. Due to the strong difference in turbidity between open lake and reed belt, the importance of particulate matter in distinguishing the open lake is not surprising. In an attempt to removing this macroscopic effect, the PCA was repeated excluding PM as variable. The results remained almost unchanged, both with respect to the identified clusters and to the explained variance. Nutrients were not identified as relevant parameters in determining clusters in the study of.14 The difference lies most likely in the different scope and thus in the different distribution of sampling points in the two studies, focusing more on the open lake and on the reed belt respectively, as well as in the choice of removing highly correlated variables in this analysis. One last important observation is that removing the highly correlated variables allowed identifying two parameters (pH and Ca2+), which would be otherwise masked and which seem to play an important role in explaining part of the variance along a third clustering direction. Although not shown among the 10 most contributing variables, DO has a contribution quite similar to that of pH. This is in line with the findings of,14 who identified lower pH and DO in reed belt channels to be determining in differentiating the water chemistry of these locations from the open lake.
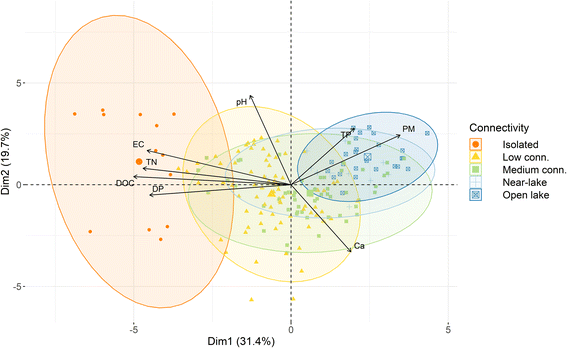 |
| Fig. 3 Biplot with results of the PCA analysis based on the measured hydro-chemical data after removal of the highly correlated variables. Coloured ellipses group the sampling sites according to their grade of connectivity to the open lake. Only the top ten contributing variables are shown. | |
Particulate matter and particulate-bound nutrients
As mentioned before, Lake Neusiedl is characterized throughout the whole year by high turbidity primarily provoked by calcite, dolomite/protodolomite precipitation coupled with a constant wind-induced turbulence. In combination with the prevailing wind and current conditions, this results in a distinct sediment distribution pattern.41–43 During the studied period, PM in the open lake had median values of 74–78 mg L−1, with the relative share of inorganic fraction fluctuating between 60% and 80%. Along both transects, the Kruskal–Wallis and Dunn tests indicate the existence of highly significant changes in the concentration level of suspended solids. In the gradient from open lake towards land the effect of wind and waves loses force, causing PM to sink and deposit on the bottom and leaving low median concentration levels in the water phase in the range of 8–25 mg L−1 (Fig. 4). Interestingly, in the Illmitz test area the lowest values for particulate matter and both of its fractions were found at IL6, which besides being low connected to the open lake, is also the sampling site with the lowest grade of exposure (E5). In contrast, the sites IL7, IL8, IL9, low connected or even completely isolated, but located within large open water areas (E2), are at the upper range of the concentration levels within the reed belt, similarly to the medium connected sites. Apparently, the surface area in these regions of the inner reed belt is sufficiently large for the wind action to effectively mix the water column and keep solids in suspension. Although POM and PIM generally follow the same pattern as PM, some considerable differences are noteworthy (Fig. 4). Whereas e.g. at the sites IL7, IL8 and IL9 the median value of PIM fluctuates at about 10–25% of its level in the open lake, POM is only about 50% lower. This points to a shift from lake, predominantly inorganic, turbidity to particulate organic matter produced locally within the reed belt. Unsurprisingly, the particulate-bound fractions of phosphorus and nitrogen present a highly significant correlation with particulate matter. The correlation coefficients for PN are however rather low and only in the Mörbisch site a clear and statistically significant decreasing pattern land-inward can be observed. In contrast, the very high correlation coefficients of PP (0.8, 0.85 and 0.9 with PIM, PM and POM respectively) are reflected in highly significant declines in both test areas (Fig. 4). It is straightforward that with the deposition of suspended particulate matter transported from the open lake especially PP is deposited and stored in the sediments as well. However, the lack of significant differences for PN and the similarity of PP concentrations in medium and low connected sites in Illmitz suggest the importance of additional factors and processes affecting particulate nutrients within the reed belt area.
