DOI:
10.1039/D0QM00247J
(Research Article)
Mater. Chem. Front., 2020,
4, 2047-2053
AIE-active multicolor tunable luminogens: simultaneous mechanochromism and acidochromism with high contrast beyond 100 nm†
Received
17th April 2020
, Accepted 25th May 2020
First published on 26th May 2020
Abstract
The development of intelligent luminescent materials, particularly those exhibiting high-contrast (Δλem,max > 100 nm) and multicolor mechanochromic luminescent (MCL) characteristics, is highly desirable but a formidable challenge. In this work, we designed three luminogens with aggregation-induced emission (AIE) features, namely 7PTP-BPXZ, 5PTP-BPXZ and 5,7PTP-BPXZ. The synergistic effect between the folded conformation of the benzo[a]phenoxazine fragment and various intermolecular non-covalent interactions is beneficial for multicolor tunable emission upon external stimuli. Impressively, 7PTP-BPXZ and 5PTP-BPXZ displayed reversible tricolor MCL behavior with high contrast (Δλem,max, 114 nm and 137 nm, respectively). Furthermore, 7PTP-BPXZ possessed acidochromic function with Δλem,max of 132 nm. This is the first report of single-component small organic molecules featuring both multicolor and high-contrast beyond 100 nm mechanochromism and acidochromism.
Introduction
Organic solid-state intelligent luminescent materials (or luminogens) are a category of versatile materials with tunable appearance color or emission in response to external stimuli. During the past decade, smart luminogens have drawn intensive research enthusiasm in the fields of mechanosensors, organic light-emitting diodes, organic lasers, security systems, optical storage, etc.1 According to stimulating types, the color switching phenomena can be further divided into photochromism, electrochromism, thermochromism, mechanochromism, acidochromism, and so forth.2 Specifically, great progress has been made in mechanochromic luminescent (MCL) materials with emission features governed by physical channels, such as anisotropic grinding, shearing or rubbing. These approaches only change molecular stacking patterns or conformations, but do not alter the native chemical structures. Nowadays, plenty of MCL materials involving metal complexes, polymers and pure organic (metal-free) molecules have been reported to exhibit bicolor switching or low-contrast luminescence, but they are still unsatisfactory for practical applications in anti-counterfeiting complexity and density of optical storage.3 Meanwhile, single-component metal-free molecules simultaneously featuring multicolor (more than two colors) and high-contrast mechanochromism (especially with Δλem,max > 100 nm) are underexplored owing to the lack of an effective design strategy.
Aggregation-induced emission (AIE) materials, referring to a type of luminogen which emits faintly in pure solution but shows remarkably enhanced emission in aggregated states, enjoy intrinsic advantages such as multicolor and high-contrast mechanochromic candidates.4 These luminogens usually adopt twisted configurations which are facilely manipulated by moderate anisotropic grinding.5 Besides, heterocycles, namely cyclic hydrocarbons embedded with heteroatoms (N, O, S, etc.), are often established as building blocks in intelligent luminescent materials. The heteroatoms enable variable conformations of molecules and easily produce intermolecular and intramolecular hydrogen bonds, such as C–H⋯N and C–H⋯O bonds, which can result in the modulation of the solid-state emission under the external stimuli. Moreover, luminogens constructed by the combination of large conjugation cores and peripheral phenyl rings may exhibit high-contrast MCL phenomena. Recently, Tang and Li designed an AIE tetraphenylethylene derivative with tricolor mechanochromic properties. It showed fluorescence responses to acid/base vapor, and the emission could be altered among blue (462 nm), bright cyan (482 nm) and yellow (496 nm) by grinding, heating or solvent fuming.6 Takeda and Minakata synthesized phenothiazine-dibenzophenazine-phenothiazine molecules with tricolor MCL features.7 However, the development of simultaneous multicolor and high-contrast mechanochromic small molecules remains a challenging task.
To achieve such a perfect MCL system, the benzo[a]phenoxazine (BPXZ) unit was chosen as the donor because phenoxazine could adopt the folded conformation and the introduction of the peripheral phenyl ring could further rigidify the molecular architecture. Furthermore, phenyl substituted triazolopyrimidine (PTP) was selected as the acceptor core in virtue of multiple nitrogen atoms which could benefit the formation of weak intermolecular interactions and impede the intramolecular motions in the solid state. Subsequently, three heterocyclic molecules, 12-(4-(7-phenyl-[1,2,4]triazolo[1,5-a]pyrimidin-5-yl)phenyl)-12H-benzo[a]phenoxazine (7PTP-BPXZ), 12-(4-(5-phenyl-[1,2,4]triazolo[1,5-a]pyrimidin-7-yl)phenyl)-12H-benzo[a]phenoxazine (5PTP-BPXZ) and 12,12′-([1,2,4]triazolo[1,5-a]pyrimidine-5,7-diylbis(4,1-phenylene)bis-(12H-benzo[a]phenoxazine)) (5,7PTP-BPXZ) were designed and prepared in this work (Scheme 1). Three luminogens exhibited obvious AIE behaviors which were attributed to the restriction of intramolecular motion in solid states by deeply analyzing their crystal packing arrangements. Significantly, 7PTP-BPXZ and 5PTP-BPXZ showed high-contrast and tricolor MCL features. More impressively, 7PTP-BPXZ displayed a high-contrast (Δλem,max = 132 nm) acidochromic characteristic as well. To the best of our knowledge, this is the first example of single-component small organic molecules with mechanochromism and acidochromism manifesting both multi colors and high contrast (Δλem,max > 100 nm).
