DOI:
10.1039/D0QM00484G
(Review Article)
Mater. Chem. Front., 2020,
4, 3190-3200
Pyrene-based metallocycles and metallocages: more than fluorophores
Received
14th July 2020
, Accepted 25th August 2020
First published on 26th August 2020
Abstract
Attributed to their synthetic accessibility, unique structural and intriguing photophysical features, pyrene moieties have recently been introduced into supramolecular coordination complexes (SCCs) such as metallocycles and metallocages as either functional groups or key panels. The resultant pyrene-based SCCs displayed not only attractive emission properties but also impressive binding abilities towards diverse guests, endowing them with wide applications in sensing, host–guest chemistry, light harvesting etc. In this review, a comprehensive summary of pyrene-based metallocycles and metallocages is presented, and the emphasis will be on the intriguing photophysical features and unique host–guest chemistry that the pyrene moieties bring to the SCCs.
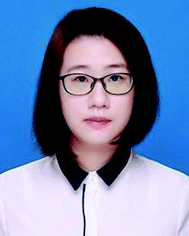
Xu-Qing Wang
| Xu-Qing Wang received her BS degree in chemistry from Northwest A & F University in 2014. She obtained her PhD degree from East China Normal University in 2019 under the supervision of Prof. Hai-Bo Yang. Then she joined Professor Yang's group as a Postdoctoral Fellow in June 2019. Her current research interest is focused on well-controlled self-assembly of supramolecular coordination architectures. |
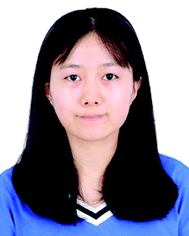
Qing-Hui Ling
| Qing-Hui Ling received her BS degree in applied chemistry from Anhui University of Science and Technology in 2019. She is now pursuing her MS degree in Prof. Hai-Bo Yang's group at East China Normal University. Her research interests focus on the design and synthesis of functional fluorescent metallacages. |
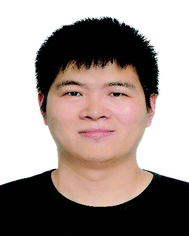
Wei Wang
| Wei Wang obtained his BSc degree from Sichuan University in 2011 and PhD degree from the East China Normal University in 2016 under the supervision of Prof. Hai-Bo Yang. He then joined Prof. A. Dieter Schlüter's group at ETH Zürich to pursue postdoctoral study (2016–2019). After that, he has become a research professor (Zijiang Young Scholar) at East China Normal University. His research interest includes interlocked macromolecules and topological chirality. |
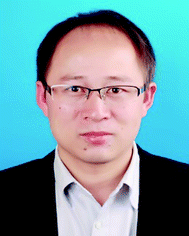
Lin Xu
| Lin Xu received his PhD degree in Chemistry from the East China University of Science and Technology (ECUST, Shanghai) in 2012 under the supervision of Professor Xuhong Qian. Subsequently, he joined the East China Normal University (ECNU, Shanghai) as an assistant professor and was promoted to an associate professor and a full professor in 2014 and 2018, respectively. He carried out two-years postdoctoral research at the University of Cambridge with Prof. Jonathan Nitschke during 2015–2017. His research interests focus mainly on supramolecular fluorescent chemistry. |
Introduction
During the past few decades, on the basis of the relatively rigid yet dynamic metal–ligand coordination bonds, coordination-driven self-assembly has evolved as a general and powerful strategy towards the construction of diverse delicate and elegant two-dimensional (2D) and three-dimensional (3D) supramolecular coordination complexes (SCCs) such as metallocycles and metallocages.1 Taking advantage of their well-defined geometry and well-tuned cavities as well as well-arranged functional groups within their skeletons, the resultant SCCs have shown wide applications in diverse fields such as host–guest chemistry, catalysis, drug delivery, functional materials, and so on.2 Recently, increasing interest in the construction of fluorescent SCCs has been witnessed since they can serve as privileged platforms for sensing study, the mimicking of natural photosynthetic processes, the fabrication of photoelectric devices, etc.3 In general, the fluorophores can be introduced as either functional groups that are attached to the key building blocks or key panels that are incorporated into the main skeletons of the targeted fluorescent SCCs.
As one of the most common and useful fluorophores, pyrene is an excellent candidate for the construction of novel fluorescent SCCs.4 First of all, the synthetic accessibility of pyrene derivates with diverse functional groups and well-defined structural features lays the foundation for the construction of diverse SCCs with pyrene moieties either as functional groups or building blocks; secondly, compared with other fluorophores, pyrene derivates usually displayed intriguing photophysical properties, such as high fluorescence quantum yield, relatively long excited-state lifetime, unique excimer emission etc. Notably, the fluorescence bands for the monomer and excimer of pyrene derivates reveal exceptional distinction and, moreover, their excitation spectra are very sensitive toward the changes of microenvironments.5 Taking advantage of all these attractive photophysical features, pyrene derivates can serve as versatile fluorophores for the incorporation of expected photophysical functions into the resultant SCCs. Last but not least, the relatively large conjugated π-electron-rich surface of pyrene derivates makes them excellent candidates as panels for the construction of SCCs with well-defined and confined pockets for the investigations of host–guest chemistry. During the past few years, a growing number of reports on the construction of pyrene-based SCCs has been witnessed, and thus it is time to summarize these developments and achievements. Herein, divided by the different types of SCC cores, pyrene-based 2D metallocycles and 3D metallocages will be discussed, with an emphasis on the intriguing photophysical features and unique host–guest chemistry that the pyrene moieties bring to the SCCs.
Pyrene-based 2D metallocycles
Aiming at mimicking natural light harvesting systems with circular dye arrays, Würthner and coworkers6 reported the construction of a well-defined molecular square 2, in which sixteen pyrene and four perylene bisimide chromophores are arranged in a circular way (Scheme 1). In the resultant metallocycle, the outer pyrene moieties served as antenna dyes, from which highly efficient and fast photo-induced energy transfer as well as very fast and efficient electron transfer to the inner perylene bisimide chromophores was demonstrated. Such photophysical processes were clearly confirmed by the combination of steady-state and time-resolved emission as well as femtosecond transient absorption studies. More impressively, compared with the free ligand 1, the metallocycle 2 revealed substantially faster and more efficient electron-transfer processes, making it a novel monodisperse nanoaggregate with well-defined multiple-chromophore arrangements.