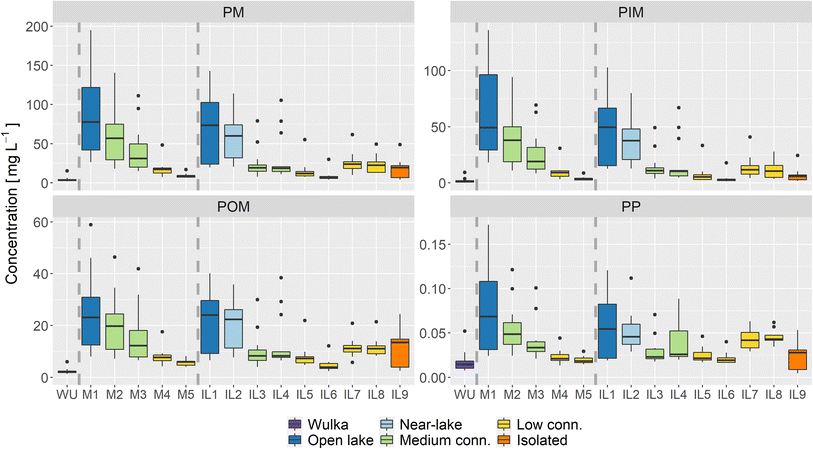 |
| Fig. 4 Boxplots of particulate matter (PM), particulate inorganic matter (PIM), particulate organic matter (POM) and particulate phosphorus (PP) in the Wulka estuary (left) and at each individual sampling site within the test areas Mörbisch (centre) and Illmitz (right), plotted in order of decreasing connectivity with the open lake. | |
Dissolved parameters
The results reveal a completely opposite spatial gradient for the dissolved parameters, with a tendency of rising concentrations in the reed belt along with decreasing connectivity. Within the transect in the Mörbisch area, the Kruskal–Wallis test identifies significant differences for EC, ANC and for all measured ions. The gradient, exemplified by Cl− in Fig. 5, is gradual and not of large magnitude (about 15% increase of the median values for each ion and for EC from the open lake to the last site), so that the Dunn test fails to find significant differences between single pairs of sites. In the Illmitz test area, the higher statistical significance of the tests for these parameters is most likely due to the isolated site IL9, in which the concentration of most ions and the EC level are twice as high as in the open lake or even higher. For the rest of the sites, an increasing but gradual tendency is visible, so that the Dunn test identifies only statistically significant differences between the open lake and the low connected IL5. Cl− is highly soluble and almost not involved in biological processes, thus its rising concentration land-inward points to an enrichment process driven by evapotranspiration. In this respect, it is important to consider that within the reed belt area, approximately from April to October, reed transpiration adds to evaporation from water surfaces.21,31 Another process probably underlying the higher concentration of most ions in the reed belt is the release and remobilization from the sediments. In this respect, it is important to highlight that not all ions behave in the same way in Lake Neusiedl. Whereas in the studied period Cl− showed an enrichment from the Wulka estuary to the open lake by a factor 3 (medians 84 to 260 mg L−1, respectively), Ca2+ dropped from 100 to 15 mg L−1 (Fig. 5). The importance of calcite precipitation for the characteristic turbidity of the lake has already been mentioned earlier and its higher tendency to precipitation is also visible in the reed belt. Its concentration in the water column, and its relative ratio to Mg2+, visibly decrease e.g. in the isolated site IL9. Further, the results reveal that within low connected and isolated regions of the reed belt with high EC the relative share of monovalent cations Na+ and K+ prevails over the bivalent cations Ca2+ and Mg2+.
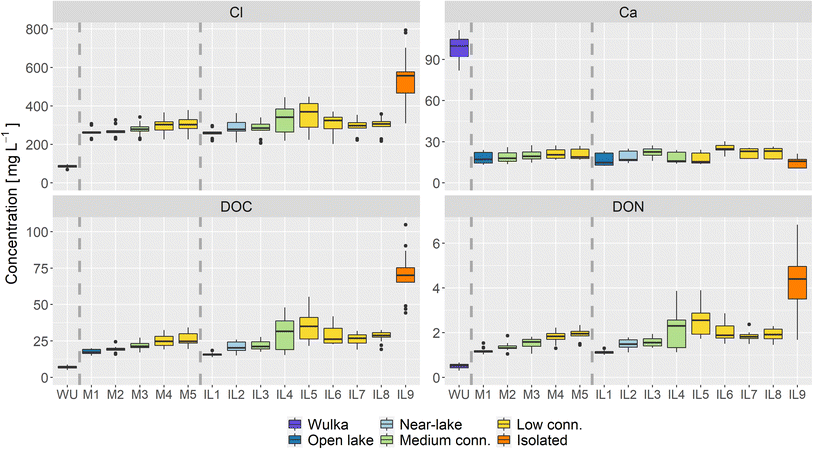 |
| Fig. 5 Boxplots of chloride (Cl−), calcium (Ca2+) dissolved organic carbon (DOC) and dissolved organic nitrogen (DON) in the Wulka estuary (left) and at each individual sampling site within the test areas Mörbisch (centre) and Illmitz (right), plotted in order of decreasing connectivity with the open lake. | |
Differences among ions regarding the evaporation-driven enrichment factor can also be attributed to the origin of salts in Lake Neusiedl. Earlier studies have hypothesized upwelling brines from mineral water deposits underneath Lake Neusiedl,44 while ascending groundwater inflows through faults were rejected more recently.45,46 A matter balance model calculated by18 for several years allowed predicting in-lake concentration changes of Cl− with high significance based mainly on loads from tributaries and wastewater discharges and only with a negligible share from other sources such as precipitation and groundwater inflow. However, discrepancies of the same model for sodium as well as significant differences in the evaporation-driven enrichment factors between Cl− and Na+ suggest that Na+ also enters the lake partly from groundwater inflows.47,48 Also, sediment pore water analyses point to brines with high NaSO4 and/or NaHCO3 concentrations.49 The enrichment factors of the ions (Ca2+ 0.2–0.3, Mg2+ 3.0–3.2, Na+ 6.6–7.5, K+ 3.0–3.2, ANC 1.7–2.0, Cl− 3.2–3.5, SO2−4 2.8–3.0) suggest similarities and differences of origin and allow conclusions on the main physical, chemical and biological factors influencing the salt concentrations in the lake. However, the behavior of different salts and the changes of their concentrations in time and space is more complex than outlined here in brief. Especially the role of upwelling brines for the lake's salt budget clearly requires further investigation.
DOC and DON originate mainly from the decomposition of reed and the production of aquatic humic acids.50 Therefore, they reach high concentrations in small water bodies within dense reed stands. However, they are also a subject to evaporation processes and exhibit a spatial gradient very similar to that of EC and Cl− (Fig. 5), with which they are highly correlated (r = 0.76–0.83, p < 0.001). The fact that their increase is more accentuated than for EC and Cl− (factor 2–4 from open lake to sites with highest levels) supports the hypothesis of generation of humic substances due to the degradation of reed material. Once generated, however, humic substances follow very similar evaporation-driven short-term concentration changes as dissolved salts, in spite of their different origin (salts mainly from the Wulka river, DOC/DON autochthonously in the lake).