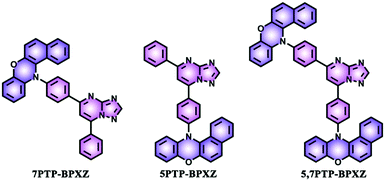 |
| Scheme 1 Chemical structures of the luminogens. | |
Results and discussion
Synthesis and characterization
The synthetic route of the compounds is outlined in Scheme S1 (ESI†). The donor segment (BPXZ) was synthesized in a three-step procedure involving aromatic nucleophilic substitution, nitro-reduction by Fe powder and palladium-catalyzed Buchwald–Hartwig coupling reaction. The PTP acceptors were synthesized according to the previous protocols.8 Finally, the target compounds were assembled via an intermolecular Buchwald–Hartwig amination reaction between BPXZ and PTP acceptors. The final products were carefully characterized by 1H NMR, 13C NMR, high-resolution mass spectrometry and single-crystal X-ray diffraction.
Theoretical simulation and electrochemical properties
Density functional theory (DFT) calculations were implemented to predict the frontier molecular orbitals (FMOs) and electronic states of the molecules. As shown in Fig. 1, all BPXZ subunits were largely folded along their central N⋯O axis and adopted quasi-axial (QA) conformations with small donor–acceptor torsion angles (θa) of 9–11°. The QA geometries facilitated the conjugation between nitrogen lone pair electrons with the adjacent acceptor moiety, resulting in large overlap of the highest occupied molecular orbitals (HOMOs) and lowest unoccupied molecular orbitals (LUMOs) on the PTP units and more localized electronic transitions upon excitation.
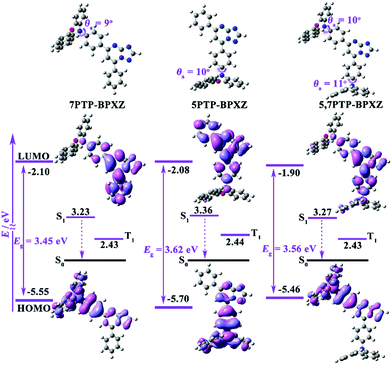 |
| Fig. 1 The optimized configurations and HOMO/LUMO distributions of the luminogens evaluated using the B3LYP-D3(BJ)/def2-SVP level, and the energy level diagram for S1 and T1 states calculated with TD-DFT:PBE0/def2-SVP. | |
The luminogens displayed a reversible one-electron oxidation process in the cyclic voltammetry (CV) curves (Fig. S1, ESI†). According to the onset voltages of the oxidation curves, the HOMO levels of 7PTP-BPXZ, 5PTP-BPXZ and 5,7PTP-BPXZ were determined to be −5.06 eV, −5.13 eV and −5.10 eV, respectively. The LUMO levels deduced from the HOMOs and corresponding energy gaps (Egs, obtained from their absorption onset) were individually calculated to be −2.13 eV, −2.19 eV and −2.17 eV. These results matched well with the theoretical analysis.
Basic photophysical properties
The UV-vis absorption and fluorescence spectra of 7PTP-BPXZ, 5PTP-BPXZ and 5,7PTP-BPXZ were measured in solvents with different polarity (i.e. n-hexane, toluene, tetrahydrofuran (THF), chloroform), and the pertinent photophysical data are summarized in Table S1 (ESI†). As shown in Fig. S2 (ESI†), three luminogens exhibited similar strong absorption peaks at around 350 nm assigned to the local π–π* transition. However, no obvious absorption from the charge transfer state was observed, indicating favorable QA conformation in the solution state. The fluorescence spectra of all luminogens displayed unstructured emissions. The emission bands underwent a bathochromic shift with decreased intensity when the solvent polarity was increased, mainly ascribed to the intense positive solvation effect and the increased detrimental non-radiative decay in polar media.
To investigate their AIE features, THF/water mixtures with different water fractions (fws) were utilized as a solvent system. As depicted in Fig. 2, three luminogens exhibited typical AIE properties. Taking 5PTP-BPXZ as an example, the emission was extremely weak with fw below 80%. Afterwards, the emission intensity was boosted markedly along with further raising fw owing to the restriction of intramolecular rotations (RIRs) by molecular aggregation, and reached the maximum at fw of 99%, which was 348-fold higher than that in pure THF solution. Additionally, the aggregates excluded the numerous solvent molecules with high polarity, and thus the luminogen felt less relaxed and the intramolecular charge transfer process became weakened. Consequently, the emission was strengthened and hypochromically shifted in their aggregation states. In the case of 7PTP-BPXZ and 5,7PTP-BPXZ, their maximum emission intensity in the aggregated state was 160-fold and 168-fold higher than that in THF, respectively. Unlike 5PTP-BPXZ, their emission was slightly decreased when fw was 99%. There was a possible reason that the molecules might cluster together to form ordered aggregates when fw was 80–95%, which contributed to the emission enhancement.9,10a Nevertheless, the molecules might commence agglomerating to form amorphous aggregates while fw was 99%, which was detrimental to the emission.