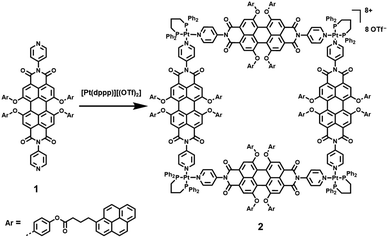 |
| Scheme 1 The construction of molecular square 2 with sixteen pyrene and four perylene bisimide chromophores arranged in a circular way. | |
On the basis of the coordination-driven self-assembly strategy, by employing various pyrene-modified ligands, Yang and coworkers7 demonstrated a simple and highly efficient approach for the construction of multipyrene hexagonal metallocycles with well-defined shape and size in 2013. As shown in Scheme 2, a mixture of pyrene-functionalized 120° Pt(II) acceptor 3 with a 120° or 180° dipyridyl donor resulted in the formation of corresponding multipyrene hexagons 4 or 5 with three or six pyrene moieties, respectively. In addition, the combination of pyrene-functionalized 120° dipyridyl acceptor 6 and a 120° or 180° Pt(II) acceptor could also give rise to similar tris- and hexakis(pyrene) hexagonal metallocycles 7 and 8, respectively. Interestingly, preliminary spectroscopic study of these resultant hexagonal metallocycles revealed dramatically different emission behaviours. For instance, hexagons 7 and 8 displayed a longer fluorescence emission band at λmax 550 nm in CH2Cl2, which corresponded to the excimer emission of the pyrene chromophore. However, hexagons 4 and 5 could not form excimers at the same conditions. The results indicated that the combination mode of building blocks played an important role in the excimer formation of the resulting self-assemblies, which might be reasonably explained by the varied charge densities of the resultant hexagons originating from the different combinations. Furthermore, by employing the same directional metal–ligand bonding strategy, the same group8 reported a series of pyrene-functionalized metallodendrimers 11 and 12 derived from pyrene-containing 120° Pt(II) acceptors (3 and 9) and 60° dendritic donors 10 (Scheme 3). Notably, compared to the pyrene-containing precursors, improved fluorescence quantum yields of the corresponding pyrene-modified dendrimers were observed, which might be due to the fact that the steric hindrance of dendrons inhibited the aggregation of pyrenes.
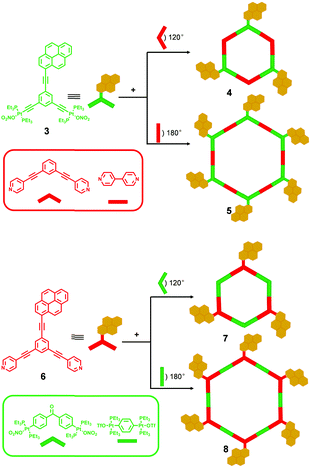 |
| Scheme 2 Coordination-driven self-assembly of multipyrene hexagonal metallocycles 4, 5, 7, and 8. | |
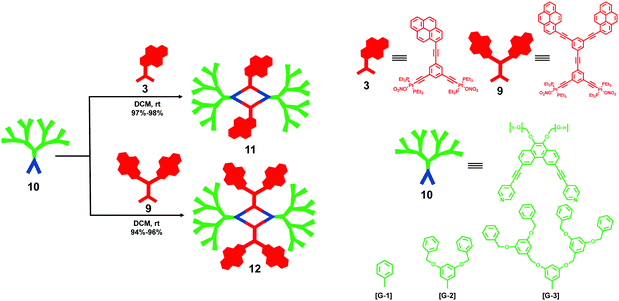 |
| Scheme 3 Coordination-driven self-assembly of multipyrene hexagonal metallocycles 11 and 12. | |
Similarly, Stang et al.9 prepared two rhomboids 15 and 16 by mixing pyrene-functionalized 120° donor 13 and 60° Pt(II) acceptor 14 (Scheme 4) via [2+2] self-assembly. Due to the existence of pyrene groups, both rhomboids were emissive and exhibited excimer emission bands, which derived from intermolecular interactions. Additionally, it was found that both the length of the spacers between the metallocycles and pyrene units and the solvent polarity had a great influence on the emission properties. For instance, as revealed by the emission spectra, rhomboids 15 and 16 displayed very different emission bands in sole CH2Cl2 solution. Meanwhile, the increase of the fraction of CH3OH in CH2Cl2 could further remarkably modulate the emission behaviours of both rhomboids.
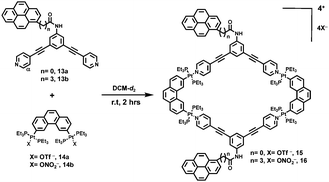 |
| Scheme 4 Synthesis of pyrene-functionalized molecular rhomboids 15 and 16. | |
As shown in Fig. 1, the three-component coordination-driven self-assembly of pyrene-cored dipyridyl donor 17, a 90° di-Pt(II) acceptor [Pt(PEt3)2][(OTf)2] and dicarboxylates 18–20 resulted in the formation of three pyrene-based metallocycles 21–23, respectively, which exhibited enhanced solubility in common organic solvents compared with the pyrene-cored ligand 17. The resultant three metallocycles displayed similar emission properties in the solution state, but different emission wavelengths in the aggregated states. Interestingly, it was found that the solution emission of these metallocycles could be well-tuned through the modulation of the temperature and solvent, which is expectable since both factors could remarkably influence the aggregation states of the metallocycles mainly formed through the intermolecular π–π interaction between the pyrenyl moieties. Notably, taking advantage of the excellent photochemical stability, a homemade aromatic amine sensing platform with ligand 17-based or metallocycle 22-based fluorescent films as the core structure was built. More importantly, regarding the response speed and recovery time, the metallocycle 22-based one revealed superior sensing performance (Fig. 1B), indicating that the formation of the metallocycles contributed a remarkable enhancement effect in the film state.10
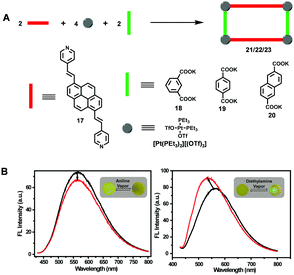 |
| Fig. 1 The construction of pyrene-based metallocycles 21–23via coordination-driven self-assembly (A) and the sensing performance of 22-based fluorescent films towards aniline (left) and diethylamine (right) (B). Adapted with permission from ref. 10. (Copyright 2019 American Chemical Society.) | |
In 2019, Stang et al.11 developed a new strategy for the construction of a cross-linked supramolecular polymer based on platinum(II) metallocycles. As shown in Fig. 2, self-assembled [2+2] metallocycle 26 was prepared by mixing pyrene-functionalized donor 24 with 180° di-Pt(II) acceptor 25, which contained two pyrene moieties at the periphery. Attributed to the existence of C–H⋯π interactions, host–guest interactions between the metallocycle core and the pyrene periphery were observed, as indicated by solid-state single-crystal structure and solution-state NMR study, respectively. Moreover, based on such an interesting driving force, a cross-linked supramolecular polymer was spontaneously formed in both the solid and solution state. Similar to other platinum metallocycle-based supramolecular polymers,12 the resultant cross-linked supramolecular polymer displayed interesting thermo-responsiveness and ion responsiveness. For instance, reversible thermo-induced phase transitions of the gel-like viscous liquids prepared from a highly concentrated solution of 26 were observed. In addition, attributed to the breaking and reforming of the Pt–N bonds in metallocycle 26, a reversible transition between the gel-like supramolecular polymer and turbid solution was successfully achieved through the addition and removal of bromide anions.