As far as it concerns dissolved phosphorus, the inorganic fraction of dissolved nitrogen and soluble reactive silica, the results reveal a more complex picture. Contrary to DOC and DON, these parameters have a weak or very weak correlation with Cl− and EC. Thus, their concentration in the reed belt seems not to be dominated by evaporation, but rather by additional local processes. The substantial, statistically highly significant and almost linear increase of DP (Fig. 6) and SUP along both transects, and to a lower extent of SRP in Illmitz (for SUP and SRP see Fig. A11–A13†), may be explained via internal loading and remobilization of phosphorus previously transferred and stored into the sediments of the reed belt via deposition of particulate matter. An inverse decreasing gradient land-inward, though only slightly significant and of modest extent, can be observed for SRP in the Mörbisch area. This observation acquires special interest when coupled with the spatial gradients identified for SRSi (Fig. 6). This compound undergoes a clear and substantial decrease land-inward. The observed decline for SRP, but even more for SRSi, could be due to an uptake by algae (diatoms, chrysophytes) which exceeds the limited supply from the open lake in the more remote areas of the littoral zone. As shown in Fig. 6, in the pelagic zone NO3–N has remarkably low concentration levels, over an order of magnitude smaller than in the Wulka river estuary, where it enters the lake with a median concentration of 0.7 mg L−1. In both test areas, it further decreases land-inward, reaching in low connected areas even levels below analytical limits. NH4–N does not present clear spatial patterns, but its concentration level remains low (median below 100 μg L−1) along both transects. In the case of the inorganic forms of dissolved N, such low levels and even observed depletion are most likely the result of the ammonification, nitrification and denitrification processes, which transform the N imported into the lake and release it as N2, as well as N2O via incomplete denitrification, into the atmosphere. The important function of littoral and shallow waters for nitrogen retention primarily through the mechanism of denitrification has been amply reported in the literature.51–56 More in detail, the studies of57 and58 highlighted the enhancement of this process by Phragmites australis. Further, in an investigation of greenhouse gas emissions from Lake Neusiedl,59 identified the highest average diffusive emissions rates of N2O in the reed belt and at its lakeside margin, although at individual dates considerable emission rates were also observed in the open lake. The findings of this work provide thus further evidence of the significant contribution of the reed belt region in closing the N cycle within the Lake Neusiedl system.
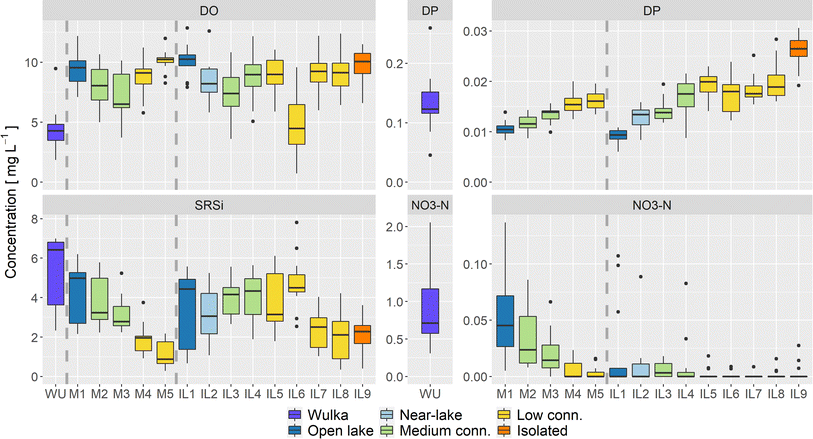 |
| Fig. 6 Boxplots of dissolved oxygen (DO), nitrate–nitrogen (NO3–N), dissolved phosphorus (DP) and soluble reactive silica (SRSi) in the Wulka estuary (left) and at each individual sampling site within the test areas Mörbisch (centre) and Illmitz (right), plotted in order of decreasing connectivity with the open lake. | |
A last noteworthy spatial gradient revealed by this study is the significantly lower pH and DO level in sites, which have at the same time a medium-low degree of connectivity and a reduced exposure, such as M3 (C3, E3) and IL6 (C4, E5). While in the open lake and in many areas of the reed belt pH is close to 9, at the site IL6 it drops down to 8.4 (median). With respect to DO, whereas median values fluctuate between 8 and 10 mg L−1 between open lake and reed belt without a clear gradient along the transects, the median concentration level drops down to 6.5 mg L−1 at M3 and 4.5 mg L−1 at IL6 (Fig. 6). The combination of low connectivity and low exposure simultaneously means a lower supply of DO from the well-mixed open lake water and a lower input from the air due to a smaller exchange surface. In addition, reduced light availability in dense reed stands (partly also due to the humic substance-rich reddish-brown colored water) limits oxygen production through photosynthesis, while on the other hand decomposition processes fuel oxygen consumption.60,61 This leads to the observed oxygen deficits in isolated areas of the reed belt. The imbalance between oxygen production and CO2 production by decomposition processes explains well the pH drop at site IL6.
Primary production in Lake Neusiedl is well studied since the 1970s,62–67 especially in the open lake, less extensively in the reed belt. Our knowledge on the decomposition of organic material is much poorer and can often only be indirectly inferred from hydro-chemical findings. A particular challenge is the strongly changing water level, which influences currents into and within the reed belt and thus causes varying oxygen availability. Apart from the redox conditions, degradation rates of reed are also dependent on inundation, as they are higher under water than above the water surface.57,68 Enhanced degradation, however, may in turn influence and aggravate the oxygen situation and even the reed structure, since prolonged anoxic phases may cause reed die-back through the production of toxic substances.69 As a consequence, reed structure and habitat quality can be significantly altered,70 and – via increased wind fetch and changed flow patterns – may in turn restore the importance of oxygen input from the atmosphere and through channels from the open lake. Similar antagonistic effects exist with respect to pH: while high pH values and the enormous surface area on the turbidity particles ensure very effective decomposition of organic material, exactly these conditions are not available in the reed belt. Especially in dense reed stand and isolated areas within the reed belt (e.g., sites IL6 and IL9), the water is clear and the pH much lower than in the open lake. Reduced degradation effectivity at low pH71 might be a factor responsible for the high concentrations of humic substances (DOC, DON).
Little is known about the microbiological processes behind the changes described.72 addressed bacterial community diversity in Lake Neusiedl, while73 focused on bacterioplankton productivity. According to their findings, bacterioplankton metabolism in Lake Neusiedl is heavily dependent on non-phytoplankton sources of DOC. However, these results were obtained during a period of high-water level, when the reed belt was largely inundated. They are certainly not equally valid during periods, when the reed belt is completely dry, as e.g. in autumn 2022. Such situations will probably be observed much more frequently and last longer in the future as a result of global warming and an increased negative water balance of the Lake Neusiedl.