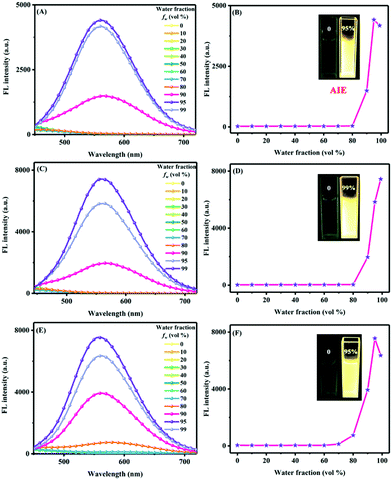 |
| Fig. 2 The fluorescence spectra of luminogens and plot of fluorescence intensity in aqueous mixtures. A/B: 7PTP-BPXZ; C/D: 5PTP-BPXZ; E/F: 5,7PTP-BPXZ. Inset: Photographs under UV illumination. | |
In order to gain further insights into the geometrical conformations and packing modes, crystals of 7PTP-BPXZ, 5PTP-BPXZ and 5,7PTP-BPXZ were cultivated by solvent diffusion of hexane into the chloroform solution at room temperature. As shown in Fig. S3 (ESI†), three crystals adopted QA conformations with comparable bending angles (θb). The dihedral angles (θa) between donors and acceptors were small, in good accordance with the theoretical predictions. It should be noted that the folded conformation of benzo[a]phenoxazine substitution is facilely manipulated by external stimuli, which may contribute to their multicolor tunable emission. Crystal 7PTP-BPXZ was a monoclinic system with the space group P21/n. It could be seen that the dimer with two kinds of intermolecular interactions (Fig. 3A), such as C15–H15⋯N4 (d = 2.90 Å) and C21–H21⋯π (namely ring1, C23–C24–C25–N3–C26–N2, d = 3.42 Å), was involved in the unit cell of 7PTP-BPXZ. Then, these dimers formed a one-dimensional (1D) chain through C30–H30⋯π (ring2, C11–C12–C13–C14–C15–C16, d = 3.22 Å) and C30–H30⋯π (ring3, C28–C29–C30–C31–C32–C33, d = 3.49 Å) interactions (Fig. 3B). Furthermore, 2D layers were constructed through C3–H3⋯π (ring4, C1–C2–C3–C4–C5–C6, d = 3.19 Å), C3–H3⋯π (ring5, C5–C6–C7–C8–C9–C10, d = 2.88 Å), C4–H4⋯π (ring4, d = 3.14 Å) and C10–H10⋯π (ring2, d = 2.83 Å) (Fig. 3C and D), which suppressed the intramolecular motion and blocked the nonradiative decay channel to emit intense light in the aggregated state. Besides, these weak intermolecular non-covalent interactions are easily varied upon external stimuli, which are also beneficial for the switchable emission.
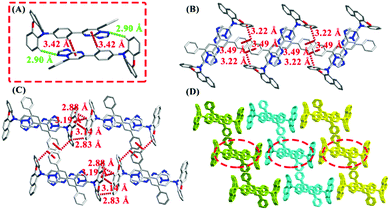 |
| Fig. 3 The packing mode of the 7PTP-BPXZ crystal and intermolecular interactions in the dimers (C–H⋯π interactions: red, C–H⋯N hydrogen bonds: green). Hydrogen atoms except H3, H4, H10, H15, H21 and H30 were omitted for clarity. | |
Crystal 5PTP-BPXZ was a monoclinic system with the space group P21. As depicted in Fig. 4, CHCl3 molecules were entrapped in the crystalline arrangement and held the dimers together. Meanwhile, three kinds of strong C–H⋯π interactions and a weak π⋯π interaction existed in the dimers (Fig. 4A). Then, they were linked by C13–H13⋯N4 (d = 2.88 Å), C14–H14⋯Cl3 (d = 2.96 Å), C32–H32⋯N2 (d = 2.65 Å), C33–H33⋯π (ring6, C11–C12-C13–C14–C15–C16, d = 3.14 Å) and C34–H34⋯π (ring6, d = 3.59 Å), and facilitated the self-assembling into a 1D chain (Fig. 4B and Table S2, ESI†). Furthermore, 2D layers were arranged by four types of C–H⋯π interactions (Fig. 4C and D), such as C5–H5⋯π (ring4, C1–C2–C3–C4–C5–C6, d = 3.24 Å), C6–H6⋯π (ring4, d = 3.30 Å), C6–H6⋯π (ring5, C3–C4–C7–C8–C9–C10, d = 3.01 Å) and C7–H7⋯π (ring6, d = 2.99 Å). The multiple weak interactions in the crystal lattice restricted intramolecular motions and allowed the excited-state energy to dissipate via radiative relaxation, thus resulting in bright fluorescence.