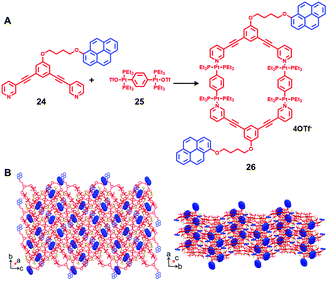 |
| Fig. 2 The construction of metallocycles 26 bearing two pyrene moieties via coordination-driven self-assembly (A) and the single-crystal structure of the cross-linked supramolecular polymer of 26 (B). Adapted with permission from ref. 11. (Copyright 2019 American Chemical Society.) | |
Based on their continuous interest in poly-N-heterocyclic carbenes (NHCs),13 in 2017, Peris and coworkers14 demonstrated the preparation of a novel dimetallic complex 28 by mixing pyrene-linked di-NHC ligand 27 with nickelocene. Then, based on a metal-directed self-assembly strategy, in the presence of AgBF4, the reaction of 28 with pyrazine or 4,4′-bipyridine resulted in the successful synthesis of nickel-cornered metallo-rectangles 29 and 30, respectively (Scheme 5). The resultant molecular rectangles were then tested to serve as receptors for the recognition of polycyclic aromatic hydrocarbons (PAHs). Due to the varied length of one of their sides, rectangle 29 could host one guest molecule within its cavity, whereas rectangle 30 was able to encapsulate up to two guest molecules, and the binding affinities strongly depended on the dimensional matching between the metallocycle hosts and guests. Notably, both rectangles could be used as efficient PAH scavengers in heptane though the formation of insoluble host–guest complexes.
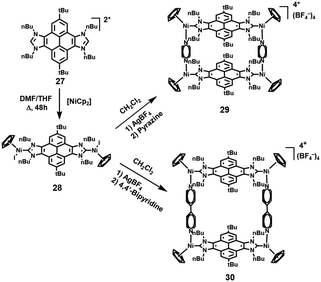 |
| Scheme 5 The synthesis of nickel-cornered metallo-rectangles 29 and 30. | |
Starting from the same pyrene-linked di-NHC ligand, the same group15 further prepared a palladium-cornered metallosquare 31 in 2019 via the metal-directed self-assembly strategy. Due to the existence of the flat polyaromatic surface of the four pyrene moieties, the resultant 3D cubic box with a large internal cavity could encapsulate both C60 and C70 fullerenes. The molecular structures of 31, C60@31 and C70@31 were determined by single-crystal X-ray diffraction (Fig. 3). More importantly, as revealed by these solid-state structures, the resulting metallo-square was size-flexible and adaptable, which was able to adapt its size to the size of guest molecules. Furthermore, taking advantage of the excellent spin-converting properties of fullerenes, Peris et al. employed both inclusion complexes C60@31 and C70@31 as photochemically stable photosensitizers to efficiently generate singlet oxygen, which could oxidize a series of cyclic and acyclic alkenes at room temperature.16 In addition, considering that the distance between the palladium atoms of metallosquare 31 is about 13 Å, which is large enough to host three polyaromatic guests, metallo-square 31 was further employed for the encapsulation of three heteroguests including two electron-rich PAH molecules such as pyrene, triphenylene or coronene and one electron-poor N,N′-dimethyl-naphthalene-tetracarboxy diimide (NTCDI) molecule, giving rise to the formation of interesting quintuple D–A–D–A–D stacks (Fig. 4).17
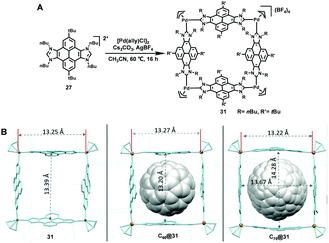 |
| Fig. 3 The preparation of palladium-cornered metallosquare 31 (A) and the X-ray diffraction structures of 31 (left), C60@31 (middle), and C70@31 (right) (B). Adapted with permission from ref. 15. (Copyright 2019 Wiley-VCH.) | |
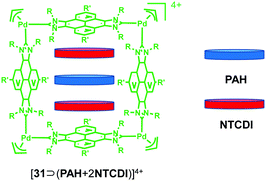 |
| Fig. 4 The formation of the quintuple D–A–D–A–D stacks based on palladium-cornered metallosquare 31. | |
Recently, as an extension of their previous work, Peris et al.18 demonstrated the synthesis of a novel tetragold metallo-rectangle 34 by the reaction of the pyrene-bis-imidazolydene-gold(I) complex 33 and di-tert-butyl-diethynyl-carbazole 32 in the presence of NaOH as a base. Due to the existence of two cofacial pyrene panels separated by almost exactly 7 Å within its structure, the resultant tetragold metallo-rectangle was able to encapsulate a series of PAHs, such as anthracene, pyrene, triphenylene, perylene, coronene and corannulene (Fig. 5). It was found that for the planar PAH guests, the binding affinities increased with the number of electrons, which derived from dimensional matching. Meanwhile, for a bowl-shaped guest such as corannulene, a mutual induced-fit arrangement in the inclusion complex with a remarkable compression of the guest molecule and the concomitant expansion of the metallo-host was revealed, which was accompanied by a reduced binding affinity.
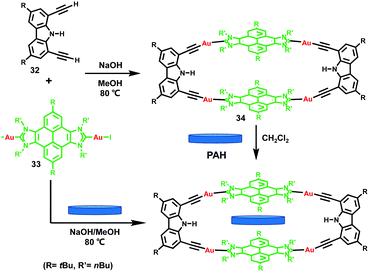 |
| Fig. 5 The synthesis of the tetragold metallo-rectangle 34 and its complexes with diverse PAHs. | |
Pyrene-based 3D metallocages
Similar to the cases of 2D metallocycles as described above,19 pyrene could also be introduced into 3D metallocages as functional groups that endowed novel photophysical properties such as attractive emission properties as described below to the resultant SCCs. For instance, Johnson and coworkers20 demonstrated the preparation of a novel class of polymer network, i.e. star PolyMOCs, in which metal–organic cages (MOCs) served as junctions that were cross-linked by polymer chains. Starting from bispyridine-functionalized tetra-arm star polymers 35, small bispyridine ligands 36, and Pd2+ ions, a three-component assembly approach led to the modular synthesis of star polyMOC gels with Fujita's famous M12L24 MOCs as junctions (Fig. 6A). More importantly, by varying the amounts of the small bipyridine ligands and Pd2+ ions while keeping the concentration of polymer constant, a series of polyMOC gels with well-tailored structures, mechanical properties and dynamics was successfully constructed. In order to further install functionality into the resultant polyMOC gels, the pyrene-modified bispyridine ligand 37 was introduced as the fourth component to take part in the self-assembly process, which gave rise to novel fluorescent metallogels. As expected, upon the increase of the amount of the incorporated pyrene-modified bispyridine ligand, the fluorescence intensity of the corresponding metallogels is qualitatively proportional (Fig. 6B).