Well-designed science-based management strategies to ensure the lake's good status in the future must consider the risk of increased nutrient loads, changes of internal nutrient cycling and changed processes of organic matter production, accumulation and degradation. This topic links to unsolved questions regarding the lake's fate under varying and increasingly low water levels and highlights the importance to deepen our understanding in organic matter transformation processes.
Trace contaminants
The perfluoroalkyl substances (PFAS) PFOA and PFOS have been in use in several applications and products for decades and due to severe concerns for human and ecological health they have been increasingly banned in Europe. Nevertheless, they are still emitted into the environment at considerable levels mostly due to legacy effects and they reach surface water bodies predominantly via wastewater effluent discharges, but also via diffuse pathways owing to their ubiquitous distribution.28 In the Wulka estuary, they were both found at median concentrations of 3 ng L−1, i.e. far above the environmental quality standard (EQS) of 0.65 ng L−1 for PFOS74 and the newly proposed EQS for PFAS.75 According to the results of this study, in the lake these two compounds have a very different fate. While PFOA undergoes a statistically highly significant increase reaching in the open lake a median concentration above 9 ng L−1 (Fig. 7), PFOS concentration drops down to a median of 0.8 ng L−1. For both compounds the concentration reached in the open lake remains almost unaltered across the littoral zone. Given the extreme environmental persistence and low to moderate adsorption affinity, the behavior of PFOA may be explained via the same enrichment mechanism induced by the evaporation observed e.g. for Cl−. Why PFOS behaves so differently is difficult to interpret. One possible hypothesis is the transfer in the sediments of the reed belt. Laboratory experiments on the adsorption and mobilization of these two compounds, conducted by76 specifically with sediments of Lake Neusiedl, identified a higher log
Koc coefficient for PFOS than for PFOA and thus support this hypothesis. However, they also indicated the labile equilibrium between adsorption and release processes for these substances and thus hinted at the risk of remobilization and potential reverse transport into the open lake. Another possible explanation for the elimination of PFOS lies in the process of photodegradation.77 revealed that although PFOS undergoes a relatively slow direct photolysis in pure water, the presence of humic acids can notably accelerate this elimination process. Further, they found that the enhancement effect of humic acids on the photolysis improves at pH values between 7 and 10. With an average pH of 8.5–9, the humic-rich78 Lake Neusiedl might thus offer favorable conditions for this degradation pathway.
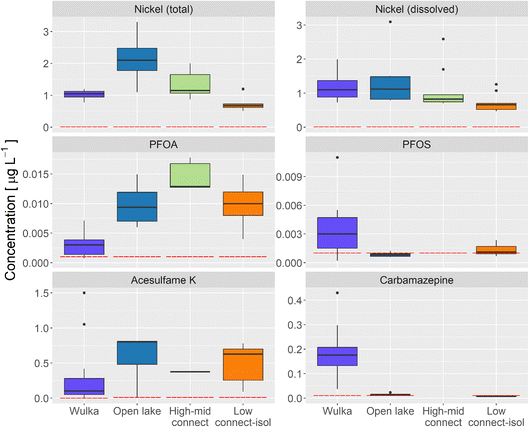 |
| Fig. 7 Boxplots of nickel (total and dissolved), PFOA, PFOS, acesulfame K and carbamazepine in the Wulka estuary and at three groups of sites within the lake according to the connectivity with the open lake: “open lake”, “high-mid connected” and “low connected-isolated”. Dotted red lines indicate the LOQ. For PFOS, acesulfame K and carbamazepine no boxplot could be generated for “high-mid connected” sites due to lack of sufficient measurements or sufficient values above LOQ. | |
Acesulfame K and the two pharmaceuticals are emitted almost exclusively via municipal wastewater effluent discharges, so that they are considered good indicators of this pathway in surface waters. The very persistent and poorly adsorbable acesulfame K is transported in the Wulka estuary at a median concentration of 0.1 μg L−1 and the results suggest a fate in the lake system similar to that of PFOA, although of smaller magnitude and not statistically significant (maybe due to the smaller sample size), namely an enrichment in the open lake, still visible across the reed belt. Carbamazepine (Fig. 7) and diclofenac enter the lake system with median concentration levels of 0.18 and 0.65 μg L−1, respectively. Once there, both decrease by one or even two orders of magnitude, being often found below the limit of quantification (0.001 μg L−1). These two compounds have low sorption affinity and very low biodegradability,79–81 but it is known that diclofenac can undergo fast photolysis/photodegradation in surface waters.82,83 In contrast, carbamazepine is considered highly persistent and previous studies conducted in Lake Greifensee and Lake Tegel revealed a low-moderate degradation with a half-life >70 days and >50–100 days and an annual elimination rate of 40%, respectively.82,83 Photodegradation can occur via two pathways, namely through direct absorption of sunlight and indirectly through degradation by reactive oxygen species produced e.g. from natural organic matter, nitrate and nitrite under sunlight.79 In this context, the laboratory experiments of84 revealed that the elimination of carbamazepine is mainly attributable to indirect photolysis, which is enhanced by DOM. It can thus be hypothesized that the combination of a long residence time, a shallow and well-mixed water column and the availability of photosensitizers creates particularly favorable conditions for the extensive photodegradation of this compound in Lake Neusiedl.
Metals have been transported into Lake Neusiedl for decades, both via point discharges and via erosion-driven diffuse emissions from the largely agricultural catchment of the lake. Contrary to the organic trace contaminants examined in this study, metals exhibit high adsorption affinity. Thus, the high turbidity in the open lake may be the reason for their significantly higher concentrations in the lake compared to the Wulka estuary (nickel is depicted by way of example in Fig. 7). Further, due to their high adsorbability, their fate follows the same spatial pattern observed for particle-bound phosphorus, namely a strong decline land-inward in the reed belt, due to the deposition of suspended solids. Interestingly, this pattern is observed not only for total concentrations. The dissolved fractions of Cu, Ni and Zn show a significant decrease by factor 2–4 from the open lake to the low connected sites.