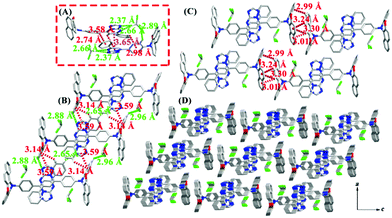 |
| Fig. 4 The packing mode of 5PTP-BPXZ crystal and intermolecular interactions in dimers (C–H⋯π interactions: red, C–H⋯N hydrogen bonds: green, π⋯π interactions: purple). Hydrogen atoms except H1S, H5, H6, H7, H13, H14, H19, H22, H24, H30, H32, H33 and H34 were omitted for clarity. | |
Crystal 5,7PTP-BPXZ was a monoclinic system with the space group P21/n. As illustrated in Fig. 5A, the molecules were organized in dimers by the strong C33–H33⋯O1 (d = 2.67 Å) hydrogen bond, C27–H27⋯π (ring1, C11–C12–C13–C14–C15–C16, d = 2.97 Å), C32–H32⋯π (ring2, C1–C2–C7–C8–C9–C10, d = 3.42 Å), C33–H33⋯π (ring2, d = 3.59 Å) and π⋯π (ring3, C17–C18–C19–C20–C21–C22, and ring4, C23–C24–C25–N4–C26–N2, d = 3.61 Å) interactions. Moreover, a 3D staggered pattern was constructed via the adjacent dimers with abundant C3–H3⋯O2 (d = 2.61 Å), C49–H49⋯π (ring4, d = 3.40 Å) and π⋯π interactions (ring4 and C43–C44–C45–C46–C47–C49, d = 3.59 Å). These weak interactions could rigidify the molecular structure and largely alleviate the nonradiative decay pathway, which was conducive to enhancing the emission efficiency in condensed states.
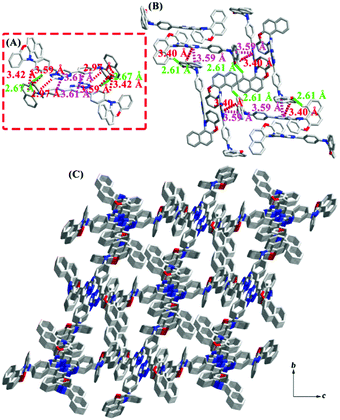 |
| Fig. 5 The packing mode of 5,7PTP-BPXZ crystal and intermolecular interactions in dimers (C–H⋯π interactions: red, C–H⋯N hydrogen bonds: green, π⋯π interactions: purple). Hydrogen atoms except H3, H27, H32, H33 and H49 were omitted for clarity. | |
High contrast mechanochromism and acidochromism
As defined in Fig. 6, 7PTP-BPXZ and 5PTP-BPXZ showed distinct tricolor mechanochromic behavior in response to external stimuli. The pristine powder of 7PTP-BPXZ gave sky-blue emission under a 365 nm UV lamp and the maximum emission wavelength (λem) was 472 nm (Fig. S4, ESI†). After grinding with a pestle, the powder exhibited deep yellow luminescence and underwent a notable bathochromic shift (λem = 586 nm, Δλem = 114 nm), which revealed its high contrast mechanochromic characteristic. More impressively, the ground powder became green-emitting (λem = 492 nm) when fuming with dichloromethane (CH2Cl2) vapor (Fig. 6A). The green state could revert back to the ground state by grinding. Besides, the ground or fumed samples of 7PTP-BPXZ both could return to the pristine state by recrystallizing from an ethyl acetate (EA) and petroleum ether (PE) mixture. Similarly, 5PTP-BPXZ showed blue, orange and bluish green switching mechanochromic behavior (Fig. 6B). The maximum emission wavelengths of the pristine, ground and fumed samples were 462 nm, 599 nm, and 488 nm respectively (Fig. S4, ESI†), displaying better mechanochromic contrast (Δλem = 137 nm) than 7PTP-BPXZ. The blue emission of pristine 5PTP-BPXZ powder turned to orange by grinding. When fuming the orange sample with CH2Cl2 vapor, it became bluish green emission. Conversely, the bluish green state could change to the orange state by grinding. Moreover, the ground and fumed samples of 5PTP-BPXZ could also recover to the pristine state by recrystallizing from an EA and PE mixture. 5,7PTP-BPXZ showed reversible bicolor MCL properties. As shown in Fig. 6C, its pristine powder exhibited green emission (λem = 536 nm). However, the sample showed pronounced red-shifted fluorescence (λem = 602 nm) after grinding and could also revert back to the pristine green state by recrystallizing or heating.