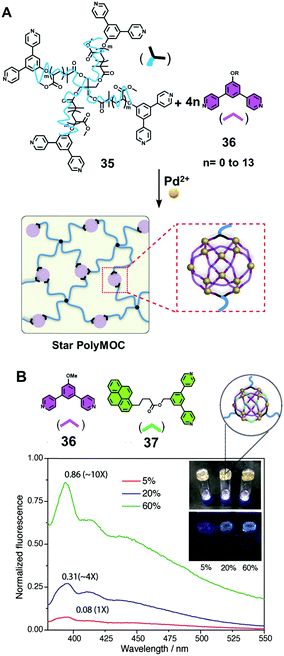 |
| Fig. 6 The preparation of the star PolyMOCs via a three-component assembly approach (A) and the incorporation of pyrene moieties as functional groups (B). Adapted with permission from ref. 20. (Copyright 2017 Wiley-VCH.) | |
By using bis(aminophenyl)BODIPY and pyrene-appended formylpyridine as key subcomponents, a novel fluorescent metallo-supramolecular capsule 38 was successfully prepared by Nitschke and coworkers21 through the subcomponent self-assembly approach (Fig. 7A). In the resultant metallocage, the BODIPY moieties arranged along the edges and the pyrene moieties located at the vertices of the cage. Upon the binding of anionic guests such as acetate anions, a significant enhancement in the fluorescence of BODIPY was observed, while the pyrene fluorescence remained unchanged, which indicated that the binding of the anions was within the cage cavity. More interestingly, white light emission was observed when mixing metallocage 38 and perylene in a 1
:
3 ratio. Upon interacting with perylene, the emission intensity of the BODIPY moieties in the red region was remarkably enhanced, which played a key role in the generation of white emission (Fig. 7B). A control experiment, in which the mixture of the corresponding subcomponents as well as perylene only resulted in fluorescence in the blue and green regions rather than white emission, confirmed the importance of the cage architecture and its guest binding behaviour for white light emission.
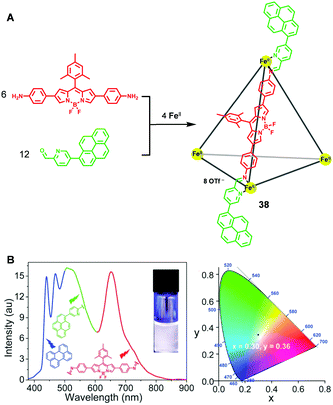 |
| Fig. 7 The construction of the tetrahedral metallocage 38via the subcomponent self-assembly approach (A) and the white light emission of the complex of the resultant metallocage and perylene (B). | |
Aiming at the design of novel fluorescent receptors for explosive detection, Mukherjee and coworkers22 demonstrated the construction of the first example of a platinum(II) tetragonal prism 41 through the two-component self-assembly of a new star-shaped pyrene-based planar Pt4 acceptor 39 and an amide-based “clip” donor 40 (Fig. 8). Attributed to the existence of highly conjugated tetraethynylpyrene moieties, the resultant self-assembled prism displayed an excellent fluorescence quenching response even at parts per billion (ppb) concentrations of TNT. More importantly, compared with the planar tetratopic acceptor 39, superior selectivity of prism 41 towards the nitro-aromatic explosives (NAEs) was observed, thus highlighting the important role of the π-electron-rich confined nanopocket for NAE encapsulation and detection. It is worth noting that, by employing a C–H-activation-directed assembly approach, Jin and coworkers23 demonstrated the successful synthesis of a cuboid-shaped metallosupramolecular structure, in which two pyrene moieties also served as the floor and roof.
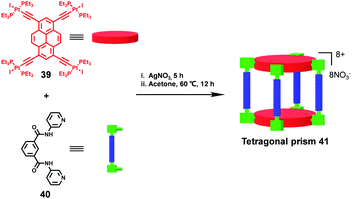 |
| Fig. 8 The construction of the tetragonal prism 41via coordination-driven self-assembly for nitroaromatic sensing. | |
Through the self-assembly of aforementioned bis-nickel-pyrene-di-imidazolylidene complex 28 with 1,3,5-tripyridyl-triazine (TPT) in the presence of AgBF4, Peris et al.24 demonstrated the construction of a nickel-conjoined molecular prism 42 in 2018, whose molecular structure was determined by X-ray diffraction (Scheme 6). Similar to previously described metallo-square 31, the resultant metallocage was also size-flexible and adaptable, which could also encapsulate both C60 and C70 fullerenes. Moreover, as evaluated by the binding constants, the metallocage preferred complexing with C70 over C60, which was further confirmed by competitive experiments.
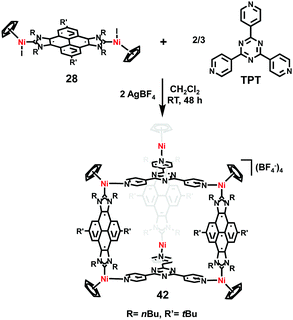 |
| Scheme 6 The synthesis of nickel-cornered metallocage 42 for the encapsulation of fullerenes. | |
Considering the privileged role of pyrenes as planar and electron-rich panels for guest binding, two novel pyrene-edged metallocages 43 and 44 based on 1,6-pyrene and 2,7-pyrene scaffolds, respectively, were prepared by Nitschke and coworkers25 though the subcomponent self-assembly approach (Scheme 7). As revealed by the 1H NMR spectra, both metallocages consisted of three diastereomers, i.e. homochiral T (ΔΔΔΔ/ΛΛΛΛ), heterochiral C3 (ΔΔΔΛ/ΛΛΛΔ) and achiral S4 (ΔΔΛΛ). The ratios of these diastereomers for both metallocages were similar, both of which were close to the expected statistical distribution. Guest binding tests indicated that these two isomeric pyrene-edged metallocages displayed very different host–guest properties. For metallocage 43 based on a 1,6-pyrene scaffold, attributed to its well-enclosed cavity, it could serve as an excellent host for diverse guests ranging from large hydrophobic guests including fullerenes and PAHs to large, structurally complex natural products such as steroids. Interestingly, along with the fullerene binding processes, altered diastereomer distributions of 43 were observed, leading to the amplification of the diastereomers that can maximize the binding affinity. Meanwhile, in the case of metallocage 44 based on a 2,7-pyrene scaffold, due to the existence of an open cavity, it was unable to bind neutral guests. In a following paper,26 the same group further examined the important role of the cavity enclosure for strong guest binding. In this study, besides pyrene, other PAHs such as triphenylene, naphthalene, anthracene and chrysene were introduced as the edges, leading to a series of aromatic-paneled metallocages. By evaluating the binding affinities of these resultant metallocges towards diverse guests, metallocages with large, offset aromatic panels such as chrysene that could maximize the cavity enclosure were proven to be the most effective hosts.
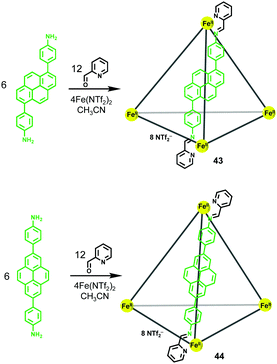 |
| Scheme 7 The synthesis of pyrene-edged metallocages 43 and 44via subcomponent self-assembly. | |
By using two isomeric bis(3-aminophenyl)pyrene derivatives A and B, rather than the isomeric bis(4-aminophenyl)pyrene derivatives described above, as key subcomponents, two unique metallosupramolecular structures, i.e. a C2-symmetric MII4L6 assembly 45 with meridionally coordinated metal centers, and a C3-symmetric self-included MII4L6 assembly 46 with facially coordinated metal centers (MII = FeII, CoII, and ZnII), were constructed by Nitschke and coworkers27 through the subcomponent self-assembly approach.