Conclusions
For the first time, the spatial distribution of hydro-chemical parameters in Lake Neusiedl was investigated with an explicit and detailed focus on the reed belt area and on the fate of trace organic contaminants, for which little is known in this lake system. The results provide a so far lacking solid evidence of underlying processes and mechanisms, which had been previously hypothesized in the literature. The identified spatial gradients demonstrate that the water chemical composition in the reed belt and in the open lake is largely determined by the connectivity between these two regions of the lake as well as by the presence and characteristics of inner water areas, which influence local factors such as sedimentation, evapotranspiration and biological processes. This is of high relevance in a context of probably changing water levels in the future due to climate change, which could alter the connection between the open lake and different areas of the reed belt. The evaporation process was recognized as major driver for the concentration enrichment from the tributary river to the open lake of organic trace contaminants such as PFOA as well as of dissolved ions such as chloride. In combination with mechanisms of release from sediments and local production of humic acids, it was also found responsible for a further significant concentration enrichment of several dissolved parameters in low connected and isolated areas of the reed belt. In view of the predicted increasing evaporation in the future due to climate change, this is a major finding, which should be investigated in greater depth to assess how the exacerbation of this process will impact the chemism and the integrity of the lake. Interestingly, the results of this study reveal a substantial loss in the open lake of selected organic trace contaminants transported via the inflow tributary and which are typically considered persistent in the environment, such as carbamazepine and PFOS. Although these compounds undergo a rather slow direct photolysis, the availability of photosensitizers, the high pH, the wind-induced turbulence and the long residence time in Lake Neusiedl seem to offer very favorable conditions to enhance the process of indirect photodegradation.
Conflicts of interest
There are no conflicts to declare.
Acknowledgements
This work was carried out within the project “REBEN” (Reed Belt Management Neusiedler See/Fertő) funded by the EU INTERREG program (Project No. AT-HU, 2014–20). The authors acknowledge TU Wien Bibliothek for financial support through its Open Access Funding Programme. We thank three anonymous reviewers for their critical and constructive comments.
References
- M. Beklioğlu, M. Meerhoff, T. A. Davidson, K. A. Ger, K. Havens and B. Moss, Preface: shallow lakes in a fast changing world, Hydrobiologia, 2016, 778(1), 9–11 CrossRef.
- A. Bartczak, S. Słowińska, S. Tyszkowski, M. Kramkowski, H. Kaczmarek and J. Kordowski,
et al.
, Ecohydrological changes and resilience of a shallow lake ecosystem under intense human pressure and recent climate change, Water, 2019, 11(1), 32 CrossRef.
- M. T. Dokulil, E. de Eyto, S. C. Maberly, L. May, G. A. Weyhenmeyer and R. I. Woolway, Increasing maximum lake surface temperature under climate change, Clim. Change, 2021, 165(3), 56 CrossRef CAS.
- K. E. Havens and A. D. Steinman, Ecological responses of a large shallow lake (Okeechobee, Florida) to climate change and potential future hydrologic regimes, Environ. Manage., 2015, 55(4), 763–775 CrossRef PubMed.
- M. Tolotti, G. Guella, A. Herzig, M. Rodeghiero, N. L. Rose and G. Soja,
et al.
, Assessing the ecological vulnerability of the shallow steppe Lake Neusiedl (Austria-Hungary) to climate-driven hydrological changes using a palaeolimnological approach, J. Great Lakes Res., 2021, 47, 1327–1344 CrossRef.
-
L. De Meester, S. Declerck, J. H. Janse, J. J. Dagevos, R. Portielje, E. Lammens, et al., Biodiversity in European shallow lakes: a multilevel-multifactorial field study, in Wetlands: Functioning, Biodiversity Conservation, and Restoration, 2006, pp. 149–167 Search PubMed.
- J. Zhou, P. R. Leavitt, Y. Zhang and B. Qin, Anthropogenic eutrophication of shallow lakes: is it occasional?, Water Res., 2022, 221, 118728 CrossRef CAS PubMed.
- T. L. Crisman, C. Mitraki and G. Zalidis, Integrating vertical and horizontal approaches for management of shallow lakes and wetlands, Ecol. Eng., 2005, 24(4), 379–389 CrossRef.
- A. Idrizaj, A. Laas, U. Anijalg and P. Nõges, Horizontal differences in ecosystem metabolism of a large shallow lake, J. Hydrol., 2016, 535, 93–100 CrossRef CAS.
- S. Liu, Q. Ye, S. Wu and M. J. F. Stive, Horizontal circulation patterns in a large Shallow Lake: Taihu Lake, China, Water, 2018, 10(6), 792 CrossRef.
- M. A. Naumenko, V. V. Guzivaty and S. G. Karetnikov, Variability of the horizontal gradients of the air and the water surface temperatures in the vernal frontal zone period of Lake Ladoga, Oceanology, 2012, 52(6), 735–740 CrossRef.
- E. Csaplovics, Der Schilfgürtel des Neusiedler Sees, Österr. Wasser–Abfallwirtsch., 2019, 71(11), 494–507 CrossRef.
-
G. Wolfram, L. Déri and S. Zech, Strategiestudie Neusiedler See – Phase 1. Studie im Auftrag der Österreichisch-Ungarischen Gewässerkommission, Wien-Szombathely, 2014 Search PubMed.
- N. Magyar, I. G. Hatvani, I. K. Székely, A. Herzig, M. Dinka and J. Kovács, Application of multivariate statistical methods in determining spatial changes in water quality in the Austrian part of Neusiedler See, Ecol. Eng., 2013, 55, 82–92 CrossRef.
- M. Dinka, E. Ágoston-Szabó, Á. Berczik and G. Kutrucz, Influence of water level fluctuation on the spatial dynamic of the water chemistry at Lake Fertõ/Neusiedler See, Limnologica, 2004, 34(1), 48–56 CrossRef CAS.