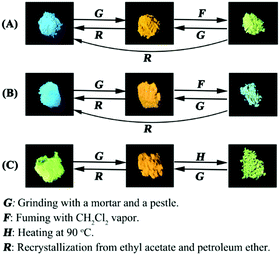 |
| Fig. 6 Schematic diagrams of the mechanochromic behaviors of 7PTP-BPXZ (A), 5PTP-BPXZ (B) and 5,7PTP-BPXZ (C). Fluorescence images were taken under a 365 nm UV lamp. | |
Besides, all luminogens displayed reversible acidochromic properties in their solid states (Fig. 7). Taking 7PTP-BPXZ as an example, upon exposure to the vapor of trifluoroacetic acid (TFA) for ten seconds, the maximum emission wavelength of the pristine sample was changed from 472 nm to 604 nm with a significant red-shift of 132 nm (Fig. S4, ESI†). The color transition after fuming with TFA could be easily distinguished by the naked eye in daylight (Fig. 7B), representing high-contrast acidochromism possibly originated from the amine protonation of the PTP moiety.
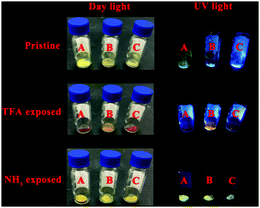 |
| Fig. 7 On–off fluorescence switching of the luminogens by TFA and NH3 exposure under daylight and 365 nm light. A: 5,7PTP-BPXZ. B: 7PTP-BPXZ. C: 5PTP-BPXZ. | |
Powder X-ray diffraction (PXRD) and differential scanning calorimetry (DSC) measurements were performed to acquire deeper insight into their underlying mechanisms of mechanochromism and acidochromism. For instance, the pristine sample of 7PTP-BPXZ exhibited sharp and intense diffraction peaks at around 9°, demonstrating its good crystallinity (Fig. 8). However, the peaks disappeared after grinding, indicating that the lattice of 7PTP-BPXZ was destroyed by mechanical forces.10 When fuming by CH2Cl2 vapor, the original sharp signals were restored, manifesting the recovery of the lattice. Furthermore, the diffraction curves of TFA and NH3 vapor treated samples were not obviously altered compared with those of the pristine samples, indicative of their consistent crystalline patterns. DSC curves illustrated that the melting point of 7PTP-BPXZ was 226 °C (Fig. S5, ESI†).
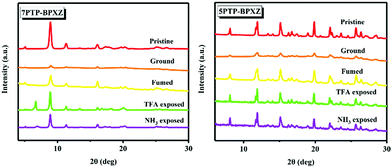 |
| Fig. 8 PXRD curves of luminogens in different states. | |
Additionally, the ground sample showed an exothermic recrystallization point at 155 °C. In contrast, the pristine samples showed no such exothermic signal. This revealed that the thermodynamically-stable states were converted into the metastable states by grinding and thus the pristine crystal phase was destroyed.
Conclusions
In summary, we have developed the first example of single-component metal-free small molecules with both multicolor and high contrast (Δλem,max > 100 nm) mechanochromism and acidochromism. The folded conformation of benzo[a]phenoxazine substitution and the introduction of multiple nitrogen atoms in the triazolopyrimidine moiety have a synergistic effect on these excellent MCL features. As demonstrated by their crystal packing arrangements, the AIE behaviors of the three luminogens can be ascribed to the multiple intermolecular non-covalent interactions which restrict the molecular motion. We believe that the rational molecular design strategy towards multicolor controllable mechanochromic and acidochromic luminogens with distinct emission color contrast will provide a valuable insight into the development of organic intelligent luminescent materials.
Experimental
Materials and apparatus
Toluene was dried by sodium-potassium alloy. The other reagents and solvents were used as received from commercial sources without further purification. 1H NMR and 13C NMR spectra were recorded by Bruker Advanced II (400 MHz) spectrometers and MERCURYVX300 using CDCl3 or CD3OD as solvents and tetramethylsilane as the internal standards. High resolution mass spectra were measured on a LCQ-Orbitrap Elite (Thermo-Fisher Scientific, Waltham, MA, USA) mass spectrometer. UV-vis absorption spectra were collected on a Shimadzu UV-2700 spectrophotometer. Photoluminescence (PL) spectra were recorded on a Hitachi F-4600 fluorescence spectrophotometer. Differential scanning calorimetry (DSC) was performed on a NETZSCH DSC 200 PC unit at a heating rate of 10 °C min−1 under argon. Powder X-ray diffraction (PXRD) patterns were recorded on a Rigaku (Ultima IV) diffractometer. Single crystal X-ray diffraction (SXRD) data were measured on a Bruker Smart APEX CCD-based diffractometer with graphite-monochromatic Mo Kα radiation (λ = 0.71073 Å). Cyclic voltammetry (CV) was carried out in nitrogen-purged THF (oxidation scan) at room temperature with a CHI voltammetric analyser. Tetrabutylammonium hexafluorophosphate (TBAPF6) (0.1 M) was used as the supporting electrolyte. The conventional three-electrode configuration consists of a platinum working electrode, a platinum wire auxiliary electrode, and an Ag wire pseudo-reference electrode with ferrocenium–ferrocene (Fc+/Fc) as the internal standard. Cyclic voltammograms were obtained at a scan rate of 100 mV s−1. Formal potentials are calculated as the average of cyclic voltammetric anodic and cathodic peaks. CCDC numbers, 1994907 for 7PTP-BPXZ, 1994908 for 5PTP-BPXZ, 1994909 for 5,7PTP-BPXZ.