As revealed by the solid-state structures, face-to-face π-stacking interactions between the pyrene moieties were observed in both structures, which helped to stabilize the skeletons. Interestingly, the self-assembly of these two diamines, 2-formylpyridine (12 equiv.) and Zn(NTf2)2 in a 3
:
3
:
12
:
4 ratio resulted in the formation of a clean mixture of the homoleptic assemblies 45-Zn and 46-Zn, indicating a narcissistic self-sorting process that might be due to the geometrical differences as well as aforementioned favorable π-stacking within each individual metallosupramolecular structure. Furthermore, considering the favourable donor–acceptor stacking interactions between the pyrene units and naphthalenediimide (NDI) units, NDI-based diamine C was additionally chosen as a key subcomponent for the construction of heteroleptic metallosupramolecular assemblies. As expected, through either the direct subcomponent self-assembly approach (path v) or supramolecular transformation approach (path vi) as shown in Scheme 8, heteroleptic assemblies 47 were successfully prepared, from whose solid-state structure pyrene–pyrene–NDI stacks as the most favorable arrangements were identified.
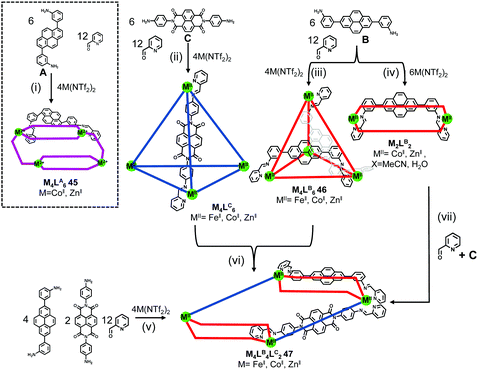 |
| Scheme 8 The preparation of pyrene-based homeleptic and heteroleptic metallosupramolecular assemblies via subcomponent self-assembly. | |
Conclusion and outlook
During the past decades, more and more attention has been paid to the construction of pyrene-based SCCs including 2D metallocycles and 3D metallocages. Starting from pyrene-functionalized building blocks or pyrene derivates themselves as key building blocks, diverse pyrene-based SCCs have been successfully constructed. For pyrene-based 2D metallocycles, more attention was paid to the investigation of their photophysical properties originating from the pyrene moieties. Meanwhile, in the case of pyrene-based 3D metallocages, due to the existence of well-defined cavities with pyrene panels, research interest mainly focusing on the host–guest chemistry of such novel metallo-hosts was witnessed. Attributed to the unique structural and intriguing photophysical features of the incorporated pyrene units, the resultant SCCs displayed not only attractive emission properties but also impressive binding abilities towards diverse guests, endowing them with wide applications in sensing, host–guest chemistry, light harvesting, and so on. Although great achievements have been made in this area, the research on this topic is still in its infancy, and more in-depth investigations are in great demand. To name a few, firstly, aiming at further biological applications, the design and synthesis of water-soluble and biocompatible pyrene-based SCCs will be of great importance. Secondly, considering the wide applications of pyrene-based materials for organic electronics, how to construct and employ novel pyrene-based SCCs for the fabrication of photoelectric devices will be worth being further explored. Thirdly, regarding pyrene-based metallo-hosts, the expansion of the scope of guests and the improvement of the selectivity are still needed, which will be essential for practical applications such as separation, sensing, controllable capture/release etc. Considering the great power of coordination-driven self-assembly in the facile and efficient construction of novel SCCs with increasing complexity, a bright future of pyrene-based metallocycles and metallocages will be anticipated.
Conflicts of interest
The authors declare no competing interest.
Acknowledgements
X.-Q. W. is grateful to the Guanghua Excellent Postdoctoral Scholarship and China Postdoctoral Science Foundation (No. 2019M660084 and 2020T130198) for support. W. W. acknowledges the financial support sponsored by Shanghai Sailing Program (20YF1411500) and the Fundamental Research Funds for the Central Universities. L. X. acknowledges the financial support sponsored by the NSFC (No. 21922506, 21871092, and 21672070), the Fundamental Research Funds for the Central Universities, the Opening Projects of Shanghai Key Laboratory of Green Chemistry and Chemical Processes, and State Key Laboratory of Fine Chemicals (KF 1801).
Notes and references
-
(a) P. J. Stang and B. Olenyuk, Self-assembly, symmetry, and molecular architecture:
coordination as the motif in the rational design of supramolecular metallacyclic polygons and polyhedra, Acc. Chem. Res., 1997, 30, 502 CrossRef CAS
;
(b) D. Fiedler, D. H. Leung, R. G. Bergman and K. N. Raymond, Selective molecular recognition, C−H bond activation, and catalysis in nanoscale reaction vessels, Acc. Chem. Res., 2005, 38, 349 CrossRef CAS
;
(c) M. Fujita, M. Tominaga, A. Hori and B. Therrien, Coordination assemblies from a Pd(II)-cornered square complex, Acc. Chem. Res., 2005, 38, 369 CrossRef CAS
;
(d) S. Liu, Y.-F. Han and G.-X. Jin, Formation of direct metal-metal bonds from 16-electron “pseudo-aromatic” half-sandwich complexes Cp”M[E2C2(B10H10)], Chem. Soc. Rev., 2007, 36, 1543 RSC
;
(e) R. Chakrabarty, P. S. Mukherjee and P. J. Stang, Supramolecular coordination: self-assembly of finite two- and three-dimensional ensembles, Chem. Rev., 2011, 111, 6810 CrossRef CAS
;
(f) M. J. Wiester, P. A. Ulmann and C. A. Mirkin, Enzyme mimics based upon supramolecular coordination chemistry, Angew. Chem., Int. Ed., 2011, 50, 114 CrossRef CAS
;
(g) T. R. Cook and P. J. Stang, Recent developments in the preparation and chemistry of metallacycles and metallacages via coordination, Chem. Rev., 2015, 115, 7001 CrossRef CAS
;
(h) L. Xu, Y.-X. Wang, L.-J. Chen and H.-B. Yang, Construction of multiferrocenyl metallacycles and metallacages via coordination-driven self-assembly: from structure to functions, Chem. Soc. Rev., 2015, 44, 2148 RSC
;
(i) W. Wang, Y.-X. Wang and H.-B. Yang, Supramolecular transformations within discrete coordination-driven supramolecular architectures, Chem. Soc. Rev., 2016, 45, 2656 RSC
;
(j) Y. Li, D. J. Young and X. J. Loh, Fluorescent gels: a review of synthesis, properties, applications and challenges, Mater. Chem. Front., 2019, 3, 1489 RSC
;
(k) L. Xu, D. Zhang, T. K. Ronson and J. R. Nitschke, Improved Acid Resistance of a Metal–Organic Cage Enables Cargo Release and Exchange between Hosts, Angew. Chem., Int. Ed., 2020, 59, 7435 CrossRef CAS
;
(l) Y.-X. Hu, X. Hao, L. Xu, X. Xie, B. Xiong, Z. Hu, H. Sun, G.-Q. Yin, X. Li, H. Peng and H.-B. Yang, Construction of Supramolecular Liquid-Crystalline Metallacycles for Holographic Storage of Colored Images, J. Am. Chem. Soc., 2020, 142, 6285 CrossRef CAS
;
(m) D. H. Kim, N. Singh, J. Oh, E.-H. Kim, J. Jung, H. Kim and K.-W. Chi, Coordination-driven self-assembly of a molecular knot comprising sixteen crossings, Angew. Chem., Int. Ed., 2018, 57, 5669 CrossRef CAS
;
(n) D. Zhang, T. K. Ronson, L. Xu and J. R. Nitschke, Transformation Network Culminating in a Heteroleptic Cd6L6L’2 Twisted Trigonal Prism, J. Am. Chem. Soc., 2020, 142, 9152 CrossRef CAS
;
(o) A. J. McConnell, C. J. E. Haynes, A. B. Grommet, C. M. Aitchison, J. Guilleme, S. Mikutis and J. R. Nitschke, Orthogonal stimuli trigger self-assembly and phase transfer of FeII4L4 cages and cargoes, J. Am. Chem. Soc., 2018, 140, 16952 CrossRef CAS
.