- L. Forró and H. Metz, Observations on the zooplankton in the reedbelt area of the Neusiedlersee, Hydrobiologia, 1987, 145(1), 299–307 CrossRef.
-
BMLRT, Nationaler Gewässerbewirtschaftungsplan (River Basin Management Plan), Wien, 2021 Search PubMed.
-
G. Wolfram and A. Herzig, Nährstoffbilanz Neusiedler See, Wiener Mitteilungen, 2013, Report number: 228, pp. 317–338 Search PubMed.
- C. Sailer and K. Maracek, Der Neusiedler See – ein Überblick (Lake Neusiedl—an overview), Österr. Wasser–Abfallwirtsch., 2019, 71, 483–493 CrossRef.
- G. Blöschl, A. P. Blaschke, K. Haslinger, M. Hofstätter, J. Parajka and J. Salinas,
et al.
, Auswirkungen der Klimaänderung auf Österreichs Wasserwirtschaft – ein aktualisierter Statusbericht, Österr. Wasser–Abfallwirtsch., 2018, 70(9), 462–473 CrossRef.
-
J. Eitzinger, G. Kubu, H. Formayer, P. Haas, T. Gerersdorfer and H. Kromp-Kolb, Auswirkungen einer Klimaänderung auf den Wasserhaushalt des Neusiedlersees, Burgenländische Landesregierung, Wien, 2009 Search PubMed.
- M. Dinka, Schwermetallbelastung zweier seichter Seen (Neusiedler See und Balaton — Österreich und Ungarn), Mitt. Österr. Geol. Ges., 1991, 83(9), 22 Search PubMed.
- G. Lakatos, M. Kiss and I. Mészáros, Heavy metal content of common reed (Phragmites australis/Cay./Trin. ex Steudel) and its periphyton in Hungarian shallow standing waters, Hydrobiologia, 1999, 415, 47–53 CrossRef CAS.
-
O. Gabriel, K. Schilling, M. Pannonhalmi, L. Sutheo, M. Kovács, I. Toth, et al., Neusiedlersee – Ökodynamische Rehabilitation. Betrachtungen zur Wasserqualität der Raab. Wien, Budapest, Györ: Amt der Burgenländischen Landesregierung, Abteilung 9 – Wasser und Abfallwirtschaft, 2012 Search PubMed.
- F. Jirsa, D. Pirker, R. Krachler and B. K. Keppler, Total mercury in sediments, macrophytes, and fish from a shallow Steppe Lake in Eastern Austria, Chem. Biodivers., 2014, 11(8), 1263–1275 CrossRef CAS PubMed.
- J. Windisch, C. Plessl, C. Christian, T. Zechmeister and F. Jirsa, Unexpected pathways of mercury in an alkaline, biologically productive, saline lake: a mesocosm approach, J. Hazard. Mater., 2022, 427, 128163 CrossRef CAS PubMed.
-
A. Amann, M. Clara, O. Gabriel, G. Hochedlinger, M. Humer, F. Humer, et al., STOBIMO Spurenstoffe, Bundesministerium für Nachhaltigkeit und Tourismus, Wien, 2019, p. 371 Search PubMed.
- S. Kittlaus, M. Clara, J. van Gils, O. Gabriel, M. B. Broer and G. Hochedlinger,
et al.
, Coupling a pathway-oriented approach with tailor-made monitoring as key to well-performing regionalized modelling of PFAS emissions and river concentrations, Sci. Total Environ., 2022, 849, 157764 CrossRef CAS PubMed.
- P. Meister, S. Frisia, I. Dódony, P. Pekker, Z. Molnár and S. Neuhuber,
et al.
, Nanoscale pathway of modern dolomite formation in a Shallow, Alkaline Lake, Cryst. Growth Des., 2023, 23(5), 3202–3212 CrossRef CAS PubMed.
-
A. Herzig and M. Dokulil, Neusiedlersee – ein Steppensee in Europa, in Ökologie und schutz von Seen, Fakultas-Univ.-Verlag, 2001, pp. 401–415 Search PubMed.
- G. Soja, J. Züger, M. Knoflacher, P. Kinner and A. M. Soja, Climate impacts on water balance of a shallow steppe lake in Eastern Austria (Lake Neusiedl), J. Hydrol, 2013, 480, 115–124 CrossRef.
-
R. Braun, C. Hartmann, N. Kreuzinger, K. Lenz, H. Schaar and C. Scheffknecht, Untersuchung von Abwässern und Gewässern auf unterschiedliche toxikologische Endpunkte. Biologische Wirktests mittels in vitro Verfahren, Bundesministerium für Landwirtschaft, Regionen und Tourismus, Wien, 2020 Search PubMed.
-
M. Clara, C. Hartmann and K. Deutsch, Arzneimittelwirkstoffe und Hormone in Fließgewässern – GZÜV Sondermessprogramm 2017/2018, Bundesministerium für Nachhaltigkeit und Tourismus, Wien, 2019 Search PubMed.
- A. Ghirardini, O. Zoboli, M. Zessner and P. Verlicchi, Most relevant sources and emission pathways of pollution for selected pharmaceuticals in a catchment area based on substance flow analysis, Sci. Total Environ., 2021, 751, 142328 CrossRef CAS PubMed.
- O. Zoboli, M. Clara, O. Gabriel, C. Scheffknecht, M. Humer and H. Brielmann,
et al.
, Occurrence and levels of micropollutants across environmental and engineered compartments in Austria, J. Environ. Manage., 2019, 232, 636–653 CrossRef CAS PubMed.
- W. H. Kruskal and W. A. Wallis, Use of ranks in one-criterion variance analysis, J. Am. Stat. Assoc., 1952, 47(260), 583–621 CrossRef.
- O. J. Dunn, Multiple comparisons using rank sums, Technometrics, 1964,(6), 241–252 CrossRef.