Synthesis
Synthesis of 12H-benzo[a]phenoxazine (BPXZ). A mixture of 0.31 g (1.0 mmol) 2-((1-bromonaphthalen-2-yl)oxy)aniline, 0.12 g (1.2 mmol) sodium tert-butoxide, 0.02 g (0.1 mmol) palladium(II) acetate and 0.06 g (0.2 mmol) tri-tert-butylphosphine tetrafluoroborate was dissolved in dry toluene (20 mL) under an argon atmosphere. After stirring at 110 °C overnight, the suspension was mixed thoroughly with dichloromethane and washed with water three times. After drying with anhydrous Na2SO4, the organic phase was concentrated and the crude product was purified by column chromatography on silica gel with petroleum ether/dichloromethane as the eluent. The product was obtained as a greenish yellow solid. Yield: 68%. 1H NMR (400 MHz, CD3OD) δ (ppm): 7.83–7.81 (m, 1H), 7.67–7.65 (m, 1H), 7.40–7.31 (m, 1H), 7.30–7.29 (m, 1H), 7.18 (d, J = 8.7 Hz, 1H), 6.86 (d, J = 8.7 Hz, 1H), 6.75–6.71 (m, 1H), 6.68–6.64 (m, 1H), 6.63–6.56 (m, 2H).
Synthesis of 12-(4-(7-phenyl-[1,2,4]triazolo[1,5-a]pyrimidin-5-yl)phenyl)-12H-benzo[a]phenoxazine (7PTP-BPXZ).
A mixture of 0.11 g (0.30 mmol) 5-(4-bromophenyl)-7-phenyl-[1,2,4]triazolo[1,5-a]pyrimidine, 0.08 g (0.33 mmol) BPXZ, 0.04 g (0.40 mmol) sodium tert-butoxide, 0.01 g (0.03 mmol) palladium(II) acetate and 0.02 g (0.06 mmol) tri-tert-butylphosphine tetrafluoroborate was dissolved in dry toluene (5 mL) under an argon atmosphere. After stirring at 110 °C overnight, the suspension was mixed thoroughly with dichloromethane and washed with water four times. After drying with anhydrous Na2SO4, the organic phase was concentrated and the crude product was purified by column chromatography on silica gel with petroleum ether/ethyl acetate as the eluent. The product was obtained as a yellow solid, which was further crystallized from ethyl acetate. Yield: 81%. 1H NMR (400 MHz, CDCl3) δ (ppm): 8.47 (s, 1H), 8.08–8.03 (m, 4H), 7.92–7.87 (m, 2H), 7.78 (d, J = 8.8 Hz, 1H), 7.70–7.63 (m, 1H), 7.61–7.57 (m, 3H), 7.51–7.39 (m, 4H), 7.26–7.19 (m, 3H), 7.15 (d, J = 9.0 Hz, 2H). 13C NMR (100 MHz, CDCl3) δ (ppm): 161.0, 156.0, 153.8, 151.6, 151.5, 147.7, 133.8, 131.6, 131.1, 129.5, 129.4, 129.2, 128.9, 128.7, 128.4, 127.4, 127.0, 126.6, 126.2, 125.0, 123.8, 122.9, 118.0, 117.7, 117.5, 106.0. HRMS (ESI): m/z [M + H]+ calcd for C33H22N5O+: 504.1819; found: 504.1813.
Synthesis of 12-(4-(5-phenyl-[1,2,4]triazolo[1,5-a]pyrimidin-7-yl)phenyl)-12H-benzo[a]phenoxazine (5PTP-BPXZ).
5PTP-BPXZ was synthesized in the same way as described for the synthesis of 7PTP-BPXZ. Yield: 69%. 1H NMR (400 MHz, CDCl3) δ (ppm): 8.47 (s, 1H), 8.24–8.16 (m, 2H), 8.03–7.94 (m, 3H), 7.93–7.88 (m, 1H), 7.81 (dd, J = 9.0, 0.7 Hz, 1H), 7.70–7.63 (m, 1H), 7.56–7.41 (m, 7H), 7.30–7.26 (m, 2H), 7.25–7.23 (m, 1H), 7.21–7.14 (m, 2H). 13C NMR (100 MHz, CDCl3) δ (ppm): 161.4, 156.1, 154.1, 151.9, 151.6, 147.7, 136.6, 133.4, 131.2, 131.0, 130.3, 129.5, 129.0, 128.5, 127.7, 127.6, 127.2, 127.0, 126.9, 126.0, 125.1, 123.8, 122.8, 122.6, 118.0, 117.6, 116.8, 105.4. HRMS (ESI): m/z [M + H]+ calcd for C33H22N5O+: 504.1819; found: 504.1813.