-
(a) M. Yoshizawa, J. K. Klosterman and M. Fujita, Functional molecular flasks: new properties and reactions within discrete, self-assembled hosts, Angew. Chem., Int. Ed., 2009, 48, 3418 CrossRef CAS
;
(b) J. Zhu, X. Liu, J. Huang and L. Xu, Our expedition in the construction of fluorescent supramolecular metallacycles, Chin. Chem. Lett., 2019, 30, 1767 CrossRef CAS
;
(c) K. Harris, D. Fujita and M. Fujita, Giant hollow MnL2n spherical complexes: structure, functionalisation and applications, Chem. Commun., 2013, 49, 6703 RSC
;
(d) N. C. Gianneschi, M. S. Masar and C. A. Mirkin, Development of a coordination chemistry-based approach for functional supramolecular structures, Acc. Chem. Res., 2005, 38, 825 CrossRef CAS
;
(e) D. L. Caulder and K. N. Raymond, Supermolecules by design, Acc. Chem. Res., 1999, 32, 975 CrossRef CAS
;
(f) C. Xu, L. Xu and X. Ma, A linear supramolecular polymer based on host-guest recognition and metal-ligand coordination, Chin. Chem. Lett., 2018, 29, 970 CrossRef CAS
;
(g) A. M. Castilla, W. J. Ramsay and J. R. Nitschke, Stereochemistry in subcomponent self-assembly, Acc. Chem. Res., 2014, 47, 2063 CrossRef CAS
;
(h) G.-Y. Wu, L.-J. Chen, L. Xu, X.-L. Zhao and H.-B. Yang, Construction of supramolecular hexagonal metallacycles via coordination-driven self-assembly: structure, properties and application, Coord. Chem. Rev., 2018, 369, 39 CrossRef CAS
;
(i) L.-J. Chen and H.-B. Yang, Construction of stimuli-responsive functional materials via hierarchical self-assembly involving coordination interactions, Acc. Chem. Res., 2018, 51, 2699 CrossRef CAS
;
(j) W. Xue, Z. Di, Y. Zhao, A. Zhang and L. Li, DNA-mediated coordinative assembly of upconversion hetero-nanostructures for targeted dual-modality imaging of cancer cells, Chin. Chem. Lett., 2019, 30, 899 CrossRef CAS
;
(k) S. Fu, Q. Luo, M. Zang, J. Tian, Z. Zhang, M. Zeng, Y. Ji, J. Xu and J. Liu, Light-triggered reversible disassembly of stimuli-responsive coordination metallosupramolecular Pd2L4 cages mediated by azobenzene-containing ligands, Mater. Chem. Front., 2019, 3, 1238 RSC
;
(l) L.-X. Cai, S.-C. Li, D.-N. Yan, L.-P. Zhou, F. Guo and Q.-F. Sun, Water-soluble redox-active cage hosting polyoxometalates for selective desulfurization catalysis, J. Am. Chem. Soc., 2018, 140, 4869 CrossRef CAS
;
(m) G. Li, Z. Zhou, C. Yuan, Z. Guo, Y. Liu, D. Zhao, K. Liu, J. Zhao, H. Tan and X. Yan, Trackable supramolecular fusion: cage to cage transformation of tetraphenylethylene-based metalloassemblies, Angew. Chem., Int. Ed., 2020, 59, 10013 CrossRef CAS
;
(n) J.-L. Zhu, L. Xu, Y.-Y. Ren, Y. Zhang, X. Liu, G.-Q. Yin, B. Sun, X. Cao, Z. Chen, X.-L. Zhao, H. Tan, J. Chen, X. Li and H.-B. Yang, Switchable Organoplatinum Metallacycles with High Quantum Yields and Tunable Fluorescence Wavelengths, Nat. Commun., 2019, 10, 4285 CrossRef
;
(o) L.-J. Chen, S. Chen, Y. Qin, L. Xu, G.-Q. Yin, J.-L. Zhu, F.-F. Zhu, W. Zheng, X. Li and H.-B. Yang, Construction of Porphyrin-Containing Metallacycle with Improved Stability and Activity within Mesoporous Carbon, J. Am. Chem. Soc., 2018, 140, 5049 CrossRef CAS
;
(p) X. Yan, S. Li, T. R. Cook, X. Ji, Y. Yao, J. B. Pollock, Y. Shi, G. Yu, J. Li, F. Huang and P. J. Stang, Hierarchical self-assembly: well-defined supramolecular nanostructures and metallohydrogels via amphiphilic discrete organoplatinum(II) metallacycles, J. Am. Chem. Soc., 2013, 135, 14036 CrossRef CAS
;
(q) H. Ube, K. Endo, H. Sato and M. Shionoya, Synthesis of hetero-multinuclear metal complexes by site-selective redox switching and transmetalation on a homo-multinuclear complex, J. Am. Chem. Soc., 2019, 141, 10384 CrossRef CAS
;
(r) N. Singh, J.-H. Jo, Y. H. Song, H. Kim, D. Kim, M. S. Lah and K.-W. Chi, Coordination-driven self-assembly of an iridium-cornered prismatic cage and encapsulation of three heteroguests in its large cavity, Chem. Commun., 2015, 51, 4492 RSC
;
(s) A. J. McConnell, Spin-state switching in Fe(II) helicates and cages, Supramol. Chem., 2018, 30, 858 CrossRef CAS
.