-
D. R. Helsel, Statistics for Censored Environmental Data Using Minitab and R, Wiley, Hoboken, N.J, 2nd edn, 2012, p. 324, (Wiley series in statistics in practice) Search PubMed.
-
R Core Team, R: A Language and Environment for Statistical Computing [Internet], R Foundation for Statistical Computing, Vienna, Austria, 2022, Available at: https://www.R-project.org/ Search PubMed.
-
P. Julian and D. Helsel, NADA2: Data Analysis for Censored Environmental Data [Internet], 2021, Available at: https://github.com/SwampThingPaul/NADA2 Search PubMed.
-
M. Jungwirth, The superficial sediments: their characterization and distribution, in Neusiedlersee: The Limnology of a Shallow Lake in Central Europe, Springer, Dordrecht, 1979. pp. 139–143 Search PubMed.
-
A. Preisinger, Sediment, in Neusiedlersee: the Limnology of a Shallow Lake in Central Europe, Springer, Dordrecht, 1979, pp. 131–137 Search PubMed.
-
S. Józsa, T. Krámer and J. Sarkkula, Wind-Induced Hydrodynamics and Sediment Transport of Lake Neusiedl – Hungarian–Austrian–Finnish Research Cooperation from Lake-wide to Bay-wide Scale, Budapest University of Technology and Economics, Department of Hydraulic and Water Resources Engineering, Budapest, 2008 Search PubMed.
- V. Fritsch and A. F. Tauber, Die Mineralwässer des Neusiedlersee-Gebiets, Wiss Arb Aus Dem Burgenland, 1959, 21, 34–39 Search PubMed.
-
H. Häusler, Geologische Karte Österreich. Erläuterungen zu den Blättern 79 Neusiedl am See, 80 Ungarisch-Altenburg, 109 Pamhagen, Geologische Bundesanstalt, Wien, 2007 Search PubMed.
-
H. Häusler, Erläuterungen zur Geologischen Karte 78 Rust, Geologische Bundesanstalt, Wien, 2010 Search PubMed.
-
G. Wolfram, A. P. Blaschke and M. Zessner, Chemische und gewässerökologische Auswirkungen einer Dotation des Grundwassers im burgenländischen Seewinkel sowie des Neusiedler Sees mit Wasser aus der Moson-Donau, Amt der Burgenländischen Landesregierung, Eisenstadt, 2021 Search PubMed.
-
M. Zessner, O. Gabriel, K. Schilling, M. Pannonhalmi, L. Sutheo, M. Kóvacs, et al., Neusiedlersee – Ökodynamische Rehabilitation – Betrachtungen zur Wasserqualität der Raab, Amt der Burgenländischen Landesregierung, Wien, Budapest, Györ, 2012 Search PubMed.
- R. Krachler, R. Krachler, F. Gülce, B. K. Keppler and G. Wallner, Uranium concentrations in sediment pore waters of Lake Neusiedl, Austria, Sci. Total Environ., 2018, 633, 981–988 CrossRef CAS PubMed.
- F. Panis, R. F. Krachler, R. Krachler and A. Rompel, Expression, purification, and characterization of a well-adapted tyrosinase from peatlands identified by partial community analysis, Environ. Sci. Technol., 2021, 55(16), 11445–11454 CrossRef CAS PubMed.
- B. Moss, E. Jeppesen, M. Søndergaard, T. L. Lauridsen and Z. Liu, Nitrogen, macrophytes, shallow lakes and nutrient limitation: resolution of a current controversy?, Hydrobiologia, 2013, 710(1), 3–21 CrossRef CAS.
- E. Piña-Ochoa and M. Álvarez-Cobelas, Denitrification in aquatic environments: a cross-system analysis, Biogeochemistry, 2006, 81(1), 111–130 CrossRef.
- D. L. Saunders and J. Kalff, Denitrification rates in the sediments of Lake Memphremagog, Canada–USA, Water Res., 2001, 35(8), 1897–1904 CrossRef CAS PubMed.
- D. L. Saunders and J. Kalff, Nitrogen retention in wetlands, lakes and rivers, Hydrobiologia, 2001, 443(1), 205–212 CrossRef CAS.
- H. L. Golterman, Denitrification and a numerical modelling approach for shallow waters, Hydrobiologia, 2000, 431(1), 93–104 CrossRef CAS.
- J. Windolf, E. Jeppesen, J. P. Jensen and P. Kristensen, Modelling of seasonal variation in nitrogen retention and in-lake concentration: a four-year mass balance study in 16 shallow Danish lakes, Biogeochemistry, 1996, 33(1), 25–44 CrossRef.
- P. Hietz, Decomposition and nutrient dynamics of reed (Phragmites australis (Cav.) Trin. ex Steud.) litter
in Lake Neusiedl, Austria, Aquat. Bot., 1992, 43(3), 211–230 CrossRef CAS.
- Z. Yang, Y. Zhao and X. Xia, Nitrous oxide emissions from phragmites australis-dominated zones in a shallow lake, Environ. Pollut., 2012, 166, 116–124 CrossRef CAS PubMed.
- G. Soja, B. Kitzler and A. M. Soja, Emissions of greenhouse gases from Lake Neusiedl, a shallow steppe lake in Eastern Austria, Hydrobiologia, 2014, 731(1), 125–138 CrossRef CAS.
-
F. Neuhuber and L. Hammer, Oxygen conditions, in Neusiedlersee: The Limnology of a Shallow Lake in Central Europe, Springer, Dordrecht, 1979, pp. 121–130 Search PubMed.
- M. Dinka, Some characteristics of reed (Phragmites australis/Cav./Trin ex Steudel) that indicate different health between vigorous and die-back stands, Int. Ver Für Theor. Angew. Limnol. Verhandlungen, 2001, 27(6), 3364–3369 Search PubMed.
-
K. Burian and H. Sieghardt, The primary producers of the Phragmites belt, their energy utilization and water balance, in Neusiedlersee: the Limnology of a Shallow Lake in Central Europe, Springer, Dordrecht, 1979, pp. 251–72 Search PubMed.