Synthesis of 12,12′-([1,2,4]triazolo[1,5-a]pyrimidine-5,7-diylbis(4,1-phenylene))bis(12H-benzo[a]phenoxazine) (5,7PTP-BPXZ).
5,7PTP-BPXZ was synthesized in the same way as described for the synthesis of 7PTP-BPXZ. Yield: 85%. 1H NMR (400 MHz, CDCl3) δ (ppm): 8.39 (s, 1H), 7.99 (d, J = 9.0 Hz, 2H), 7.95–7.85 (m, 6H), 7.78 (t, J = 9.1 Hz, 2H), 7.64–7.62 (m, 2H), 7.52–7.36 (m, 7H), 7.27 (s, 1H), 7.25–7.19 (m, 5H), 7.16–7.11 (m, 4H). 13C NMR (100 MHz, CDCl3) δ (ppm): 160.7, 155.8, 154.1, 153.9, 153.7, 151.8, 151.5, 151.4, 147.3, 133.9, 131.1, 131.0, 130.2, 129.9, 129.5, 129.3, 128.6, 128.4, 128.3, 127.6, 127.4, 127.1, 126.9, 126.8, 126.5, 126.4, 126.2, 126.0, 125.8, 124.9, 123.8, 122.9, 122.8, 118.0, 117.9, 117.8, 117.6, 117.4, 116.8, 104.8. HRMS (ESI): m/z [M + H]+ calcd for C49H31N6O2+: 735.2503; found: 735.2505.
Conflicts of interest
There are no conflicts to declare.
Acknowledgements
This work was supported by grants from the National Natural Science Foundation of China (No. 21721005, 91833304, 21805195 and 51903159), the Shenzhen Science and Technology Program (KQTD20170330110107046) and the Shenzhen Technology and Innovation Commission (JCYJ20180507182244027). The numerical calculations in this paper were carried out on the supercomputing system in the Supercomputing Center of Wuhan University.
Notes and references
-
(a) J. Zhang, A. Li, H. Zou, J. Peng, J. Guo, W. Wu, H. Zhang, J. Zhang, X. Gu, W. Xu, S. Xu, S. H. Liu, A. Qin, J. W. Y. Lam and B. Z. Tang, A simple donor-acceptor AIEgen with multi-stimuli responsive behavior, Mater. Horiz., 2020, 7, 135–142 RSC;
(b) W. Zhao, Z. He, Q. Peng, J. W. Y. Lam, H. Ma, Z. Qiu, Y. Chen, Z. Zhao, Z. Shuai, Y. Dong and B. Z. Tang, Highly sensitive switching of solid-state luminescence by controlling intersystem crossing, Nat. Commun., 2018, 9, 3044–3051 CrossRef PubMed;
(c) K. Zheng, H. Yang, F. Ni, Z. Chen, S. Gong, Z. Lu and C. Yang, Multifunctional thermally activated delayed fluorescence emitters and insight into multicolor-mechanochromism promoted by weak intra- and intermolecular interactions, Adv. Opt. Mater., 2019, 7, 1900727–1900735 CrossRef CAS;
(d) W. Yang, Y. Yang, L. Zhan, K. Zheng, Z. Chen, X. Zeng, S. Gong and C. Yang, Polymorphism-dependent thermally activated delayed fluorescence materials with diverse three dimensional supramolecular frameworks, Chem. Eng. J., 2020, 390, 124626–124634 CrossRef CAS;
(e) B. Roy, M. C. Reddy and P. Hazra, Developing the structure-property relationship to design solid state multi-stimuli responsive materials and their potential applications in different fields, Chem. Sci., 2018, 9, 3592–3606 RSC.
-
(a) G. Huang, Q. Xia, W. Huang, J. Tian, Z. He, B. S. Li and B. Z. Tang, Multiple anti-counterfeiting guarantees from a simple tetraphenylethylene derivative high-contrasted and multi-state mechanochromism and photochromism, Angew. Chem., Int. Ed., 2019, 58, 17814–17819 CrossRef CAS PubMed;
(b) K. Zheng, F. Ni, Z. Chen, C. Zhong and C. Yang, Polymorph-dependent thermally activated delayed fluorescence emitters: Understanding TADF from a perspective of aggregation state, Angew. Chem., Int. Ed., 2019, 58, 1–6 CrossRef;
(c) X. Zheng, W. Zhu, C. Zhang, Y. Zhang, C. Zhong, H. Li, G. Xie, X. Wang and C. Yang, Self-assembly of a highly emissive pure organic imine-based stack for electroluminescence and cell imaging, J. Am. Chem. Soc., 2019, 141, 4704–4710 CrossRef CAS PubMed;
(d) Z. Yang, Z. Chi, Z. Mao, Y. Zhang, S. Liu, M. P. Aldred and Z. Chi, Recent advances in mechano-responsive luminescence of tetraphenylethylene derivatives with aggregation-induced emission properties, Mater. Chem. Front., 2018, 2, 861–890 RSC.