-
(a) L. Xu, Y.-X. Wang and H.-B. Yang, Recent advances in the construction of fluorescent metallocycles and metallocages via coordination-driven self-assembly, Dalton Trans., 2015, 44, 867 RSC
;
(b) M. Lal Saha, X. Yan and P. J. Stang, Photophysical properties of organoplatinum(II) compounds and derived self-assembled metallacycles and metallacages: fluorescence and its applications, Acc. Chem. Res., 2016, 49, 2527 CrossRef
;
(c) D. R. Martir and E. Zysman-Colman, Photoactive supramolecular cages incorporating Ru(II) and Ir(III) metal complexes, Chem. Commun., 2019, 55, 139 RSC
;
(d) L. Ma, T. Yang, Z. Zhang, S. Yin, Z. Song, W. Shi, D. Chu, Y. Zhang and M. Zhang, Cyanostilbene-based near-infrared emissive platinum(II) metallacycles for cancer theranostics, Chin. Chem. Lett., 2019, 30, 1942 CrossRef CAS
;
(e) W. Zheng, G. Yang, S.-T. Jiang, N. Shao, G.-Q. Yin, L. Xu, X. Li, G. Chen and H.-B. Yang, A tetraphenylethylene (TPE)-based supra-amphiphilic organoplatinum(II) metallacycle and its self-assembly behaviour, Mater. Chem. Front., 2017, 1, 1823 RSC
;
(f) C.-L. Liu, R.-L. Zhang, C.-S. Lin, L.-P. Zhou, L.-X. Cai, J.-T. Kong, S.-Q. Yang, K.-L. Han and Q.-F. Sun, Intraligand charge transfer sensitization on self-assembled europium tetrahedral cage leads to dual-selective luminescent sensing toward anion and cation, J. Am. Chem. Soc., 2017, 139, 12474 CrossRef CAS
;
(g) C.-B. Huang, L. Xu, J.-L. Zhu, Y.-X. Wang, B. Sun, X. Li and H.-B. Yang, Real-Time Monitoring the Dynamics of Coordination-Driven Self-Assembly by Fluorescence-Resonance Energy Transfer, J. Am. Chem. Soc., 2017, 139, 9459 CrossRef CAS
;
(h) X. Yan, P. Wei, Y. Liu, M. Wang, C. Chen, J. Zhao, G. Li, M. L. Saha, Z. Zhou, Z. An, X. Li and P. J. Stang,
Endo- and exo-functionalized tetraphenylethylene M12L24 nanospheres: fluorescence emission inside a donfined space, J. Am. Chem. Soc., 2019, 141, 9673 CrossRef CAS
;
(i) T. Nakamura, H. Ube, R. Miyake and M. Shionoya, A C60-templated tetrameric porphyrin barrel complex via zinc-mediated self-assembly utilizing labile capping ligands, J. Am. Chem. Soc., 2013, 135, 18790 CrossRef CAS
.
-
(a) S. Shanmugaraju and P. S. Mukherjee, π-Electron rich small molecule sensors for the recognition of nitroaromatics, Chem. Commun., 2015, 51, 16014 RSC
;
(b) T. M. Figueira-Duarte and K. Müllen, Pyrene-based materials for organic electronics, Chem. Rev., 2011, 111, 7260 CrossRef CAS
;
(c) M. A. Winnik and F. M. Winnik, Fluorescence studies of polymer association in water, Adv. Chem. Ser., 1993, 485 CrossRef CAS
;
(d) F. M. Winnik, Photophysics of preassociated pyrenes in aqueous polymer solutions and in other organized media, Chem. Rev., 1993, 93, 587 CrossRef CAS
;
(e) X.-D. Xu, J. Zhang, X. Yu, L.-J. Chen, D.-X. Wang, T. Yi, F. Li and H.-B. Yang, Design and preparation of platinum–acetylide organogelators containing ethynyl-pyrene moieties as the main skeleton, Chem. – Eur. J., 2012, 18, 16000 CrossRef CAS
;
(f) J. Zhu, P. Jia, N. Li, S. Tan, J. Huang and L. Xu, Small-molecule fluorescent probes for the detection of carbon dioxide, Chin. Chem. Lett., 2018, 29, 1445 CrossRef CAS
;
(g) S. Long, W. Chi, L. Miao, Q. Qiao, X. Liu and Z. Xu, Strong π–π stacking interactions led to the mis-assignment of dimer emissions to the monomers of 1-acetylpyrene, Chin. Chem. Lett., 2019, 30, 601 CrossRef CAS
;
(h) X. Song, J. Zhao, W. Zhang and L. Chen, Novel n-channel organic semiconductor based on pyrene-phenazine fused monoimide and bisimides, Chin. Chem. Lett., 2018, 29, 331 CrossRef CAS
;
(i) N.-W. Wu, J. Zhang, C.-H. Wang, L. Xu and H.-B. Yang, Synthesis and characterization of multipyrene-modified platinum acetylide oligomers, Monatsh. Chem., 2013, 144, 553 CrossRef CAS
;
(j) Z. Li, Y. Li, D. Wang, Q. Cui, Z. Li, L. Wang and H. Yang, Unique fluorescence properties of a self-assembling bis-pyrene molecule, Chin. Chem. Lett., 2018, 29, 1645 CrossRef CAS
;
(k) Z.-Y. Li, L. Xu, C.-H. Wang, X.-L. Zhao and H.-B. Yang, Novel platinum–acetylide metallocycles constructed via a stepwise fragment coupling approach and their aggregation behaviour, Chem. Commun., 2013, 49, 6194 RSC
;
(l) N.-W. Wu, J. Zhang, X.-D. Xu and H.-B. Yang, Design and preparation of ethynyl-pyrene modified platinum–acetylide gelators and their application in dispersion of graphene, Chem. Commun., 2014, 50, 10269 RSC
;
(m) S. Long, Q. Qiao, L. Miao and Z. Xu, A self-assembly/disassembly two-photo ratiometric fluorogenic probe for bacteria imaging, Chin. Chem. Lett., 2019, 30, 573 CrossRef CAS
.
-
(a)
J. B. Birks, Photophysics of aromatic molecules, Wiley-Interscience, London, 1970 Search PubMed
;
(b) K. Kalyanasundaram and J. K. Thomas, Environmental effects on vibronic band intensities in pyrene monomer fluorescence and their application in studies of micellar systems, J. Am. Chem. Soc., 1977, 99, 2039 CrossRef CAS
;
(c) S. Cicchi, P. Fabbrizzi, G. Ghini, A. Brandi, P. Foggi, A. Marcelli, R. Righini and C. Botta, Pyrene-excimers-based antenna systems, Chem. – Eur. J., 2009, 15, 754 CrossRef CAS
;
(d) Y. Ohishi and M. Inouye, Circularly polarized luminescence from pyrene excimers, Tetrahedron Lett., 2019, 60, 151232 CrossRef CAS
.
-
(a) F. Würthner and A. Sautter, Energy transfer in multichromophoric self-assembled molecular squares, Org. Biomol. Chem., 2003, 1, 240 RSC
;
(b) A. Sautter, B. K. Kaletaş, D. G. Schmid, R. Dobrawa, M. Zimine, G. Jung, I. H. M. van Stokkum, L. De Cola, R. M. Williams and F. Würthner, Ultrafast energy-electron transfer cascade in a multichromophoric light-harvesting molecular square, J. Am. Chem. Soc., 2005, 127, 6719 CrossRef CAS
;
(c) C.-C. You, C. Hippius, M. Grüne and F. Würthner, Light-harvesting metallosupramolecular squares composed of perylene bisimide walls and fluorescent antenna dyes, Chem. – Eur. J., 2006, 12, 7510 CrossRef CAS
.
- N.-W. Wu, J. Zhang, D. Ciren, Q. Han, L.-J. Chen, L. Xu and H.-B. Yang, Construction of supramolecular pyrene-modified metallacycles via coordination-driven self-assembly and their spectroscopic behavior, Organometallics, 2013, 32, 2536 CrossRef CAS
.