- M. Dokulil and M. Dokulil, Planktonic primary production within the Phragmites community of Lake Neusiedlersee (Austria), Pol. Arch. Hydrobiol., 1973, 20, 175 Search PubMed.
-
M. Dokulil, Phytoplankton primary production, in Neusiedlersee: The Limnology of a Shallow Lake in Central Europe, Springer, Dordrecht, 1979, pp. 241–252 Search PubMed.
- M. Khondker and M. Dokulil, Seasonality, biomass and primary productivity of Epipelic Algae in a shallow lake (Neusiedlersee, Austria), Acta Hydrochim. Hydrobiol., 1988, 16(5), 499–515 CrossRef CAS.
- J. Padisák, Dynamics of phytoplankton in brown-water lakes enclosed with reed-belts (Fertő/Neusiedlersee; Hungary/Austria), Verh. – Int. Ver. Theor. Angew. Limnol., 1993, 25(2), 675–679 Search PubMed.
-
F. Schiemer, Submerged macrophytes in the open lake. Distribution pattern, production and long term changes, in Neusiedlersee: The Limnology of a Shallow Lake in Central Europe, Springer, Dordrecht, 1979, pp. 235–250 Search PubMed.
- T. Asaeda, L. H. Nam, P. Hietz, N. Tanaka and S. Karunaratne, Seasonal fluctuations in live and dead biomass of Phragmites australis as described by a growth and decomposition model: implications of duration of aerobic conditions for litter mineralization and sedimentation, Aquat. Bot., 2002, 73(3), 223–239 CrossRef.
- J. Armstrong and W. Armstrong, Phragmites die-back: toxic effects of propionic, butyric and caproic acids in relation to pH, New Phytol., 1999, 142(2), 201–217 CrossRef CAS.
- E. Nemeth and M. Dvorak, Reed die-back and conservation of small reed birds at Lake Neusiedl, Austria, J. Ornithol., 2022, 163(3), 683–693 CrossRef.
- R. F. Krachler, R. Krachler, A. Stojanovic, B. Wielander and A. Herzig, Effects of pH on aquatic biodegradation processes, Biogeosci. Discuss., 2009, 6(1), 491–514 Search PubMed.
- A. K. Borsodi, I. Farkas and P. Kurdi, Numerical analysis of planktonic and reed biofilm bacterial communities of Lake Fertő (Neusiedlersee, Hungary/Austria), Water Res., 1998, 32(6), 1831–1840 CrossRef CAS.
- B. Reitner, A. Herzig and G. J. Herndl, Dynamics in bacterioplankton production in a shallow, temperate lake (Lake Neusiedl, Austria): evidence for dependence on macrophyte production rather than on phytoplankton, Aquat. Microb. Ecol., 1999, 19(3), 245–254 CrossRef.
-
EU, Directive 2013/39/EU of the European Parliament and of the Council of 12 August 2013 Amending Directives 2000/60/EC and 2008/105/EC as Regards Priority Substances in the Field of Water Policy Text with EEA Relevance, 2013 Search PubMed.
-
EU, Proposal for a Directive of the European Parliament and of the Council Amending Directive 2000/60/EC Establishing a Framework for Community Action in the Field of Water Policy, Directive 2006/118/EC on the Protection of Groundwater against Pollution and Deterioration and Directive 2008/105/EC on Environmental Quality Standards in the Field of Water Policy, 2022 Search PubMed.
- D. Reif, O. Zoboli, G. Wolfram, A. Amann, E. Saracevic and P. Riedler,
et al.
, Pollutant source or sink? Adsorption and mobilization of PFOS and PFOA from sediments in a large shallow lake with extended reed belt, J. Environ. Manage., 2022, 320, 115871 CrossRef CAS PubMed.
- Z. Sun, C. Zhang, P. Chen, Q. Zhou and M. R. Hoffmann, Impact of humic acid on the photoreductive degradation of perfluorooctane sulfonate (PFOS) by
UV/Iodide process, Water Res., 2017, 127, 50–58 CrossRef CAS PubMed.
- B. Reitner, A. Herzig and G. J. Herndl, Photoreactivity and bacterioplankton availability of aliphatic versus aromatic amino acids and a protein, Aquat. Microb. Ecol., 2002, 26(3), 305–311 CrossRef.
- D. Löffler, J. Römbke, M. Meller and T. A. Ternes, Environmental fate of pharmaceuticals in water/sediment systems, Environ. Sci. Technol., 2005, 39(14), 5209–5218 CrossRef PubMed.
- A. Kiecak, L. Sassine, M. Boy-Roura, M. Elsner, J. Mas-Pla and C. Le Gal La Salle,
et al.
, Sorption properties and behaviour at laboratory scale of selected pharmaceuticals using batch experiments, J. Contam. Hydrol., 2019, 225, 103500 CrossRef CAS PubMed.
- E. R. Christensen, Y. Wang, J. Huo and A. Li, Properties and fate and transport of persistent and mobile polar organic water pollutants: a review, J. Environ. Chem. Eng., 2022, 10(2), 107201 CrossRef CAS.
- C. Tixier, H. P. Singer, S. Oellers and S. R. Müller, Occurrence and fate of carbamazepine, clofibric acid, diclofenac, ibuprofen, ketoprofen, and naproxen in surface waters, Environ. Sci. Technol., 2003, 37(6), 1061–1068 CrossRef CAS PubMed.
- S. Schimmelpfennig, G. Kirillin, C. Engelhardt, U. Dünnbier and G. Nützmann, Fate of pharmaceutical micro-pollutants in Lake Tegel (Berlin, Germany): the impact of lake-specific mechanisms, Environ. Earth Sci., 2016, 75(10), 893 CrossRef.
- M. W. Lam and S. A. Mabury, Photodegradation of the pharmaceuticals atorvastatin, carbamazepine, levofloxacin, and sulfamethoxazole in natural waters, Aquat. Sci., 2005, 67(2), 177–188 CrossRef CAS.
|
This journal is © The Royal Society of Chemistry 2023 |
Click here to see how this site uses Cookies. View our privacy policy here.