- J. Zhao, Z. Chi, Y. Zhang, Z. Mao, Z. Yang, E. Ubba and Z. Chi, Recent progress in the mechanofluorochromism of cyanoethylene derivatives with aggregation-induced emission, J. Mater. Chem. C, 2018, 6, 6327–6353 RSC.
- J. Luo, Z. Xie, J. W. Y. Lam, L. Cheng, H. Chen, C. Qiu, H. S. Kwok, X. Zhan, Y. Liu, D. Zhu and B. Z. Tang, Aggregation-induced emission of 1-methyl-1,2,3,4,5-pentaphenylsilole, Chem. Commun., 2001, 1740–1741 RSC.
-
(a) D. T. Hogan, B. S. Gelfand, D. M. Spasyuk and T. C. Sutherland, Subtle substitution controls the rainbow chromatic behaviour of multi-stimuli responsive core-expanded pyrenes, Mater. Chem. Front., 2020, 4, 268–276 RSC;
(b) J. Zhu, C. Li, P. Chen, Z. Ma, B. Zou, L. Niu, G. Cui and Q. Yang, A polymorphic fluorescent material with strong solid state emission and multi-stimuli-responsive properties, Mater. Chem. Front., 2020, 4, 176–181 RSC;
(c) B. Huang, X. Gu, Y. Feng, Y. Zhang, D. Jiang, W. C. Chen, G. Dai, Y. Ji, Q. Zhao and C. S. Lee, Solid-state fluorophore based on π-extended heteroaromatic acceptor: Polymorphism, mechanochromic luminescence, and electroluminescence, Cryst. Growth Des., 2020, 20, 2454–2461 CrossRef CAS;
(d) H. Tian, P. Wang, J. Liu, Y. Duan and Y. Q. Dong, Construction of a tetraphenylethene derivative exhibiting high contrast and multicolored emission switching, J. Mater. Chem. C, 2017, 5, 12785–12791 RSC.
- G. Huang, Y. Jiang, S. Yang, B. S. Li and B. Z. Tang, Multistimuli response and polymorphism of a novel tetraphenylethylene derivative, Adv. Funct. Mater., 2019, 29, 1900516–1900524 CrossRef.
- M. Okazaki, Y. Takeda, P. Data, P. Pander, H. Higginbotham, A. P. Monkman and S. Minakata, Thermally activated delayed fluorescent phenothiazine-dibenzo[a,j]phenazine-phenothiazine triads exhibiting tricolor-changing mechanochromic luminescence, Chem. Sci., 2017, 8, 2677–2686 RSC.
- X. Zeng, T. Zhou, J. Liu, K. Wu, S. Li, X. Xiao, Y. Zhang, S. Gong, G. Xie and C. Yang, Incorporating thermally activated delayed fluorescence into mechanochromic luminescent emitters: high-performance solution-processed yellow organic light emitting diodes, Adv. Opt. Mater., 2018, 6, 1801071–1801079 CrossRef.
- M. D. Yang, D. L. Xu, W. G. Xi, L. K. Wang, J. Zheng, J. Huang, J. Y. Zhang, H. P. Zhou, J. Y. Wu and Y. P. Tian, Aggregation-induced fluorescence behavior of triphenylamine-based schiff bases: the combined effect of multiple forces, J. Org. Chem., 2013, 78, 10344–10359 CrossRef CAS PubMed.
-
(a) W. Yang, C. Liu, S. Lu, J. Du, Q. Gao, R. Zhang, Y. Liu and C. Yang, AIE-active smart cyanostyrene luminogens: polymorphism-dependent multicolor mechanochromism, J. Mater. Chem. C, 2018, 6, 290–298 RSC;
(b) Y. Li, Z. Ma, A. Li, W. Xu, Y. Wang, H. Jiang, K. Wang, Y. Zhao and X. Jia, A single crystal with multiple functions of optical waveguide, aggregation-induced emission, and mechanochromism, ACS Appl. Mater. Interfaces, 2017, 9, 8910–8918 CrossRef CAS PubMed;
(c) J. Mei, N. L. C. Leung, R. T. K. Kwok, J. W. Y. Lam and B. Z. Tang, Aggregation-induced emission: together we shine, united we soar, Chem. Rev., 2015, 115, 11718–11940 CrossRef CAS PubMed;
(d) L. Pan, Y. Cai, H. Wu, F. Zhou, A. Qin, Z. Wang and B. Z. Tang, Tetraphenylpyrazine-based luminogens with full-colour emission, Mater. Chem. Front., 2018, 2, 1310–1316 RSC.
Footnote |
† Electronic supplementary information (ESI) available: Synthesis and additional figure details. CCDC 1994907–1994909. For ESI and crystallographic data in CIF or other electronic format see DOI: 10.1039/d0qm00247j |
|
This journal is © the Partner Organisations 2020 |
Click here to see how this site uses Cookies. View our privacy policy here.