- M. He, Q. Han, J. He, Q. Li, Z. Abliz, H. Tan, L. Xu and H. Yang, Self-assembly of pyrene-modified rhomboidal metallodendrimers via directional metal-ligand bonding approach, Chin. J. Chem., 2013, 31, 663 CrossRef CAS
.
- S. Kobayashi, M. Lal Saha and P. J. Stang, Pyrene functionalized metallosupramolecular rhomboids and their photophysical properties, J. Organomet. Chem., 2017, 847, 294 CrossRef CAS
.
- X. Chang, Z. Zhou, C. Shang, G. Wang, Z. Wang, Y. Qi, Z.-Y. Li, H. Wang, L. Cao, X. Li, Y. Fang and P. J. Stang, Coordination-driven self-assembled metallacycles
incorporating pyrene: fluorescence mutability, tunability, and aromatic amine sensing, J. Am. Chem. Soc., 2019, 141, 1757 CrossRef CAS
.
- B. Shi, Y. Liu, H. Zhu, R. T. Vanderlinden, L. Shangguan, R. Ni, K. Acharyya, J.-H. Tang, Z. Zhou, X. Li, F. Huang and P. J. Stang, Spontaneous formation of a cross-linked supramolecular polymer both in the solid state and in solution, driven by platinum(II) metallacycle-based host–guest interactions, J. Am. Chem. Soc., 2019, 141, 6494 CrossRef CAS
.
-
(a) P. Wei, X. Yan and F. Huang, Supramolecular polymers constructed by orthogonal self-assembly based on host–guest and metal–ligand interactions, Chem. Soc. Rev., 2015, 44, 815 RSC
;
(b) Y. Sun, C. Chen, J. Liu and P. J. Stang, Recent developments in the construction and applications of platinum-based metallacycles and metallacages via coordination, Chem. Soc. Rev., 2020, 49, 3889 RSC
;
(c) G.-Z. Zhao, L.-J. Chen, W. Wang, J. Zhang, G. Yang, D.-X. Wang, Y. Yu and H.-B. Yang, Stimuli-responsive supramolecular gels through hierarchical self-assembly of discrete rhomboidal metallacycles, Chem. – Eur. J., 2013, 19, 10094 CrossRef CAS
;
(d) Z.-Y. Li, Y. Zhang, C.-W. Zhang, L.-J. Chen, C. Wang, H. Tan, Y. Yu, X. Li and H.-B. Yang, Cross-linked supramolecular polymer gels constructed from discrete multi-pillar[5]arene metallacycles and their multiple stimuli-responsive behavior, J. Am. Chem. Soc., 2014, 136, 8577 CrossRef CAS
;
(e) W. Zheng, L.-J. Chen, G. Yang, B. Sun, X. Wang, B. Jiang, G.-Q. Yin, L. Zhang, X. Li, M. Liu, G. Chen and H.-B. Yang, Construction of smart supramolecular polymeric hydrogels cross-linked by discrete organoplatinum(II) metallacycles via post-assembly polymerization, J. Am. Chem. Soc., 2016, 138, 4927 CrossRef CAS
.
-
(a) M. Poyatos, J. A. Mata and E. Peris, Complexes with poly(N-heterocyclic carbene) ligands: structural features and catalytic applications, Chem. Rev., 2009, 109, 3677 CrossRef CAS
;
(b) E. Peris, Smart N-heterocyclic carbene ligands in catalysis, Chem. Rev., 2018, 118, 9988 CrossRef CAS
.
- V. Martínez-Agramunt, S. Ruiz-Botella and E. Peris, Nickel-cornered molecular rectangles as polycyclic aromatic hydrocarbon receptors, Chem. – Eur. J., 2017, 23, 6675 CrossRef
.
- V. Martínez-Agramunt, T. Eder, H. Darmandeh, G. Guisado-Barrios and E. Peris, A size-flexible organometallic box for the encapsulation of fullerenes, Angew. Chem., Int. Ed., 2019, 58, 5682 CrossRef
.
- V. Martínez-Agramunt and E. Peris, Photocatalytic properties of a palladium metallosquare with encapsulated fullerenes via singlet oxygen generation, Inorg. Chem., 2019, 58, 11836 CrossRef
.
- V. Martínez-Agramunt and E. Peris, A palladium-hinged organometallic square with a perfect-sized cavity for the encapsulation of three heteroguests, Chem. Commun., 2019, 55, 14972 RSC
.
- S. Ibáñez and E. Peris, Dimensional matching versus induced-fit distortions: binding affinities of planar and curved polyaromatic hydrocarbons with a tetragold metallorectangle, Angew. Chem., Int. Ed., 2020, 59, 6860 CrossRef
.
-
(a) R. Chakrabarty and P. J. Stang, Post-assembly functionalization of organoplatinum(II) metallacycles via copper-free click chemistry, J. Am. Chem. Soc., 2012, 134, 14738 CrossRef CAS
;
(b) B. Eggers and U. Ziener, Optically functionalized grid-type complexes by a post-assembly strategy, Chem. – Eur. J., 2018, 24, 14968 CrossRef CAS
.
- Y. Wang, Y. Gu, E. G. Keeler, J. V. Park, R. G. Griffin and J. A. Johnson, Star polyMOCs with diverse structures, dynamics, and functions by three-component assembly, Angew. Chem., Int. Ed., 2017, 56, 188 CrossRef CAS
.
- P. P. Neelakandan, A. Jiménez and J. R. Nitschke, Fluorophore incorporation allows nanomolar guest sensing and white-light emission in M4L6 cage complexes, Chem. Sci., 2014, 5, 908 RSC
.
- S. Shanmugaraju, H. Jadhav, Y. P. Patil and P. S. Mukherjee, Self-assembly of an octanuclear platinum(II) tetragonal prism from a new Pt4II organometallic star-shaped acceptor and its nitroaromatic sensing study, Inorg. Chem., 2012, 51, 13072 CrossRef CAS
.
- B.-B. Guo, W.-X. Gao, Y.-J. Lin and G.-X. Jin, Construction of half-sandwich multinuclear complexes including tunnel architectures via C–H-activation-directed assembly, Dalton Trans., 2018, 47, 7701 RSC
.
- V. Martínez-Agramunt, D. G. Gusev and E. Peris, A shape-adaptable organometallic supramolecular coordination cage for the encapsulation of fullerenes, Chem. – Eur. J., 2018, 24, 14802 CrossRef
.
- T. K. Ronson, A. B. League, L. Gagliardi, C. J. Cramer and J. R. Nitschke, Pyrene-edged Fe4IIL6 cages adaptively reconfigure during guest binding, J. Am. Chem. Soc., 2014, 136, 15615 CrossRef CAS
.
- T. K. Ronson, W. Meng and J. R. Nitschke, Design principles for the optimization of guest binding in aromatic-paneled Fe4IIL6 cages, J. Am. Chem. Soc., 2017, 139, 9698 CrossRef CAS
.
- T. K. Ronson, D. A. Roberts, S. P. Black and J. R. Nitschke, Stacking interactions drive selective self-assembly and self-sorting of pyrene-based M4IIL6 architectures, J. Am. Chem. Soc., 2015, 137, 14502 CrossRef CAS
.
|
This journal is © the Partner Organisations 2020 |
Click here to see how this site uses Cookies. View our privacy policy here.